- 1Section of Biology and Genetics Giovanni Sichel, Department of Biomedical and Biotechnological Sciences, University of Catania, Catania, Italy
- 2Section of Child and Adolescent Psychiatry, Department of Clinical and Experimental Medicine, University of Catania, Catania, Italy
- 3Radiology Unit 1, Department of Medical Surgical Sciences and Advanced Technologies, University Hospital “Policlinico-Vittorio Emanuele”, University of Catania, Catania, Italy
- 4Oasi Research Institute–IRCCS, Troina, Italy
Due to its rarity, coupled to a multifactorial and very heterogeneous nature, the molecular etiology of Arnold-Chiari (AC) syndrome remains almost totally unknown. Its relationship with other neuropsychiatric disorders such as Tourette syndrome (TS) is also undetermined. The rare comorbid status between both disorders (ACTS) complicates the framework of diagnosis and negatively affects the patients' quality of life. In this exploratory study, we aimed to identify serum microRNA expression profiles as molecular fingerprints for AC, TS, and ACTS, by using a high-throughput approach. For this aim, 10 AC patients, 11 ACTS patients, 6 TS patients, and 8 unaffected controls (NC) were recruited. Nine miRNAs resulted significantly differentially expressed (DE): let-7b-5p (upregulated in ACTS vs. TS); miR-21-5p (upregulated in ACTS vs. AC; downregulated in AC vs. TS); miR-23a-3p (upregulated in TS vs. NCs; downregulated in AC vs. TS); miR-25-3p (upregulated in AC vs. TS and NCs; downregulated in ACTS vs. AC); miR-93-5p (upregulated in AC vs. TS); miR-130a-3p (downregulated in ACTS and TS vs. NCs); miR-144-3p (downregulated in ACTS vs. AC; upregulated in AC vs. TS); miR-222-3p (upregulated in ACTS vs. NCs); miR-451a (upregulated in AC vs. TS and NCs; in ACTS vs. NCs). Altered expression of miRNAs was statistically correlated to neuroimaging and neuropsychological anomalies. Furthermore, computational analyses indicated that DE miRNAs are involved in AC and TS pathomechanisms. Finally, we propose the dysregulation of the miRNA set as a potential molecular tool for supporting the current diagnosis of AC, TS, and ACTS by using liquid biopsies, in an unbiased and non-invasive way.
Introduction
Arnold-Chiari syndrome (AC) is a group of four types of anomalies sharing a malformation of the cerebellum and brainstem. In particular, Chiari malformation type I (CM-I) is characterized by caudal displacement of cerebellar tonsils, which can either be both or singularly herniated, 3 or 5 mm, respectively, below the foramen magnum (FM) (Chiari, 1987; George and Higginbotham, 2011; Urbizu et al., 2013; Markunas et al., 2014).
CM-I is the most common type of anomaly affecting from 1/5,000 to 1/1,000 individuals, affecting females more than males (1.3–1) (Urbizu et al., 2013). Currently, diagnosis is based on symptom evaluation (occipital headaches, ocular disturbances, vertigo, weakness, dysphagia or dysarthria), together with a cranial midsagittal magnetic resonance (MR) analysis (Epstein, 2018). Thanks to the MR analysis, it is possible to detect the degree of cerebellar tonsil herniation not only in patients who show typical debilitating neurologic symptoms, but often also incidentally in those patients who are asymptomatic (Urbizu et al., 2013; Markunas et al., 2014; Epstein, 2018).
Although the etiology of CM-I still remains unclarified and multifactorial, different pathogenic mechanisms have been proposed to define the critical factors causing it (Milhorat et al., 2010; Shoja et al., 2018). Among these, the most accepted consists in a developmental defect of paraxial mesoderm and occipital somites, which leads to a volumetrically-reduced posterior cranial fossa (PCF), a main feature of CMI (Marin-Padilla, 1991; Langridge et al., 2017). The small size of the PCF is unable to hold a normal hindbrain, resulting in tonsillar herniation and an altered hydrodynamic of cerebrospinal fluid (CSF) flow (Barry et al., 1957; Marin-Padilla and Marin-Padilla, 1981; Milhorat et al., 2010; Shaffer et al., 2011).
Furthermore, a genetic basis has been suggested to contribute to CM-I pathogenesis (Speer et al., 2000, 2003). In particular, Urbizu et al. performed a case-control association study on CM-I patients, revealing significant associations with four SNPs within ALDH1A2, CDX1, and FLT1 genes involved in somitogenesis and fetal vascular development (Urbizu et al., 2013).
Several neuropsychiatric disorders may coexist with AC condition (Lacy et al., 2018): anxiety (Caykoylu et al., 2008), epilepsy (Granata and Valentini, 2011), intellectual disability (Grosso et al., 2001), attention deficit hyperactivity disorder (ADHD) and, less recognized, autism spectrum disorders (ASD) (Jayarao et al., 2015; Osuagwu et al., 2016), thus worsening the patients' quality of life (Bakim et al., 2013). Interestingly, AC malformations have been reported to be part of secondary tics and tourettism causes (Mejia and Jankovic, 2005).
TS is characterized by multiple involuntary motor and vocal tics, as well as functional and behavioral impairment (Rizzo et al., 2014, 2015; Cirnigliaro et al., 2017; Efron and Dale, 2018). Diagnosis is based on clinical criteria evaluated according to the Diagnostic and Statistical Manual of Mental Disorders (DSM-V), which requires the presence of two or more motor tics and at least one vocal tic, occurring at least once a day and for over 1 year since onset (Ganos and Martino, 2015; Rizzo et al., 2015). TS prevalence is estimated to be ~1% of the population, with a male:female ratio of 4:1. The onset occurs typically in childhood (Robertson, 2015) and in most cases patients improve by late adolescence (Ganos and Martino, 2015).
It has been revealed that up to 85% of children affected by TS present various comorbid neurobehavioural problems (e.g., ADHD, obsessive-compulsive disorder, anxiety, ASD, learning, and sleep disorders), which cause worse functional outcomes (Rizzo et al., 2014; Cirnigliaro et al., 2017; Efron and Dale, 2018). TS pathogenesis is not completely clear, but evidence suggests that complex genetic factors, together with environmental factors, are involved. Susceptibility genes such as SLITRK1, IMMP2L, CNTNAP2, NLGN4X, LIM homeobox (LHX6, LHX8), HDC, and FLT3 have been identified as associated with TS (Verkerk et al., 2003; Lawson-Yuen et al., 2008; Karagiannidis et al., 2012; Paschou et al., 2012; Baldan et al., 2014; Bertelsen et al., 2014; Yu et al., 2019).
Delineating neuro-psychiatric disorders is still challenging because of the diagnosis complexity and heterogeneity, especially in comorbid conditions. A molecular signature may pave the way to strengthen the traditional approaches for clinical discrimination of TS, AC, and ACTS patients, who show a co-existence of both pathologies.
Over the past few years microRNAs (miRNAs) have attracted increasing attention for several reasons. MiRNAs are small non-coding RNAs, ranging from 18 to 25 nucleotides, which negatively regulate gene expression through post-transcriptional mechanisms. Numerous studies have shown that miRNAs affect cellular pathways and biological processes (Kim and Nam, 2006; Ragusa et al., 2017; O'Brien et al., 2018; Treiber et al., 2019), also playing an essential role in many aspects of neural development (e.g., neurogenesis, neuronal differentiation, maturation, synaptogenesis, neuronal plasticity, neuronal apoptosis) (Bian and Sun, 2011). Furthermore, miRNAs show specific expression and spatiotemporal patterns in mammalian central nervous systems (CNS) (Kosik, 2006; Rao et al., 2013); alterations of miRNA expression have been associated with various brain anomalies and neuropsychiatric disorders (Xu et al., 2010, 2012; Mundalil Vasu et al., 2014; Wu et al., 2015; Hicks et al., 2016, 2019; Alural et al., 2017; Cirnigliaro et al., 2017; Kichukova et al., 2017; Nt et al., 2018; Zadehbagheri et al., 2019), delineating a discriminative molecular signature (Salta and De Strooper, 2012). Furthermore, the evidence that extracellular RNA can be detected in all mammalian body fluids, including peripheral blood (Kosaka et al., 2010; Turchinovich et al., 2011, 2013), opened the way to an important source of biomarkers (Rao et al., 2013; Kichukova et al., 2015; Rizzo et al., 2015): this finding revealed the possibility to analyze the expression of circulating miRNAs (cmiRNAs) through liquid biopsies, in a minimally invasive way (Rao et al., 2013; Nevel et al., 2018). On the other hand, this may objectively support the current diagnosis strategies, unveiling evasive cases that are otherwise not easily detectable.
All these findings underline the importance of using cmiRNAs as a new promising tool in the diagnostic management of Arnold-Chiari Syndrome, Tourette syndrome and their comorbid conditions. To date, only few studies have focused on cmiRNAs in the serum of patients with pure TS and in TS comorbid with ASD (Rizzo et al., 2015; Cirnigliaro et al., 2017), while, to our knowledge, no one has characterized cmiRNAs in AC or AC comorbid with TS.
