Introduction
The coronavirus disease 2019 (COVID-19) pandemic, caused by severe acute respiratory syndrome corona virus 2 (SARS-CoV-2), has been affecting lifestyles and health care worldwide (West et al., 2020; Patel et al., 2021). Social distancing to avoid SARS-CoV-2 infection became a new normal during the COVID-19 pandemic. Scholars working in the fields of basic, translational and clinical medicine have adapted to COVID-19 turmoil through dynamic changes in their research methods and noticed some convenient aspects of remote work, teleconference and telemedicine (Ohannessian et al., 2020; Wang et al., 2021).
Molecular medicine is a field of medical sciences that addresses the mechanisms of human diseases (Figure 1). Preclinical studies using patient-derived cell lines, organoids and xenograft as well as animal models, such as monkey, mouse, Xenopus and zebrafish, are driving apparatuses for mechanistic understanding, target discovery and therapeutic optimization (Ramani et al., 2020; Rockx et al., 2020). Clinical trials are safety apparatuses to investigate the benefits and adverse effects of investigational diagnostics and therapeutics (Gyawali et al., 2019; Jung et al., 2021). Preclinical studies and clinical trials constitute a valuable core of molecular medicine to achieve innovation and improve medical practices (Figure 1).
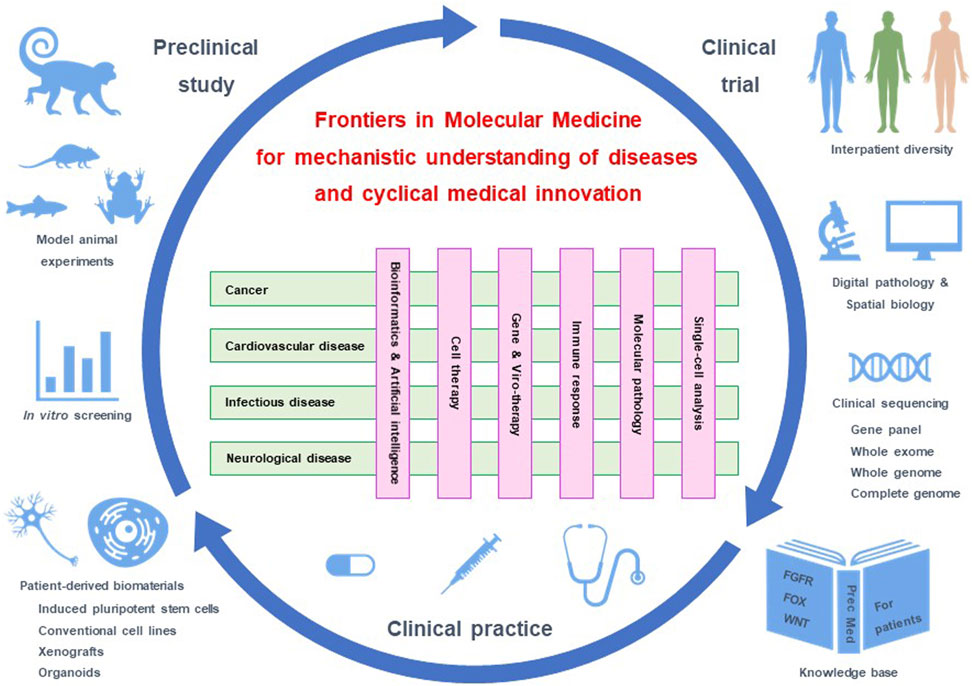
FIGURE 1. Molecular medicine and cyclical innovation. Frontiers in Molecular Medicine consists of four sections focused on diseases and six sections focused on methodologies. Preclinical studies using model animals and patient-derived materials, clinical trials using cutting-edge biomarkers, and clinical practice based on a knowledge base constitute a medical innovation cycle. Our journal addresses the understanding of disease mechanisms through cross-disciplinary interactions of scholars and the promotion of innovation cycles for the prevention and treatment of human diseases.
There are three major obstacles that hinder cyclical medical innovation. Basic studies are not always recapitulated in clinical trials because of intrinsic biases of cell lines, engineered mouse models and human organoids; investigational drugs are not always approved for the treatment of patients owing to unknown on-target adverse effects; and approved drugs are not always beneficial for patients even after selection using companion diagnostics (Katoh, 2019; Katoh and Katoh, 2020). Integrative interactions of researchers in the fields of basic, translational and clinical medicine as well as those of cancerous and noncancerous diseases are essential to promoting innovation cycles in the healthcare and medicine sector.
Frontiers Media SA in Lausanne, Switzerland, is now launching a new journal, Frontiers in Molecular Medicine, to establish a platform of knowledge generation through timely publication of cutting-edge manuscripts and global networking of multidisciplinary scholars. The section structure of this journal will be briefly introduced, and then our perspectives on COVID-19, tumor heterogeneity and precision medicine will be discussed.
Structure of Frontiers in Molecular Medicine
This journal comprises ten sections, each of which consists of Specialty Chief Editors, Associate Editors, Review Editors and Guest Associate Editors. Applications for Associate or Review Editors and proposals for Research Topics are welcome.
