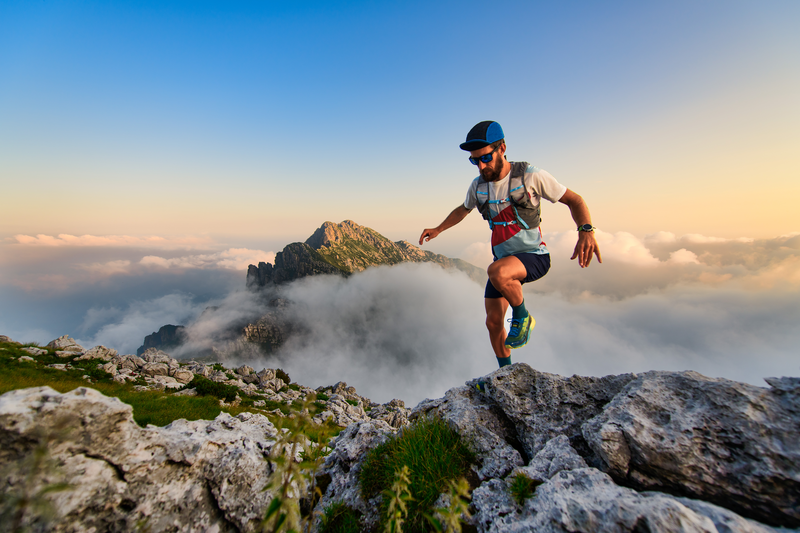
95% of researchers rate our articles as excellent or good
Learn more about the work of our research integrity team to safeguard the quality of each article we publish.
Find out more
MINI REVIEW article
Front. Mol. Neurosci. , 05 November 2019
Sec. Neuroplasticity and Development
Volume 12 - 2019 | https://doi.org/10.3389/fnmol.2019.00266
This article is part of the Research Topic Emerging Mechanisms in Dynamic GABAergic Inhibition View all 16 articles
The axon initial segment (AIS) is the site of action potential (AP) initiation in most neurons and is thus a critical site in the regulation of neuronal excitability. Normal function within the discrete AIS compartment requires intricate molecular machinery to ensure the proper concentration and organization of voltage-gated and ligand-gated ion channels; in humans, dysfunction at the AIS due to channel mutations is commonly associated with epileptic disorders. In this review, we will examine the molecular mechanisms underlying the formation of the only synapses found at the AIS: synapses containing γ-aminobutyric type A receptors (GABAARs). GABAARs are heteropentamers assembled from 19 possible subunits and are the primary mediators of fast synaptic inhibition in the brain. Although the total GABAAR population is incredibly heterogeneous, only one specific GABAAR subtype—the α2-containing receptor—is enriched at the AIS. These AIS synapses are innervated by GABAergic chandelier cells, and this inhibitory signaling is thought to contribute to the tight control of AP firing. Here, we will summarize the progress made in understanding the regulation of GABAAR synapse formation, concentrating on post-translational modifications of subunits and on interactions with intracellular proteins. We will then discuss subtype-specific synapse formation, with a focus on synapses found at the AIS, and how these synapses influence neuronal excitation.
The firing of glutamatergic pyramidal cells is tightly controlled by inhibitory interneurons (INs). By precisely directing pyramidal cell activity, INs are able to regulate network activity, generate oscillations, and even terminate pathological hyperexcitability (Fritschy, 2008; Roux and Buzsáki, 2015). On a molecular level, INs regulate pyramidal cell firing through GABAergic neurotransmission: releasing the neurotransmitter γ-aminobutyric acid (GABA) onto inhibitory postsynaptic specializations containing GABA type A receptors (GABAARs) on pyramidal neuron dendrites, soma, and axon initial segments (AISs). Thus, the construction and maintenance of GABAergic synapses are essential for normal inhibitory neurotransmission and brain function. However, relatively little is known about inhibitory synaptogenesis compared to glutamatergic synapses. To complicate the picture, there are many GABAAR subtypes composed of different subunits, which confer distinct physiological properties on the receptors. In addition, different GABAAR subtypes are selectively stabilized at different types of synapses; the AIS, for example, contains primarily one kind of GABAAR. Thus, the type of receptor present at a given synapse determines the type of inhibition that takes place. Again, little is known about how neurons direct different types of GABAARs to different synapses. The following review will briefly summarize what is known about the formation and trafficking of GABAAR subtypes and the construction of inhibitory synapses overall and specifically at the AIS.
In the adult mammalian central nervous system, most fast, synaptic inhibitory neurotransmission is mediated by GABAARs, a group of heteropentameric, ligand-gated anion channels (Connolly and Wafford, 2004). When the neurotransmitter GABA binds to the receptor, the intrinsic ion pore opens and allows permeable ions to pass through (Bormann et al., 1987). GABAARs are primarily permeable to chloride (Cl−) anions (Fatima-Shad and Barry, 1993), and in the mature brain—where the Cl− reversal potential is more negative than the resting membrane potential—the opening of the GABAAR channel allows Cl− ions to flow down their electrochemical gradient into the neuron, lowering the neuron’s membrane potential and producing a hyperpolarizing response that reduces the probability of action potential (AP) firing (Busch and Sakmann, 1990; Blaesse et al., 2009).