Based on these premises, we exploited a high-throughput approach to investigate cmiRNA expression profiles in sera from AC, TS, and ACTS patients compared to each other and to unaffected controls (NCs), aiming to identify a differential expression among groups. We then evaluated the existence of a molecular relationship between serum miRNA profiles and the scores clinically assigned through a neuropsychological and neuroimaging assessment. Finally, through pathway enrichment analyses, we explored the biological functions of the differentially expressed miRNAs, and thus their potential etiological role in AC, ACTS, and TS pathogenesis.
Materials and Methods
Ethics Approval and Consent to Participate
All experiments were approved by the local ethical committee “Comitato Etico Catania 1” (ID: 0024-36-19) prior to sample collection and in accordance with the Helsinki Declaration and its later amendments or comparable ethical standards. Written, informed consent was obtained from parents of all minor age participants (range age 12–13).
Patient Selection
Twenty-seven Caucasian patients were consecutively recruited from July 2017 to December 2018 at the Section of Child and Adolescent Psychiatry (Department of Clinical and Experimental Medicine, University of Catania).
Patients were affected by AC (n = 10), ACTS (n = 11), and TS (n = 6). They were studied and compared to NCs recruited from local schools (n = 8).
Inclusion Criteria
Patients who were included in the study fulfilled the following criteria for each clinical group:
(i) AC group: presence of Arnold Chiari malformation type I, defined by a cerebellar tonsillar position (TP) >3–5 mm below the FM; absence of other neurological or metabolic conditions; absence of movement disorders and comorbid conditions such as obsessive-compulsive disorder (OCD) and/or attention deficit/ hyperactivity disorder.
(ii) ACTS group: clinical diagnosis of Tourette Syndrome, according to the criteria of the Diagnostic and Statistical Manual of Mental Disorders, Fifth Edition (DSM-5, APA 2013); the presence of Arnold Chiari type I malformation is based on brain magnetic resonance imaging (MRI): this malformation is defined by cerebellar TP >3–5 mm below the FM.
(iii) TS group: clinical diagnosis of Tourette Syndrome, according to the criteria of the Diagnostic and Statistical Manual of Mental Disorders, Fifth Edition (DSM-5, APA 2013); the absence of other neurological or metabolic conditions such as AC.
Unaffected controls (NCs) were considered neurologically intact children and adolescents, without any history of movement disorder and normal position of cerebellar tonsillar and without chronic neurological, psychiatric, metabolic or genetic diseases. To avoid confounding factors, we did not include either children with minor neuropsychiatric disease (e.g., language delay/disorders or transient tic disorders that did not fulfill criteria for TS) or with minor neurological signs.
Clinical and Neuroimaging Assessment
A medical history was obtained from all participants and their parents with a focus on neurological and psychiatric conditions. Moreover, all participants underwent a physical and neurological examination and weight, height, head circumference were measured. Venous blood samples were collected. Patients and controls underwent a brain MRI with measurements of posterior fossa (PF) to evaluate the position of cerebellar tonsils.
T1 weighted sagittal brain MRI images were used. All measurements were taken for right and left herniation. Particular attention was paid to the measurements of the following areas: upper posterior cranial fossa, lower posterior cranial fossa, and total posterior cranial fossa; finally, basal, Boogaard, occipital, and tentorial angles were measured (Markunas et al., 2014).
Neuropsychological Assessment
All participants (AC, ACTS, TS patients and NCs) were assessed by child and adolescent neurologists and psychiatrists (RR; MG) with the following instruments:
(I) Wechsler scale (WISC-III), applied to evaluate Intelligence Quotient (IQ), is a test used to evaluate the intellectual ability of children ranging from 6 to 16 years. To this aim, the assessment provides full-scale intelligence quotient (IQ) scores, as well as Performance IQ and Verbal IQ, two secondary scores (Koyama et al., 2008).
(II) The Yale Global Tic Severity Scale (YGTSS) is an 11-item clinician-rated interview, which is able to evaluate motor and phonic tic severity, considering not only the number and frequency, but also the impairment that tics provoke in the patient. The score of the YGTSS is 0–100, including the impairment section. Higher scores indicate higher severity of symptoms and impairment (Leckman et al., 1989).
(III) The Children Yale Brown Obsessive Compulsive Scale (CY-BOCS) is a semi-structured interview, conducted principally with parents, even if patients are encouraged to participate. This interview is able to assess the severity of obsessive-compulsive symptoms in children. The total score of CY-BOCS ranges between 0 and 40. It is possible to evaluate an obsession and a compulsion score separately. Again, higher scores indicate higher severity of symptoms and impairment (Scahill et al., 1997).
(IV) The Conners' Comprehensive Behavior Rating Scales (Conners) is a questionnaire that provides an overview of child and adolescent impairments. Conners is a multi-informant assessment of children and adolescents across multiple settings, with rating forms for parents, teachers, and patients. It is a validated, self- and proxy-rated (parent, teacher) scale used with 12–18-year-olds. It is used to diagnose ADHD and can allow discrimination between subtypes (e.g., predominantly inattentive/ hyperactive-impulsive) (Gianarris et al., 2001).
(V) The Child Behavior Checklist (CBCL) is a report form, which evaluates behavioral problems in children. The CBCL is a validated, parent-rated scale assessing the frequency and intensity of behavioral and emotional difficulties shown by a child over the preceding 6 months. It contains eight syndrome scales (withdrawn, somatic complaints, anxious/depressed, social problems, thought problems, attention problems, delinquent behavior, and aggressive behavior) and two composite scales (externalizing and internalizing problems) (Achenbach and Rescorla, 2001).
Sample Collection and Processing
Peripheral blood samples from all participants were collected in the morning through a butterfly device, inserted into serum separation collection tubes with Clot activator and gel for serum separation (BD Biosciences). Collection tubes were treated according to current procedures for clinical samples. Tubes were rotated end-over-end at 20°C for 30′ to separate serum from blood cells. Subsequently, they were centrifuged at 3,500 rpm at 4°C for 15′ in a Beckman J-6M/E, supernatants were distributed into 1.5 ml RNase-free tubes, and finally stored at −80°C until analysis (Rizzo et al., 2015).
RNA Extraction
Extraction of RNA was carried out from 800 μl of serum samples by using a Qiagen miRNeasy Mini Kit (Qiagen, GmbH, Hilden, Germany), according to Qiagen Supplementary Protocol for purification of total RNA (including small RNAs) from serum and plasma (Rizzo et al., 2015). RNA was eluted in 200 μl RNAse-free water and then precipitated by adding 20 μg glycogen, 0.1 volume of 3 M sodium acetate and 2.5 volumes of ice-cold 100% ethanol. After incubation at −80°C overnight, RNA was centrifuged, washed twice in ice-cold 75% ethanol and resuspended in 7 μl RNAse-free water. The yield and quality of the RNA samples were assessed by using NanoDrop Lite Spectrophotometer (Thermo Fisher Scientific, Wilmington, DE, USA).
MiRNA Profiling
Circulating miRNA expression profiling from serum was performed through the NanoString nCounter system assays by using nCounter Human v3 miRNA Expression Assay Kits (NanoString Technologies, Seattle, USA) and the NanoString platform, according to the manufacturer's instructions. MiRNA profiling was performed on 3 μl (~150 ng) of isolated RNA of 10 AC, 11 ACTS, 6 TS patients, and 8 NCs. RNA samples were processed and immobilized in a sample cartridge for quantification and data collection by using the nCounter Prep Station and Digital Analyzer, respectively. Data analysis was performed using nSolver 3.0 software. Quality of raw data was assessed through an evaluation of imaging, binding density, positive control linearity, and positive control limit of detection parameters. A code-set normalization was applied to minimize technical noise (variations in purification, binding, hybridization efficiency), according to the nSolver analysis software protocol. For each comparison, SAM (Significance of Microarrays Analysis) statistical analyses were computed with MeV (Multi experiment viewer v4.8.1) statistical analysis software. A two-class unpaired test, based on 100 permutations and with a False Discovery Rate (FDR) < 0.05, was computed. We obtained fold change (FC) values, calculating the ratio between the normalized count mean of each group. The data discussed in this publication have been deposited in NCBI's Gene Expression Omnibus (Edgar et al., 2002) and are accessible through GEO Series accession number GSE146509 (https://www.ncbi.nlm.nih.gov/geo/query/acc.cgi?acc=GSE146509).
Correlation Analysis
In order to investigate whether a linear relationship existed, correlation analyses were computed between the normalized counts of serum miRNAs and the neuropsychological and neurological scores of the study participants for each comparison.
The neuropsychological parameters chosen for this analysis were: total intelligence quotient (TIQ); verbal intelligence quotient (VIQ); performance intelligence quotient (PIQ); Total YGTSS; motor and phonic Yale Global Tic Severity Scale separately; Total CYBOCS; Children's Yale Brown Obsessive Compulsive Scale for obsession and compulsion, separately; Conners scale; Child Behavior Check list internalizing (CBCL-Int); Child Behavior Check list externalizing (CBCL-Ext); total Child Behavior Check list (Total CBCL). Neurological parameters referred to measurements of right and left protrusions (mm), upper posterior cranial fossa area, lower posterior cranial fossa area, total posterior cranial fossa and basal area, Boogaard, occipital, and tentorial angles.
