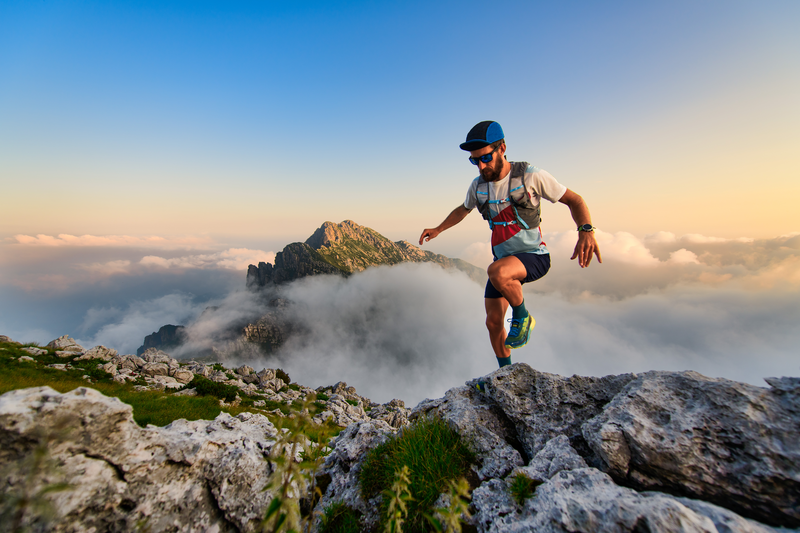
94% of researchers rate our articles as excellent or good
Learn more about the work of our research integrity team to safeguard the quality of each article we publish.
Find out more
ORIGINAL RESEARCH article
Front. Mol. Neurosci. , 29 March 2017
Sec. Molecular Signalling and Pathways
Volume 10 - 2017 | https://doi.org/10.3389/fnmol.2017.00086
Sensorineural hearing loss (SNHL) is caused by an irreversible impairment of cochlear hair cells and subsequent progressive degeneration of spiral ganglion neurons (SGNs). Eighty-five requiring 3 (Efr3) is a plasma membrane protein conserved from yeast to human, and knockout of Efr3a was reported to facilitate the survival of hippocampal newborn neurons in adult mice. Previously, we found Efr3a expression in the auditory neural pathway is upregulated soon after the destruction of hair cells. Here we conducted a time-course analysis of drug-caused damage to hearing ability, hair cells and SGNs in Efr3a knocking down mice (Efr3a−/+, Efr3a KD) and their wild type littermates. Functional examination showed that both groups of mice suffered from serious hearing loss with a higher level of severity in wild type (WT) mice. Morphologic observation following drugs administration showed that both WT and Efr3a KD mice went through progressive loss of hair cells and SGNs, in association with degenerative changes in the perikarya, intracellular organelles, cell body conformation in SGNs, and the changes of SGNs in WT mice were more severe than in Efr3a KD mice. These beneficial effects of Efr3a KD could be ascribed to an increase in the expression of some neurotrophic factors and their receptors in Efr3a KD mice. Our results indicate that Efr3a insufficiency suppresses drug-caused SNHL neurodegeneration in association with an increase in the expression of some neurotrophic factors and their receptors, which may be targeted in the treatment of neurodegeneration.
The majority of the deafness patients suffer from sensorineural hearing loss (SNHL). SNHL, resulting from noise exposure, aging, genetic deficiency or ototoxic drugs, is characterized by the irreversible damage of cochlear hair cells followed by a progressive degeneration of spiral ganglion neurons (SGNs) and auditory nerve fibers (Webster and Webster, 1981; Lawner et al., 1997; Hardie and Shepherd, 1999; Fetoni et al., 2013). SGNs are the primary bipolar neurons indispensable to the pathway of auditory system that relays auditory information from the hair cells to the auditory center (Martinez-Monedero et al., 2006; Richardson et al., 2006).
In humans or other mammals, the loss of the cochlear hair cells cause permanent hearing impairment for its inability to regenerate. Cochlear implantation technology is the major option to treat hearing impairment due to the loss of cochlear hair cells, of which the efficacy depends on the number of surviving functional SGN (Nadol et al., 1989; Gantz et al., 1993; Xu et al., 2012). Therefore, the current primary strategy to further advances in cochlear implantation is to preserve SGN and induce SGN regeneration to maximize the number of functional neurons. Recently, some research progress has been made in repairing damaged SGN and regeneration of cochlear nerve fiber, such as via stem cell transplantation or exogenous neurotrophic factor (Agterberg et al., 2008; Jang et al., 2015). But there are relatively less studies about blocking or alleviating the degeneration of SGN and their synaptic terminal.
After noise, antibiotic or toxic insult to the cochlea, synapses between peripheral nerve fibers of the SGN and the hair cells in the cochlea are the first to degenerate, followed by the swollen or retraction of the cochlear nerve fiber over various time periods and finally dysfunction and loss of SGN (Dodson and Mohuiddin, 2000; Agterberg et al., 2008; Kujawa and Liberman, 2009; Nie et al., 2015). However, the molecular mechanism for the retrograde degeneration of SGN is unclear. Dying back is a common and chronic pathological process of neural degeneration which arises gradually from distal to proximal and is found in a wide variety of degenerative and toxic conditions of the peripheral and central nervous system in neurodegenerative disorders, and several possibilities have been suggested and tested to explain the dying back, involving undernourishment of the distal axon, impairment of axonal transport of organelles and vesicles, target-derived neurotrophic factors (Fischer et al., 2004; Dadon-Nachum et al., 2011). In the SNHL, retrograde degeneration of SGN subsequent to the hair cell loss in the cochlea is morphologically similar to “dying back”, but the molecular mechanism is largely unknown.