Structurally, GABAARs are diverse. The receptors are assembled from 19 different known subunits: α(1–6), β(1–3), γ(1–3), δ, ε, θ, π, and ρ(1–3; Olsen and Sieghart, 2008), putting the number of possible subunit combinations in the thousands; however, only certain subtypes are expressed in the brain. For synaptic GABAARs, which this review will focus on, the typical stoichiometric ratio is as follows: 2α:2β:1γ (Wisden et al., 1992; Baumann et al., 2003). GABAAR subunits possess a similar amino acid sequence and protein structure, with each subunit composed of an extracellular N-terminal domain, four transmembrane domains (TM1–4), an intracellular loop domain (ICD) between TM3 and TM4, and an extracellular C-terminal domain (Schofield et al., 1987; Miller and Aricescu, 2014). The ICD is important for regulating GABAAR activity, as it is the site of phosphorylation and protein-protein interactions that alter receptor trafficking and plasma membrane (PM) expression (Moss et al., 1992; Nymann-Andersen et al., 2002; O’Toole and Jenkins, 2011). In addition, the ICD is the site of greatest sequence variability between subunits, making it an attractive candidate for a locus of subtype-specific GABAAR regulation (Arancibia-Cárcamo and Kittler, 2009). It seems likely that such differential regulation occurs, as different types of synaptic GABAARs are restricted to certain synapses. For instance, within pyramidal neurons in the cortex and hippocampus, GABAARs that contain the α1 subunit tend to be found at synapses in the soma and dendrites, while α2-containing GABAARs are enriched at synapses on the AIS (Nusser et al., 1996).
The subunit composition of a given GABAAR not only influences receptor localization, but also determines the physiological properties of that receptor (see Table 1 for summary). In addition, the specific α subunit composition of GABAARs determines receptor kinetics. α1-GABAARs mediate an inhibitory current with a longer decay time than α2-GABAARs (Goldstein et al., 2002). Thus, GABAAR subtypes mediate specific kinds of inhibition; restricting GABAAR subtypes to different spatial domains allows INs to control pyramidal neuron firing in a precise but dynamic manner.
Table 1. The distribution and synaptic roles of γ-aminobutyric acid type A receptor (GABAAR) α subunits.
GABAAR subunits are assembled into receptors in the endoplasmic reticulum (ER; Kittler et al., 2002). Oligomerization is controlled by the subunits’ N-terminal domains, with assistance from resident ER chaperone proteins to ensure appropriate protein assembly and folding (Connolly et al., 1996; Moss and Smart, 2001). Only those receptors that are conformationally mature are permitted to exit the ER and continue along the GABAAR lifecycle; receptors that are found to be incomplete or composed of inappropriate subunit combinations are retained in the ER and degraded (Gorrie et al., 1997; Saliba et al., 2007).
Conformationally mature GABAARs travel from the ER to the Golgi apparatus, where receptors are segregated into vesicles and transported to the PM (Vithlani et al., 2011). This forward trafficking delivers GABAARs to and insert them into the PM, primarily in extrasynaptic areas (Bogdanov et al., 2006). GABAAR surface expression is also regulated by receptor internalization via clathrin-mediated endocytosis (Lorenz-Guertin and Jacob, 2018). The clathrin adaptor protein (AP)-2 binds GABAAR subunits—the ICD of the GABAAR β1–3 and γ2 subunits both contain AP2 binding motifs—and clathrin, anchoring receptors in endocytotic pits.
GABAARs are inserted into the PM at extrasynaptic locations (Bogdanov et al., 2006). At the surface, GABAARs are highly dynamic and diffuse laterally within the PM, where they continually move between the synaptic and extrasynaptic space (Thomas et al., 2005). Recent single-particle trafficking experiments show that both synaptic (α1–3-containing) and extrasynaptic (α4–6-containing) receptors can access the inhibitory synapse; however, when within the synaptic domain, the diffusion rate of synaptic GABAARs was reduced relative to extrasynaptic receptors, suggesting that GABAARs with “synaptic” subunit compositions are selectively stabilized at synapses (Hannan et al., 2019).
How are these receptors stabilized in the inhibitory synapse? Research to date suggests that protein-protein interactions play an essential role in this process: structural proteins present at the inhibitory synapse bind to GABAARs, reducing their lateral diffusion rate and effectively anchoring them at the synapse (Hannan et al., 2019). Though the composition of the multimolecular protein complexes present at the inhibitory synapse remains relatively unknown, a number of proteins that reside at the inhibitory synapse and appear to regulate GABAAR clustering have been identified.
One of the first inhibitory synaptic proteins described was gephyrin (GPN), which is still considered to be an integral structural component of the inhibitory postsynaptic domain (Tyagarajan and Fritschy, 2014). The most common splice variant of GPN is composed of three domains: an N-terminal G domain, a linker C domain, and a C-terminal E domain (Feng et al., 1998; Schwarz et al., 2001). The E and G domains of GPN self-aggregate, leading to the hypothesized formation of hexameric macromolecular GPN complexes that could serve as a lattice to stabilize receptors at the synapse (Saiyed et al., 2007). GPN was first identified as a binding partner of glycine receptors, which mediate inhibition in the spine (Prior et al., 1992). Constitutive knock-out of GPN in the mouse leads to a complete loss of glycine receptor clusters in the periphery, resulting in early postnatal death (Feng et al., 1998). However, it was also found that GPN knock-out mice show a dramatic reduction in the presence of GABAARs at brain synapses, providing the first evidence that GPN is also crucial for inhibitory synapse formation in the central nervous system (Kneussel et al., 1999; Fischer et al., 2000).