Correlation analyses were performed by GraphPad Prism v8.01 (GraphPad Software, La Jolla, California, USA). Since data were not distributed normally, the Spearman's test was used, applying two-sided p-values. Statistical significance was established at p ≤ 0.05.
Computational Analysis
To investigate the biological functions of all the DE miRNAs and their potential etiological involvement in AC, ACTS, and TS, the DIANA-mirPath v.3 web server (Vlachos et al., 2015) and miRNet (Fan et al., 2016) tool were used for functional enrichment analyses from KEGG (Kyoto Encyclopedia of Genes and Genomes) and Reactome gene annotation databases. A class-specific functional enrichment analysis was performed to separately consider miRNAs that were found DE for each pathological condition with respect to the NC group. Fisher's exact t-test with FDR correction and Hypergeometric test (p ≤ 0.05) were used for enrichment analysis.
Results
Demographics
We recruited a total of 35 children from various socio-economic contexts; 10 affected by Arnold Chiari Syndrome (AC) (M:F = 6:4), mean age 13.1 (±3.1) (age range 9–17); 11 affected by Arnold Chiari Syndrome and comorbid Tourette Syndrome (ACTS) (M:F = 9:2), mean age 12.3 (±2.7) (age range 8–16 years); 6 affected by Tourette Syndrome (TS) (M:F = 6:0), mean age 13.8 (±2) (age range 11–16); 8 unaffected controls (NCs) (M:F = 8:0), mean age 12.6 (±2.6) (age range 9–16 years), recruited from local schools. Demographic and neuropsychological characteristics of the clinical sample are shown in Table 1.
Neuropsychological Findings
(i) Intelligent quotient: with regards to the intelligence quotients (Total, Verbal, and Performance), measured with Wechsler scales, the TS group showed the highest mean value; no statistically significant differences were found in the comparison between clinical groups, except AC vs. TS (p-value = 0.005).
(ii) Tourette Syndrome: with regards to tic evaluation, performed with YGTSS, we found scores equal to zero in the AC group and in the normal control group: this could be explained by the exclusion of patients with a tic who did not fulfill criteria for TS to avoid confounding factors (see section Inclusion). TS presented higher scores compared to ACTS, but these differences were not statistically significant in any subscale (motor, vocal, total).
(iii) Obsessive compulsive disorder: with regards to the evaluation of obsessions and compulsions, performed by CYBOCS, AC, and NC presented statistically significant lower scores compared to TS and ACTS. These scores are not able to identify, by themselves, an obsessive-compulsive disorder, but could be the expression of obsessive and/or compulsive behavior that does not interfere with the normal life of the patient. Comparing TS and ACTS groups, TS presented the higher scores, and these scores are identifiable with OCD disorder. No statistically significant differences were found, even if the disorder in ACTS is less severe than in TS.
(iv) Behavioral Problems: with regards to behavioral problems measured with CBCL and Conners' scales, we found the following results: ACTS presented higher scores (total, internalizing, and externalizing), but no statistically significant differences were found with the TS score group. The scores presented in the AC and NC groups were statistically significantly lower compared to the other clinical groups.
Finally, with regards to the Conners' score, the TS group presented the highest statistically significant score compared with the other clinical groups and the NC group. In the comparison between AC and ACTS, no statistically significant differences were found; on the other hand, the score of the NC group was statistically significantly lower compared to the three clinical groups.
Neuroimaging Measurements
Patients and unaffected controls underwent MRI and all the measurements described in methods section were performed. All measurement details are reported in Table 2. By applying 2-tailed Student t tests, statistically significant differences were found between the areas of total PCF between ACTS and NC (p-value = 0.0053); AC and TS (p-value = 0.0316); AC and NC (p-value = 0.0053) groups. No statistically significant differences were found in all the other comparisons.
Circulating miRNA Expression Profiling
A high-throughput expression analysis of 800 microRNAs in sera of 10 AC, 11 ACTS, 6 TS patients, and 8 NC subjects was computed by using nCounter NanoString technology.
We identified 9 miRNAs as significantly differentially expressed in sera from the different groups (Table 3). More specifically: (1) let-7b-5p upregulated in ACTS compared to TS patients; (2) miR-21-5p upregulated in ACTS compared to AC patients and was downregulated in AC compared to TS patients; (3) miR-23a-3p was upregulated in TS compared to NCs and was downregulated in AC compared to TS patients; (4) miR-25-3p was upregulated in AC compared to TS patients and NCs and was downregulated in ACTS compared to AC patients; (5) miR-93-5p was upregulated in AC compared to TS patients; (6) miR-130a-3p was downregulated in ACTS and TS patients compared to NCs; (7) miR-144-3p was downregulated in ACTS compared to AC patients and upregulated in AC compared to TS patients; (8) miR-222-3p was upregulated in ACTS compared to NCs; (9) miR-451a was upregulated in AC compared to TS patients and NCs, and in ACTS patients compared to NCs, with a FDR < 0.05 for each pairwise comparison. The relative expression of DE miRNAs is shown in Figure 1.
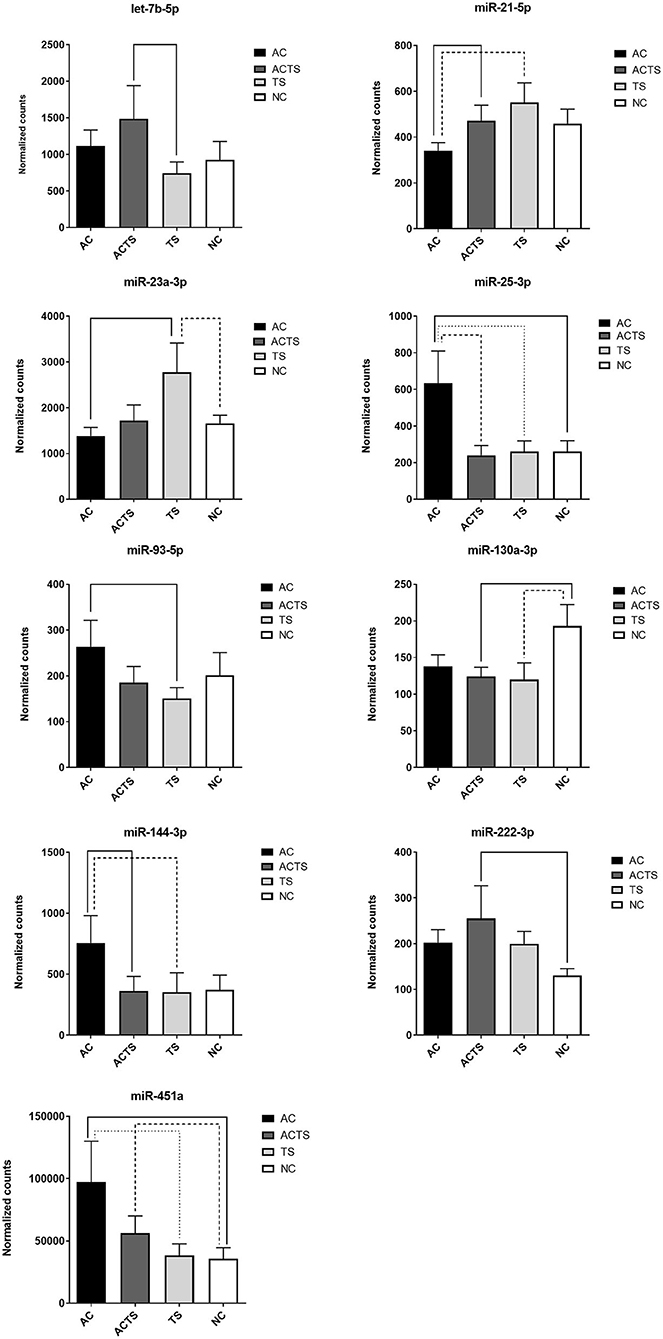
Figure 1. DE-miRNA expression in the serum of AC, TS, ACTS, and NC subjects. Bar plots of the relative expression of the 9 miRNAs for each pairwise comparison: let-7b-5p, miR-21-5p, miR-23a-3p, miR-25-3p, miR-93-5p, miR-130a-3p, miR-144-3p, miR-222-3p, and miR-451a. Each pair whose miRNA dysregulation was found statistically significant (FDR< 0.05) is linked by a continuous or dotted line. Data are shown as means with the standard error of the mean (SEM). Y-axis represents the means of normalized counts with standard error.
Correlation Analysis Between Serum miRNA Expression Levels and Neuropsychological, Neurological Parameters
Using a Spearman correlation analysis and applying two-sided p-values, the normalized counts of serum miRNAs were correlated with neuropsychological and neurological scores for each comparison (Figure 2).