The yeast Eighty-five requiring 3 (Efr3) gene, and its Drosophila and mammalian homologs, rolling blackout (rbo) and Efr3a/Efr3b respectively, encode a membrane-localized protein that forms a protein complex with another two proteins on the plasma membrane to maintain the plasmalemmal level of phosphoinositol-4-phosphate (PI4P) and PI4,5P (Huang et al., 2004; Baird et al., 2008; Hammond et al., 2012; Nakatsu et al., 2012), which have widely direct functions in the sensory and motor nervous system, including production of IP3 and DAG, regulation of signal transduction, exocytosis, endocytosis, ion channel and neurotransmitter receptor functions, cell adhesion, and nucleation of the actin cytoskeleton (Di Paolo and De Camilli, 2006). The endocytosis mediated by PI4,5P is involved in a variety of processes at the cell surface (Kumari et al., 2010; McMahon and Boucrot, 2011), including axonal and neuronal degeneration, collapse, disintegration and death (Weinkove et al., 2008; Kuboyama et al., 2015). In Drosophila, the RBO played a pivotal role in PI4,5P-mediated signaling in photoreceptors, and in bulk endocytosis and macropinocytosis in neuronal and non-neuronal cells (Huang et al., 2004, 2006; Vijayakrishnan et al., 2009). In mammalian cells, Efr3 is also important in the control of G-protein-coupled receptor (GPCR)-mediated signaling by affecting the phosphorylation of GPCR (Bojjireddy et al., 2015). In adult mice, Efr3 plays an important role in the survival of newborn hippocampal neurons possibly via regulating the brain-derived neurotrophic factor (BDNF) pathway (Qian et al., 2017). Moreover, in rodents, Efr3a expression in the medial olivocochlear neurons in the brain stem and in the cochlear SGNs is up-regulated soon after the destruction of hair cells (Munemoto et al., 2004; Nie et al., 2015), suggesting a role of Efr3a in the auditory remodeling or degeneration subsequent to the deprivation of acoustic signal. In addition, we previously showed that the expression of Efr3a in the cochlear SGNs was increased mainly at the early stage of SGNs degeneration, which indicated that Efr3a may play an intermediary role in initiating cochlear SGNs degeneration (Nie et al., 2015).
In this study, we investigated the effect of partial knockout of Efr3a on the hair cells loss, SGN degeneration and hearing loss caused by kanamycin and furosemide treatment, and found that Efr3a insufficiency attenuates the progressive SGN degeneration. It is known that SGN degeneration during hearing loss could be alleviated by the treatment with neurotrophic factors, such as BDNF or neurotrophins-3 (NT-3) (Staecker et al., 1996; Richardson et al., 2005; Shepherd et al., 2005; Agterberg et al., 2008). We further examined the effect of Efr3a insufficiency on the expression levels of several neurotrophic factors and their receptors, and found some were elevated. Thus, our results show that Efr3a insufficiency attenuates drug-caused SGN degeneration possibly by up-regulating some neurotrophins-mediated signalings.
Efr3a were generated by breeding Efr3afl/fl mice (Qian et al., 2017) to Ella-Cre mice, which expresses efficient Cre activity in one-cell zygote stage of embryonic development (Lakso et al., 1996). The progenies with the second exon of Efr3a deleted and a reading frame shift in Efr3a mRNA (Efr3a−/+) were backcrossed to C57BL/6 mice for over 10 generations to obtain genetic background-purified Efr3a−/+ mice.
All animals were raised under standard laboratory conditions with food and water freely available. Humidity and temperature were kept constant at 60 ± 5% and 24 ± 2°C, respectively. Lights were on between 7:00 am and 7:00 pm. The care and process of animals were complied with the approval of the Institutional Authority for Laboratory Animal Care of Xinhua Hospital Affiliated Shanghai Jiao Tong University School of Medicine (Shanghai, China).
Genotypes were determined by polymerase chain reaction (PCR) using genomic DNA from mouse tails. The mutant Efr3a−/+ allele was detected using primer F 5′-TTATTTAGTATGTTGGACGA G-3′ and primer R 5′-ACAAACTTAACCTCCATGTT-3′. A 500 bp band was detected in mice with Efr3a−/+ (Efr3a knocking down mice, Efr3a KD mice), while no signal was examined in wild type mice (WT mice; data not shown).
Efr3a protein level was determined by fluorescent immunohistochemistry and western blotting, and the detail method referred to the previously report (Nie et al., 2015). For fluorescent immunohistochemistry, the cochleae of 8 weeks old mice without any treatment were isolated, and dealt with decalcification and dehydration. Then frozen sections (7 μm) paralleled to the modiolus were obtained. After incubation with 3% BSA, cryosections were incubated with diluted primary antibodies (1:500 mouse anti-TUJ1 (Covance) and 1:200 rabbit anti-Efr3a (Sigma)) at 4°C overnight and then were incubated in 1:400 FITC-conjugated donkey anti mouse and 1:400 Alexa-594 conjugated anti-rabbit goat IgG (Beyotime Biotechnology) for 1 h at room temperature. Lastly, the sections were labeled with DAPI and images were captured using a fluorescent microscope (Leica, Berlin, Germany). For western blotting, modiolus (not the intact cochlear organs) in eight cochleae were isolated, digested and separated for the further experiment.
After genotype analysis, all animals (8–10 weeks old) were assigned randomly into experimental or control group in both WT and Efr3a KD mice. The kanamycin and furosemide treatment was the same as previously described (Nie et al., 2015). Briefly, experimental animals were treated with a hypodermic injection of kanamycin (1000 mg/kg) and followed with a peritoneal injection of furosemide (400 mg/kg) 30–45 min after. All the experimental mice were divided into the 1st, 5th, 15th, 30th and 60th day groups after injection. Mice administered with same volume of saline were used as controls.
Auditory brainstem response (ABR) was carried out at the 3rd day before any injection (baseline measurement) and 5th, 15th and 60th day following the kanamycin and furosemide administration or saline injection (post measurement) in order to determine the ABR threshold shifts. It was tested using a MEB-3102 physiological response recorder (Nihon Kohden, Japan) as previously reported (Nie et al., 2015). Briefly, mice were anesthetized with ketamine (100 mg/kg) and xylazine (10 mg/kg) and kept in a sound-proof room during the test. The active electrode was inserted into the subcutaneous tissue of vertex, the reference electrode into the mastoid process, and the ground electrode into the contralateral thigh. Acoustic signal stimuli, consisting of pure tone bursts at frequencies of 4, 8, 12, 16, 24 and 32 kHz, were generated with Tucker Davis Technologies device (SigGen) with a rate of 21.1 times per second. For each test, 512 responses for every frequency were recorded and the evoked potentials were filtered with a bandpass of 100–3000 Hz. Stimuli started at 70 dB for the pretreatment groups and at 110 dB for the post-treatment groups, and decreased by 5 dB until the threshold was reached. The threshold was determined as the lowest intensity that wave III could be recorded repeatedly.
Mice cochleae were harvested at the 1st, 5th, 15th, 30th and 60th day after drugs administration. The animals were euthanized with overdose of chloral hydrate and both cochleae were dissected out. Then the cochleae were perfused and fixed in 4% phosphate buffered paraformaldehyde (pH7.5) overnight at 4°C and then decalcified in 10% ethylene diamine tetraacetic acid (EDTA) at room temperature for 1 week.
The organ of Corti was carefully separated into apex turn and base turn. Immunohistochemistry for myosin VIIa were performed to evaluate cochlear hair cells. The detail procedure was carried out following the method described previously (Nie et al., 2015). The tissues were incubated in rabbit anti-myosin VIIa antibody (1:300; Proteus Bioscience) at 4°C overnight and then were incubated with Alexa-594 conjugated anti-rabbit goat IgG (1:400; Beyotime Biotechnology) for 1 h at room temperature. Afterwards, the basilar membranes were fixed on glass slides with antifade solution and images were acquired with a confocal fluorescent microscopy (Zeiss LSM710).
