- 1Instituto de Neurobiología, Universidad Nacional Autónoma de México, Juriquilla, Mexico
- 2Achucarro Basque Center for Neuroscience, Departamento de Neurociencias, Universidad del País Vasco, CIBERNED, Leioa, Spain
- 3Institute of Neurobiology, Slovak Academy of Sciences, Kosice, Slovakia
- 4Department of Immunology, University Hospital, Hamburg, Germany
Shortage of oxygen and nutrients in the brain induces the release of glutamate and ATP that can cause excitotoxicity and contribute to neuronal and glial damage. Our understanding of the mechanisms of ATP release and toxicity in cerebrovascular diseases is incomplete. This review aims at summarizing current knowledge about the participation of key elements in the ATP-mediated deleterious effects in these pathologies. This includes pannexin-1 hemichannels, calcium homeostasis modulator-1 (CALHM1), purinergic P2X7 receptors, and other intermediaries of CNS injury downstream of ATP release. Available data together with recent pharmacological developments in purinergic signaling may constitute a new opportunity to translate preclinical findings into more effective therapies in cerebrovascular diseases.
Introduction
Cerebrovascular diseases (CVDs) are referred to as a group of conditions that eventually lead to a reduction of blood supply to the brain as a consequence of a blockage (thrombosis or atherosclerosis), malformation (aneurysm), hemorrhage, or transient ischemia. In all instances, a decrease in oxygenation and nutrient supply ultimately leads to brain damage. Cerebrovascular diseases, principally stroke, constitute the second leading cause of death in adults worldwide and are major contributors to disability and reduced quality of life (Truelsen et al., 2000), so it is important to know the mechanisms behind this damage to reduce their consequences with the design and use of therapies against these diseases.
At the cellular level, damaged neurons and glial cells during and after stressful events in CVDs release glutamate into the extracellular space, which finally induces cytosolic Ca2+ overload and excitotoxicity (Braun et al., 1998; Jurányi et al., 1999; Melani et al., 2005; Takeuchi et al., 2006; for reviews, see also Rossi et al., 2007; Yenari et al., 2010). More recently, ATP was also defined as a potent excitotoxic signal to oligodendrocytes and neurons (Matute et al., 2007; Domercq et al., 2010; Cisneros-Mejorado et al., 2015b). In addition to ATP being among the molecules that are released by cell damage, recent evidence suggests that ATP acts as a damage-associated molecular pattern (DAMP) to initiate the innate immune response, induce pro-inflammation, and contribute to progressive neurological injury (Braun et al., 2017). Moreover, the massive increase in the cytosolic concentration of Ca2+ is due in part to the overactivation of P2X7 receptors, a nonselective ligand-gated cation channel expressed at the cell surface of various cell types and activated by extracellular ATP. In this review, we summarize the state of the art regarding the P2X7 receptor role in cerebrovascular damage and its possible use as a therapeutic target. In addition, we discuss its relationship with other molecular agents, such as pannexins (Panxs) and calcium-permeable channels, representing all together a pathological orchestrated cluster that can contribute to the onset of tissue damage and its propagation in CVDs.
P2X7 Receptors Are Major Mediators of Tissue Damage in Cerebrovascular Diseases
Energy deprivation after stroke causes anoxic and irreversible depolarization. These events subsequently lead to massive release of excitatory neurotransmitters, including glutamate and ATP (Braun et al., 1998; Jurányi et al., 1999; Melani et al., 2005; Rossi et al., 2007), the latter causing neuronal and glial cell death through P2X7 receptor activation (Matute et al., 2007; Domercq et al., 2010; Arbeloa et al., 2012). However, the mechanisms of deleterious ATP release during brain ischemia are only partially known (Dale and Frenguelli, 2009; Cisneros-Mejorado et al., 2015b). Thus, during ischemia, the lack of oxygen causes a reduction in ATP production with an ensuing failure of plasma membrane ion pumps and loss of ion concentration homeostasis, all of which can finally lead to activation of Panx1 and calcium homeostasis modulator-1 (CALHM1) and the subsequent release of ATP (Cisneros-Mejorado et al., 2015b). These events lead to sustained activation of P2X7 receptors and pore formation with ensuing further ATP release that together creates a vicious circle, resulting in enhanced ATP-mediated excitotoxicity (Cisneros-Mejorado et al., 2015b). Indeed, pharmacological blockade or gene ablation of P2X7, Panx1, and CALHM1 results in substantial delayed post-anoxic depolarization following oxygen-glucose deprivation (OGD) and reduced brain tissue damage after transient middle cerebral artery occlusion (MCAO; Cisneros-Mejorado et al., 2015a, 2018; Figure 1).