“Molecular Medicine and Cancer Treatment”, “Molecular Medicine for Cardiology”, “Molecular Microbes and Disease”, and “Molecular Mechanisms of Neurodegeneration” are disease-oriented sections that address human diseases, such as cancers, cardiovascular diseases, infectious diseases, and neurological diseases. Other common or rare diseases are also within the scope of our journal. Please consult editorial staff about the section to submit. A proposal of disease-oriented Section other than those mentioned above will be considered positively from viewpoints of innovativeness and networking potential of the applicant for its Specialty Chief Editor.
In contrast, “Bioinformatics and Artificial Intelligence”, “Cell Therapy”, “Gene and Viro-therapy”, “Molecular Mechanisms of Immune Response”, “Molecular Pathology”, and “Single Cell Analysis” are methodology-oriented Sections that address cutting-edge technologies, including antibody-drug conjugates (ADCs), bispecific antibodies, chimeric antigen receptor-modified T (CAR-T) cells, clinical bioinformatics, clinical sequencing, complete-genome sequencing, digital pathology, explainable artificial intelligence, liquid biopsy, oncolytic viruses, pluripotent stem cells, protein degraders, single-cell analyses, spatial biology, and telemedicine.
Cross-boundary interactions among disease- and methodology-oriented sections during the editorial process of submitted manuscripts as well as through arrangements of joint Research Topics are critical features of our journal for driving knowledge generation and horizontal innovation in the field of molecular medicine (Figure 1).
COVID-19
SARS-CoV-2 enters and infects host cells through angiotensin converting enzyme 2 (ACE2) on endothelial cells, enterocytes and type II pneumocytes (Ziegler et al., 2020) as well as neuropilin-1 (NRP1) on endothelial cells, lung epithelium and olfactory epithelium (Cantuti-Castelvetri et al., 2020). COVID-19 patients present with fever, cough, fatigue, headache, hyposmia, hypogeusia, diarrhea and other symptoms, and some patients progress to severe conditions owing to respiratory, cardiovascular or cerebrovascular complications (Chen et al., 2020; Ellul et al., 2020; Nishiga et al., 2020). The case fatality rate of the general population with COVID-19 infection in the Worldmeters database is approximately 2.1% (3,331,763 of 160, 339, 530, as of May 12, 2021) (Worldometer, 2021); however, the rates of hospitalized COVID-19 patients in subgroups, such as the elderly, males, patients with diabetes, and the patients with hypertension and cardiovascular disease are much higher (Zhou F. et al., 2020; Grasselli et al., 2020).
Vaccines (BNT162b2, mRNA-1273, NVX-CoV2373, AZD1222 and Ad26.COV2.S) and therapeutic antibody cocktails (REGN-COV2) are SARS-CoV-2-targeted drugs for preventing infection or aggravation of COVID-19 (Polack et al., 2020; Ramasamy et al., 2020; Baden et al., 2021; Sadoff et al., 2021; Shinde et al., 2021; Weinreich et al., 2021). SARS-CoV-2 N501Y variants (B.1.1.7 in the United Kingdom) spread rapidly owing to increased affinity to ACE2 receptor, whereas SARS-CoV-2 N501Y/E484K variants (B.1.351 in South Africa) prone to escape antibody-mediated neutralization (Collier et al., 2021; Zhu et al., 2021). BNT162b2 vaccine showed 89.5 and 75.0% efficacies against B.1.1.7 and B.1.351 variants (Abu-Raddad et al., 2021). Because neutralization antibodies and T cell immunity are anti-viral mechanisms of dual wielding vaccines, vaccine-elicited T cell immunity might explain the benefits of vaccination against B.1.351 variants. Vaccine-based elimination is an optimal strategy to contain SARS-CoV-2 worldwide; however, global surveillance of SARS-CoV-2 variations and cyclic vaccinations targeting escape mutants might be necessary.
Repurposed drugs, such as anti-interleukin six receptor monoclonal antibodies (tocilizumab), broad-spectrum antiviral drugs (remdesivir) and corticosteroids (dexamethasone), are being applied to treat or ameliorate the symptoms of COVID-19 patients (Goldman et al., 2020; Stone et al., 2020; Tomazini et al., 2020), while an investigational ATR inhibitor (berzosertib) for the treatment of cancer patients with ARID1A, ATM and SMARCA4 alterations based on synthetic lethal strategy (Williamson et al., 2016; Gupta et al., 2020; Yap et al., 2020) showed anti-SARS-CoV-2 activity in preclinical experiments (Garcia et al., 2021). Small-molecule compounds that block replication of SARS-CoV-2 would be game changers to end the COVID-19 pandemic in a few years.
In contrast, SARS-CoV-2 elicits versatile COVID-19 pathologies through direct infections, immunological responses and vascular damage in multiple organs or tissues. Because chronic persistent infection with hepatitis viruses and Helicobacter pylori cause liver cancer and gastric cancer, respectively (Ajani et al., 2017; McGlynn et al., 2021), and intracranial inflammation causes neurodegenerative diseases, such as Alzheimer’s disease and Parkinson’s disease (Heppner et al., 2015; Poewe et al., 2017), SARS-CoV-2 might also promote carcinogenesis or dementia through persistent chronic infection, dysregulated host immunity or vasculopathy. Epidemiological studies based on genomic analyses and mechanistic studies based on single-cell analyses should be conducted to elucidate the spatiotemporal profiles of COVID-19 pathologies in the future.