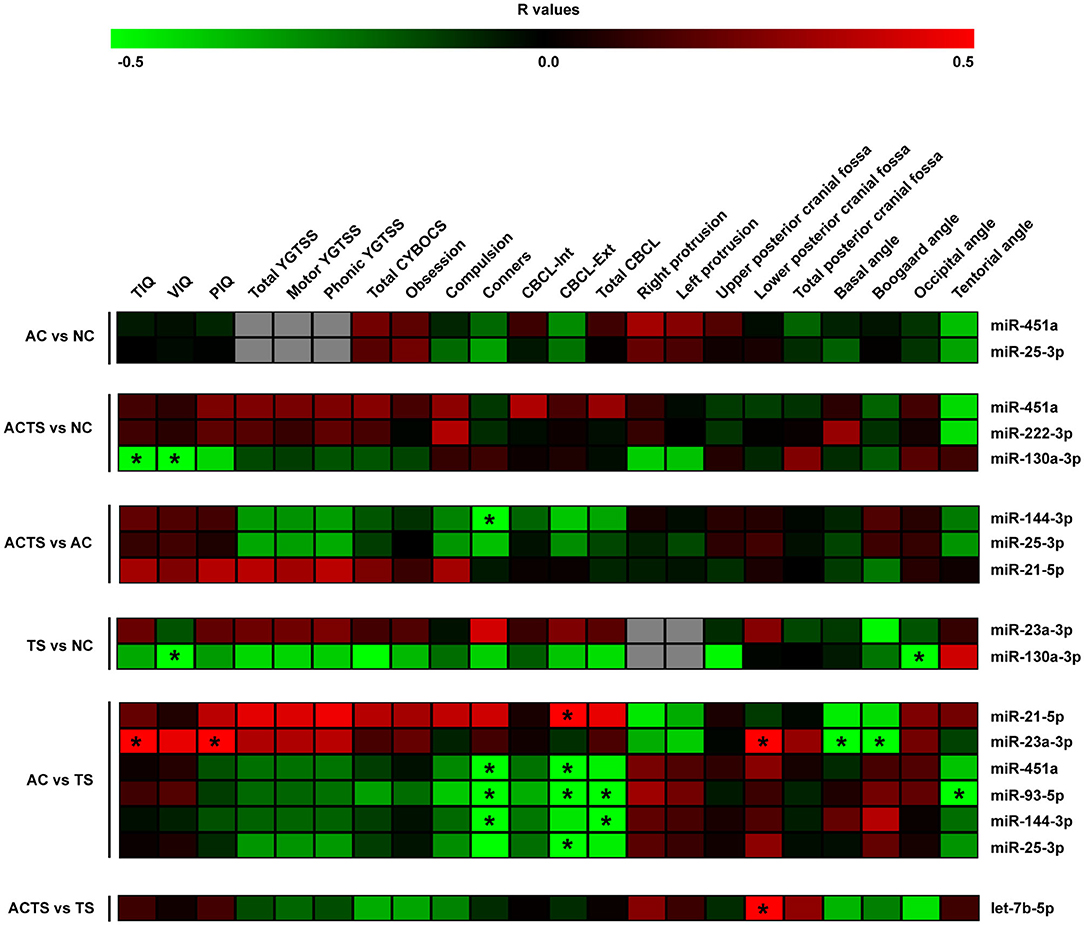
Figure 2. Correlation analysis of serum miRNA expression, cognitive impairments and neuroimaging measurements. Heat-maps of the correlations obtained by calculating Spearman correlation coefficients for miRNA expression, cognitive impairments, and neuroimaging measurements of the assessed participants and for each group of comparison. The correlation coefficient is indicated by a color gradient from green (negative correlation) to red (positive correlation), as shown in the colored bar. Statistically significant p-values (p < 0.05) are indicated by asterisks. TIQ, Total Intelligence Quotient; VIQ, Verbal Intelligence Quotient; PIQ, performance Intelligence Quotient; YGTSS, Yale Global Tic Severity Scale; Total CYBOCS, total Children Yale Brown Obsessive Compulsive Scale; CBCL-Int, Child Behavior Check list internalizing; CBCL-Ext, Child Behavior Check list externalizing; Total CBCL, total Child Behavior Check list.
By comparing ACTS and NC groups, we found a negative relationship between intelligent quotient scores (e.g., TIQ and VIQ) and miR-130a-3p (r = −0.49, r = −0.53, respectively), as well as between VIQ and miR-130a-3p (r = −0.65) by comparing TS and NC groups and a positive correlation between TIQ, PIQ and miR-23a-3p (r = 0.57, r = 0.54, respectively), in AC vs. TS comparison. We also found a negative relationship between Conners' scale and (i) miR-144-3p (r = −0.5) by comparing ACTS and AC groups and (ii) miR-451a (r = −0.8), miR-93-5p (r = −0.79), miR-144-3p (r = −0.79) comparing AC and TS groups. CBCL-ext scores were found positively correlated with miR-21-5p levels (r = 0.51) and negatively correlated with miR-451a (r = −0.52), miR-93-5p (r = −0.66), miR-25-3p (r = −0.50) in AC vs. TS comparison. From the same comparison, total CBCL scores were found negatively correlated with miR-93-5p (r = −0.67) and miR-144-3p (r = −0.50). On the other hand, neuroimaging measurements (e.g., lower posterior cranial fossa) were found positively correlated with miR-23a-3p (r = 0.63) by comparing AC and TS groups and let-7b-p (r = 0.53) by comparing ACTS vs. TS groups. From the same comparison, we found a negative correlation between Basal and Boogard angle measurements and miR-23a-3p (r = −0.66, r = −0.64, respectively). Finally, we found negative correlations between occipital angle measurements and miR-130a-3p (r = −0.56) by comparing TS vs. NC groups; tentorial angle and miR-93-5p (r = −0.63) by comparing AC vs. TS groups.
Functional Enrichment Analyses
In order to evaluate the potential biological impact related to the differential expression of all the miRNAs identified in this study, we computed pathway enrichment analyses. This analysis showed a list of statistically over-represented biological pathways (Figure 3), potentially related to the analyzed clinical conditions. Supplementary Figures 1–3 report all the data from the class-specific functional enrichment analysis, including those miRNAs found DE for each pathological condition with respect to the NC group.
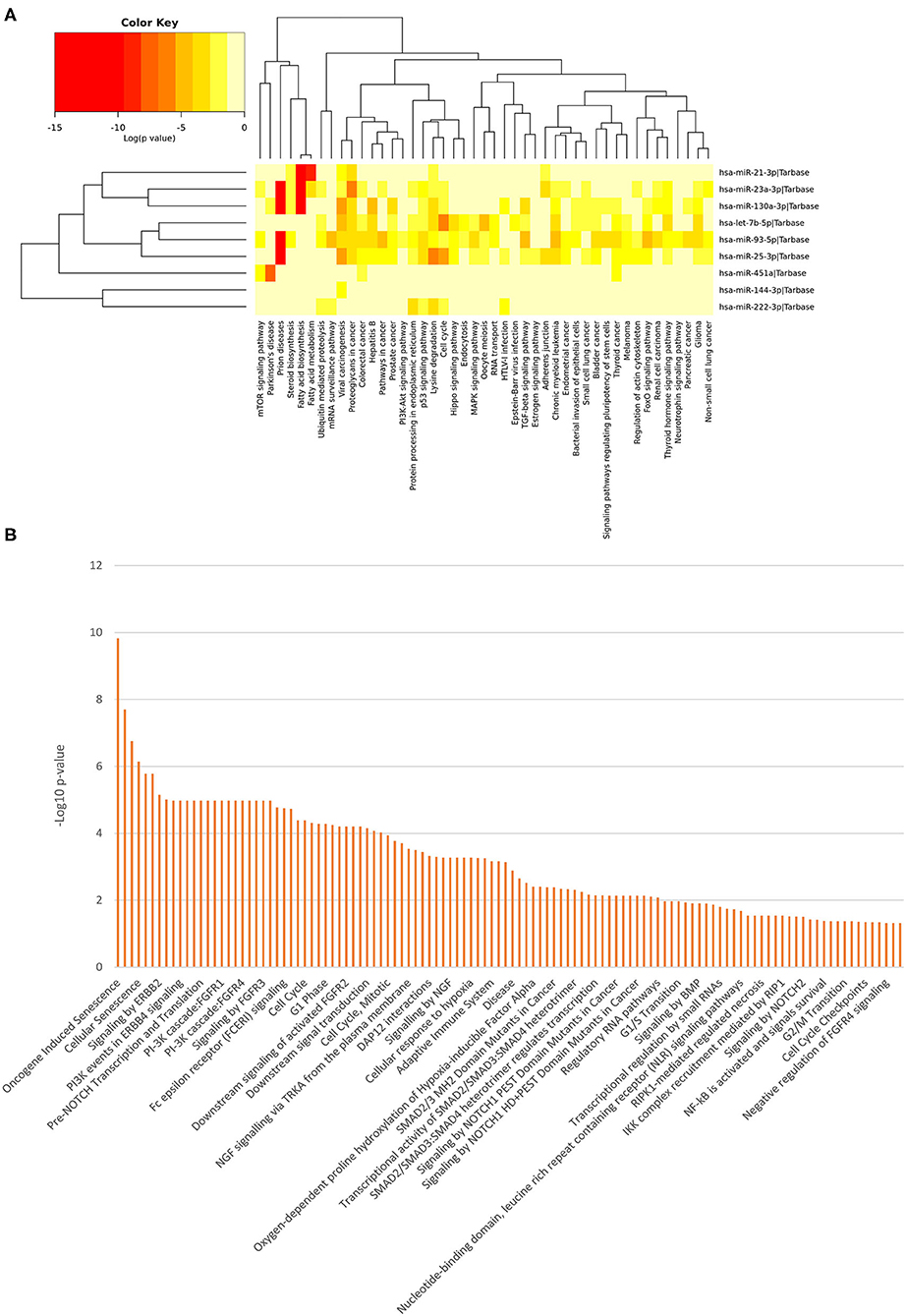
Figure 3. Functional Enrichment analysis of DE miRNAs. Functional enrichment analysis of all DE miRNA targets using KEGG pathway (hierarchical clustering based on a complete linkage method and the significance levels of the interactions) (A) and Reactome databases (B) by DIANA-mirPath v.3 web server and the miRNet tool, respectively.