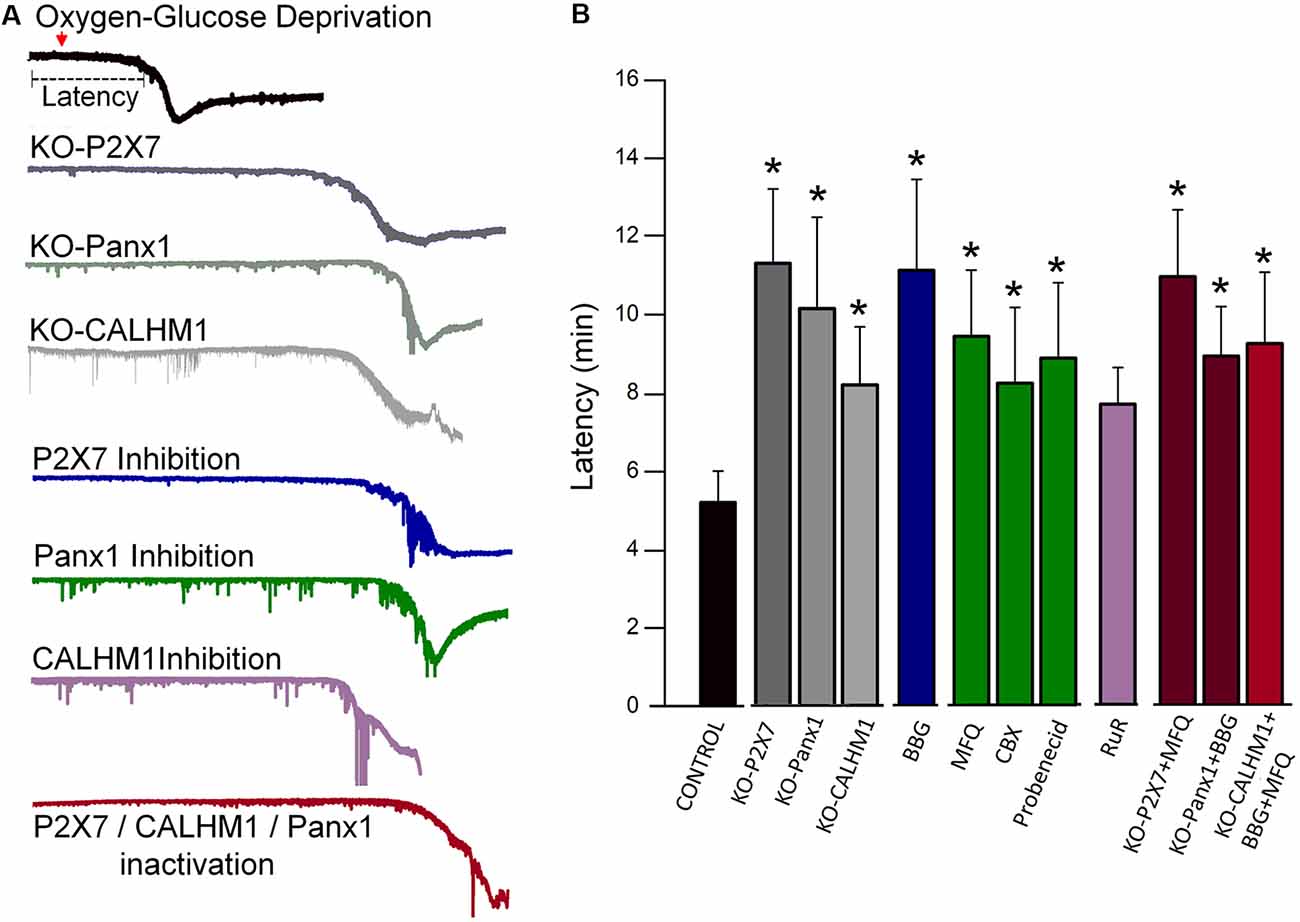
Figure 1. Blockade or genetic ablation of P2X7 receptors, pannexin-1 (Panx1), or calcium homeostasis modulator-1 (CALHM1) channels delays ischemic ionic currents in acute cortical slices. (A) Representative electrophysiological recordings in whole-cell configuration (holding potential at −80 mV), of ischemic (oxygen-glucose deprivation, OGD) ionic currents in cortical neurons in acute brain slices from wild-type mice as well as from either P2X7, Panx1, or CALHM1 KO mice in the presence or absence of inhibitors of these channels. (B) Histogram showing the latency of the onset of ischemic ionic currents following OGD in the absence (Control) or in the presence of P2X7 receptor antagonist Brilliant Blue G (BBG, 50 nM); Panx1 inhibitors mefloquine (MFQ, 100 nM), carbenoxolone (CBX, 100 μM), and probenecid (1 mM); and CALHM1 inhibitor ruthenium red (RuR, 20 μM). Similar increased latency values were observed in neurons from acute slices obtained from P2X7 receptor, Panx1, and CALHM1 knockout mice (KO-P2X7, KO-Panx1, and KO-CALHM1, respectively). Concomitant blockade of more than one target (red columns) did not result in further delay of the post-anoxic current. All the data included in this graph was published earlier (Cisneros-Mejorado et al., 2015a; Cisneros-Mejorado et al., 2018; *p < 0.05 vs. control).
In addition to neuronal and oligodendroglial excitotoxicity, activation of P2X7 receptors triggers the formation of the inflammasome, a multiprotein complex that mediates the release of cytokines, such as IL-1β, IL-18, and IL-33 (Giuliani et al., 2017; Baroja-Mazo et al., 2013), which can expand the initial ischemic damage.
P2X7 receptors are expressed in neurons and glia (see Table 1). Neurons express P2X7 receptors (Yu et al., 2008; Díaz-Hernández et al., 2009; Miras-Portugal et al., 2017; but see also Illes et al., 2017), and its blockade prevents ATP excitotoxicity and reduces the damage in models of both in vivo and in vitro ischemia (Arbeloa et al., 2012; Cisneros-Mejorado et al., 2015a). Likewise, oligodendrocytes, which are the major cellular component of white matter in the CNS, can undergo direct ATP-mediated excitotoxicity via activation of P2X7 receptors expressed in their membrane (James and Butt, 2001; Agresti et al., 2005; Matute et al., 2007; Yu et al., 2008; Domercq et al., 2010). Indeed, P2X7 receptors mediate ischemic damage to oligodendrocytes in culture and in optic nerve explants (Domercq et al., 2010). Moreover, oligodendrocyte precursor cells (OPCs) also express P2X7 receptors that contribute to periventricular white matter during perinatal hypoxic-ischemic injury as this condition is attenuated with selective antagonists of these receptors (Wang et al., 2009).
On the other hand, microglia express P2X7 receptors (Ferrari et al., 1996; Collo et al., 1997; Visentin et al., 1999; Sanz and Di Virgilio, 2000; Hide et al., 2000; Verderio and Metteoli, 2001; Chafke et al., 2002) that can promote their activation and proliferation (Bianco et al., 2006; Monif et al., 2009). This can indirectly cause neurotoxicity by stimulating the production of reactive oxygen species (Bartlett et al., 2013) as well as the release of pro-inflammatory cytokines (Suzuki et al., 2004; Shieh et al., 2014). Interestingly, P2X7 receptors in primary adult human microglia kept in culture modulate key components of innate immunity (Janks et al., 2018). Finally, P2X7 receptors are present in astrocytes (Ballerini et al., 1996; Sun et al., 1999; Panenka et al., 2001; Franke et al., 2001; James and Butt, 2001; Kukley et al., 2001), whereby they can raise intracellular Ca2+ concentration and ATP release (Suadicani et al., 2006) as well as contribute to non-vesicular glutamate release (Duan et al., 2003). Table 1 summarizes the evidence supporting the expression of P2X7 receptors in neurons and glia.
Together, these data indicate that activation of P2X7 receptors can activate deleterious signals after ischemia by altering Ca2+ homeostasis and promoting the release of pro-inflammatory cytokines as well as causing oxidative stress. Accordingly, P2X7 blockade reduces tissue damage in experimental models of CVDs. Thus, Brilliant Blue G (BBG), a P2X7 selective antagonist, attenuates the extent of brain damage following transient MCAO (Arbeloa et al., 2012; Cisneros-Mejorado et al., 2015a). Similarly, BBG treatment ameliorates neuronal apoptosis in an experimental subarachnoid hemorrhage model (Chen et al., 2013). Moreover, three different P2X7 antagonists (BBG, A0438079, and OxATP) significantly increase survival rates and reduce cognitive deficits and cell death in transient global ischemia-reperfusion injury (Chu et al., 2012). Notably, P2X7 receptor attenuated glial activation and inflammatory cytokine overexpression in the hippocampus (Chu et al., 2012). Table 2 sums up the protective effects of the inhibition or deletion of P2X7 described above.