Cancer Therapeutics and Tumor Heterogeneity
Whole-exome and whole-genome sequencing analyses on bulk tumors has revealed the genomic landscape of human cancers, including point mutations, fusions, gene amplifications and (super)enhancer alterations in cancer-related genes as well as numerous variants of unknown clinical significance (Rheinbay et al., 2017; Wong et al., 2020). Fusions of the BCR and ABL genes (BCR-ABL) in chronic myelogenous leukemia (Rosti et al., 2017), gain-of-function mutations of the epidermal growth factor receptor (EGFR) gene in lung cancer (Herbst et al., 2018), fusions of the fibroblast growth factor receptor 2 (FGFR2) gene with the BICC1, KIAA1598, MGEA5, PPHLN1 or TACC3 gene in cholangiocarcinoma (Katoh, 2019), amplifications of the HER2 (ERBB2) gene in breast cancer (Oh and Bang, 2020) and exon 14 skipping mutations of the MET gene in lung cancer (Paik et al., 2020) are representative cancer drivers that are targeted by small-molecule inhibitors or antibody-based biologics in the clinic; however, there remain many cancer drivers that have not yet been successfully targeted in clinical practice.
For example, WNT signals are transduced to canonical and non-canonical pathways (Katoh and Katoh, 2007), and the canonical WNT signaling cascade is aberrantly activated in cancer patients owing to loss-of-function alterations in the APC, AXIN1, AXIN2, RNF43 and ZNRF3 genes or gain-of-function alterations in the CTNNB1 gene encoding β-catenin (Clara et al., 2020; Jung and Park, 2020). Investigational WNT signaling blockers of diverse therapeutic modalities, such as small-molecule compounds, peptide mimetics, antibody-based drugs and CAR-T cells, have shown striking benefits in preclinical studies but have not yet been approved for the treatment of cancer patients. Because the canonical WNT signaling cascade is involved in tumorigenesis as well as gastrointestinal, osteogenic and neuronal homeostasis (Katoh and Katoh, 2017), the therapeutic range of WNT signaling blockers might be too narrow for clinical application. Oncodevelopment signaling pathways with versatile functions in adult tissue homeostasis are hard targets for anticancer drug development.
Tumor heterogeneities, classified into interspecies, interpatient and intrapatient heterogeneity, are other obstacles to therapeutic development. Interspecies heterogeneity between human tumors and engineered mouse models is caused by species divergence in the coding regions and noncoding regulatory regions (Cornelissen et al., 2019; Pembroke et al., 2021). Interpatient heterogeneity or diversity is not completely recapitulated in human cell lines, xenografts and organoids owing to relatively small sample size and biases during the establishment procedure (Pauli et al., 2017; Bleijs et al., 2019). Engineered mouse models and patient-derived cell lines, xenografts and organoids are valuable tools for the screening and optimization of investigational drugs in preclinical studies; however, interspecies heterogeneity and interpatient heterogeneity might lead to the development failure of investigational drugs. A deep understanding of interspecies and interpatient heterogeneities with the aid of artificial intelligence and human intelligence would improve the success rate of drug development through orchestration of preclinical studies depending on therapeutic targets and drug moieties.
Intrapatient heterogeneity, further subclassified into intratumor and intertumor heterogeneities, is a hallmark of real tumors. Because antitumor immunity and therapeutic insults damage cancer cells but promote intratumor heterogeneity through acquired genetic alterations (Katoh, 2017; Marusyk et al., 2020), intratumor heterogeneity induces tumor evolution and subsequent intertumor heterogeneity of primary and metastatic lesions (Katoh, 2019; Vitale et al., 2021). Diagnostic genome sequencing based on a panel of approximately 500 cancer-related genes has been applied in the clinic to identify targetable cancer drivers (Katoh and Katoh, 2020; Yu et al., 2021); however, after targeted therapies, cancer drivers and dominant clones could be substituted by others to elicit therapeutic resistance. Temporal monitoring of cancer drivers for the optimization of targeted therapy is mandatory to improve the benefits and response rates of genome-based medicine.
The tumor microenvironment consists of slow-cycling cancer stem cells, proliferating cancer cells and noncancerous cells, such as cancer-associated fibroblasts, endothelial cells, immune cells and neurons (Lambrechts et al., 2018; Altorki et al., 2019). Paracrine and juxtacrine signaling networks within the tumor microenvironment, such as the WNT, FGF and Notch signaling cascades, maintain slow-cycling cancer stem cells and regulate tumor plasticity through omics reprogramming (Katoh, 2017; Katoh and Katoh, 2020). Complete or whole genome sequencing and single-cell analyses should be practiced in preclinical studies to decipher components of and networking within the tumor microenvironment for drug target discovery. Targeting aberrant features of the tumor microenvironment, such as immune evasion, matrix remodeling, metabolic adaptation and tumor angiogenesis, is an alternative strategy for cancer therapy compared with direct targeting of oncogenic drivers in cancer cells themselves (Valkenburg et al., 2018; Sahai et al., 2020).