More recent experiments have shown that GPN co-localizes with GABAARs containing α1–3 subunits at synapses (Sassoè-Pognetto et al., 2000). Isothermal titration calorimetry experiments performed with the GPN E domain and the ICDs of GABAAR α1–3 subunits have demonstrated that GPN interacts directly with the ICD of GABAAR α subunits at an amino acid stretch between ICD residues 360–375 (Hines et al., 2018). The amino acid sequence in this region is not well conserved between α subunit subtypes, thus it follows that GPN binds α1–3 with differing affinities: the α1 and α3 ICDs formed tight complexes with the GPN E domain, while the α2 ICD formed a comparatively weaker complex (Hines et al., 2018). These data suggest a GABAAR subtype-specific affinity for GPN, dependent on the amino acid composition of the 360–375 ICD motif of the α subunit and raise the possibility that GPN, or other proteins that bind the 360–375 motif, can selectively stabilize GABAAR subtypes at certain synapses.
A more recently identified inhibitory synapse protein is collybistin (CB), a guanine nucleotide exchange factor (Reid et al., 1999). Most functional CB isoforms are composed of three domains: a catalytic double homology domain, a PM-binding pleckstrin homology domain, and an N-terminal Src homology (SH)-3 domain (Harvey et al., 2004). CB was first identified as a GPN interacting protein (Kins et al., 2000). Indeed, the GPN E domain directly binds CB’s double homology domain, and co-expression of CB with GPN in heterologous cells causes the translocation of GPN clusters to the PM (Kins et al., 2000; Grosskreutz et al., 2001). CB knock-out mice show a loss of GPN clustering at inhibitory synapses in certain brain regions, such as the hippocampus, suggesting that CB plays a role in postsynaptic GPN clustering at a subset of inhibitory synapses (Papadopoulos et al., 2007, 2008).
Recent evidence showed that CB also directly interacts with certain GABAAR subtypes. Yeast tri-hybrid screens revealed that the GABAAR α2 subunit interacts with the CB SH3 domain, and in fact the GPN/CB interaction is strengthened by the addition of α2, suggesting that these three proteins can act synergistically (Saiepour et al., 2010). In vitro isothermal titration calorimetry showed that the CB SH3 domain preferentially binds the α2 ICD, over either the α1 or α3 ICD, at residues 360–375, suggesting that this ICD motif is integral to GABAAR subtype-specific protein-protein interactions (Hines et al., 2018). Supporting this hypothesis, knocking the α2 360–375 motif into the α1 subunit in mice leads to increased immunoprecipitation of endogenous CB with the chimeric α1 subunit (Nathanson et al., 2019). This same study also showed an increase in the pull-down of GPN with mutant α1, demonstrating a possible synergistic interaction between CB/GPN/α2 ICD that is overall strengthened when the interaction between two partner proteins is enhanced (Nathanson et al., 2019). The overarching question becomes: does this α2 ICD motif and its preferential protein interactions play a role in subtype-specific synapse formation in the brain, particularly in the construction of α2-enriched synapses at the AIS?
At the interface between the somatodendritic and axonal compartments lies the AIS. This discrete region is composed of unique molecular machinery and maintains a barrier between the somatodendritic and axonal environments, sustaining the neuronal anatomical asymmetry necessary for the unidirectional propagation of information (Leterrier, 2018). Morphologically, the AIS displays an electron-dense submembranous granular layer composed of a high density of voltage-gated ion channels and the highly organized, periodic protein scaffold that supports them (Xu et al., 2013). A number of electrophysiological studies established that the AIS is not only a barrier but is also the site of AP generation, as belied by its high resident concentrations of voltage-gated sodium and potassium channels, which are essential for the propagation of APs (Araki and Otani, 1955; Coombs et al., 1957; Fuortes et al., 1957).
Giant Ankyrin G is the key scaffolding protein and master organizer at the AIS; it recruits other essential AIS components, such as βIV-spectrin and voltage-gated ion channels, through either direct or indirect interactions (Zhou et al., 1998; Jenkins and Bennett, 2001; Han et al., 2017). Ankyrin G also interacts with microtubules, anchoring the entire complex in place (Leterrier et al., 2011). The AIS protein scaffold is dense and super-stable, maintaining axonal integrity and serving as a barrier to the entry of inappropriate somatodendritic proteins: the expression and/or stabilization of proteins at the AIS is tightly controlled (Albrecht et al., 2016; Huang and Rasband, 2016).
To current knowledge, the only ligand-gated ion channels mediating neurotransmission at the AIS are GABAARs (Leterrier, 2018). The AIS of certain cell types—pyramidal cells of the forebrain, for instance—contain inhibitory synapses that are exclusively innervated by one type of IN: the chandelier cell (Somogyi et al., 1983; Wang et al., 2016). Given that the AIS is the site of AP firing, any inhibitory signaling in this domain has an outsize effect on neuronal excitability (Zhu et al., 2004; Glickfeld et al., 2009). As previously discussed, α2-GABAARs are specifically enriched at the AIS (Nusser et al., 1996; Nyíri et al., 2001); since different GABAAR subtypes have their own kinetics and mediate distinct types of inhibition, it follows that the enrichment of a particular GABAAR subtype in a restricted domain like the AIS would have functional relevance.