Activation and Biophysical Properties of P2X7 Receptors in Neurons and Glia
P2X7 receptors have two possible states of conductance. First, low concentrations of agonist (ATP or BzATP) induce activation of a nonselective monophasic conductance allowing monovalent (Na+, K+) and divalent (Ca2+) cation influx and plasma membrane depolarization (North, 2002). In addition, after prolonged exposure to high agonist concentrations (exposure to high agonist concentrations >1 mM ATP, >30 μM BzATP) P2X7 receptors can form a large nonselective pore allowing the passage of organic cations and molecules of up to 900 Da and the leakage of metabolites, including ATP (North, 2002; Yan et al., 2010), as shown in astrocytes (see above; Suadicani et al., 2006) and microglia (Ferrari et al., 1997). Although it is not clear whether oligodendrocytes leak ATP by this mechanism, there is evidence showing that glucocorticoids increase hemichannel activity in these cells in a P2X7R-dependent manner, suggesting a possible formation of a nonselective pore in these conditions (Maturana et al., 2017). Interestingly, P2X7 receptors in cultured cortical neurons form a large pore only at very high concentrations of BzATP and following a prolonged (10–40 s) exposure to the agonist (Cisneros-Mejorado et al., 2015b) although, in astrocytes in vitro or HEK cells expressing P2X7 receptors, the large pore formation occurs more readily (Yan et al., 2010). Macropore formation cannot be due to receptor pore dilation per se because the single-channel current amplitude and permeation characteristics remain constant (Riedel et al., 2007; Pippel et al., 2017; Di Virgilio et al., 2018). Thus, it appears that additional mechanisms must underlie the opening of the macropore (see Braun et al., 2017). This suggests that large pore formation in cultured neurons depends not only on prolonged stimulation of the P2X7 receptor. Alternatively, a role in ATP release has also been assigned to membrane channels formed by Panx1, which are large-pore ion channels with broad expression in the CNS (MacVicar and Thompson, 2010). Panx1 is permeable to molecules up to 900 Da and directly mediates ATP release (Locovei et al., 2007; Iglesias et al., 2009). The mechanisms by which P2X7 receptors and Panx1 are involved in pore formation are not well defined. However, the evidence indicates in astrocytes, for example, that Panx1 channels are activated after P2X7 receptor stimulation (Iglesias et al., 2009) or on concomitant membrane depolarization to induce Panx1 opening as reported earlier (Locovei et al., 2007). Thus, Panx1 can be activated following Ca2+ influx via P2X7 receptors along or in conjunction with subsequent Ca2+-induced Ca2+ mobilization from intracellular stores (North, 2002; Locovei et al., 2006). These findings suggest that P2X7 receptors and Panx1 act synergistically, at least in the CNS, because, in other systems, pore formation after prolonged activation of the P2X7 receptor does not occur through Panx1 channels (Qu et al., 2011; Alberto et al., 2013). One of the best characterized aspects of P2X7 receptor function is its ability to activate indirectly the NACHT, LRR, and PYD domains containing protein 3 (NLRP3) inflammasome in macrophages, thereby initiating caspase-1-mediated IL-1β; processing and release and ultimately inducing macrophage pyroptotic cell death (Chen et al., 2014). In the brain, exogenous ATP induces NLRP3 inflammasome activation in astrocytes and microglia along with an increase in IL-1β production that activates caspase-1 and amplification of the stress response (Murphy et al., 2012). Consistent with this idea, stressed glia increases connexin 43 (Cx43) and Panx1 hemichannel activity in microglia and astrocytes of adult mice (Orellana et al., 2015). Moreover, exposure to high levels of glucocorticoids during gestation induces long-lasting neuroinflammation and activates the inflammasome in hippocampal oligodendrocytes of mouse offspring (Maturana et al., 2017). Thus, oligodendrocytes of control pups showed expression of inflammasome components (NLRP3, ACS, and caspase-1), and their levels were increased by prenatal administration of dexamethasone, a synthetic glucocorticoid. These cells also showed high levels of IL-1β; and TNF-α; in accordance with activation of the inflammasome. Notably, oligodendrocytes showed increased levels of the P2X7 receptors and Panx1, molecules associated with inflammasome activation (Maturana et al., 2017). However, it is not known whether glucocorticoids also modify the activation properties of the P2X7/Panx1 axis in neurons, a feature that might be relevant in CVDs.
P2X7 Receptors Are Involved in Ischemic Pre- and Post-Conditioning Effects
Brief, non-harmful, ischemic preconditioning can confer tolerance and protection from subsequent cerebrovascular damage. Thus, preconditioning attenuates damage in models of cerebral ischemia associated with oxidative stress and glutamate excitotoxicity (Jachova et al., 2019). Although the mechanisms of this phenomenon are unclear, recent data suggest that P2X7 receptors have a role in ischemia preconditioning as this is ineffective in P2X7 receptor knockout mice (Hirayama et al., 2015). In turn, after preconditioning, P2X7 receptors in astrocytes can orchestrate the initiation of neuroprotective cascades including those mediated by HIF-1α; Hirayama et al., 2015). Indeed, P2X7 receptor expression itself is increased in astrocytes and followed by an elevation of hypoxia-inducible factor (HIF)-1α in these cells after preconditioning using an MCAO model in mice (Hirayama et al., 2015). Moreover, inhibiting the astroglial metabolism with fluorocitrate abolished the induction of ischemic tolerance, which strongly suggests that astrocytes play an essential role in its inception (Hirayama et al., 2015).
In addition, hypoxic preconditioning protects cultured neurons against hypoxic stress via tumor necrosis factor-α; TNF-α; Jie Liu et al., 2000; Ruscher et al., 1998). Notably, the release of TNF-α appears to be dependent on the P2X7 receptor because microglia treated with BzATP in neuron-microglia cultures leads to significant reduction in glutamate-induced neuronal cell death, and either TNF-α-converting enzyme inhibitor or anti-TNF-α IgG readily suppresses this protective effect (Suzuki et al., 2004). These findings provide evidence that, as in astrocytes, P2X7 receptors in microglia contribute to brain ischemic tolerance though different mechanisms.
The above data argues in favor of a dual role of P2X7 receptors in CVDs with a protective edge during mild ischemia (preconditioning) and a deleterious excitotoxic role in more severe ischemia. This duality adds further complexity to the development of effective therapies to prevent ischemic injury by promoting, for example, preconditioning with P2X7 receptor agonists and/or allosteric modulators while having at hand antagonists as neuroprotectants to limit the extension of the ischemic core into the penumbra once stroke occurs. To define the parameters that limit this double-edged behavior of P2X7 receptors constitutes a major challenge in experimental ischemia as a previous step for an effective translation into CVDs patients.
On the other hand, ischemic post-conditioning may be more amenable and effective for therapeutic use. Thus, repetitive short periods of OGD alternated with reperfusion after prolonged OGD attenuates neuronal apoptosis by increasing Bcl-2 expression while reducing Bax levels and overexpressing heat-shock protein 70 (HSP70; Zhao et al., 2014). Intriguingly, proteomic and functional characterization of the P2X7 receptor signaling complex showed that HSP70 co-immunoprecipitates with P2X7 receptors (Kim et al., 2001). This finding strongly suggests an interaction between HSP70 and P2X7 receptors; however, a direct link between P2X7 activity and post-conditioning neuroprotection is still missing.
Therapeutic Potential of P2X7 Receptor in Cerebrovascular Diseases
All the above evidence suggests that P2X7 receptor activation or blockade is involved in the onset and propagation of tissue damage as well as in neuroprotection and neuroinflammation in CVDs. Therefore, a thorough understanding of the upstream events leading to P2X7 receptor activation along with subsequent downstream signaling cascades they trigger will eventually allow identifying new cellular and molecular targets amenable for the design of novel drugs to use in clinical studies.
P2X7 receptor agonists may facilitate ischemia preconditioning by promoting the release of protective factors and signals in neurons and glia that ultimately attenuate major damage (Hirayama et al., 2015). Alternatively, specific P2X7 agonists may enhance the phagocytic capacity of microglia as shown in a model of phagocytic function in fresh human monocytes without promoting pore formation, thus avoiding unwanted side effects, such as excitotoxicity or enhanced neuroinflammation (Ou et al., 2018). This novel role of the P2X7 receptor as a scavenger receptor in microglia/macrophages and possibly in other cells in the CNS creates new pharmacological possibilities as it is not affected by potent selective P2X7 receptor antagonists, and its phagocytic function has features distinct from its pore function (Ou et al., 2018). Therefore, differential drug targeting both on P2X7 pore formation and P2X7-mediated phagocytosis has a great potential as a single or combined treatment in CVDs.