Precision Medicine in the Post-coronavirus Era
Precision medicine is defined as a medical system that utilizes clinical records, diagnostic imaging, laboratory tests, omics data and wearable device data for the prevention and treatment of human diseases (Collins and Varmus, 2015). Artificial intelligence or machine learning technologies are applied for target discovery and drug screening in preclinical studies as well as diagnostic medical devices in clinical practices of cardiology, endocrinology, gastroenterology, neurology, oncology, ophthalmology, pathology and radiology (Bera et al., 2019; Bi et al., 2019; Benjamens et al., 2020; Zhou Y. et al., 2020). Because risk of bias is a critical issue for black box-type artificial intelligence (Katoh and Katoh, 2020; Nagendran et al., 2020), artificial intelligence and human intelligence are both necessary to analyze multilayers of biodata and develop optimal diagnostics and therapeutics for the future implementation of precision medicine.
G protein-coupled receptors (GPCRs; ADRB1, GLP1R, HRH2, SMO and OPRM), receptor tyrosine kinases (RTKs; EGFR, FGFRs, HER2, MET, and VEGFR), intracellular enzymes (BRAF, CDK4/6, mTOR, PARP, and PI3K) and nuclear receptors (NRs; AR, ER and RAR) have been targeted using small-molecule compounds (Hauser et al., 2017; Katoh, 2019; Zhao et al., 2019; Roskoski, 2020; Wilcock and Webster, 2021), while RTKs (HER2), immune regulators (BCMA/TNFRSF17, CTLA-4, PD-L1, and PD-1), adhesion molecules (CLDN18.2 and NECTIN4) and miscellaneous transmembrane proteins (SLC39A6/LIV1 and TROP2) have been targeted using antibody-based biologics (Andrews et al., 2019; Bardia et al., 2019; Katoh and Katoh, 2020; Powles et al., 2021). Complete-genome sequencing based on long-read nucleotide sequences is superior to whole-genome sequencing based on short-read nucleotide sequences for the identification of novel biomarkers and therapeutic targets because of improved power for repetitive regions and segmentally duplicated regions (Logsdon et al., 2021; Rhie et al., 2021). Spatial omics approaches that reconstitute the tissue microenvironment at the single-cell level are necessary to decipher precise mechanisms of human pathologies and identify vulnerable targets to cure human diseases (Mathys et al., 2019; Travaglini et al., 2020).
Drug development for cancers and other diseases has been carried out by independent teams of experts in specific human diseases. The merits of disease-oriented approach include effective concentration of resources for drug development, such as clinical samples, patient-derived cell lines, organoids and xenografts as well as knowledge of experts in its specific field, whereas the demerits of such a traditional approach are overlooking or ignoring of the deleterious effects of drug candidates on other types of diseases. Artificial intelligence is applied for the discovery of drug targets and the development of therapeutics, while the human intelligence of multidisciplinary experts should be utilized to decide whether “druggable targets” are to be targeted.
Economic stimulus packages of governments and interest rate policies of national banks to support firms, health care systems and households have been alleviating the global economic crisis caused by the COVID-19 pandemic but might increase the financial burdens of societies in the future owing to unprecedented rise of government deficits and debt (International Monetary Fund, 2021a; International Monetary Fund, 2021b). Taking into account the unknown effects of COVID-19 and aging demographics, the implementation of precision medicine is mandatory for the improvement of human health care and the stability of the social insurance system in the post-coronavirus era.
Conclusion
Frontiers in Molecular Medicine provides a platform of knowledge generation and horizontal innovation through the networking of scholars with diverse backgrounds, careers and ethnicities. We aim to advance the mechanistic understanding of human diseases for the development of novel diagnostics and therapeutics and contribute to the implementation of precision medicine through the promotion of cyclical medical innovation.
Author Contributions
MasuK and MasaK designed and wrote this manuscript.
Funding
This study was supported in part by grants-in-aid from MK’s Fund for the Knowledge-Base Project and the Global Network Project.
Conflict of Interest
The authors declare that the research was conducted in the absence of any commercial or financial relationships that could be construed as a potential conflict of interest.