To investigate the above hypothesis, mice in which residues 360–375 of the GABAAR α1 subunit have been knocked-in to the α2 subunit (Gabra2–1 mice) were generated. This mutation abolished α2’s preferential interaction with CB and led to loss of α2+ synapses at the AIS. Strikingly, Gabra2–1 animals display postnatal spontaneous seizures; these seizures are often lethal, causing death around postnatal day 20 (Hines et al., 2018). These data demonstrate that the localization of α2-GABAARs to the AIS is essential to inhibitory control of pathological excitation.
Clearly then, inhibition at the AIS is integral to maintaining the dynamic balance between inhibition and excitation. However, the manner in which GABAAR subtype-specific axo-axonic synapses are constructed and maintained remains unclear. Although α2-GABAARs are enriched at the AIS, live imaging of α1- and α2-GABAARs coupled to quantum dots showed that both subtypes can enter the AIS: the AIS diffusion barrier does not seem to select for α2-GABAARs (Muir and Kittler, 2014). However, these same studies demonstrated that α2-GABAARs were less mobile at the AIS than α1-GABAARs, indicating that while both subtypes can access the AIS compartment, α2-GABAARs are somehow preferentially anchored at there. Given that inhibitory synapse formation in other neuronal compartments has been shown to depend on protein-protein interactions, it stands to reason that synapse formation at the AIS would follow the same principles.
Indeed, GPN is expressed at the AIS, forming co-clusters with α2-GABAARs (Panzanelli et al., 2011), although GPN’s association with GABAARs at the AIS is relatively weaker than its association with GABAARs at the soma and dendrites (Gao and Heldt, 2016), suggesting that another protein present in the AIS multimolecular scaffold could play a more important role. CB is also present at AIS inhibitory synapses in cortical and hippocampal neurons (Panzanelli et al., 2011), and its specific interactions with α2-GABAARs provide a putative model for inhibitory synapse formation at the AIS: removing the 360–375 motif from the α2 subunit ICD prevents the accumulation of α2-GABAARs at axo-axonic synapses, suggesting that this motif, and the preferential protein interactions it mediates—such as that with CB—is necessary for GABAAR stabilization at the AIS (Hines et al., 2018). Experiments performed in another mutant mouse, in which residues 360–375 of the α2 subunit are knocked-in to the α1 subunit (the Gabra1–2 mouse), increases the affinity of the α1 subunit for CB and leads to an increase in α1-GABAARs expression at axo-axonic synapses. These data show that residues 360–375 of the α2 subunit are sufficient for GABAAR stabilization at the AIS (Nathanson et al., 2019). Given that CB has a relatively stronger association with the α2 360–375 motif and is present at the AIS, it stands to reason that CB interactions selectively stabilize α2-GABAARs at inhibitory AIS synapses.
Together, these data provide a potential model for axo-axonic synapse formation: after GABAARs are inserted into the extrasynaptic PM at the AIS those receptors that contain the α2 ICD motif are able to bind intracellular scaffolding proteins, such as CB, to form stable complexes that anchor the receptor at axo-axonic synapses. Receptors that do not contain the α2 ICD motif are not stabilized at synapses and diffuse back into the extrasynaptic space (see Figure 1). Other proteins in the AIS scaffold, especially Ankyrin G, might also play a role in the selective stabilization of GABAARs at the AIS. Future experiments utilizing the Gabra1–2 and Gabra2–1 mice could provide more information about the importance of these proteins in GABAAR stabilization. In addition, the above model only describes the postsynaptic side of inhibitory synapse formation. Additional mechanisms regulate the formation of presynaptic chandelier cell boutons apposing the AIS. Most recently, a transsynaptic mechanism was described: the cell adhesion molecule L1CAM, localized to the AIS of neocortical pyramidal neurons, was found to be necessary for the targeting of chandelier cell boutons to the AIS (Tai et al., 2019). Although the presynaptic interactor of L1CAM remains unidentified, such transsynaptic interactions provide an intriguing path for future research into synapse formation at the AIS.
Figure 1. The inhibitory postsynaptic specialization at the axon initial segment (AIS). A cartoon showing a putative model of the postsynaptic inhibitory synapse at the AIS in a hippocampal pyramidal neuron. Ankyrin G and the β4/α2-spectrin tetramer associate to stabilize voltage-gated ion channels and link the periodic domain to the actin and microtubule cytoskeleton. α2-GABAARs are enriched at inhibitory synapses at the AIS, where they are selectively stabilized by protein-protein interactions at their intracellular loop domain (ICD). This review proposes that collybistin is a candidate for an AIS selective stabilizer, linking the α2-GABAAR to the AIS plasma membrane (PM).