In addition to agonists and antagonists, other P2X7 receptor ligands may have therapeutic value. This includes positive allosteric modulators, such as clemastine, an anti-allergy drug, which binds extracellularly to P2X7 receptors and potentiates their ATP-sensitivity while it increases the release of IL-1β; from lipopolysaccharide-primed macrophages, thus modulating native immune responses (Nörenberg et al., 2011). In turn, clemastine may also favor myelination of damaged myelin and the rescue of behavioral changes that occur after stroke (Liu et al., 2016; Cohen and Tesar, 2017). Likewise, ginsenosides of the protopanaxdiol series potently activate P2X7 receptors, leading to an increase of sustained calcium ion influx in mouse macrophages that may account for their reported immune modulatory actions in vivo (Helliwell et al., 2015).
Discovering new uses for approved drugs acting at P2X7 may provide the quickest possible transition from bench to bedside in CVDs. Thus, A-740003 (N-(1-[(cyanoimino)(5-quinolinylamino) methyl] amino-2,2-dimethylpropyl)-2-(3,4-dimethoxyphenyl)acetamide), a competitive antagonist of P2X7 receptors, produces significant antinociception in animal models of neuropathic and inflammatory pain (Honore et al., 2006) and has been evaluated for neuroinflammation (Janssen et al., 2014). On the other hand, AZD9056, an adamantane amide that was discovered through a program designed to identify potent and selective P2X7 antagonists, provides a significant inhibition of ATP-induced IL-1β release in monocytes ex vivo, suggesting that circulating leucocytes were blocked by P2X7 (Keystone et al., 2012). AZD9056 is well tolerated and induces statistically significant changes in parameters of clinical relevance; however, it failed to ameliorate symptoms in patients with rheumatoid arthritis, an immunologically mediated disease in which cytokines are key regulatory molecules. Similarly, high throughput screening of a compound library provided an attractive lead compound with modest P2X7 receptor antagonist potency and high selectivity against a panel of receptors and channels (Duplantier et al., 2011). Multi-parameter optimization led to a potent P2X7 antagonist, CE-224,535, which was advanced to clinical studies for the treatment of rheumatoid arthritis (Duplantier et al., 2011). This compound is currently under scrutiny for others brain diseases and has therapeutic potential in CVDs.
Another interesting approach in the development of biologics targeting P2X7 receptors are antibodies and nanobodies that antagonize or potentiate gating of P2X7. Their potential advantages over small-molecule drugs include high specificity, lower off-target effects, and tunable in vivo half-life (for a recent review see Koch-Nolte et al., 2019). Therapeutic antibodies are commonly injected systemically to maximize delivery; however, they can be also administered as aerosols to treat respiratory tract and lung diseases (Van Heeke et al., 2017). In addition, genetic fusion of P2X7-specific biologics to binding modules may enable targeting of specific cell subsets; besides, directly modulating P2X7 function, antibodies can also initiate specific depletion of P2X7-expressing cells (Koch-Nolte et al., 2019). Furthermore, adeno-associated viral vectors can be used to express P2X7-specific antibodies in vivo to achieve long-lasting biological effects and enable modulation of the function of P2X7-expressing immune cells via encoded transgenic RNA or proteins (Koch-Nolte et al., 2019). Indeed, functional antibodies and nanobodies have already shown promising therapeutic benefit in animal models of sterile inflammation (Menzel et al., 2018).
Conclusions
The present review provides an outlook about the role of P2X7 receptors in CVDs and their dual function as a cationic channel and as a precursor of large pore formation. It summarizes a wealth of evidence demonstrating that inhibition of P2X7 receptors promotes neuron and glia protection against brain injury and that they can be essential for the release of cell-supporting factors. At the same time, these receptors are also relevant to preconditioning and post-conditioning and, therefore, emerge as possible targets to attenuate tissue damage in CVDs and modulate neuroinflammation to constrain the expansion of the core lesion into the penumbra.
New, groundbreaking research on therapeutic targeting on P2X7 receptors is constantly being made available. A notable example is the relatively recent discovery of a structural basis for subtype-specific inhibition, which provides novel mechanistic insights to facilitate the development of P2X7-specific drugs for treating human diseases (Karasawa and Kawate, 2016). Moreover, new data on the structural and functional properties, in combination with cell-based functional studies, suggest that the P2X7 receptor itself constitutes a lipid-composition-dependent, dye-permeable pore, whose opening is facilitated by palmitoylated cysteines near the pore-lining helix (Karasawa et al., 2017).
In summary, P2X7 receptors contribute to neurotransmission and glia signaling using Ca2+ as a key second messenger (Figure 2) although the precise mechanisms mediating their effects in neurons and glial cells are still unclear. In mild CVDs, P2X7 receptors are involved directly or indirectly in preconditioning or post-conditioning by conditions by promoting the release of protective factors, such as HIF-1 (Hirayama et al., 2015), resulting in a pro-survival cascade against subsequent harmful events in neurons and possibly in oligodendrocytes. Finally, in severe CVDs, P2X7 receptors promote pore formation, thus allowing the efflux of large molecules including ATP and Ca2+ influx causing cytosolic Ca2+ overload and generating a detrimental feedback loop that ultimately results in neuronal and oligodendroglial death with the consequent demyelination along with astrocytic and microglial activation as well as pro-inflammatory cytokine release. Further work is warranted to elucidate the exact underlying mechanisms of the P2X7 receptor in the pathophysiology of CVDs and to shed light on therapies that simultaneously target multiple cell types and mechanisms of injury in these diseases.