References
Abu-Raddad, L. J., Chemaitelly, H., and Butt, A. A.National Study Group for COVID-19 Vaccination (2021). Effectiveness of the BNT162b2 Covid-19 Vaccine against the B.1.1.7 and B.1.351 Variants. N. Engl. J. Med. doi:10.1056/NEJMc2104974
Ajani, J. A., Lee, J., Sano, T., Janjigian, Y. Y., Fan, D., and Song, S. (2017). Gastric Adenocarcinoma. Nat. Rev. Dis. Primers 3, 17036. doi:10.1038/nrdp.2017.36
Altorki, N. K., Markowitz, G. J., Gao, D., Port, J. L., Saxena, A., Stiles, B., et al. (2019). The Lung Microenvironment: an Important Regulator of Tumour Growth and Metastasis. Nat. Rev. Cancer 19, 9–31. doi:10.1038/s41568-018-0081-9
Andrews, L. P., Yano, H., and Vignali, D. A. A. (2019). Inhibitory Receptors and Ligands beyond PD-1, PD-L1 and CTLA-4: Breakthroughs or Backups. Nat. Immunol. 20, 1425–1434. doi:10.1038/s41590-019-0512-0
Baden, L. R., El Sahly, H. M., Essink, B., Kotloff, K., Frey, S., Novak, R., et al. (2021). Efficacy and Safety of the mRNA-1273 SARS-CoV-2 Vaccine. N. Engl. J. Med. 384, 403–416. doi:10.1056/NEJMoa2035389
Bardia, A., Mayer, I. A., Vahdat, L. T., Tolaney, S. M., Isakoff, S. J., Diamond, J. R., et al. (2019). Sacituzumab Govitecan-Hziy in Refractory Metastatic Triple-Negative Breast Cancer. N. Engl. J. Med. 380, 741–751. doi:10.1056/NEJMoa1814213
Benjamens, S., Dhunnoo, P., and Meskó, B. (2020). The State of Artificial Intelligence-Based FDA-Approved Medical Devices and Algorithms: an Online Database. NPJ Digit. Med. 3, 118. doi:10.1038/s41746-020-00324-0
Bera, K., Schalper, K. A., Rimm, D. L., Velcheti, V., and Madabhushi, A. (2019). Artificial Intelligence in Digital Pathology - New Tools for Diagnosis and Precision Oncology. Nat. Rev. Clin. Oncol. 16, 703–715. doi:10.1038/s41571-019-0252-y
Bi, W. L., Hosny, A., Schabath, M. B., Giger, M. L., Birkbak, N. J., Mehrtash, A., et al. (2019). Artificial Intelligence in Cancer Imaging: Clinical Challenges and Applications. CA A. Cancer J. Clin. 69, 127–157. doi:10.3322/caac.21552
Bleijs, M., Wetering, M., Clevers, H., and Drost, J. (2019). Xenograft and Organoid Model Systems in Cancer Research. EMBO J. 38, e101654. doi:10.15252/embj.2019101654
Cantuti-Castelvetri, L., Ojha, R., Pedro, L. D., Djannatian, M., Franz, J., Kuivanen, S., et al. (2020). Neuropilin-1 Facilitates SARS-CoV-2 Cell Entry and Infectivity. Science 370, 856–860. doi:10.1126/science.abd2985
Chen, N., Zhou, M., Dong, X., Qu, J., Gong, F., Han, Y., et al. (2020). Epidemiological and Clinical Characteristics of 99 Cases of 2019 Novel Coronavirus Pneumonia in Wuhan, China: a Descriptive Study. The Lancet 395, 507–513. doi:10.1016/S0140-6736(20)30211-7
Clara, J. A., Monge, C., Yang, Y., and Takebe, N. (2020). Targeting Signalling Pathways and the Immune Microenvironment of Cancer Stem Cells - a Clinical Update. Nat. Rev. Clin. Oncol. 17, 204–232. doi:10.1038/s41571-019-0293-2
Collier, D. A., De Marco, A., De Marco, A., Ferreira, I. A. T. M., Meng, B., Datir, R. P., et al. (2021). Sensitivity of SARS-CoV-2 B.1.1.7 to mRNA Vaccine-Elicited Antibodies. Nature 593, 136–141. doi:10.1038/s41586-021-03412-7
Collins, F. S., and Varmus, H. (2015). A New Initiative on Precision Medicine. N. Engl. J. Med. 372, 793–795. doi:10.1056/NEJMp1500523
Cornelissen, L. M., Henneman, L., Drenth, A. P., Schut, E., de Bruijn, R., Klarenbeek, S., et al. (2019). Exogenous ERα Expression in the Mammary Epithelium Decreases over Time and Does Not Contribute to P53-Deficient Mammary Tumor Formation in Mice. J. Mammary Gland Biol. Neoplasia 24, 305–321. doi:10.1007/s10911-019-09437-z
Ellul, M. A., Benjamin, L., Singh, B., Lant, S., Michael, B. D., Easton, A., et al. (2020). Neurological Associations of COVID-19. Lancet Neurol. 19, 767–783. doi:10.1016/S1474-4422(20)30221-0
Garcia, G., Sharma, A., Ramaiah, A., Sen, C., Purkayastha, A., Kohn, D. B., et al. (2021). Antiviral Drug Screen Identifies DNA-Damage Response Inhibitor as Potent Blocker of SARS-CoV-2 Replication. Cel Rep. 35, 108940. doi:10.1016/j.celrep.2021.108940
Goldman, J. D., Lye, D. C. B., Hui, D. S., Marks, K. M., Bruno, R., Montejano, R., et al. (2020). Remdesivir for 5 or 10 Days in Patients with Severe Covid-19. N. Engl. J. Med. 383, 1827–1837. doi:10.1056/NEJMoa2015301
Grasselli, G., Greco, M., Zanella, A., Albano, G., Antonelli, M., Bellani, G., et al. (2020). Risk Factors Associated with Mortality Among Patients with COVID-19 in Intensive Care Units in Lombardy, Italy. JAMA Intern. Med. 180, 1345–1355. doi:10.1001/jamainternmed.2020.3539
Gupta, M., Concepcion, C. P., Fahey, C. G., Keshishian, H., Bhutkar, A., Brainson, C. F., et al. (2020). BRG1 Loss Predisposes Lung Cancers to Replicative Stress and ATR Dependency. Cancer Res. 80, 3841–3854. doi:10.1158/0008-5472.CAN-20-1744
Gyawali, B., Hey, S. P., and Kesselheim, A. S. (2019). Assessment of the Clinical Benefit of Cancer Drugs Receiving Accelerated Approval. JAMA Intern. Med. 179, 906–913. doi:10.1001/jamainternmed.2019.0462
Hauser, A. S., Attwood, M. M., Rask-Andersen, M., Schiöth, H. B., and Gloriam, D. E. (2017). Trends in GPCR Drug Discovery: New Agents, Targets and Indications. Nat. Rev. Drug Discov. 16, 829–842. doi:10.1038/nrd.2017.178
Heppner, F. L., Ransohoff, R. M., and Becher, B. (2015). Immune Attack: the Role of Inflammation in Alzheimer Disease. Nat. Rev. Neurosci. 16, 358–372. doi:10.1038/nrn3880
Herbst, R. S., Morgensztern, D., and Boshoff, C. (2018). The Biology and Management of Non-small Cell Lung Cancer. Nature 553, 446–454. doi:10.1038/nature25183
International Monetary Fund (2021a). Fiscal Monitor April 2021. Available at: https://www.imf.org/en/Publications/FM/Issues/2021/03/29/fiscal-monitor-april-2021 (Accessed May 12, 2021).