After dissecting the bulla, the cochleae were perfused with 2.5% glutaraldehyde and fixed at least 2 h, decalcified with EDTA for 7 days and then embedded in Eponate 12. The detail protocol was performed by following the procedure described previously (Nie et al., 2015). Serial sections (1 μm thick) approximately parallel to the modiolus were obtained to count the density of SGNs. Altogether, six cross-sections from Rosenthal’s canal with an interval approximately 30 μm were taken from each cochlea. All sections were observed under a light microscope after staining with 1% toluidine blue. The SGN counts were calculated including apical, middle and basal turns. NIH Image J software was used to determine the cross-sectional area of Rosenthal’s canal. SGN density was calculated by dividing the number of perikaryon by the cross-sectional area. Five cochleae were included in each group.
Ultrathin (50–60 nm) sections parallel to the modiolus were prepared to study the ultrastructural morphology of SGNs. Sections were stained sequentially with uranyl acetate and lead citrate, and images were captured under a transmission electron microscope (TEM; Philips CM-120). The morphology of SGN was detected in the cochlea basal turn, a location where the influence of hair cell loss on SGN density is obvious.
Based on the close inspection of SGN from WT and KD cochleae, perikaryal area and cell circularity, two cellular characteristics of the SGN were selected for quantitative analysis. One hundred to one hundred and fifty cochlear SGNs with an obvious nucleus in three mice were counted and measured in each group. The perikaryal area and circularity were measured by NIH Image J software. The ultrastructural changes of the mitochondrial and endoplasmic reticulum morphology, lipofuscin-like granule in perikaryon were also observed. In addition, quantitative analysis of lipofuscin-like granules was performed by measuring the ratio of lipofuscin area/SGN cytoplasm area (excluding the nucleus).
Total RNA of the modiolus including spiral ganglia from the same brood of control WT and Efr3a KD mice at 8-week old was prepared using TRIzol reagent (Takara Bio Inc., Japan). Total RNA was further purified using DNase I to eliminate contaminating genomic DNA, then the cDNA was synthesized using the PrimeScriptTM RT Master Mix (Takara Bio Inc., Japan). A portion of the cDNAs was used as template for the real-time PCR, which were carried out with the ABI PRISM 7500 System (Thermo Fisher Scientific) using SYBR® Premix Ex TaqTM (Tli RNaseH Plus; Takara Bio Inc. Japan). The primer sequences of targeted genes are listed below. The relative concentration of targeted genes relative to GAPDH was determined by the 2(−ΔΔCT) method. Then, another portion of the cDNAs was used for normal PCR, and the PCR products were identified with 3% agarose gel electrophoresis. The gray intensity of detected bands was quantified using Image Lab software (v 5.2; Bio-Rad, USA) and normalized them against the density of GAPDH.
• Neural growth factor (NGF):
5′ TCCACCCACCCAGTCTTCCA 3′ (forward)
5′ CCTTCCTGCTGAGCACACA 3′ (reverse)
• BDNF:
5′ GCGGCAGATAAAAAGACTGC 3′ (forward)
5′ CTTATGAATCGCCAGCCAAT 3′ (reverse)
• NT-3:
5′ CTACTACGGCAACAGAGACGCT 3′ (forward)
5′ GGTGAGGTTCTATTGGCTACCAC 3′ (reverse)
• Tyrosine kinase receptor A (TrkA):
5′ ACGGTAACAGCACATCAAGAG 3′(forward)
5′ GGAGGGCAGAAAGGAAGAG 3′(reverse)
• Tyrosine kinase receptor B (TrkB):
5′ AAGGACTTTCATCGGGAAGCTG 3′(forward)
5′ TCGCCCTCCACACAGACAC 3′(reverse)
• Tyrosine kinase receptor C (TrkC):
5′ CAACTCTCAAACACGGAGGTC 3′(forward)
5′ CCAGCATGACATCGTACACC 3′(reverse)
• GAPDH:
5′ GGTGAAGGTCGGTGTGAACG 3′ (forward)
5′ CTCGCTCCTGGAAGATGGTG 3′ (reverse)
The 8-week old WT and Efr3a KD mice were sacrificed and the modiolus including spiral ganglia were rapidly isolated from the cochleae. The protein was extracted as reported previously (Nie et al., 2015). Tissues were lysed in radio immunoprecipitation assay buffer (Beyotime) and homogenized, then subjected to western blotting analysis. Protein were separated by 12% SDS-PAGE gels for 120–150 min at 80 V and transferred onto polyvinylidene fluoride (PVDF) membranes (Millipore, USA). The membranes were blocked with 5% skim milk in TBST for 1 h at room temperature and incubated with primary antibodies for TrkA (Abcam, USA; 1:1000), TrkB (CST, USA; 1:1000), TrkC (CST, USA; 1:1000), NGF (Abcam, USA; 1:1000), BDNF (Sigma, USA; 1:1000), NT-3 (Santa Cruz, USA; 1:1000) and β-actin (Beyotime, 1:1000) overnight at 4°C, and then incubated with HRP-conjugated secondary antibody for 1 h at room temperature after being washed three times in TBST. The immunoreactive signals were detected using enhanced chemiluminescence system with BeyoECL Star kit (Beyotime Biotechnology) and the bands were semi-quantified using Image Lab software (v 5.2; Bio-Rad, USA).
The SPSS statistical software (v 19.0; SPSS Inc., Chicago, IL, USA) was used for all statistical analysis. Distributions of all data in each group were analyzed for normality using the Shapiro-Wilk test. Group comparisons between WT and KD mice at the same time point were made using Student’s t-test or Mann-Whitney test. Statistically significant differences of perikaryal area, circularity and lipofuscin area between experimental and control mice within WT and KD mice were determined via one-way ANOVA testing followed by Dunnett post hoc testing. A p-value of <0.05 was deemed to indicate statistical significance.
Unconditional Efr3a knockout mice (Efr3a−/−) are embryonic lethal. Thus, we used Efr3a−/+ heterozygotes, here named as Efr3a KD mice, for the following experiments. We first examined the expression level of Efr3a protein in Efr3a KD mice and their WT littermates. Immunofluorescence staining in the frozen sections showed that, compared with WT mice, the expression of Efr3a was obviously decreased in the Efr3a KD mice (Figure 1A). Consistently, quantitative analysis of Efr3a protein in cochlea by western blotting showed an intense band from the cochleae of WT mice, but a weaker band from the cochleae of KD mice at 92.5 kDa position, the expression of Efr3a was decreased by approximately 50% (p < 0.05) in the Efr3a KD mice, confirming the reduction of Efr3a in Efr3a KD mice (Figure 1B).