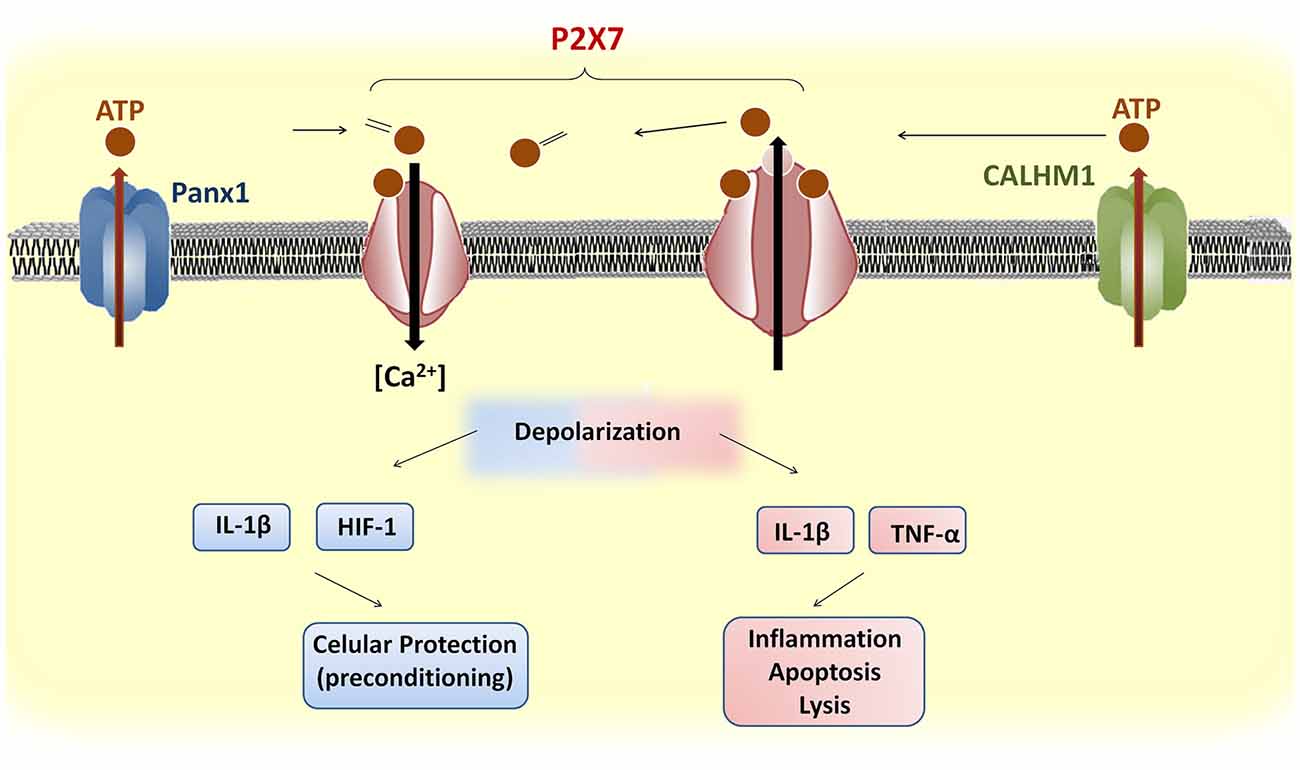
Figure 2. Upstream and downstream events of P2X7 receptor activation in brain cells. ATP release through Panx1 or CALHM1 activates P2X7 receptors, which induces influx of Ca2+. The prolonged stimulation of P2X7 receptors induces pore formation and further ATP release. Brief depolarization during mild cerebrovascular diseases (CVDs) may promote the release of protective factors, such as hypoxia-inducible factor (HIF)-1, that confer cellular protection against subsequent ischemic stimuli. In contrast, severe CVDs trigger a sustained P2X7 receptor depolarization and the release of pro-inflammatory cytokines that cause apoptosis or lysis.
Author Contributions
AC-M and CM conceived and described the initial draft of the manuscript. AC-M prepared graphic material. AP-S, MD, RA, MG, and FK-N contributed numerous comments and suggestions to the final manuscript.
Funding
This study was supported by grants from CONACYT-México No. 252121 and PAPIITUNAM-México No. IN203519 to ROA laboratory; by Spanish Ministry of Education and Science/FEDER (SAF2016-75292-R), Basque Government (IT1203/19), CIBERNED, Eranet-Neuron and Universidad del País Vasco to CM’s laboratory. AC-M is a researcher from Cátedras-CONACYT commissioned at Instituto de Neurobiología at Universidad Nacional Autónoma de México (UNAM).
Conflict of Interest
The authors declare that the research was conducted in the absence of any commercial or financial relationships that could be construed as a potential conflict of interest.
Acknowledgments
We thank Dr. Edith Garay and Saioa Marcos for technical assistance.
References
Agresti, C., Meomartini, M. E., Amadio, S., Ambrosini, E., Serafini, B., Franchini, L., et al. (2005). Metabotropic P2 receptor activation regulates oligodendrocyte progenitor migration and development. Glia 50, 132–144. doi: 10.1002/glia.20160
Alberto, A. V. P., Faria, R. X., Couto, C. G. C., Ferreira, L. G. B., Souza, C. A. M., Teixeira, P. C. N., et al. (2013). Is pannexin the pore associated with the P2X7 receptor? Naunyn Schmiedebergs Arch. Pharmacol. 386, 775–787. doi: 10.1007/s00210-013-0868-x
Arbeloa, J., Pérez-Samartín, A., Gottlieb, M., and Matute, C. (2012). P2X7 receptor blockade prevents ATP excitotoxicity in neurons and reduces brain damage after ischemia. Neurobiol. Dis. 45, 954–961. doi: 10.1016/j.nbd.2011.12.014
Ballerini, P., Rathbone, M. P., Di, P. I., Renzetti, A., Giuliani, P., D’Alimonte, I., et al. (1996). Rat astroglial P2Z (P2X7) receptors regulate intracellular calcium and purine release. Neuroreport 7, 2533–2537. doi: 10.1097/00001756-199611040-00026
Baroja-Mazo, A., Barberà-Cremades, M., and Pelegrín, P. (2013). The participation of plasma membrane hemichannels to purinergic signaling. Biochim. Biophys. Acta 1828, 79–93. doi: 10.1016/j.bbamem.2012.01.002
Bartlett, R., Yerbury, J. J., and Sluyter, R. (2013). P2X7 receptor activation induces reactive oxygen species formation and cell death in murine EOC13 microglia. Mediators Inflamm. 2013:271813. doi: 10.1155/2013/271813
Bianco, F., Ceruti, S., Colombo, A., Fumagalli, M., Ferrari, D., Pizzirani, C., et al. (2006). A role for P2X7 in microglial proliferation. J. Neurochem. 99, 745–758. doi: 10.1111/j.1471-4159.2006.04101.x
Braun, M., Vaibhav, K., Saad, N. M., Fatima, S., Vender, J. R., Baban, B., et al. (2017). White matter damage after traumatic brain injury: a role for damage associated molecular patterns. Biochim. Biophys. Acta Mol. Basis Dis. 1863, 2614–2626. doi: 10.1016/j.bbadis.2017.05.020
Braun, N., Zhu, Y., Krieglstein, J., Culmsee, C., and Zimmermann, H. (1998). Upregulation of the enzyme chain hydrolyzing extracellular ATP after transient forebrain ischemia in the rat. J. Neurosci. 18, 4891–4900. doi: 10.1523/jneurosci.18-13-04891.1998
Chafke, Y., Seguin, R., Antel, J. P., Morissette, C., Malo, D., Henderson, D., et al. (2002). ADP and AMP induce interleukin-1β release from microglial cells through activation of ATP-primed P2X7 receptor channels. J. Neurosci. 22, 3061–3069. doi: 10.1523/jneurosci.22-08-03061.2002
Chen, Q., Jin, Y., Zhang, K., Li, H., Chen, W., Meng, G., et al. (2014). Alarmin HNP-1 promotes pyroptosis and IL-1β release through different roles of NLRP3 inflammasome via P2X7 in LPS-primed macrophages. Innate Immun. 20, 290–300. doi: 10.1177/1753425913490575
Chen, S., Ma, Q., Krafft, P. R., Chen, Y., Tang, J., Zhang, J., et al. (2013). P2X7 receptor antagonism inhibits p38 MAPK activation and ameliorates neuronal apoptosis after subarachnoid hemorrhage in the rat. Crit. Care Med. 41, e466–e474. doi: 10.1097/ccm.0b013e31829a8246
Chu, K., Yin, B., Wang, J., Peng, G., Liang, H., Xu, Z., et al. (2012). Inhibition of P2X7 receptor ameliorates transient global cerebral ischemia/reperfusion injury via modulating inflammatory responses in the rat hippocampus. J. Neuroinflammation 9:69. doi: 10.1186/1742-2094-9-69
Cisneros-Mejorado, A., Gottlieb, M., Cavaliere, F., Magnus, T., Koch-Nolte, F., Scemes, E., et al. (2015a). Blockade of P2X7 receptors or pannexin-1 channels similarly attenuates postischemic damage. J. Cereb. Blood Flow Metab. 35, 843–850. doi: 10.1038/jcbfm.2014.262
Cisneros-Mejorado, A., Pérez-Samartín, A., Gottlieb, M., and Matute, C. (2015b). ATP signaling in brain: release, excitotoxicity and potential therapeutic targets. Cell. Mol. Neurobiol. 35, 1–6. doi: 10.1007/s10571-014-0092-3
Cisneros-Mejorado, A., Gottlieb, M., Ruiz, A., Chara, J. C., Pérez-Samartín, A., Marambaud, P., et al. (2018). Blockade and knock-out of CALHM1 channels attenuate ischemic brain damage. J. Cereb. Blood Flow Metab. 38, 1060–1069. doi: 10.1177/0271678x17713587