Among the most interesting terms, we found signaling by NOTCH (p = 0.00000166), pre-NOTCH expression and processing signaling pathway (p = 0.0000106), NGF signaling via TRKA from the plasma membrane (p = 0.000293), signaling by NGF (p = 0.00053), NOTCH1 intracellular domain regulated transcription (p = 0.000551), signaling by NOTCH1 (p = 0.00391), constitutive signaling by NOTCH1 HD+PEST Domain Mutants (p = 0.00729), signaling by NOTCH2 (p = 0.0315), and signaling by Wnt (p = 0.0108), through which neuronal function and development are regulated. Moreover, we found pathways that mediate fear conditioning and behavior signaling by ERBB2 (p = 0.00000711) and ERBB4 (p = 0.0000106), also involved in the development of neurodegenerative diseases. Additionally, the PI3K/AKT activation pathway (p = 0.0000169) was also pinpointed, which is a major regulator of neuron survival, as well as FGFR1/2/3/4 (p = 0.0000106), signaling by FGFR1/2/3/4 (p = 0.0000106), downstream signaling of activated FGFR1/2/3/4 (p = 0.0000624), FoxO signaling pathway (p = 3.22E-07), Hippo signaling pathway (p = 1.58E-05), neurotrophin signaling pathway (p = 0.04106071) and recycling pathway of L1 (p = 0.0187), involved in neural development, including axon outgrowth and neuronal migration.
Other molecular signaling pathways were revealed, such as signaling by BMP (p = 0.0123), Ca2+ pathway (p = 0.0438), TGF-beta receptor signaling activates SMADs (p = 0.00053) and transcriptional activity of SMAD2/SMAD3:SMAD4 heterotrimer (p = 0.00491). These pathways are intriguing since they are related to disorders in bone tissue, muscle contraction, embryonic skeletal development, postnatal bone homeostasis dorsoventral polarity, which analyzed together suggest that the differential expression of these miRNAs could cause the developmental impairments leading to AC pathogenesis.
Discussion
Chiari Type I malformation and Tourette Syndrome are heterogeneous disorders with a high degree of clinical variability and an elusive pathophysiology (Markunas et al., 2014; Cavanna, 2018; Singer and Augustine, 2019).
The complexity of phenotypes and their diagnostic recognition increases in comorbid conditions with other psychopathologies: it is unclear whether or not they all contribute to the genetic expression, or whether some etiological pathways components may be shared, as well as how the comorbid disorders influence each other (Robertson, 2000). To date, a coexistence of both AC and TS in the same individual (i.e., ACTS) has not been described. For this reason, in our study we designed to assess patients affected by AC, TS, NCs and comorbid ACTS, through standardized approaches for clinical diagnostic examinations (i.e., MRI and DSM-5, APA 2013).
Moreover, delineating neuropsychiatric disorders like TS is still challenging and needs potential molecular biomarkers, considering the etiological and clinical heterogeneity, the lack of an unbiased diagnostic test and no existing associations with any neuroimaging abnormality (Robertson, 2000; Cavanna, 2018).
Therefore, we hypothesized that serum profiles of circulating miRNAs may contain molecular fingerprints for AC, TS, and ACTS, which could support the clinical discrimination process.
MiRNAs play pivotal roles in the regulation of complex networks and biological pathways. Their deregulated expression is associated with disease pathogenesis, including neuropsychiatric disorders (Alural et al., 2017). Analyzing miRNA expression profiles in body fluids could reflect the real pathophysiologic processes of patients through very simple and non-invasive approaches, since brain tissue is not easily accessible (Kichukova et al., 2015).
Despite many studies investigating whether miRNA might contain specific fingerprints for neurological and psychiatric disorders (Fineberg et al., 2009; Xu et al., 2012; De Sena Cortabitarte et al., 2018; Nguyen et al., 2018; Tonacci et al., 2019), only a few included Tourette syndrome (Rizzo et al., 2015; Cirnigliaro et al., 2017) and none is focused on AC and ACTS.
Through a high-throughput approach, we investigated cmiRNA expression in the sera of 10 AC, 11 ACTS, 6 TS, and 8 unaffected (NC) subjects. Results showed the identification of nine serum miRNAs as significantly differentially expressed in different groups of comparison (Figure 1). Interestingly, we observed that DE miRNAs in comparisons among ACTS, AC, and TS groups (i.e., miR-451a, miR-21, miR-25-3p, miR-144-3p, 5p) showed a marked different expression trend between AC and ACTS groups, unlike that between ACTS and TS (Figure 1). This observation suggests that miRNA expression could be mostly affected by the typical TS impairments and physiological conditions, when in comorbid conditions. Further investigations are needed to evaluate this hypothesis.
The nine DE miRNAs identified in this study, had been previously identified as dysregulated in cellular and extra-cellular frameworks of other neurological and/or bone disorders, suggesting a critical role in developing cognitive functions and bone morphogenesis (Gao et al., 2011; Mor et al., 2015; Xu et al., 2015; Hicks and Middleton, 2016; Hicks et al., 2016; Yan et al., 2016; Cirnigliaro et al., 2017; Fujiwara et al., 2017; Zhang et al., 2017; Yang et al., 2019).
Molecular pathways identified in our functional enrichment analysis would support the involvement of these miRNAs in AC and TS mechanisms. Among these, the Wnt pathway could be associated with AC pathogenesis by affecting the bone homeostasis and bone remodeling process (Monroe et al., 2012). Wnt pathway is also involved in a crosstalk with Bone Morphogenetic Protein (BMP) and TGF-β signaling during osteoblast and chondrocyte differentiation (Wu et al., 2016). As key regulators of TGFβ-induced chondrogenesis of human mesenchymal stem cells (BMSCs), activated SMAD2 and SMAD3 proteins form complexes with co-factor SMAD4 to regulate gene transcription (Heldin et al., 1997; De Kroon et al., 2017).
Notch signaling, in bone marrow, plays an important role determining the maintenance of a pool of mesenchymal progenitors by suppressing osteoblast differentiation (Hilton et al., 2008). Furthermore, alterations in signaling by Notch and NGF could have important effects on neurogenesis and neurodevelopmental processes (Chao et al., 2006; Lathia et al., 2008), together with Hippo signaling (Wang and Wang, 2016), PI3K/AKT activation (Cuesto et al., 2011), FoxO signaling (Polter et al., 2009) and signaling by ERBB (Mei and Nave, 2014), which overall control neuronal maintenance, as well as behavioral manifestation, and may be crucial for TS pathogenesis.
Finally, we evaluated whether a relationship could exist between miRNA expression and TS and AC clinical parameters, commonly used in the diagnostic evaluation.
We found several linear correlations between miRNA serum expression levels, intelligent quotient scores, cranial disproportions, and behavioral impairments (Figure 2).
Among the most interesting, the lower concentrations of miR-144-3p in ACTS group, and miR-451a,−93-5p,−144-3p in TS, both compared to AC group, have been found associated to higher Conners' scale scores, commonly used to measure behavioral problems. The associations between lower concentrations of miR-144-3p and higher Conner's scale scores both in TS and ACTS vs. AC, suggest that the TS phenotype, in comorbid condition, could contribute to impair serum abundance of this miRNA more strongly than AC phenotype. This hypothesis is consistent with the markedly different expression of miR-144-3p between the AC and ACTS groups, differently than ACTS and TS comparison showing no difference (Figure 1). Moreover, we found significant correlations between the abundance of miR-21-5p,−451a,−93-5p,−144-3p,−25-3p in TS, compared to AC group, and higher CBCL scores, which assessed behavior impairments in children.
Overall, the multiplicity of such correlations strongly suggests that the miRNA differential abundance in serum is potentially associated with the occurrence and worsening of behavioral impairments related to TS psychopathology.
Other interesting associations between some DE miRNAs and neuroimaging measurements were revealed. Among these, by comparing the AC vs. TS groups, we found a relevant association between the lower serum concentrations of miR-23a-3p and the smaller area measures of the lower PCF in AC group, which is a clinical hallmark of AC patients (Marin-Padilla and Marin-Padilla, 1981; Milhorat et al., 2010).
Taken together, these associations might indicate that miRNA profiling are consistent with clinical findings and could potentially support the current diagnostic evaluation.
Despite the exploratory nature and the limited sample size, due to the rarity of the pathological conditions examined, our study offers new insights into the molecular bases of AC, TS, and ACTS diseases. The preliminary results strongly encourage further investigations in independent cohorts that could validate the diagnostic power of the identified circulating miRNAs as appropriate non-invasive biomarkers.