Despite the progress made in understanding the formation of inhibitory synapses, little is known about how neurons direct GABAAR subtype-specific synapse formation. This subtype specificity is important for the maintenance of neuronal excitability. α2-GABAAR-enriched synapse formation at the AIS is an especially intriguing case, as AIS inhibition is essential for normal brain function. Better understanding axo-axonic synapse formation will not only shed light on the molecular mechanisms of subtype-specific inhibitory synapse formation but may also provide new avenues of research into treatment for neurological disorders like epilepsy, which result from pathological hyperexcitability. It appears that protein-protein interactions between the ICD of GABAAR subunits and intracellular scaffolding proteins at inhibitory synapses play an important role in this process. The make-up of the inhibitory synaptic scaffold is variable depending on cell type and subcellular domain, making such interactions good candidates for synapse-specific GABAAR subtype enrichment. Further research will need to be done to fully explore the “interactome” of each GABAAR subtype and the importance of each interaction at the many different types of synapses present in even one neuron.
AN wrote the manuscript, with input from PD and SM.
This work was supported by funding from the National Institute of Mental Health (NIMH) grant MH097466.
SM serves as a consultant for AstraZeneca, Bain Capital, and Sage Therapeutics, relationships that are regulated by Tufts University. SM is also a shareholder of SAGE Therapeutics.
The remaining authors declare that the research was conducted in the absence of any commercial or financial relationships that could be construed as a potential conflict of interest.
The handling Editor declared a past co-authorship with one of the authors SM.
Albrecht, D., Winterflood, C. M., Sadeghi, M., Tschager, T., Noé, F., and Ewers, H. (2016). Nanoscopic compartmentalization of membrane protein motion at the axon initial segment. J. Cell Biol. 215, 37–46. doi: 10.1083/jcb.201603108
Araki, T., and Otani, T. (1955). Response of single motoneurons to direct stimulation in toad’s spinal cord. J. Neurophysiol. 18, 472–485. doi: 10.1152/jn.1955.18.5.472
Arancibia-Cárcamo, I. L., and Kittler, J. T. (2009). Regulation of GABAA receptor membrane trafficking and synaptic localization. Pharmacol. Ther. 123, 17–31. doi: 10.1016/j.pharmthera.2009.03.012
Baumann, S. W., Baur, R., and Sigel, E. (2003). Individual properties of the two functional agonist sites in GABAA receptors. J. Neurosci. 23, 11158–11166. doi: 10.1523/JNEUROSCI.23-35-11158.2003
Blaesse, P., Airaksinen, M. S., Rivera, C., and Kaila, K. (2009). Cation-chloride cotransporters and neuronal function. Neuron 61, 820–838. doi: 10.1016/j.neuron.2009.03.003
Bogdanov, Y., Michels, G., Armstrong-Gold, C., Haydon, P. G., Lindstrom, J., Pangalos, M., et al. (2006). Synaptic GABAA receptors are directly recruited from their extrasynaptic counterparts. EMBO J. 25, 4381–4389. doi: 10.1038/sj.emboj.7601309
Bormann, J., Hamill, O. P., and Sakmann, B. (1987). Mechanism of anion permeation through channels gated by glycine and γ-aminobutyric acid in mouse cultured spinal neurones. J. Physiol. 385, 243–286. doi: 10.1113/jphysiol.1987.sp016493
Busch, C., and Sakmann, B. (1990). Synaptic transmission in hippocampal neurons: numerical reconstruction of quantal IPSCs. Cold Spring Harb. Symp. Quant. Biol. 55, 69–80. doi: 10.1101/sqb.1990.055.01.009
Connolly, C. N., Krishek, B. J., McDonald, B. J., Smart, T. G., and Moss, S. J. (1996). Assembly and cell surface expression of heteromeric and homomeric γ-aminobutyric acid type A receptors. J. Biol. Chem. 271, 89–96. doi: 10.1074/jbc.271.1.89
Connolly, C. N., and Wafford, K. A. (2004). The Cys-Loop superfamily of ligand-gated ion channels: the impact of receptor structure on function. Biochem. Soc. Trans. 32, 529–534. doi: 10.1042/bst0320529
Coombs, J. S., Curtis, D. R., and Eccles, J. C. (1957). The generation of impulses in motoneurones. J. Physiol. 139, 232–249. doi: 10.1113/jphysiol.1957.sp005888
Fatima-Shad, K., and Barry, P. H. (1993). Anion permeation in GABA- and glycine-gated channels of mammalian cultured hippocampal neurons. Proc. R. Soc. Lond. B Biol. Sci. 253, 69–75. doi: 10.1098/rspb.1993.0083
Feng, G., Tintrup, H., Kirsch, J., Nichol, M. C., Kuhse, J., Betz, H., et al. (1998). Dual-requirement for gephyrin in glycine receptor clustering and molybdoenzyme activity. Science 282, 1321–1324. doi: 10.1126/science.282.5392.1321
Fritschy, J.-M. (2008). Epilepsy, E/I balance and GABAA receptor plasticity. Front. Mol. Neurosci. 1:5. doi: 10.3389/neuro.02.005.2008