Cohen, J. A., and Tesar, P. J. (2017). Clemastine fumarate for promotion of optic nerve remyelination. Lancet 390, 2421–2424. doi: 10.1016/s0140-6736(17)32639-9
Collo, G., Neidhart, S., Kawashima, E., Kosco-Vilbois, M., North, R. A., and Buell, G. (1997). Tissue distribution of the P2X7 receptor. Neuropharmacology 36, 1277–1283. doi: 10.1016/s0028-3908(97)00140-8
Dale, N., and Frenguelli, B. G. (2009). Release of adenosine and ATP during ischemia and epilepsy. Current Neuropharmacol. 7, 160–179. doi: 10.2174/157015909789152146
Danquah, W., Meyer-Schwesinger, C., Rissiek, B., Pinto, C., Serracant-Prat, A., Amadi, M., et al. (2016). Nanobodies that block gating of the P2X7 ion channel ameliorate inflammation. Sci. Transl. Med. 8:366ra162. doi: 10.1126/scitranslmed.aaf8463
Di Virgilio, F., Schmalzing, G., and Markwardt, F. (2018). The elusive P2X7 macropore. Trends Cell Biol. 28, 392–404. doi: 10.1016/j.tcb.2018.01.005
Díaz-Hernández, M., Díez-Zaera, M., Sánchez-Nogueiro, J., Gómez-Villafuertes, R., Canals, J. M., Alberch, J., et al. (2009). Altered P2X7-receptor level and function in mouse models of Huntington’s disease and therapeutic efficacy of antagonist administration. FASEB J. 23, 1893–1906. doi: 10.1096/fj.08-122275
Domercq, M., Perez-Samartin, A., Aparicio, D., Alberdi, E., Pampliega, O., and Matute, C. (2010). P2X7 receptors mediate ischemic damage to oligodendrocytes. Glia 58, 730–740. doi: 10.1002/glia.20958
Duan, S., Anderson, C. M., Keung, E. C., Chen, Y., Chen, Y., and Swanson, R. A. (2003). P2X7 receptor-mediated release of excitatory amino acids from astrocytes. J. Neurosci. 23, 1320–1328. doi: 10.1523/jneurosci.23-04-01320.2003
Duplantier, A. J., Dombroski, M. A., Subramanyam, C., Beaulieu, A. M., Chang, S. P., Gabel, C. A., et al. (2011). Optimization of the physicochemical and pharmacokinetic attributes in a 6-azauracil series of P2X7 receptor antagonists leading to the discovery of the clinical candidate CE-224,535. Bioorg. Med. Chem. Lett. 21, 3708–3711. doi: 10.1016/j.bmcl.2011.04.077
Ferrari, D., Chiozzi, P., Falzoni, S., Dal Susino, M., Collo, G., Buell, G., et al. (1997). ATP-mediated cytotoxicity in microglial cells. Neuropharmacology 36, 1295–1301. doi: 10.1016/s0028-3908(97)00137-8
Ferrari, D., Villalba, M., Chiozzi, P., Falzoni, S., Ricciardi-Castagnoli, P., and Di Virgilio, F. (1996). Mouse microglial cells express a plasma membrane pore gated by extracellular ATP. J. Immunol. 156, 1531–1539.
Franke, H., Grosche, J., Schädlich, H., Krügel, U., Allgaier, C., and Illes, P. (2001). P2X receptor expression on astrocytes in the nucleus accumbens of rats. Neuroscience 108, 421–429. doi: 10.1016/s0306-4522(01)00416-x
Giuliani, A. L., Sarti, A. C., Falzoni, S., and Di Virgilio, F. (2017). The P2X7 receptor-interleukin-1 liaison. Front. Pharmacol. 8:123. doi: 10.3389/fphar.2017.00123
Helliwell, R. M., ShioukHuey, C. O., Dhuna, K., Molero, J. C., Ye, J. M., Xue, C. C., et al. (2015). Selected ginsenosides of the protopanaxdiol series are novel positive allosteric modulators of P2X7 receptors. Br. J. Pharmacol. 172, 3326–3340. doi: 10.1111/bph.13123
Hide, I., Tanaka, M., Inoue, A., Nakajima, K., Kohsaka, S., Inoue, K., et al. (2000). Extracellular ATP triggers tumor necrosis factor-α release from rat microglia. J. Neurochem. 75, 965–972. doi: 10.1046/j.1471-4159.2000.0750965.x
Hirayama, Y., Ikeda-Matsuo, Y., Notomi, S., Enaida, H., Kinouchi, H., and Koizumi, S. (2015). Astrocyte-mediated ischemic tolerance. J. Neurosci. 35, 3794–3805. doi: 10.1523/jneurosci.4218-14.2015
Honore, P., Donnelly-Roberts, D., Namovic, M. T., Hsieh, G., Zhu, C. Z., Mikusa, J. P., et al. (2006). A-740003, a novel and selective P2X 7 receptor antagonist, dose-dependently reduces neuropathic pain in rat. J. Pharmacol. Exp. Ther. 319, 1376–1385. doi: 10.1124/jpet.106.111559
Iglesias, R., Dahl, G., Qiu, F., Spray, D. C., and Scemes, E. (2009). Pannexin 1: the molecular substrate of astrocyte “hemichannels”. J. Neurosci. 29, 7092–7097. doi: 10.1523/jneurosci.6062-08.2009
Illes, P., Khan, T. M., and Rubini, P. (2017). Neuronal P2X7 receptors revisited: do they really exist? J. Neurosci. 37, 7049–7062. doi: 10.1523/jneurosci.3103-16.2017
Jachova, J., Gottlieb, M., Nemethova, M., Macakova, L., Bona, M., and Bonova, P. (2019). Neuroprotection mediated by remote preconditioning is associated with a decrease in systemic oxidative stress and changes in brain and blood glutamate concentration. Neurochem. Int. 129:104461. doi: 10.1016/j.neuint.2019.05.005
James, G., and Butt, A. M. (2001). P2X and P2Y purinoreceptors mediate ATP-evoked calcium signalling in optic nerve glia in situ. Cell Calcium 30, 251–259. doi: 10.1054/ceca.2001.0232
Janks, L., Sharma, C. V., and Egan, T. M. (2018). A central role for P2X7 receptors in human microglia. J. Neuroinflammation 15:325. doi: 10.1186/s12974-018-1353-8
Janssen, B., Vugts, D. J., Funke, U., Spaans, A., Schuit, R. C., Kooijman, E., et al. (2014). Synthesis and initial preclinical evaluation of the P2X7 receptor antagonist [11C] A-740003 as a novel tracer of neuroinflammation. J. Labelled Comp. Radiopharm. 57, 509–516. doi: 10.1002/jlcr.3206
Jurányi, Z., Sperlágh, B., and Vizi, E. S. (1999). Involvement of P2 purinoceptors and the nitric oxide pathway in [3H] purine outflow evoked by short-term hypoxia and hypoglycemia in rat hippocampal slices. Brain Res. 823, 183–190. doi: 10.1016/s0006-8993(99)01169-5
Karasawa, A., and Kawate, T. (2016). Structural basis for subtype-specific inhibition of the P2X7 receptor. Elife 5:e22153. doi: 10.7554/eLife.22153