Data Availability Statement
The datasets generated for this study can be found in online repositories. The names of the repository/repositories and accession number(s) can be found in the article/Supplementary Material.
Ethics Statement
The studies involving human participants were reviewed and approved by Comitato Etico Catania 1. Written informed consent to participate in this study was provided by the participants' legal guardian/next of kin.
Author Contributions
RR, MR, and MP conceived the project and planned the experiments. MG and MCa carried out patient recruitment. RR performed the clinical diagnosis. SP performed magnetic resonance scans and neuroimaging measurements. FM, GL, and MCi performed the experiments. FM, GL, MCi, DB, and MS performed the bioinformatics analysis and data statistics. MR and FM wrote the manuscript. RR, MR, MP, and CDP revised the manuscript. All authors read and approved the final manuscript.
Funding
This study was funded by the 2016/2018 Research Plan of University of Catania, Department of Clinical and Experimental Medicine and 2016/2018 Department Research Plan of University of Catania (2nd line of intervention).
Conflict of Interest
The authors declare that the research was conducted in the absence of any commercial or financial relationships that could be construed as a potential conflict of interest.
Acknowledgments
We wish to thank the Scientific Bureau of the University of Catania for language support.
Supplementary Material
The Supplementary Material for this article can be found online at: https://www.frontiersin.org/articles/10.3389/fnmol.2020.608355/full#supplementary-material
References
Achenbach, T. M., and Rescorla, L. A. (2001). Manual for the ASEBA School-Age Forms and Profiles: Child Behavior Checklist for Ages 6-18, Teacher's Report Form, Youth Self-Report: An Integrated System of Multi-Informant Assessment. Burlington, VT: University of Vermont, Research Center for Children Youth and Families.
Alural, B., Genc, S., and Haggarty, S. J. (2017). Diagnostic and therapeutic potential of microRNAs in neuropsychiatric disorders: past, present, and future. Prog. Neuropsychopharmacol. Biol. Psychiatry 73, 87–103. doi: 10.1016/j.pnpbp.2016.03.010
Bakim, B., Goksan Yavuz, B., Yilmaz, A., Karamustafalioglu, O., Akbiyik, M., Yayla, S., et al. (2013). The quality of life and psychiatric morbidity in patients operated for Arnold-Chiari malformation type I. Int. J. Psychiatry Clin. Pract. 17, 259–263. doi: 10.3109/13651501.2013.778295
Baldan, L. C., Williams, K. A., Gallezot, J. D., Pogorelov, V., Rapanelli, M., Crowley, M., et al. (2014). Histidine decarboxylase deficiency causes Tourette syndrome: parallel findings in humans and mice. Neuron 81, 77–90. doi: 10.1016/j.neuron.2013.10.052
Barry, A., Patten, B. M., and Stewart, B. H. (1957). Possible factors in the development of the Arnold-Chiari malformation. J. Neurosurg. 14, 285–301. doi: 10.3171/jns.1957.14.3.0285
Bertelsen, B., Melchior, L., Jensen, L. R., Groth, C., Glenthoj, B., Rizzo, R., et al. (2014). Intragenic deletions affecting two alternative transcripts of the IMMP2L gene in patients with Tourette syndrome. Eur. J. Hum. Genet. 22, 1283–1289. doi: 10.1038/ejhg.2014.24
Bian, S., and Sun, T. (2011). Functions of noncoding RNAs in neural development and neurological diseases. Mol. Neurobiol. 44, 359–373. doi: 10.1007/s12035-011-8211-3
Cavanna, A. E. (2018). Gilles de la Tourette syndrome as a paradigmatic neuropsychiatric disorder. CNS Spectr. 23, 213–218. doi: 10.1017/S1092852918000834
Caykoylu, A., Ekinci, O., Albayrak, Y., Kuloglu, M., and Deniz, O. (2008). Arnold-Chiari I malformation association with generalized anxiety disorder: a case report. Prog. Neuropsychopharmacol. Biol. Psychiatry 32, 1613–1614. doi: 10.1016/j.pnpbp.2008.05.018
Chao, M. V., Rajagopal, R., and Lee, F. S. (2006). Neurotrophin signalling in health and disease. Clin. Sci. (Lond.) 110, 167–173. doi: 10.1042/CS20050163
Chiari, H. (1987). Concerning alterations in the cerebellum resulting from cerebral hydrocephalus. 1891. Pediatr. Neurosci. 13, 3–8. doi: 10.1159/000120293
Cirnigliaro, M., Barbagallo, C., Gulisano, M., Domini, C. N., Barone, R., Barbagallo, D., et al. (2017). Expression and regulatory network analysis of miR-140-3p, a new potential serum biomarker for autism spectrum disorder. Front. Mol. Neurosci. 10:250. doi: 10.3389/fnmol.2017.00250
Cuesto, G., Enriquez-Barreto, L., Carames, C., Cantarero, M., Gasull, X., Sandi, C., et al. (2011). Phosphoinositide-3-kinase activation controls synaptogenesis and spinogenesis in hippocampal neurons. J. Neurosci. 31, 2721–2733. doi: 10.1523/JNEUROSCI.4477-10.2011
De Kroon, L. M., Narcisi, R., Van Den Akker, G. G., Vitters, E. L., Blaney Davidson, E. N., Van Osch, G. J., et al. (2017). SMAD3 and SMAD4 have a more dominant role than SMAD2 in TGFbeta-induced chondrogenic differentiation of bone marrow-derived mesenchymal stem cells. Sci. Rep. 7:43164. doi: 10.1038/srep43164
De Sena Cortabitarte, A., Berkel, S., Cristian, F. B., Fischer, C., and Rappold, G. A. (2018). A direct regulatory link between microRNA-137 and SHANK2: implications for neuropsychiatric disorders. J. Neurodev. Disord. 10:15. doi: 10.1186/s11689-018-9233-1
Edgar, R., Domrachev, M., and Lash, A. E. (2002). Gene expression omnibus: NCBI gene expression and hybridization array data repository. Nucleic Acids Res. 30, 207–210. doi: 10.1093/nar/30.1.207
Efron, D., and Dale, R. C. (2018). Tics and Tourette syndrome. J. Paediatr. Child Health 54, 1148–1153. doi: 10.1111/jpc.14165
Epstein, N. E. (2018). Definitions and treatments for chiari-1 malformations and its variants: focused review. Surg. Neurol. Int. 9:152. doi: 10.4103/sni.sni_208_18
Fan, Y., Siklenka, K., Arora, S. K., Ribeiro, P., Kimmins, S., and Xia, J. (2016). miRNet - dissecting miRNA-target interactions and functional associations through network-based visual analysis. Nucleic Acids Res. 44, W135–W141. doi: 10.1093/nar/gkw288
Fineberg, S. K., Kosik, K. S., and Davidson, B. L. (2009). MicroRNAs potentiate neural development. Neuron 64, 303–309. doi: 10.1016/j.neuron.2009.10.020
Fujiwara, T., Uotani, K., Yoshida, A., Morita, T., Nezu, Y., Kobayashi, E., et al. (2017). Clinical significance of circulating miR-25-3p as a novel diagnostic and prognostic biomarker in osteosarcoma. Oncotarget 8, 33375–33392. doi: 10.18632/oncotarget.16498
Ganos, C., and Martino, D. (2015). Tics and Tourette syndrome. Neurol. Clin. 33, 115–136. doi: 10.1016/j.ncl.2014.09.008
Gao, J., Yang, T., Han, J., Yan, K., Qiu, X., Zhou, Y., et al. (2011). MicroRNA expression during osteogenic differentiation of human multipotent mesenchymal stromal cells from bone marrow. J. Cell. Biochem. 112, 1844–1856. doi: 10.1002/jcb.23106
George, T. M., and Higginbotham, N. H. (2011). Defining the signs and symptoms of Chiari malformation type I with and without syringomyelia. Neurol. Res. 33, 240–246. doi: 10.1179/016164111X12962202723760
Gianarris, W. J., Golden, C. J., and Greene, L. (2001). The Conners' parent rating scales: a critical review of the literature. Clin. Psychol. Rev. 21, 1061–1093. doi: 10.1016/S0272-7358(00)00085-4