Figure 1. Eighty-five requiring 3a knocking down (Efr3a KD) ameliorated the hearing loss induced by kanamycin and furosemide treatment. (A) Representative fluorescent immunohistochemistry images of Efr3a protein in the spiral ganglion neurons (SGNs), repeated at least three times, scale bar = 50 μm; (B) representative western blot (top) and semi-quantification (bottom) showing the Efr3a expression in the spiral ganglion of cochlea, n = 8 for wild type (WT) and Efr3a KD, respectively. T-test, unpaired, two tail; (C) mean auditory brainstem response (ABR) thresholds to sound stimulations at different frequencies. KD and WT represent Efr3a KD and WT mice without drug treatment respectively, while KD-5d and WT-5d represent Efr3a KD and WT mice with drugs treatment at the 5th day posterior to drugs application respectively. n = 16 for each data point, Mann-Whitney test or unpaired T-test, following normality test; Data are represented by mean ± SEM, “*” indicates p < 0.05 between Efr3a KD and WT mice at the same frequency.
Then, a time course analysis of the effect of Efr3a KD on the hearing impairment induced by kanamycin and furosemide treatment through measuring the ABR threshold was conducted. Total 16 Efr3a KD mice and 16 WT were used. Before drugs treatment, the hearing function observed in both WT and Efr3a KD mice are similar to that previously described for C57BL/6J (Park et al., 2010; Mistry et al., 2014; Xiong et al., 2014), the ABR thresholds at pure tones of 4, 8, 12, 16, 24 and 32 kHz frequencies between the two groups were indistinguishable (Figure 1C, bottom), indicating partial deletion of Efr3a did not significantly change the function of the auditory system. Five days after drugs treatment, the ABR thresholds in both groups were markedly increased, demonstrating a severe hearing loss in both groups. Interestingly, the ABR thresholds in KD mice were relatively lower than those in WT mice, especially at 8 kHz (91.56 ± 2.95 dB vs. 81.56 ± 2.27 dB, t test, p = 0.012) and 12 kHz (91.56 ± 2.80 dB vs. 81.25 ± 1.96 dB, t test, p = 0.005; Figure 1C, top). Fifteen and 60 days after drugs treatment, however, the ABR thresholds at all six frequencies in almost all animals were higher than 85 dB, and no significant difference of ABR thresholds between WT and KD mice was observed, which might be due to the loss of vast majority of hair cells in both strains of mice (Figure 2). Nevertheless, the data demonstrate that Efr3a reduction temporarily ameliorated the hearing impairment caused by kanamycin and furosemide.
Figure 2. Efr3a reduction did not change the loss of hair cells caused by drugs treatment Representative myosin VIIa immuno-labeled hair cells in the cochleae of the WT (WT) and Efr3a KD (KD) mice without drug treatment (A) and with drugs treatment at the 5th day (B), scale bar = 50 μm. (C) Quantification of VIIa immuno-labeled hair cells in non-treated (control) and drug-treated WT and KD mice at the 1st, 5th, 15th and 30th day posterior to drugs application, n ≥ 5 for each data point. Data are represented by mean ± SEM. No significant difference in density of hair cells in both apical and basal turns of cochlea between Efr3a KD and WT mice were observed.
We conducted immunofluorescence staining in whole mounts of the Corti organ with an antibody against Myosin VIIa to label hair cells in both Efr3a KD and WT mice with and without drug treatment. As shown in Figures 2A,C, the density, morphology and arrangement of hair cells in both groups without drug treatment were indistinguishable, indicating Efr3a KD did not affect the development of hair cells. We also did a time course analysis of the density of hair cells in both groups of mice with drugs treatment at the 1st, 5th, 15th and 30th day after drugs administration. Starting from the 1st day, a progressive severe loss of hair cells occurred in both groups (Figures 2B,C). The density of inner hair cells (IHCs) in both apical and basal cochlea was reduced by about 50% at the 1st day, followed by about 50% decrease in the density of residual hair cells by the 5th day, and a further 50% reduction by the 15th day, then no further obvious loss of hair cells. The destruction of outer hair cells (OHCs) was faster and severer than the IHCs, more than 70% by the 1st day, and almost complete loss by the 15th day. At each time point posterior to drugs treatment, there was no significant change in density of residual inner or OHCs in both apical and basal turns of cochlea between Efr3a KD and WT mice (Figure 2C).
Hair cells are innervated by the SGNs, which are known to degenerate over time following the loss of hair cells. To test whether Efr3a reduction could produce a protective role against the SGN degeneration, we conducted toluidine blue staining and light imaging, then quantitative analysis of the densities of SGNs in Rosenthal’s canal at the 5th, 15th, 30th and 60th day following drugs administration. We counted SGNs in the apical, middle and basal regions of Rosenthal’s canal. At the 5th day posterior to the drugs administration, the densities of SGN in both WT and Efr3a KD mice did not change significantly, but progressively decreased to much lower values at the following time points, 45% in WT and 53% in KD group in the apical turn at the 60th day (t test, p = 0.026; Figure 3A); 51% in WT and 61% in KD group in the middle turn at the 60th day (t test, p = 0.008; Figure 3B); 69% in WT and 76% the in KD group in the basal turn at the 30th day (t test, p = 0.002) and 49% in WT and 57% in KD group in the basal turn at the 60th day (t test, p = 0.026; Figure 3C).
Figure 3. Efr3a reduction attenuated the loss of SGNs caused by drugs treatment. Serial light microscopy changes of the SGNs from WT mice vs. Efr3a KD mice from apical (A), middle (B) and basal (C) regions of the cochlea, scale bar = 100 μm. The quantification of the SGN densities in each region is shown at the right. SGN density showed a progressive decrease in both strains, but earlier and more severe in WT mice (A–C). Data are represented by mean ± SEM, n = 5 for each data point, T-test, unpaired, two tail; “*” indicates p < 0.05.
We further studied the SGN degeneration with TEM. The TEM images of SGNs were taken from the cochlear basal turn, where the effect of hair cell loss on SGN density is most prominent. In WT and Efr3a KD mice without drug treatment, several SGNs lie typically close to each other, intracellular organelles, such as mitochondria, are intact, chromatin is distributed evenly in the prominent nucleus and the perikaryon is surrounded by the myelin sheath of the peripheral process of a Schwann cell (Figures 4A1,B1,C1,C2). However, drugs treatment induced a series of time-dependent intracellular changes in the SGNs in both WT and Efr3a KD mice. At the 5th day, similar to our previous report (Nie et al., 2015), the perikarya of only few neurons were slightly or moderately enlarged (data not shown) in both WT and Efr3a KD mice. At the 15th day, the perikarya further enlarged, abnormal mitochondria with rarefaction of the matrix and partial loss of cristae as well as cytoplasm vacuolization became evident, lipofuscin accumulated, and the cell body of SGNs deformed (reflected by the change in cell circularity) in both WT mice and Efr3a KD mice, but more severe in WT mice (Figures 4A2,B2,C3,C4,D1,D2, 5A,B). At 30th day and 60th day, the perikarya shrinked gradually, and other degenerative changes became more and more severe in both WT mice and Efr3a KD mice, and largely more severe in WT mice (Figures 4A3,B3,A4,B4,D1,D2, 5A,B). These data demonstrate that Efr3a reduction reduced the drug-induced degeneration in SGNs.