Karasawa, A., Michalski, K., Mikhelzon, P., and Kawate, T. (2017). The P2X7 receptor forms a dye-permeable pore independent of its intracellular domain but dependent on membrane lipid composition. Elife 6:e31186. doi: 10.7554/eLife.31186
Keystone, E. C., Wang, M. M., Layton, M., Hollis, S., McInnes, I. B., and D1520C00001 Study Team. (2012). Clinical evaluation of the efficacy of the P2X7 purinergic receptor antagonist AZD9056 on the signs and symptoms of rheumatoid arthritis in patients with active disease despite treatment with methotrexate or sulphasalazine. Ann. Rheum. Dis. 71, 1630–1635. doi: 10.1136/annrheumdis-2011-143578
Kim, M., Jiang, L. H., Wilson, H. L., North, R. A., and Surprenant, A. (2001). Proteomic and functional evidence for a P2X7 receptor signalling complex. EMBO J. 20, 6347–6358. doi: 10.1093/emboj/20.22.6347
Koch-Nolte, F., Eichhoff, A., Pinto-Espinoza, C., Schwarz, N., Schäfer, T., Menzel, S., et al. (2019). Novel biologics targeting the P2X7 ion channel. Curr. Opin. Pharmacol. 47, 110–118. doi: 10.1016/j.coph.2019.03.001
Kukley, M., Barden, J. A., Steinhäuser, C., and Jabs, R. (2001). Distribution of P2X receptors on astrocytes in juvenile rat hippocampus. Glia 36, 11–21. doi: 10.1002/glia.1091
Liu, J., Dupree, J. L., Gacias, M., Frawley, R., Sikder, T., Naik, P., et al. (2016). Clemastine enhances myelination in the prefrontal cortex and rescues behavioral changes in socially isolated mice. J. Neurosci. 36, 957–962. doi: 10.1523/JNEUROSCI.3608-15.2016
Liu, J., Ginis, I., Spatz, M., and Hallenbeck, J. M. (2000). Hypoxic preconditioning protects cultured neurons against hypoxic stress via TNF-α and ceramide. Am. J. Physiol. Cell Physiol. 278, C144–C153. doi: 10.1152/ajpcell.2000.278.1.c144
Locovei, S., Scemes, E., Qiu, F., Spray, D. C., and Dahl, G. (2007). Pannexin1 is part of the pore forming unit of the P2X7 receptor death complex. FEBS Lett. 581, 483–488. doi: 10.1016/j.febslet.2006.12.056
Locovei, S., Wang, J., and Dahl, G. (2006). Activation of pannexin 1 channels by ATP through P2Y receptors and by cytoplasmic calcium. FEBS Lett. 580, 239–244. doi: 10.1016/j.febslet.2005.12.004
MacVicar, B. A., and Thompson, R. J. (2010). Non-junction functions of pannexin-1 channels. Trends Neurosci. 33, 93–102. doi: 10.1016/j.tins.2009.11.007
Maturana, C. J., Aguirre, A., and Sáez, J. C. (2017). High glucocorticoid levels during gestation activate the inflammasome in hippocampal oligodendrocytes of the offspring. Dev. Neurobiol. 77, 625–642. doi: 10.1002/dneu.22409
Matute, C., Torre, I., Pérez-Cerdá, F., Pérez-Samartín, A., Alberdi, E., Etxebarria, E., et al. (2007). P2X7 receptor blockade prevents ATP excitotoxicity in oligodendrocytes and ameliorates experimental autoimmune encephalomyelitis. J. Neurosci. 27, 9525–9533. doi: 10.1523/jneurosci.0579-07.2007
Melani, A., Turchi, D., Vannucchi, M. G., Cipriani, S., Gianfriddo, M., and Pedata, F. (2005). ATP extracellular concentrations are increased in the rat striatum during in vivo ischemia. Neurochem. Int. 47, 442–448. doi: 10.1016/j.neuint.2005.05.014
Menzel, S., Schwarz, N., Haag, F., and Koch-Nolte, F. (2018). Nanobody-based biologics for modulating purinergic signaling in inflammation and immunity. Front. Pharmacol. 9:266. doi: 10.3389/fphar.2018.00266
Miras-Portugal, M. T., Sebastián-Serrano, Á., de Diego García, L., and Díaz-Hernández, M. (2017). Neuronal P2X7 receptor: involvement in neuronal physiology and pathology. J. Neurosci. 37, 7063–7072. doi: 10.1523/jneurosci.3104-16.2017
Monif, M., Reid, C. A., Powell, K. L., Smart, M. L., and Williams, D. A. (2009). The P2X7 receptor drives microglial activation and proliferation: a trophic role for P2X7R pore. J. Neurosci. 29, 3781–3791. doi: 10.1523/jneurosci.5512-08.2009
Murphy, N., Cowley, T. R., Richardson, J. C., Virley, D., Upton, N., Walter, D., et al. (2012). The neuroprotective effect of a specific P2X-receptor antagonist derives from it ability to inhibit assembly of the NLRP3 inflammasome in glial cells. Brain Pathol. 22, 295–306. doi: 10.1111/j.1750-3639.2011.00531.x
Nörenberg, W., Hempel, C., Urban, N., Sobottka, H., Illes, P., and Schaefer, M. (2011). Clemastine potentiates the human P2X7 receptor by sensitizing it to lower ATP concentrations. J. Biol. Chem. 286, 11067–11081. doi: 10.1074/jbc.m110.198879
North, R. A. (2002). Molecular physiology of P2X receptors. Physiol. Rev. 82, 1013–1067. doi: 10.1152/physrev.00015.2002
Orellana, J. A., Moraga-Amaro, R., Díaz-Galarce, R., Rojas, S., Maturana, C. J., Stehberg, J., et al. (2015). Restraint stress increases hemichannel activity in hippocampal glial cells and neurons. Front. cell. neurosci. 9:102. doi: 10.3389/fncel.2015.00102
Ou, A., Gu, B. J., and Wiley, J. S. (2018). The scavenger activity of the human P2X7 receptor differs from P2X7 pore function by insensitivity to antagonists, genetic variation and sodium concentration: relevance to inflammatory brain diseases. Biochim. Biophys. Acta 1864, 1051–1059. doi: 10.1016/j.bbadis.2018.01.012
Panenka, W., Jijon, H., Herx, L. M., Armstrong, J. N., Feighan, D., Wei, T., et al. (2001). P2X7-like receptor activation in astrocytes increases chemokine monocyte chemoattractant protein-1 expression via mitogen-activated protein kinase. J. Neurosci. 21, 7135–7142. doi: 10.1523/jneurosci.21-18-07135.2001
Pippel, A., Stolz, M., Woltersdorf, R., Kless, A., Schmalzing, G., and Markwardt, F. (2017). Localization of the gate and selectivity filter of the full-length P2X7 receptor. Proc. Natl. Acad. Sci. U S A 114, E2156–E2165. doi: 10.1073/pnas.1610414114