Fischer, F., Kneussel, M., Tintrup, H., Haverkamp, S., Rauen, T., Betz, H., et al. (2000). Reduced synaptic clustering of GABA and glycine receptors in the retina of the gephyrin null mutant mouse. J. Comp. Neurol. 427, 634–648. doi: 10.1002/1096-9861(20001127)427:4<634::aid-cne10>3.0.co;2-x
Fuortes, M. G. F., Frank, K., and Becker, M. C. (1957). Steps in the production of motoneuron spikes. J. Gen. Physiol. 40, 735–752. doi: 10.1085/jgp.40.5.735
Gao, Y., and Heldt, S. A. (2016). Enrichment of GABAA receptor α-subunits on the axonal initial segment shows regional differences. Front. Cell. Neurosci. 10:39. doi: 10.3389/fncel.2016.00039
Glickfeld, L. L., Roberts, J., Somogyi, P., and Scanziani, M. (2009). Interneurons hyperpolarize pyramidal cells along their entire somatodendritic axis. Nat. Neurosci. 12, 21–23. doi: 10.1038/nn.2230
Goldstein, P. A., Elsen, F. P., Ying, S.-W., Ferguson, C., Homanics, G. E., and Harrison, N. L. (2002). Prolongation of hippocampal miniature inhibitory postsynaptic currents in mice lacking the GABAA receptor α1 subunit. J. Neurophysiol. 88, 3208–3217. doi: 10.1152/jn.00885.2001
Gorrie, G. H., Vallis, Y., Stephenson, A., Whitfield, J., Browning, B., Smart, T. G., et al. (1997). Assembly of GABAA receptors composed of A1 and B2 subunits in both cultured neurons and fibroblasts. J. Neurosci. 17, 6587–6596. doi: 10.1523/JNEUROSCI.17-17-06587.1997
Grosskreutz, Y., Hermann, A., Kins, S., Fuhrmann, J. C., Betz, H., and Kneussel, M. (2001). Identification of a gephyrin-binding motif in the GDP/GTP exchange factor collybistin. Biol. Chem. 382, 1455–1462. doi: 10.1515/bc.2001.179
Han, B., Zhou, R., Xia, C., and Zhuang, X. (2017). Structural organization of the actin-spectrin-based membrane skeleton in dendrites and soma of neurons. Proc. Natl. Acad. Sci. U S A 114, E6678–E6685. doi: 10.1073/pnas.1705043114
Hannan, S., Minere, M., Harris, J., Izquierdo, P., Thomas, P., Tench, B., et al. (2019). GABAAR isoform and subunit structural motifs determine synaptic and extrasynaptic receptor localisation. Neuropharmacology doi: 10.1016/j.neuropharm.2019.02.022 [Epub ahead of print].
Harvey, K., Duguid, I. C., Alldred, M. J., Beatty, S. E., Ward, H., Keep, N. H., et al. (2004). The GDP-GTP exchange factor collybistin: an essential determinant of neuronal gephyrin clustering. J. Neurosci. 24, 5816–5826. doi: 10.1523/JNEUROSCI.1184-04.2004
Hines, R. M., Maric, H. M., Hines, D. J., Modgil, A., Panzanelli, P., Nakamura, Y., et al. (2018). Developmental seizures and mortality result from reducing GABAA receptor A2-subunit interaction with collybistin. Nat. Commun. 9:3130. doi: 10.1038/s41467-018-05481-1
Huang, Y.-M., and Rasband, M. N. (2016). Organization of the axon initial segment: actin like a fence. J. Cell Biol. 215, 9–11. doi: 10.1083/jcb.201609084
Jenkins, S. M., and Bennett, V. (2001). Ankyrin-G coordinates assembly of the spectrin-based membrane skeleton, voltage-gated sodium channels, and L1 CAMs at purkinje neuron initial segments. J. Cell Biol. 155, 739–746. doi: 10.1083/jcb.200109026
Kins, S., Betz, H., and Kirsch, J. (2000). Collybistin, a newly identified brain-specific GEF, induces submembrane clustering of gephyrin. Nat. Neurosci. 3, 22–29. doi: 10.1038/71096
Kittler, J. T., McAinsh, K., and Moss, S. J. (2002). Mechanisms of GABAA receptor assembly and trafficking: implications for the modulation of inhibitory neurotransmission. Mol. Neurobiol. 26, 251–268. doi: 10.1385/mn:26:2-3:251
Kneussel, M., Brandstätter, J. H., Laube, B., Stahl, S., Müller, U., and Betz, H. (1999). Loss of postsynaptic GABAA receptor clustering in gephyrin-deficient mice. J. Neurosci. 19, 9289–9297. doi: 10.1523/JNEUROSCI.19-21-09289.1999
Leterrier, C. (2018). The axon initial segment: an updated viewpoint. J. Neurosci. 38, 2135–2145. doi: 10.1523/JNEUROSCI.1922-17.2018
Leterrier, C., Vacher, H., Fache, M.-P., d’Angles, S. A., Castets, F., Autillo-Touati, A., et al. (2011). End-binding proteins EB3 and EB1 link microtubules to ankyrin G in the axon initial segment. Proc. Natl. Acad. Sci. U S A 108, 8826–8831. doi: 10.1073/pnas.1018671108
Lorenz-Guertin, J. M., and Jacob, T. C. (2018). GABA type a receptor trafficking and the architecture of synaptic inhibition. Dev. Neurobiol. 78, 238–270. doi: 10.1002/dneu.22536
Miller, P. S., and Aricescu, A. R. (2014). Crystal structure of a human GABAA receptor. Nature 512, 270–275. doi: 10.1038/nature13293
Moss, S. J., Doherty, C. A., and Huganir, R. L. (1992). Identification of the CAMP-dependent protein kinase and protein kinase C phosphorylation sites within the major intracellular domains of the β 1, γ 2S, and γ 2L subunits of the γ-aminobutyric acid type A receptor. J. Biol. Chem. 267, 14470–14476.