Figure 4. Efr3a reduction ameliorated the structural alterations of SGNs following hair cell loss. Representative transmission electron microscope (TEM) images of the SGNs in the cochlea from WT and Efr3a KD (KD) mice without (A1,B1) and with drugs treatment at the 15th (A2,B2), 30th (A3,B3) and 60th (A4,B4) day posterior to drugs application. (C1–C4) is a close view of the boxed areas in (A1,B1,A2,B2) respectively. White arrows indicate the cytoplasmic vacuoles gathered at the edge of the cytoplasm of the SGNs, with a few swelling and scattered mitochondria (m), while black arrows indicate the balloon-like appearance around the perikarya of SGNs. Scale bar = 5 μm in (A1–A4) and (B1–B4). Quantitative time-course analysis of the perikaryal area (D1) and cell circularity (D2). Data are represented by mean ± SEM, n ≥ 100 SGNs for each data point, unpaired T-test (two tail) or Mann-Whitney test between WT and KD group at the same time point, and one-way ANOVA testing followed by Dunnett post hoc testing between experimental and control group within WT and KD mice. Blue “*” indicates p < 0.05 compared with control in Efr3a KD mice, red “*” indicates p < 0.05 compared with control in WT mice, and black “#” indicates p < 0.05 between Efr3a KD and WT mice in each time point. WT vs. Efr3a KD: p = 0.038 at the 60th day for perikaryal area, p = 0.026 at the 15th day and p = 0.018 at the 30th day for cell circularity.
Figure 5. Efr3a reduction suppressed the formation of lipofuscin in SGNs following hair cell loss. (A) Representative TEM images showing lipofuscin-like granule (black arrow) in the perikarya of SGNs. Scale bars = 2 μm. (B) Quantitative time-course analysis of the ratio of lipofuscin area/SGN cytoplasm area (excluding the nucleus). Data were represented by mean ± SEM, n ≥ 50 SGNs for each data point, unpaired T-test (two tail) between WT and KD group at the same time point, and one-way ANOVA testing followed by Dunnett post hoc testing between experimental and control group within WT and KD mice. Blue “*” indicates p < 0.05 compared with control in Efr3a KD mice, red “*” indicates p < 0.05 compared with control in WT mice, and black “#” indicates p < 0.05 between Efr3a KD and WT mice in each time point. WT vs. Efr3a KD: p = 0.043 at the 15th day and p = 0.045 at the 60th day.
Lack of target-derived neurotrophic factors has been suggested and tested to explain the dying back process of neurons. Given the report that the expression of BDNF and its receptor TrkB was found to be increased in the brain of mice with nestin-CRE mediated complete knockout of Efr3a (Qian et al., 2017), Quantitative real-time PCR was performed to examine the mRNA of NGF, BDNF, NT-3 and their receptors, TrkA, TrkB and TrkC, in the spiral ganglions from the same brood of control WT and Efr3a KD mice without drug treatment. Indeed, the mRNA levels of BDNF and NT-3 and their receptors, but not NGF and TrkA, were significantly elevated in Efr3a KD mice (Figure 6A). Then we re-confirmed the changes by reverse transcription PCR (RT-PCR) and electrophoresis, and found similar elevation in BDNF, NT-3, TrkB and TrkC (Figures 6B,C). Further more, the protein levels of BDNF, NT-3 and TrkB, but not NGF, TrkA and TrkC, in Efr3a KD mice were higher than those in WT mice (Figure 7).
Figure 6. Efr3a reduction upregulated the expression of some neurotrophins and their receptors at mRNA levels. Quantification of the mRNA levels of brain-derived neurotrophic factor (BDNF), neurotrophins-3 (NT-3), tyrosine kinase receptor B (TrkB) and tyrosine kinase receptor C (TrkC) in WT and Efr3a KD mice by real-time polymerase chain reaction (PCR; A) and reverse transcription (RT)-PCR (B,C), the representative images of the electrophoresis of RT-PCR products are shown in (B). Data are represented by mean ± SEM, n = 8, T-test, unpaired, two tail. “*” indicates p < 0.05 between Efr3a KD and WT mice.
Figure 7. Efr3a reduction upregulated the expression of some neurotrophins and their receptors at protein levels. The representative immunoreactive bands and quantification of the protein levels of neural growth factor (NGF), BDNF, NT-3, tyrosine kinase receptor A (TrkA), TrkB and TrkC in WT and Efr3a KD mice by western blotting. Data are represented by mean ± SEM, n = 12, T-test, unpaired, two tail. “*” indicates p < 0.05 between Efr3a KD and WT mice.
Aminoglycoside antibiotics have substantial ototoxic effects. A single dose injection of aminoglycoside immediately following by injection of a loop diuretic induces hair cell loss rapidly, and subsequent progressive degeneration of SGNs and their auditory nerve fibers in chinchilla, rats, guinea pigs and mice at relatively slower speed, which have been frequently used to model the disease of SNHL for studying disease mechanism and therapeutic efficacy (McFadden et al., 2004; Glueckert et al., 2008; Layman et al., 2015; Wang et al., 2015). In the present study, we, with the co-administration of a single dose of kanamycin and furosemide, successfully and rapidly destroyed vast majority of cochlear hair cells by 15 days, consequently induced a progressive degeneration of SGNs in mice with a similar time course as reported in the previous studies. At the 5th day after drugs administration, WT animals developed a severe hearing impairment, at 15th day the ABR thresholds at all frequencies were higher than 85 dB, and remained high until the 60th day. In parallel to the hearing loss, there was a progressive loss of IHCs: about 50% IHCs disappeared by the 1st day, followed by 50% reduction of the residual IHCs by the 5th day, a further 50% reduction by the 15th day, then not much change during the 15th–30th days. The loss of OHCs was relatively faster and more severe, over 70% loss by the 1st day, and almost complete loss by the 15th day. Consequently, starting from the 5th day, the SGNs degenerated over time, and the ultrastructure of SGNs underwent stereotypic degenerative changes in the perikarya, intracellular organelles, cell body conformation.