International Monetary Fund (2021b). World Economic Outlook April 2021: Managing Divergent Recoveries. Available at: https://www.imf.org/en/Publications/WEO/Issues/2021/03/23/world-economic-outlook-april-2021 (Accessed May 12, 2021).
Jung, R. G., Di Santo, P., Clifford, C., Prosperi-Porta, G., Skanes, S., Hung, A., et al. (2021). Methodological Quality of COVID-19 Clinical Research. Nat. Commun. 12, 943. doi:10.1038/s41467-021-21220-5
Jung, Y.-S., and Park, J.-I. (2020). Wnt Signaling in Cancer: Therapeutic Targeting of Wnt Signaling beyond β-catenin and the Destruction Complex. Exp. Mol. Med. 52, 183–191. doi:10.1038/s12276-020-0380-6
Katoh, M. (2017). Canonical and Non-canonical WNT Signaling in Cancer Stem Cells and Their Niches: Cellular Heterogeneity, Omics Reprogramming, Targeted Therapy and Tumor Plasticity (Review). Int. J. Oncol. 51, 1357–1369. doi:10.3892/ijo.2017.4129
Katoh, M. (2019). Fibroblast Growth Factor Receptors as Treatment Targets in Clinical Oncology. Nat. Rev. Clin. Oncol. 16, 105–122. doi:10.1038/s41571-018-0115-y
Katoh, M., and Katoh, M. (2017). Molecular Genetics and Targeted Therapy of WNT-Related Human Diseases (Review). Int. J. Mol. Med. 40, 587–606. doi:10.3892/ijmm.2017.3071
Katoh, M., and Katoh, M. (2020). Precision Medicine for Human Cancers with Notch Signaling Dysregulation (Review). Int. J. Mol. Med. 45, 279–297. doi:10.3892/ijmm.2019.4418
Katoh, M., and Katoh, M. (2007). WNT Signaling Pathway and Stem Cell Signaling Network. Clin. Cancer Res. 13, 4042–4045. doi:10.1158/1078-0432.CCR-06-2316
Lambrechts, D., Wauters, E., Boeckx, B., Aibar, S., Nittner, D., Burton, O., et al. (2018). Phenotype Molding of Stromal Cells in the Lung Tumor Microenvironment. Nat. Med. 24, 1277–1289. doi:10.1038/s41591-018-0096-5
Logsdon, G. A., Vollger, M. R., Hsieh, P., Mao, Y., Liskovykh, M. A., Koren, S., et al. (2021). The Structure, Function and Evolution of a Complete Human Chromosome 8. Nature 593, 101–107. doi:10.1038/s41586-021-03420-7
Marusyk, A., Janiszewska, M., and Polyak, K. (2020). Intratumor Heterogeneity: The Rosetta Stone of Therapy Resistance. Cancer Cell 37, 471–484. doi:10.1016/j.ccell.2020.03.007
Mathys, H., Davila-Velderrain, J., Peng, Z., Gao, F., Mohammadi, S., Young, J. Z., et al. (2019). Single-cell Transcriptomic Analysis of Alzheimer's Disease. Nature 570, 332–337. doi:10.1038/s41586-019-1195-2
McGlynn, K. A., Petrick, J. L., and El‐Serag, H. B. (2021). Epidemiology of Hepatocellular Carcinoma. Hepatology 73 (Suppl. 1), 4–13. doi:10.1002/hep.31288
Nagendran, M., Chen, Y., Lovejoy, C. A., Gordon, A. C., Komorowski, M., Harvey, H., et al. (2020). Artificial Intelligence versus Clinicians: Systematic Review of Design, Reporting Standards, and Claims of Deep Learning Studies. BMJ 368, m689. doi:10.1136/bmj.m689
Nishiga, M., Wang, D. W., Han, Y., Lewis, D. B., and Wu, J. C. (2020). COVID-19 and Cardiovascular Disease: from Basic Mechanisms to Clinical Perspectives. Nat. Rev. Cardiol. 17, 543–558. doi:10.1038/s41569-020-0413-9
Oh, D.-Y., and Bang, Y.-J. (2020). HER2-targeted Therapies - a Role beyond Breast Cancer. Nat. Rev. Clin. Oncol. 17, 33–48. doi:10.1038/s41571-019-0268-3
Ohannessian, R., Duong, T. A., and Odone, A. (2020). Global Telemedicine Implementation and Integration within Health Systems to Fight the COVID-19 Pandemic: A Call to Action. JMIR Public Health Surveill. 6, e18810. doi:10.2196/18810
Paik, P. K., Felip, E., Veillon, R., Sakai, H., Cortot, A. B., Garassino, M. C., et al. (2020). Tepotinib in Non-small-cell Lung Cancer with MET Exon 14 Skipping Mutations. N. Engl. J. Med. 383, 931–943. doi:10.1056/NEJMoa2004407