Moss, S. J., and Smart, T. G. (2001). Constructing inhibitory synapses. Nat. Rev. Neurosci. 2, 240–250. doi: 10.1038/35067500
Muir, J., and Kittler, J. T. (2014). Plasticity of GABAA receptor diffusion dynamics at the axon initial segment. Front. Cell. Neurosci. 8:151. doi: 10.3389/fncel.2014.00151
Nathanson, A. J., Zhang, Y., Smalley, J. L., Ollerhead, T. A., Rodriguez Santos, M. A., Andrews, P. M., et al. (2019). Identification of a core amino acid motif within the α subunit of GABAARs that promotes inhibitory synaptogenesis and resilience to seizures. Cell Rep. 28, 670.e8–681.e8. doi: 10.1016/j.celrep.2019.06.014
Nusser, Z., Sieghart, W., Benke, D., Fritschy, J. M., and Somogyi, P. (1996). Differential synaptic localization of two major γ-aminobutyric acid type A receptor α subunits on hippocampal pyramidal cells. Proc. Natl. Acad. Sci. U S A 93, 11939–11944. doi: 10.1073/pnas.93.21.11939
Nyíri, G., Freund, T. F., and Somogyi, P. (2001). Input-dependent synaptic targeting of A2-subunit-containing GABAA receptors in synapses of hippocampal pyramidal cells of the rat. Eur. J. Neurosci. 13, 428–442. doi: 10.1046/j.1460-9568.2001.01407.x
Nymann-Andersen, J., Sawyer, G. W., and Olsen, R. W. (2002). Interaction between GABAA receptor subunit intracellular loops: implications for higher order complex formation. J. Neurochem. 83, 1164–1171. doi: 10.1046/j.1471-4159.2002.01222.x
O’Toole, K. K., and Jenkins, A. (2011). Discrete M3–M4 intracellular loop subdomains control specific aspects of γ-aminobutyric acid type A receptor function. J. Biol. Chem. 286, 37990–37999. doi: 10.1074/jbc.M111.258012
Olsen, R. W., and Sieghart, W. (2008). International union of pharmacology. LXX. Subtypes of γ-aminobutyric acid(A) receptors: classification on the basis of subunit composition, pharmacology, and function. Update. Pharmacol. Rev. 60, 243–260. doi: 10.1124/pr.108.00505
Panzanelli, P., Gunn, B. G., Schlatter, M. C., Benke, D., Tyagarajan, S. K., Scheiffele, P., et al. (2011). Distinct mechanisms regulate GABAA receptor and gephyrin clustering at perisomatic and axo-axonic synapses on CA1 pyramidal cells. J. Physiol. 589, 4959–4980. doi: 10.1113/jphysiol.2011.216028
Papadopoulos, T., Eulenburg, V., Reddy-Alla, S., Mansuy, I. M., Li, Y., and Betz, H. (2008). Collybistin is required for both the formation and maintenance of GABAergic postsynapses in the hippocampus. Mol. Cell. Neurosci. 39, 161–169. doi: 10.1016/j.mcn.2008.06.006
Papadopoulos, T., Korte, M., Eulenburg, V., Kubota, H., Retiounskaia, M., Harvey, R. J., et al. (2007). Impaired GABAergic transmission and altered hippocampal synaptic plasticity in collybistin-deficient mice. EMBO J. 26, 3888–3899. doi: 10.1038/sj.emboj.7601819
Prior, P., Schmitt, B., Grenningloh, G., Pribilla, I., Multhaup, G., Beyreuther, K., et al. (1992). Primary structure and alternative splice variants of gephyrin, a putative glycine receptor-tubulin linker protein. Neuron 8, 1161–1170. doi: 10.1016/0896-6273(92)90136-2
Reid, T., Bathoorn, A., Ahmadian, M. R., and Collard, J. G. (1999). Identification and characterization of HPEM-2, a guanine nucleotide exchange factor specific for Cdc42. J. Biol. Chem. 274, 33587–33593. doi: 10.1074/jbc.274.47.33587
Roux, L., and Buzsáki, G. (2015). Tasks for inhibitory interneurons in intact brain circuits. Neuropharmacology 88, 10–23. doi: 10.1016/j.neuropharm.2014.09.011
Saiepour, L., Fuchs, C., Patrizi, A., Sassoè-Pognetto, M., Harvey, R. J., and Harvey, K. (2010). Complex role of collybistin and gephyrin in GABAA receptor clustering. J. Biol. Chem. 285, 29623–29631. doi: 10.1074/jbc.m110.121368
Saiyed, T., Paarmann, I., Schmitt, B., Haeger, S., Sola, M., Schmalzing, G., et al. (2007). Molecular basis of gephyrin clustering at inhibitory synapses: role of G- and E-domain interactions. J. Biol. Chem. 282, 5625–5632. doi: 10.1074/jbc.m610290200
Saliba, R. S., Michels, G., Jacob, T. C., Pangalos, M. N., and Moss, S. J. (2007). Activity-dependent ubiquitination of GABAA receptors regulates their accumulation at synaptic sites. J. Neurosci. 27, 13341–13351. doi: 10.1523/JNEUROSCI.3277-07.2007