Efr3a KD temporarily suppressed the rapid loss of hearing ability, and the progressive and relatively slower degenerative alterations in SGNs in the model of hearing loss, indicating Efr3a KD produced a beneficial effect against the neurodegeneration of SGNs induced by aminoglycoside antibiotics. Moreover, Efr3a KD significantly increased the expression of BDNF, NT-3 and their receptors, TrkB and TrkC at both mRNA and protein levels, but not the expression of NGF and TrkA, which is consistent with a recent study that complete knockout of Efr3a in brain neurons elevated the expression levels of BDNF and TrkB, and suppressed the apoptosis of hippocampal newborn neurons in adult mice (Qian et al., 2017).
Neurotrophic factors and their tyrosine kinase receptors play essential roles in the establishment of neuron number through their control of cell survival/death during neuronal development and degeneration (Huang and Reichardt, 2001; Schimmang et al., 2003; Ito and Enomoto, 2016). The degeneration of SGN in SNHL was considered to be a consequence of absence of neurotrophic factor from the hair cells, which is supported by many other studies showing that the ultrastructural changes and loss of SGNs in SNHL could be attenuated by the application of BDNF or NT-3 (Staecker et al., 1996; Richardson et al., 2005; Shepherd et al., 2005; Agterberg et al., 2008; Landry et al., 2011; Fukui et al., 2012; van Loon et al., 2013). In addition, previous studies showed that Efr3a expression was up-regulated in the lateral superior olive within 2 days after the removal of cochleae (Munemoto et al., 2004) and in SGNs within 5 days after the co-administration of kanamycin and furosemide (Nie et al., 2015). Therefore, we speculate that Efr3a may negatively regulate the expression of NT-3, BDNF, TrkB and TrkC, and the beneficial effect of Efr3a KD might be ascribed to an elevation of the corresponding downstream signalings.
It is well known that Efr3/RBO forms a plasma membrane localized complex with PI4KIIIα and a scaffold protein to maintain the levels of plasmalemmal PI4P and PI4,5P, particularly PI4P (Baird et al., 2008; Hammond et al., 2012; Nakatsu et al., 2012). Whether and how this protein complex regulates the expression of neurotrophins and their receptors, and in turn regulates the down-stream signalings by controlling plasmalemmal levels of PI4P and PI4,5P remain to be determined.
Except at a narrow window of stimulating frequencies and at the 5th day after drugs treatment, there was a close correlation between ABR thresholds and the densities of SGNs and hair cells in WT and Efr3a KD mice at all stimulating frequencies and at all the days thereafter. The explanation could be that at the 5th day, the functional state of SGNs and hair cells with higher levels of neurotrophins in Efr3a KD mice was better than that in WT mice. Efr3a KD produced moderate beneficial effect on the survival of SNGs, but not on that of hair cells. The explanation is that the detrimental effect of ototoxic drugs at high dose on the preservation of hair cell density was too strong be suppressed by the beneficial effect of Efr3a insufficiency due to the higher susceptibility of hair cells to the drugs than SGNs and other cells in the body. Nevertheless, the functional state of hair cells with higher levels of neurotrophins in Efr3a KD mice might be still better than that in WT mice at the 5th days after drugs injection. Therefore, under sound stimulation at a narrow range of frequencies at the 5th day after drugs application, the ABR thresholds in Efr3a KD mice were significantly lower than those in WT mice.
Taken together, our study indicated that decrease of Efr3a attenuates the degeneration of SGN after hair cell loss, possibly by up-regulating the expression of some neurotrophic factors and their receptors.
MX and FH conceived the project; FH wrote the manuscript; MX, FH, HH and BY designed the experiments; HW provided technical and platform supports. HH participated in manuscript preparation and did most of the experiments along with BY except ABR measurement and hair cell counting, which was carried out by JL and YM, respectively; HH raised the animals along with QW; LZ, SJ and ZL generated the Efr3a−/+ mice and purified the genetic background under the instruction of YX. All authors listed, have made substantial, direct and intellectual contribution to the work, and approved it for publication.
The authors declare that the research was conducted in the absence of any commercial or financial relationships that could be construed as a potential conflict of interest.
This work was supported by National Natural Science Foundation of China (Grant No. 81271088; Grant No. 81371400 and Grant No. 81670926); National Key Basic Research Program of China (2013CB530900) and by the Science and Technology Commission of Shanghai Municipality, China (No. 15411950303 and No. 14DZ2260300).
ABR, auditory brainstem response; BDNF, brain-derived neurotrophic factor; EDTA, Ethylene Diamine Tetraacetic Acid; Efr3, Eighty-five requiring 3; Efr3a KD, Efr3a knocking down; IHCs, inner hair cells; NGF, neural growth factor; NT-3, neurotrophins-3; OHCs, outer hair cells; PCR, polymerase chain reaction; PI4P, phosphoinositol-4-phosphate; RBO, rolling blackout; RT-PCR, reverse transcription PCR; SGN, spiral ganglion neuron; SNHL, sensorineural hearing loss; TEM, transmission electron microscope; TrkA, tyrosine kinase receptor A; TrkB, tyrosine kinase receptor B; TrkC, tyrosine kinase receptor C; WT mice, wild type mice.