Patel, S. Y., Mehrotra, A., Huskamp, H. A., Uscher-Pines, L., Ganguli, I., and Barnett, M. L. (2021). Trends in Outpatient Care Delivery and Telemedicine during the COVID-19 Pandemic in the US. JAMA Intern. Med. 181, 388–391. doi:10.1001/jamainternmed.2020.5928
Pauli, C., Hopkins, B. D., Prandi, D., Shaw, R., Fedrizzi, T., Sboner, A., et al. (2017). Personalized In Vitro and In Vivo Cancer Models to Guide Precision Medicine. Cancer Discov. 7, 462–477. doi:10.1158/2159-8290.CD-16-1154
Pembroke, W. G., Hartl, C. L., and Geschwind, D. H. (2021). Evolutionary Conservation and Divergence of the Human Brain Transcriptome. Genome Biol. 22, 52. doi:10.1186/s13059-020-02257-z
Poewe, W., Seppi, K., Tanner, C. M., Halliday, G. M., Brundin, P., Volkmann, J., et al. (2017). Parkinson Disease. Nat. Rev. Dis. Primers 3, 17013. doi:10.1038/nrdp.2017.13
Polack, F. P., Thomas, S. J., Kitchin, N., Absalon, J., Gurtman, A., Lockhart, S., et al. (2020). Safety and Efficacy of the BNT162b2 mRNA Covid-19 Vaccine. N. Engl. J. Med. 383, 2603–2615. doi:10.1056/NEJMoa2034577
Powles, T., Rosenberg, J. E., Sonpavde, G. P., Loriot, Y., Durán, I., Lee, J.-L., et al. (2021). Enfortumab Vedotin in Previously Treated Advanced Urothelial Carcinoma. N. Engl. J. Med. 384, 1125–1135. doi:10.1056/NEJMoa2035807
Ramani, A., Müller, L., Ostermann, P. N., Gabriel, E., Abida‐Islam, P., Müller‐Schiffmann, A., et al. (2020). SARS ‐CoV‐2 Targets Neurons of 3D Human Brain Organoids. EMBO J. 39, e106230. doi:10.15252/embj.2020106230
Ramasamy, M. N., Minassian, A. M., Ewer, K. J., Flaxman, A. L., Folegatti, P. M., Owens, D. R., et al. (2021). Safety and Immunogenicity of ChAdOx1 nCoV-19 Vaccine Administered in a Prime-Boost Regimen in Young and Old Adults (COV002): a Single-Blind, Randomised, Controlled, Phase 2/3 Trial. Lancet 396, 1979–1993. doi:10.1016/S0140-6736(20)32466-1
Rheinbay, E., Parasuraman, P., Grimsby, J., Tiao, G., Engreitz, J. M., Kim, J., et al. (2017). Recurrent and Functional Regulatory Mutations in Breast Cancer. Nature 547, 55–60. doi:10.1038/nature22992
Rhie, A., McCarthy, S. A., Fedrigo, O., Damas, J., Formenti, G., Koren, S., et al. (2021). Towards Complete and Error-free Genome Assemblies of All Vertebrate Species. Nature 592, 737–746. doi:10.1038/s41586-021-03451-0
Rockx, B., Kuiken, T., Herfst, S., Bestebroer, T., Lamers, M. M., Oude Munnink, B. B., et al. (2020). Comparative Pathogenesis of COVID-19, MERS, and SARS in a Nonhuman Primate Model. Science 368, 1012–1015. doi:10.1126/science.abb7314
Roskoski, R. (2020). Properties of FDA-Approved Small Molecule Protein Kinase Inhibitors: A 2020 Update. Pharmacol. Res. 152, 104609. doi:10.1016/j.phrs.2019.104609
Rosti, G., Castagnetti, F., Gugliotta, G., and Baccarani, M. (2017). Tyrosine Kinase Inhibitors in Chronic Myeloid Leukaemia: Which, when, for Whom? Nat. Rev. Clin. Oncol. 14, 141–154. doi:10.1038/nrclinonc.2016.139
Sadoff, J., Le Gars, M., Shukarev, G., Heerwegh, D., Truyers, C., de Groot, A. M., et al. (2021). Interim Results of a Phase 1-2a Trial of Ad26.COV2.S Covid-19 Vaccine. N. Engl. J. Med. 384, 1824–1835. doi:10.1056/NEJMoa2034201
Sahai, E., Astsaturov, I., Cukierman, E., DeNardo, D. G., Egeblad, M., Evans, R. M., et al. (2020). A Framework for Advancing Our Understanding of Cancer-Associated Fibroblasts. Nat. Rev. Cancer 20, 174–186. doi:10.1038/s41568-019-0238-1
Shinde, V., Bhikha, S., Hoosain, Z., Archary, M., Bhorat, Q., Fairlie, L., et al. (2021). Efficacy of NVX-CoV2373 Covid-19 Vaccine against the B.1.351 Variant. N. Engl. J. Med. 384, 1899–1909. doi:10.1056/NEJMoa2103055