Sassoè-Pognetto, M., Panzanelli, P., Sieghart, W., and Fritschy, J. M. (2000). Colocalization of multiple GABAA receptor subtypes with gephyrin at postsynaptic sites. J. Comp. Neurol. 420, 481–498. doi: 10.1002/(sici)1096-9861(20000515)420:4<481::aid-cne6=3.0.co;2-5
Schofield, P. R., Darlison, M. G., Fujita, N., Burt, D. R., Stephenson, F. A., Rodriguez, H., et al. (1987). Sequence and functional expression of the GABAA receptor shows a ligand-gated receptor super-family. Nature 328, 221–227. doi: 10.1038/328221a0
Schwarz, G., Schrader, N., Mendel, R. R., Hecht, H. J., and Schindelin, H. (2001). Crystal structures of human gephyrin and plant Cnx1 G domains: comparative analysis and functional implications. J. Mol. Biol. 312, 405–418. doi: 10.2210/pdb1eav/pdb
Somogyi, P., Nunzi, M. G., Gorio, A., and Smith, A. D. (1983). A new type of specific interneuron in the monkey hippocampus forming synapses exclusively with the axon initial segments of pyramidal cells. Brain Res. 259, 137–142. doi: 10.1016/0006-8993(83)91076-4
Tai, Y., Gallo, N. B., Wang, M., Yu, J.-R., and Van Aelst, L. (2019). Axo-axonic innervation of neocortical pyramidal neurons by GABAergic chandelier cells requires AnkyrinG-associated L1CAM. Neuron 102, 358.e9–372.e9. doi: 10.1016/j.neuron.2019.02.009
Thomas, P., Mortensen, M., Hosie, A. M., and Smart, T. G. (2005). Dynamic mobility of functional GABAA receptors at inhibitory synapses. Nat. Neurosci. 8, 889–897. doi: 10.1038/nn1483
Tyagarajan, S. K., and Fritschy, J.-M. (2014). Gephyrin: a master regulator of neuronal function? Nat. Rev. Neurosci. 15, 141–156. doi: 10.1038/nrn3670
Vithlani, M., Terunuma, M., and Moss, S. J. (2011). The dynamic modulation of GABAA receptor trafficking and its role in regulating the plasticity of inhibitory synapses. Physiol. Rev. 91, 1009–1022. doi: 10.1152/physrev.00015.2010
Wang, Y., Zhang, P., and Wyskiel, D. R. (2016). Chandelier cells in functional and dysfunctional neural circuits. Front. Neural Circuits 10:33. doi: 10.3389/fncir.2016.00033
Wisden, W., Laurie, D. J., Monyer, H., and Seeburg, P. H. (1992). The distribution of 13 GABAA receptor subunit MRNAs in the rat brain. I. Telencephalon, diencephalon, mesencephalon. J. Neurosci. 12, 1040–1062. doi: 10.1523/JNEUROSCI.12-03-01040.1992
Xu, K., Zhong, G., and Zhuang, X. (2013). Actin, spectrin, and associated proteins form a periodic cytoskeletal structure in axons. Science 339, 452–456. doi: 10.1126/science.1232251
Zhou, D., Lambert, S., Malen, P. L., Carpenter, S., Boland, L. M., and Bennett, V. (1998). AnkyrinG is required for clustering of voltage-gated na channels at axon initial segments and for normal action potential firing. J. Cell Biol. 143, 1295–1304. doi: 10.1083/jcb.143.5.1295
Keywords: GABAA receptor, axon initial segment, collybistin, gephyrin, inhibition, synapse formation
Citation: Nathanson AJ, Davies PA and Moss SJ (2019) Inhibitory Synapse Formation at the Axon Initial Segment. Front. Mol. Neurosci. 12:266. doi: 10.3389/fnmol.2019.00266
Received: 14 August 2019; Accepted: 17 October 2019;
Published: 05 November 2019.
Edited by:
Shiva K. Tyagarajan, University of Zurich, SwitzerlandReviewed by:
Jaewon Ko, Daegu Gyeongbuk Institute of Science and Technology (DGIST), South KoreaCopyright © 2019 Nathanson, Davies and Moss. This is an open-access article distributed under the terms of the Creative Commons Attribution License (CC BY). The use, distribution or reproduction in other forums is permitted, provided the original author(s) and the copyright owner(s) are credited and that the original publication in this journal is cited, in accordance with accepted academic practice. No use, distribution or reproduction is permitted which does not comply with these terms.
*Correspondence: Stephen J. Moss, c3RlcGhlbi5tb3NzQHR1ZnRzLmVkdQ==
Disclaimer: All claims expressed in this article are solely those of the authors and do not necessarily represent those of their affiliated organizations, or those of the publisher, the editors and the reviewers. Any product that may be evaluated in this article or claim that may be made by its manufacturer is not guaranteed or endorsed by the publisher.
Research integrity at Frontiers
Learn more about the work of our research integrity team to safeguard the quality of each article we publish.