Agterberg, M. J., Versnel, H., de Groot, J. C., Smoorenburg, G. F., Albers, F. W., and Klis, S. F. (2008). Morphological changes in spiral ganglion cells after intracochlear application of brain-derived neurotrophic factor in deafened guinea pigs. Hear. Res. 244, 25–34. doi: 10.1016/j.heares.2008.07.004
Baird, D., Stefan, C., Audhya, A., Weys, S., and Emr, S. D. (2008). Assembly of the PtdIns 4-kinase Stt4 complex at the plasma membrane requires Ypp1 and Efr3. J. Cell Biol. 183, 1061–1074. doi: 10.1083/jcb.200804003
Bojjireddy, N., Guzman-Hernandez, M. L., Reinhard, N. R., Jovic, M., and Balla, T. (2015). EFR3s are palmitoylated plasma membrane proteins that control responsiveness to G-protein-coupled receptors. J. Cell Sci. 128, 118–128. doi: 10.1242/jcs.157495
Dadon-Nachum, M., Melamed, E., and Offen, D. (2011). The “dying-back” phenomenon of motor neurons in ALS. J. Mol. Neurosci. 43, 470–477. doi: 10.1007/s12031-010-9467-1
Di Paolo, G., and De Camilli, P. (2006). Phosphoinositides in cell regulation and membrane dynamics. Nature 443, 651–657. doi: 10.1038/nature05185
Dodson, H. C., and Mohuiddin, A. (2000). Response of spiral ganglion neurones to cochlear hair cell destruction in the guinea pig. J. Neurocytol. 29, 525–537. doi: 10.1023/A:1007201913730
Fetoni, A. R., De Bartolo, P., Eramo, S. L. M., Rolesi, R., Paciello, F., Bergamini, C., et al. (2013). Noise-induced hearing loss (NIHL) as a target of oxidative stress-mediated damage: cochlear and cortical responses after an increase in antioxidant defense. J. Neurosci. 33, 4011–4023. doi: 10.1523/jneurosci.2282-12.2013
Fischer, L. R., Culver, D. G., Tennant, P., Davis, A. A., Wang, M., Castellano-Sanchez, A., et al. (2004). Amyotrophic lateral sclerosis is a distal axonopathy: evidence in mice and man. Exp. Neurol. 185, 232–240. doi: 10.1016/j.expneurol.2003.10.004
Fukui, H., Wong, H. T., Beyer, L. A., Case, B. G., Swiderski, D. L., Di Polo, A., et al. (2012). BDNF gene therapy induces auditory nerve survival and fiber sprouting in deaf Pou4f3 mutant mice. Sci. Rep. 2:838. doi: 10.1038/srep00838
Gantz, B. J., Woodworth, G. G., Knutson, J. F., Abbas, P. J., and Tyler, R. S. (1993). Multivariate predictors of audiological success with multichannel cochlear implants. Ann. Otol. Rhinol. Laryngol. 102, 909–916. doi: 10.1177/000348949310201201
Glueckert, R., Bitsche, M., Miller, J. M., Zhu, Y., Prieskorn, D. M., Altschuler, R. A., et al. (2008). Deafferentation-associated changes in afferent and efferent processes in the guinea pig cochlea and afferent regeneration with chronic intrascalar brain-derived neurotrophic factor and acidic fibroblast growth factor. J. Comp. Neurol. 507, 1602–1621. doi: 10.1002/cne.21619
Hammond, G. R. V., Fischer, M. J., Anderson, K. E., Holdich, J., Koteci, A., Balla, T., et al. (2012). PI4P and PI(4,5)P2 are essential but independent lipid determinants of membrane identity. Science 337, 727–730. doi: 10.1126/science.1222483
Hardie, N. A., and Shepherd, R. K. (1999). Sensorineural hearing loss during development: morphological and physiological response of the cochlea and auditory brainstem. Hear. Res. 128, 147–165. doi: 10.1016/s0378-5955(98)00209-3
Huang, F. D., Matthies, H. J., Speese, S. D., Smith, M. A., and Broadie, K. (2004). Rolling blackout, a newly identified PIP2-DAG pathway lipase required for Drosophila phototransduction. Nat. Neurosci. 7, 1070–1078. doi: 10.1038/nn1313
Huang, E. J., and Reichardt, L. F. (2001). Neurotrophins: roles in neuronal development and function. Annu. Rev. Neurosci. 24, 677–736. doi: 10.1146/annurev.neuro.24.1.677
Huang, F.-D., Woodruff, E., Mohrmann, R., and Broadie, K. (2006). Rolling blackout is required for synaptic vesicle exocytosis. J. Neurosci. 26, 2369–2379. doi: 10.1523/JNEUROSCI.3770-05.2006
Ito, K., and Enomoto, H. (2016). Retrograde transport of neurotrophic factor signaling: implications in neuronal development and pathogenesis. J. Biochem. 160, 77–85. doi: 10.1093/jb/mvw037
Jang, S., Cho, H.-H., Kim, S.-H., Lee, K.-H., Jun, J. Y., Park, J. S., et al. (2015). Neural-induced human mesenchymal stem cells promote cochlear cell regeneration in deaf Guinea pigs. Clin. Exp. Otorhinolaryngol. 8, 83–91. doi: 10.3342/ceo.2015.8.2.83
Kuboyama, T., Lee, Y. A., Nishiko, H., and Tohda, C. (2015). Inhibition of clathrin-mediated endocytosis prevents amyloid β-induced axonal damage. Neurobiol. Aging 36, 1808–1819. doi: 10.1016/j.neurobiolaging.2015.02.005
Kujawa, S. G., and Liberman, M. C. (2009). Adding insult to injury: cochlear nerve degeneration after “temporary” noise-induced hearing loss. J. Neurosci. 29, 14077–14085. doi: 10.1523/JNEUROSCI.2845-09.2009
Kumari, S., Mg, S., and Mayor, S. (2010). Endocytosis unplugged: multiple ways to enter the cell. Cell Res. 20, 256–275. doi: 10.1038/cr.2010.19
Lakso, M., Pichel, J. G., Gorman, J. R., Sauer, B., Okamoto, Y., Lee, E., et al. (1996). Efficient in vivo manipulation of mouse genomic sequences at the zygote stage. Proc. Natl. Acad. Sci. U S A 93, 5860–5865. doi: 10.1073/pnas.93.12.5860
Landry, T. G., Wise, A. K., Fallon, J. B., and Shepherd, R. K. (2011). Spiral ganglion neuron survival and function in the deafened cochlea following chronic neurotrophic treatment. Hear. Res. 282, 303–313. doi: 10.1016/j.heares.2011.06.007
Lawner, B. E., Harding, G. W., and Bohne, B. A. (1997). Time course of nerve-fiber regeneration in the noise-damaged mammalian cochlea. Int. J. Dev. Neurosci. 15, 601–617. doi: 10.1016/s0736-5748(96)00115-3
Layman, W. S., Williams, D. M., Dearman, J. A., Sauceda, M. A., and Zuo, J. (2015). Histone deacetylase inhibition protects hearing against acute ototoxicity by activating the Nf-κB pathway. Cell Death Discov. 1:15012. doi: 10.1038/cddiscovery.2015.12
Martinez-Monedero, R., Corrales, C. E., Cuajungco, M. P., Heller, S., and Edge, A. S. (2006). Reinnervation of hair cells by auditory neurons after selective removal of spiral ganglion neurons. J. Neurobiol. 66, 319–331. doi: 10.1002/neu.20232
McFadden, S. L., Ding, D., Jiang, H., and Salvi, R. J. (2004). Time course of efferent fiber and spiral ganglion cell degeneration following complete hair cell loss in the chinchilla. Brain Res. 997, 40–51. doi: 10.1016/j.brainres.2003.10.031
McMahon, H. T., and Boucrot, E. (2011). Molecular mechanism and physiological functions of clathrin-mediated endocytosis. Nat. Rev. Mol. Cell Biol. 12, 517–533. doi: 10.1038/nrm3151
Mistry, N., Nolan, L. S., Saeed, S. R., Forge, A., and Taylor, R. R. (2014). Cochlear implantation in the mouse via the round window: effects of array insertion. Hear. Res. 312, 81–90. doi: 10.1016/j.heares.2014.03.005
Munemoto, Y., Houtani, T., Kase, M., Sakuma, S., Baba, K., Yamashita, T., et al. (2004). Mouse homolog of KIAA0143 protein: hearing deficit induces specific changes of expression in auditory brainstem neurons. Mol. Brain Res. 128, 131–140. doi: 10.1016/j.molbrainres.2004.06.022
Nadol, J. B. Jr., Young, Y.-S., and Glynn, R. J. (1989). Survival of spiral ganglion cells in profound sensorineural hearing loss: implications for cochlear implantation. Ann. Otol. Rhinol. Laryngol. 98, 411–416. doi: 10.1177/000348948909800602
Nakatsu, F., Baskin, J. M., Chung, J., Tanner, L. B., Shui, G., Lee, S. Y., et al. (2012). PtdIns4P synthesis by PI4KIIIα at the plasma membrane and its impact on plasma membrane identity. J. Cell Biol. 199, 1003–1016. doi: 10.1083/jcb.201206095
Nie, C., Hu, H., Shen, C., Ye, B., Wu, H., and Xiang, M. (2015). Expression of EFR3A in the mouse cochlea during degeneration of spiral ganglion following hair cell loss. PLoS One 10:e0117345. doi: 10.1371/journal.pone.0117345
Park, S.-N., Back, S.-A., Park, K.-H., Kim, D.-K., Park, S. Y., Oh, J. H., et al. (2010). Comparison of cochlear morphology and apoptosis in mouse models of presbycusis. Clin. Exp. Otorhinolaryngol. 3, 126–135. doi: 10.3342/ceo.2010.3.3.126
Qian, Q., Liu, Q., Zhou, D., Pan, H., Liu, Z., He, F., et al. (2017). Brain-specific ablation of Efr3a promotes adult hippocampal neurogenesis via the brain-derived neurotrophic factor pathway. FASEB J. doi: 10.1096/fj.201601207R [Epub ahead of print].