Granata, T., and Valentini, L. G. (2011). Epilepsy in type 1 Chiari malformation. Neurol. Sci. 32(Suppl. 3), S303–306. doi: 10.1007/s10072-011-0697-y
Grosso, S., Scattolini, R., Paolo, G., Di Bartolo, R. M., Morgese, G., and Balestri, P. (2001). Association of Chiari I malformation, mental retardation, speech delay, and epilepsy: a specific disorder? Neurosurgery 49, 1099–1103. discussion: 1103–1094. doi: 10.1227/00006123-200111000-00015
Heldin, C. H., Miyazono, K., and Ten Dijke, P. (1997). TGF-beta signalling from cell membrane to nucleus through SMAD proteins. Nature 390, 465–471. doi: 10.1038/37284
Hicks, S. D., Carpenter, R. L., Wagner, K. E., Pauley, R., Barros, M., Tierney-Aves, C., et al. (2019). Saliva microRNA differentiates children with autism from peers with typical and atypical development. J. Am. Acad. Child Adolesc. Psychiatry. 59, 296–308. doi: 10.1016/j.jaac.2019.03.017
Hicks, S. D., Ignacio, C., Gentile, K., and Middleton, F. A. (2016). Salivary miRNA profiles identify children with autism spectrum disorder, correlate with adaptive behavior, and implicate ASD candidate genes involved in neurodevelopment. BMC Pediatr. 16:52. doi: 10.1186/s12887-016-0586-x
Hicks, S. D., and Middleton, F. A. (2016). A comparative review of microRNA expression patterns in autism spectrum disorder. Front. Psychiatry 7:176. doi: 10.3389/fpsyt.2016.00176
Hilton, M. J., Tu, X., Wu, X., Bai, S., Zhao, H., Kobayashi, T., et al. (2008). Notch signaling maintains bone marrow mesenchymal progenitors by suppressing osteoblast differentiation. Nat. Med. 14, 306–314. doi: 10.1038/nm1716
Jayarao, M., Sohl, K., and Tanaka, T. (2015). Chiari malformation I and autism spectrum disorder: an underrecognized coexistence. J. Neurosurg. Pediatr. 15, 96–100. doi: 10.3171/2014.10.PEDS13562
Karagiannidis, I., Rizzo, R., Tarnok, Z., Wolanczyk, T., Hebebrand, J., Nothen, M. M., et al. (2012). Replication of association between a SLITRK1 haplotype and Tourette syndrome in a large sample of families. Mol. Psychiatry 17, 665–668. doi: 10.1038/mp.2011.151
Kichukova, T. M., Popov, N. T., Ivanov, H. Y., and Vachev, T. I. (2015). Circulating microRNAs as a novel class of potential diagnostic biomarkers in neuropsychiatric disorders. Folia Med. (Plovdiv) 57, 159–172. doi: 10.1515/folmed-2015-0035
Kichukova, T. M., Popov, N. T., Ivanov, I. S., and Vachev, T. I. (2017). Profiling of circulating serum microRNAs in children with autism spectrum disorder using stem-loop qRT-PCR assay. Folia Med. (Plovdiv) 59, 43–52. doi: 10.1515/folmed-2017-0009
Kim, V. N., and Nam, J. W. (2006). Genomics of microRNA. Trends Genet. 22, 165–173. doi: 10.1016/j.tig.2006.01.003
Kosaka, N., Iguchi, H., and Ochiya, T. (2010). Circulating microRNA in body fluid: a new potential biomarker for cancer diagnosis and prognosis. Cancer Sci. 101, 2087–2092. doi: 10.1111/j.1349-7006.2010.01650.x
Kosik, K. S. (2006). The neuronal microRNA system. Nat. Rev. Neurosci. 7, 911–920. doi: 10.1038/nrn2037
Koyama, T., Inada, N., Tsujii, H., and Kurita, H. (2008). Predicting children with pervasive developmental disorders using the wechsler intelligence scale for children-third edition. Psychiatry Clin. Neurosci. 62, 476–478. doi: 10.1111/j.1440-1819.2008.01826.x
Lacy, M., Dedios-Stern, S., Fredrickson, S., Parikh, S., Nader, T., and Frim, D. M. (2018). Prevalence of psychiatric diagnoses in pediatric chiari malformation type 1. Pediatr. Neurosurg. 53, 371–378. doi: 10.1159/000488460
Langridge, B., Phillips, E., and Choi, D. (2017). Chiari malformation type 1: a systematic review of natural history and conservative management. World Neurosurg. 104, 213–219. doi: 10.1016/j.wneu.2017.04.082
Lathia, J. D., Mattson, M. P., and Cheng, A. (2008). Notch: from neural development to neurological disorders. J. Neurochem. 107, 1471–1481. doi: 10.1111/j.1471-4159.2008.05715.x
Lawson-Yuen, A., Saldivar, J. S., Sommer, S., and Picker, J. (2008). Familial deletion within NLGN4 associated with autism and Tourette syndrome. Eur. J. Hum. Genet. 16, 614–618. doi: 10.1038/sj.ejhg.5202006
Leckman, J. F., Riddle, M. A., Hardin, M. T., Ort, S. I., Swartz, K. L., Stevenson, J., et al. (1989). The Yale global tic severity scale: initial testing of a clinician-rated scale of tic severity. J. Am. Acad. Child Adolesc. Psychiatry 28, 566–573. doi: 10.1097/00004583-198907000-00015
Marin-Padilla, M. (1991). Cephalic axial skeletal-neural dysraphic disorders: embryology and pathology. Can. J. Neurol. Sci. 18, 153–169. doi: 10.1017/S0317167100031632
Marin-Padilla, M., and Marin-Padilla, T. M. (1981). Morphogenesis of experimentally induced arnold–chiari malformation. J. Neurol. Sci. 50, 29–55. doi: 10.1016/0022-510X(81)90040-X
Markunas, C. A., Lock, E., Soldano, K., Cope, H., Ding, C. K., Enterline, D. S., et al. (2014). Identification of chiari type i malformation subtypes using whole genome expression profiles and cranial base morphometrics. BMC Med. Genomics 7:39. doi: 10.1186/1755-8794-7-39
Mei, L., and Nave, K. A. (2014). Neuregulin-ERBB signaling in the nervous system and neuropsychiatric diseases. Neuron 83, 27–49. doi: 10.1016/j.neuron.2014.06.007
Mejia, N. I., and Jankovic, J. (2005). Secondary tics and tourettism. Braz. J. Psychiatry 27, 11–17. doi: 10.1590/S1516-44462005000100006
Milhorat, T. H., Nishikawa, M., Kula, R. W., and Dlugacz, Y. D. (2010). Mechanisms of cerebellar tonsil herniation in patients with chiari malformations as guide to clinical management. Acta Neurochir. (Wien) 152, 1117–1127. doi: 10.1007/s00701-010-0636-3
Monroe, D. G., Mcgee-Lawrence, M. E., Oursler, M. J., and Westendorf, J. J. (2012). Update on Wnt signaling in bone cell biology and bone disease. Gene 492, 1–18. doi: 10.1016/j.gene.2011.10.044
Mor, M., Nardone, S., Sams, D. S., and Elliott, E. (2015). Hypomethylation of miR-142 promoter and upregulation of microRNAs that target the oxytocin receptor gene in the autism prefrontal cortex. Mol. Autism 6:46. doi: 10.1186/s13229-015-0040-1
Mundalil Vasu, M., Anitha, A., Thanseem, I., Suzuki, K., Yamada, K., Takahashi, T., et al. (2014). Serum microRNA profiles in children with autism. Mol. Autism 5:40. doi: 10.1186/2040-2392-5-40
Nevel, K. S., Wilcox, J. A., Robell, L. J., and Umemura, Y. (2018). The utility of liquid biopsy in central nervous system malignancies. Curr. Oncol. Rep. 20:60. doi: 10.1007/s11912-018-0706-x
Nguyen, L. S., Fregeac, J., Bole-Feysot, C., Cagnard, N., Iyer, A., Anink, J., et al. (2018). Role of miR-146a in neural stem cell differentiation and neural lineage determination: relevance for neurodevelopmental disorders. Mol. Autism 9:38. doi: 10.1186/s13229-018-0219-3
Nt, P., Ds, M., Mm, N., In, M., and Ti, V. (2018). Investigation of circulating serum microRNA-328-3p and microRNA-3135a expression as promising novel biomarkers for autism spectrum disorder. Balkan J. Med. Genet. 21, 5–12. doi: 10.2478/bjmg-2018-0026
O'Brien, J., Hayder, H., Zayed, Y., and Peng, C. (2018). Overview of microRNA biogenesis, mechanisms of actions, and circulation. Front. Endocrinol. (Lausanne) 9:402. doi: 10.3389/fendo.2018.00402
Osuagwu, F. C., Amalraj, B., Noveloso, B. D., Aikoye, S. A., and Bradley, R. (2016). Autism spectrum disorder and chiari 1 malformation co-occurring in a child. Tokai J. Exp. Clin. Med. 41, 54–56.