Qu, Y., Misaghi, S., Newton, K., Gilmour, L. L., Louie, S., Cupp, J. E., et al. (2011). Pannexin-1 is required for ATP release during apoptosis but not for inflammasome activation. J. Immunol. 186, 6553–6561. doi: 10.4049/jimmunol.1100478
Riedel, T., Schmalzing, G., and Markwardt, F. (2007). Influence of extracellular monovalent cations on pore and gating properties of P2X7 receptor-operated single-channel currents. Biophys. J. 93, 846–858. doi: 10.1529/biophysj.106.103614
Rossi, D. J., Brady, J. D., and Mohr, C. (2007). Astrocyte metabolism and signaling during brain ischemia. Nat. Neurosci. 10, 1377–1386. doi: 10.1038/nn2004
Ruscher, K., Isaev, N., Trendelenburg, G., Weih, M., Iurato, L., Meisel, A., et al. (1998). Induction of hypoxia inducible factor 1 by oxygen glucose deprivation is attenuated by hypoxic preconditioning in rat cultured neurons. Neurosci. Lett. 254, 117–120. doi: 10.1016/s0304-3940(98)00688-0
Sanz, J. M., and Di Virgilio, F. (2000). Kinetics and mechanism of ATP-dependent IL-1β release from microglial cells. J. Immunol. 164, 4893–4898. doi: 10.4049/jimmunol.164.9.4893
Shieh, C. H., Heinrich, A., Serchov, T., van Calker, D., and Biber, K. (2014). P2X7-dependent, but differentially regulated release of IL-6, CCL2, and TNF-α in cultured mouse microglia. Glia 62, 592–607. doi: 10.1002/glia.22628
Suadicani, S. O., Brosnan, C. F., and Scemes, E. (2006). P2X7 receptors mediate ATP release and amplification of astrocytic intercellular Ca2+ signaling. J. Neurosci. 26, 1378–1385. doi: 10.1523/jneurosci.3902-05.2006
Sun, S. H., Lin, L. B., Hung, A. C., and Kuo, J. S. (1999). ATP-stimulated Ca2+ influx and phospholipase D activities of a rat brain-derived type-2 astrocyte cell line, RBA-2, are mediated through P2X7 receptors. J. Neurochem. 73, 334–343. doi: 10.1046/j.1471-4159.1999.0730334.x
Suzuki, T., Hide, I., Ido, K., Kohsaka, S., Inoue, K., and Nakata, Y. (2004). Production and release of neuroprotective tumor necrosis factor by P2X7 receptor-activated microglia. J. Neurosci. 24, 1–7. doi: 10.1523/jneurosci.3792-03.2004
Takeuchi, H., Jin, S., Wang, J., Zhang, G., Kawanokuchi, J., Kuno, R., et al. (2006). Tumor necrosis factor-α induces neurotoxicity via glutamate release from hemichannels of activated microglia in an autocrine manner. J. Biol. Chem. 281, 21362–21368. doi: 10.1074/jbc.M600504200
Truelsen, T., Begg, S., and Colin, M. (2000). The Global Burden of Cerebrovascular Disease. Geneva: World Health Organisation.
Van Heeke, G., Allosery, K., De Brabandere, V., De Smedt, T., Detalle, L., and de Fougerolles, A. (2017). Nanobodies® as inhaled biotherapeutics for lung diseases. Pharmacol. Ther. 169, 47–56. doi: 10.1016/j.pharmthera.2016.06.012
Verderio, C., and Matteoli, M. (2001). ATP mediates calcium signaling between astrocytes and microglial cells: modulation by IFN-γ. J. Immunol. 166, 6383–6391. doi: 10.4049/jimmunol.166.10.6383
Visentin, S., Renzi, M., Frank, C., Greco, A., and Levi, G. (1999). Two different ionotropic receptors are activated by ATP in rat microglia. J. Physiol. 519, 723–736. doi: 10.1111/j.1469-7793.1999.0723n.x
Wang, L. Y., Cai, W. Q., Chen, P. H., Deng, Q. Y., and Zhao, C. M. (2009). Downregulation of P2X7 receptor expression in rat oligodendrocyte precursor cells after hypoxia ischemia. Glia 57, 307–319. doi: 10.1002/glia.20758
Yan, Z., Khadra, A., Li, S., Tomić, M., Sherman, A., and Stojilkovic, S. S. (2010). Experimental characterization and mathematical modeling of P2X7 receptor channel gating. J. Neurosci. 30, 14213–14224. doi: 10.1523/jneurosci.2390-10.2010
Yenari, M. A., Kauppinen, T. M., and Swanson, R. A. (2010). Microglial activation in stroke: therapeutic targets. Neurotherapeutics 7, 378–391. doi: 10.1016/j.nurt.2010.07.005
Yu, Y., Ugawa, S., Ueda, T., Ishida, Y., Inoue, K., Nyunt, A. K., et al. (2008). Cellular localization of P2X7 receptor mRNA in the rat brain. Brain Res. 1194, 45–55. doi: 10.1016/j.brainres.2007.11.064
Zhao, J. H., Meng, X. L., Zhang, J., Li, Y. L., Li, Y. J., and Fan, Z. M. (2014). Oxygen glucose deprivation post-conditioning protects cortical neurons against oxygen-glucose deprivation injury: role of HSP70 and inhibition of apoptosis. J. Huazhong Univ. Sci. Technol. Med. Sci. 34, 18–22. doi: 10.1007/s11596-014-1225-0
Keywords: ATP, pannexin-1, ischemia, neuron, oligodendrocyte
Citation: Cisneros-Mejorado AJ, Pérez-Samartín A, Domercq M, Arellano RO, Gottlieb M, Koch-Nolte F and Matute C (2020) P2X7 Receptors as a Therapeutic Target in Cerebrovascular Diseases. Front. Mol. Neurosci. 13:92. doi: 10.3389/fnmol.2020.00092
Received: 01 March 2020; Accepted: 05 May 2020;
Published: 18 June 2020.
Edited by:
Miguel Diaz-Hernandez, Complutense University of Madrid, SpainReviewed by:
Francesco Di Virgilio, University of Ferrara, ItalyJuan C. Saez, Pontificia Universidad Católica de Chile, Chile
Robson Coutinho-Silva, Federal University of Rio de Janeiro, Brazil
Copyright © 2020 Cisneros-Mejorado, Pérez-Samartín, Domercq, Arellano, Gottlieb, Koch-Nolte and Matute. This is an open-access article distributed under the terms of the Creative Commons Attribution License (CC BY). The use, distribution or reproduction in other forums is permitted, provided the original author(s) and the copyright owner(s) are credited and that the original publication in this journal is cited, in accordance with accepted academic practice. No use, distribution or reproduction is permitted which does not comply with these terms.
*Correspondence: Abraham J. Cisneros-Mejorado, abraham.cisneros.mejorado@gmail.com; Carlos Matute, carlos.matute@ehu.es