Richardson, R. T., Noushi, F., and O’Leary, S. (2006). Inner ear therapy for neural preservation. Audiol. Neurootol. 11, 343–356. doi: 10.1159/000095896
Richardson, R. T., O’Leary, S., Wise, A., Hardman, J., and Clark, G. (2005). A single dose of neurotrophin-3 to the cochlea surrounds spiral ganglion neurons and provides trophic support. Hear. Res. 204, 37–47. doi: 10.1016/j.heares.2005.01.001
Schimmang, T., Tan, J., Müller, M., Zimmermann, U., Rohbock, K., Kôpschall, I., et al. (2003). Lack of Bdnf and TrkB signalling in the postnatal cochlea leads to a spatial reshaping of innervation along the tonotopic axis and hearing loss. Development 130, 4741–4750. doi: 10.1242/dev.00676
Shepherd, R. K., Coco, A., Epp, S. B., and Crook, J. M. (2005). Chronic depolarization enhances the trophic effects of brain-derived neurotrophic factor in rescuing auditory neurons following a sensorineural hearing loss. J. Comp. Neurol. 486, 145–158. doi: 10.1002/cne.20564
Staecker, H., Kopke, R., Malgrange, B., Lefebvre, P., and Van de Water, T. R. (1996). NT-3 and/or BDNF therapy prevents loss of auditory neurons following loss of hair cells. Neuroreport 7, 889–894. doi: 10.1097/00001756-199603220-00011
van Loon, M. C., Ramekers, D., Agterberg, M. J., de Groot, J. C., Grolman, W., Klis, S. F., et al. (2013). Spiral ganglion cell morphology in guinea pigs after deafening and neurotrophic treatment. Hear. Res. 298, 17–26. doi: 10.1016/j.heares.2013.01.013
Vijayakrishnan, N., Woodruff, E. A. III, and Broadie, K. (2009). Rolling blackout is required for bulk endocytosis in non-neuronal cells and neuronal synapses. J. Cell Sci. 122, 114–125. doi: 10.1242/jcs.036673
Wang, Y., Qiao, L., Chen, Y., Wen, L., Yue, B., Qiu, J., et al. (2015). Establishment of a model of cochlear lesions in rats to study potential gene therapy for sensorineural hearing loss. Int. J. Pediatr. Otorhinolaryngol. 79, 2147–2154. doi: 10.1016/j.ijporl.2015.09.037
Webster, M., and Webster, D. B. (1981). Spiral ganglion neuron loss following organ of Corti loss: a quantitative study. Brain Res. 212, 17–30. doi: 10.1016/0006-8993(81)90028-7
Weinkove, D., Bastiani, M., Chessa, T. A., Joshi, D., Hauth, L., Cooke, F. T., et al. (2008). Overexpression of PPK-1, the Caenorhabditis elegans Type I PIP kinase, inhibits growth cone collapse in the developing nervous system and causes axonal degeneration in adults. Dev. Biol. 313, 384–397. doi: 10.1016/j.ydbio.2007.10.029
Xiong, H., Dai, M., Ou, Y., Pang, J., Yang, H., Huang, Q., et al. (2014). SIRT1 expression in the cochlea and auditory cortex of a mouse model of age-related hearing loss. Exp. Gerontol. 51, 8–14. doi: 10.1016/j.exger.2013.12.006
Keywords: hearing loss, Efr3a, spiral ganglion neuron, degeneration, neurotrophic factors
Citation: Hu H, Ye B, Zhang L, Wang Q, Liu Z, Ji S, Liu Q, Lv J, Ma Y, Xu Y, Wu H, Huang F and Xiang M (2017) Efr3a Insufficiency Attenuates the Degeneration of Spiral Ganglion Neurons after Hair Cell Loss. Front. Mol. Neurosci. 10:86. doi: 10.3389/fnmol.2017.00086
Received: 27 October 2016; Accepted: 10 March 2017;
Published: 29 March 2017.
Edited by:
Ildikó Rácz, University of Bonn, GermanyReviewed by:
James Henry Peters, Washington State University, USACopyright © 2017 Hu, Ye, Zhang, Wang, Liu, Ji, Liu, Lv, Ma, Xu, Wu, Huang and Xiang. This is an open-access article distributed under the terms of the Creative Commons Attribution License (CC BY). The use, distribution and reproduction in other forums is permitted, provided the original author(s) or licensor are credited and that the original publication in this journal is cited, in accordance with accepted academic practice. No use, distribution or reproduction is permitted which does not comply with these terms.
*Correspondence: Mingliang Xiang, bWluZ2xpYW5neGlhbmdAMTYzLmNvbQ==
Fude Huang, aHVhbmdmZEBzYXJpLmFjLmNu
Hao Wu, d3VoYW82MjJAc2luYS5jbg==
†These authors have contributed equally to this work.
Disclaimer: All claims expressed in this article are solely those of the authors and do not necessarily represent those of their affiliated organizations, or those of the publisher, the editors and the reviewers. Any product that may be evaluated in this article or claim that may be made by its manufacturer is not guaranteed or endorsed by the publisher.
Research integrity at Frontiers
Learn more about the work of our research integrity team to safeguard the quality of each article we publish.