Paschou, P., Stylianopoulou, E., Karagiannidis, I., Rizzo, R., Tarnok, Z., Wolanczyk, T., et al. (2012). Evaluation of the LIM homeobox genes LHX6 and LHX8 as candidates for Tourette syndrome. Genes Brain Behav. 11, 444–451. doi: 10.1111/j.1601-183X.2012.00778.x
Polter, A., Yang, S., Zmijewska, A. A., Van Groen, T., Paik, J. H., Depinho, R. A., et al. (2009). Forkhead box, class O transcription factors in brain: regulation and behavioral manifestation. Biol. Psychiatry 65, 150–159. doi: 10.1016/j.biopsych.2008.08.005
Ragusa, M., Barbagallo, C., Brex, D., Caponnetto, A., Cirnigliaro, M., Battaglia, R., et al. (2017). Molecular crosstalking among noncoding RNAs: a new network layer of genome regulation in cancer. Int. J. Genomics 2017:4723193. doi: 10.1155/2017/4723193
Rao, P., Benito, E., and Fischer, A. (2013). MicroRNAs as biomarkers for CNS disease. Front. Mol. Neurosci. 6:39. doi: 10.3389/fnmol.2013.00039
Rizzo, R., Gulisano, M., Pellico, A., Cali, P. V., and Curatolo, P. (2014). Tourette syndrome and comorbid conditions: a spectrum of different severities and complexities. J. Child Neurol. 29, 1383–1389. doi: 10.1177/0883073814534317
Rizzo, R., Ragusa, M., Barbagallo, C., Sammito, M., Gulisano, M., Cali, P. V., et al. (2015). Circulating miRNAs profiles in Tourette syndrome: molecular data and clinical implications. Mol. Brain 8:44. doi: 10.1186/s13041-015-0133-y
Robertson, M. M. (2000). Tourette syndrome, associated conditions and the complexities of treatment. Brain 123(Pt 3), 425–462. doi: 10.1093/brain/123.3.425
Robertson, M. M. (2015). A personal 35 year perspective on Gilles de la Tourette syndrome: prevalence, phenomenology, comorbidities, and coexistent psychopathologies. Lancet Psychiatry 2, 68–87. doi: 10.1016/S2215-0366(14)00132-1
Salta, E., and De Strooper, B. (2012). Non-coding RNAs with essential roles in neurodegenerative disorders. Lancet Neurol. 11, 189–200. doi: 10.1016/S1474-4422(11)70286-1
Scahill, L., Riddle, M. A., Mcswiggin-Hardin, M., Ort, S. I., King, R. A., Goodman, W. K., et al. (1997). Children's yale-brown obsessive compulsive scale: reliability and validity. J. Am. Acad. Child Adolesc. Psychiatry 36, 844–852. doi: 10.1097/00004583-199706000-00023
Shaffer, N., Martin, B., and Loth, F. (2011). Cerebrospinal fluid hydrodynamics in type I chiari malformation. Neurol. Res. 33, 247–260. doi: 10.1179/016164111X12962202723805
Shoja, M. M., Johal, J., Oakes, W. J., and Tubbs, R. S. (2018). Embryology and pathophysiology of the chiari I and II malformations: a comprehensive review. Clin. Anat. 31, 202–215. doi: 10.1002/ca.22939
Singer, H. S., and Augustine, F. (2019). Controversies surrounding the pathophysiology of tics. J. Child Neurol. 34, 851–862. doi: 10.1177/0883073819862121
Speer, M. C., Enterline, D. S., Mehltretter, L., Hammock, P., Joseph, J., Dickerson, M., et al. (2003). Review article: chiari type I malformation with or without syringomyelia: prevalence and genetics. J. Genet. Couns. 12, 297–311. doi: 10.1023/A:1023948921381
Speer, M. C., George, T. M., Enterline, D. S., Franklin, A., Wolpert, C. M., and Milhorat, T. H. (2000). A genetic hypothesis for chiari I malformation with or without syringomyelia. Neurosurg. Focus 8:E12. doi: 10.3171/foc.2000.8.3.12
Tonacci, A., Bagnato, G., Pandolfo, G., Billeci, L., Sansone, F., Conte, R., et al. (2019). MicroRNA cross-involvement in autism spectrum disorders and atopic dermatitis: a literature review. J. Clin. Med. 8:88. doi: 10.3390/jcm8010088
Treiber, T., Treiber, N., and Meister, G. (2019). Regulation of microRNA biogenesis and its crosstalk with other cellular pathways. Nat. Rev. Mol. Cell Biol. 20, 5–20. doi: 10.1038/s41580-018-0059-1
Turchinovich, A., Samatov, T. R., Tonevitsky, A. G., and Burwinkel, B. (2013). Circulating miRNAs: cell-cell communication function? Front. Genet. 4:119. doi: 10.3389/fgene.2013.00119
Turchinovich, A., Weiz, L., Langheinz, A., and Burwinkel, B. (2011). Characterization of extracellular circulating microRNA. Nucleic Acids Res. 39, 7223–7233. doi: 10.1093/nar/gkr254
Urbizu, A., Toma, C., Poca, M. A., Sahuquillo, J., Cuenca-Leon, E., Cormand, B., et al. (2013). Chiari malformation type I: a case-control association study of 58 developmental genes. PLoS ONE 8:e57241. doi: 10.1371/journal.pone.0057241
Verkerk, A. J., Mathews, C. A., Joosse, M., Eussen, B. H., Heutink, P., Oostra, B. A., et al. (2003). CNTNAP2 is disrupted in a family with Gilles de la Tourette syndrome and obsessive compulsive disorder. Genomics 82, 1–9. doi: 10.1016/S0888-7543(03)00097-1
Vlachos, I. S., Zagganas, K., Paraskevopoulou, M. D., Georgakilas, G., Karagkouni, D., Vergoulis, T., et al. (2015). DIANA-miRPath v3.0: deciphering microRNA function with experimental support. Nucleic Acids Res. 43, W460–W466. doi: 10.1093/nar/gkv403
Wang, S. P., and Wang, L. H. (2016). Disease implication of hyper-Hippo signalling. Open Biol. 6:160119. doi: 10.1098/rsob.160119
Wu, L. H., Peng, M., Yu, M., Zhao, Q. L., Li, C., Jin, Y. T., et al. (2015). Circulating microRNA Let-7d in attention-deficit/hyperactivity disorder. Neuromolecular Med. 17, 137–146. doi: 10.1007/s12017-015-8345-y
Wu, M., Chen, G., and Li, Y. P. (2016). TGF-beta and BMP signaling in osteoblast, skeletal development, and bone formation, homeostasis and disease. Bone Res. 4:16009. doi: 10.1038/boneres.2016.9
Xu, B., Hsu, P. K., Karayiorgou, M., and Gogos, J. A. (2012). MicroRNA dysregulation in neuropsychiatric disorders and cognitive dysfunction. Neurobiol. Dis. 46, 291–301. doi: 10.1016/j.nbd.2012.02.016
Xu, B., Karayiorgou, M., and Gogos, J. A. (2010). MicroRNAs in psychiatric and neurodevelopmental disorders. Brain Res. 1338, 78–88. doi: 10.1016/j.brainres.2010.03.109
Xu, L., Li, X., Liu, Y., Kong, Q., Long, D., and Li, S. (2015). [miR-93-5P SUPPRESSES OSTEOGENIC DIFFERENTIATION OF MOUSE C3H10T1/2 CELLS BY TARGETING Smad5]. Zhongguo Xiu Fu Chong Jian Wai Ke Za Zhi 29, 1288–1294.
Yan, J., Guo, D., Yang, S., Sun, H., Wu, B., and Zhou, D. (2016). Inhibition of miR-222-3p activity promoted osteogenic differentiation of hBMSCs by regulating Smad5-RUNX2 signal axis. Biochem. Biophys. Res. Commun. 470, 498–503. doi: 10.1016/j.bbrc.2016.01.133
Yang, C., Liu, X., Zhao, K., Zhu, Y., Hu, B., Zhou, Y., et al. (2019). miRNA-21 promotes osteogenesis via the PTEN/PI3K/Akt/HIF-1alpha pathway and enhances bone regeneration in critical size defects. Stem Cell Res. Ther. 10:65. doi: 10.1186/s13287-019-1168-2
Yu, D., Sul, J. H., Tsetsos, F., Nawaz, M. S., Huang, A. Y., Zelaya, I., et al. (2019). Interrogating the genetic determinants of Tourette's syndrome and other tic disorders through genome-wide association studies. Am. J. Psychiatry 176, 217–227. doi: 10.1176/appi.ajp.2018.18070857
Zadehbagheri, F., Hosseini, E., Bagheri-Hosseinabadi, Z., Rekabdarkolaee, H. M., and Sadeghi, I. (2019). Profiling of miRNAs in serum of children with attention-deficit hyperactivity disorder shows significant alterations. J. Psychiatr. Res. 109, 185–192. doi: 10.1016/j.jpsychires.2018.12.013
Keywords: circulating microRNAs, liquid biopsies, comorbidity, biological pathways, neuropsychological parameters, neuroimaging parameters
Citation: Mirabella F, Gulisano M, Capelli M, Lauretta G, Cirnigliaro M, Palmucci S, Stella M, Barbagallo D, Di Pietro C, Purrello M, Ragusa M and Rizzo R (2021) Enrichment and Correlation Analysis of Serum miRNAs in Comorbidity Between Arnold-Chiari and Tourette Syndrome Contribute to Clarify Their Molecular Bases. Front. Mol. Neurosci. 13:608355. doi: 10.3389/fnmol.2020.608355
Received: 20 September 2020; Accepted: 04 December 2020;
Published: 05 January 2021.
Edited by:
Verena Tretter, Medical University of Vienna, AustriaReviewed by:
Alicja Pacholewska, University Hospital of Cologne, GermanyIsmael Santa-Maria, Columbia University, United States
Copyright © 2021 Mirabella, Gulisano, Capelli, Lauretta, Cirnigliaro, Palmucci, Stella, Barbagallo, Di Pietro, Purrello, Ragusa and Rizzo. This is an open-access article distributed under the terms of the Creative Commons Attribution License (CC BY). The use, distribution or reproduction in other forums is permitted, provided the original author(s) and the copyright owner(s) are credited and that the original publication in this journal is cited, in accordance with accepted academic practice. No use, distribution or reproduction is permitted which does not comply with these terms.
*Correspondence: Marco Ragusa, mragusa@unict.it
†These authors share senior authorship