Stone, J. H., Frigault, M. J., Serling-Boyd, N. J., Fernandes, A. D., Harvey, L., Foulkes, A. S., et al. (2020). Efficacy of Tocilizumab in Patients Hospitalized with Covid-19. N. Engl. J. Med. 383, 2333–2344. doi:10.1056/NEJMoa2028836
Tomazini, B. M., Maia, I. S., Cavalcanti, A. B., Berwanger, O., Rosa, R. G., Veiga, V. C., et al. (2020). Effect of Dexamethasone on Days Alive and Ventilator-free in Patients with Moderate or Severe Acute Respiratory Distress Syndrome and COVID-19. JAMA 324, 1307–1316. doi:10.1001/jama.2020.17021
Travaglini, K. J., Nabhan, A. N., Penland, L., Sinha, R., Gillich, A., Sit, R. V., et al. (2020). A Molecular Cell Atlas of the Human Lung from Single-Cell RNA Sequencing. Nature 587, 619–625. doi:10.1038/s41586-020-2922-4
Valkenburg, K. C., de Groot, A. E., and Pienta, K. J. (2018). Targeting the Tumour Stroma to Improve Cancer Therapy. Nat. Rev. Clin. Oncol. 15, 366–381. doi:10.1038/s41571-018-0007-1
Vitale, I., Shema, E., Loi, S., and Galluzzi, L. (2021). Intratumoral Heterogeneity in Cancer Progression and Response to Immunotherapy. Nat. Med. 27, 212–224. doi:10.1038/s41591-021-01233-9
Wang, B., Liu, Y., Qian, J., and Parker, S. K. (2021). Achieving Effective Remote Working during the COVID‐19 Pandemic: A Work Design Perspective. Appl. Psychol. 70, 16–59. doi:10.1111/apps.1229010.1111/apps.12290
Weinreich, D. M., Sivapalasingam, S., Norton, T., Ali, S., Gao, H., Bhore, R., et al. (2021). REGN-COV2, a Neutralizing Antibody Cocktail, in Outpatients with Covid-19. N. Engl. J. Med. 384, 238–251. doi:10.1056/NEJMoa2035002
West, R., Michie, S., Rubin, G. J., and Amlôt, R. (2020). Applying Principles of Behaviour Change to Reduce SARS-CoV-2 Transmission. Nat. Hum. Behav. 4, 451–459. doi:10.1038/s41562-020-0887-9
Wilcock, P., and Webster, R. M. (2021). The Breast Cancer Drug Market. Nat. Rev. Drug Discov. 20, 339–340. doi:10.1038/d41573-021-00018-6
Williamson, C. T., Miller, R., Pemberton, H. N., Jones, S. E., Campbell, J., Konde, A., et al. (2016). ATR Inhibitors as a Synthetic Lethal Therapy for Tumours Deficient in ARID1A. Nat. Commun. 7, 13837. doi:10.1038/ncomms13837
Wong, M., Mayoh, C., Lau, L. M. S., Khuong-Quang, D.-A., Pinese, M., Kumar, A., et al. (2020). Whole Genome, Transcriptome and Methylome Profiling Enhances Actionable Target Discovery in High-Risk Pediatric Cancer. Nat. Med. 26, 1742–1753. doi:10.1038/s41591-020-1072-4
Worldometer (2021). Covid-19 Coronavirus Pandemic. Available at: https://www.worldometers.info/coronavirus (Accessed May 12, 2021).
Yap, T. A., Tan, D. S. P., Terbuch, A., Caldwell, R., Guo, C., Goh, B. C., et al. (2020). First-in-human Trial of the Oral Ataxia Telangiectasia and RAD3-Related (ATR) Inhibitor BAY 1895344 in Patients with Advanced Solid Tumors. Cancer Discov. 11, 80–91. doi:10.1158/2159-8290.CD-20-0868
Yu, P., Wang, Y., Yu, Y., Wang, A., Huang, L., Zhang, Y., et al. (2021). Deep Targeted Sequencing and its Potential Implication for Cancer Therapy in Chinese Patients with Gastric Adenocarcinoma. Oncol. 26, e756–e768. doi:10.1002/onco.13695
Zhao, L., Zhou, S., and Gustafsson, J.-Å. (2019). Nuclear Receptors: Recent Drug Discovery for Cancer Therapies. Endocr. Rev. 40, 1207–1249. doi:10.1210/er.2018-00222
Zhou, F., Yu, T., Du, R., Fan, G., Liu, Y., Liu, Z., et al. (2020a). Clinical Course and Risk Factors for Mortality of Adult Inpatients with COVID-19 in Wuhan, China: a Retrospective Cohort Study. The Lancet 395, 1054–1062. doi:10.1016/S0140-6736(20)30566-3
Zhou, Y., Wang, F., Tang, J., Nussinov, R., and Cheng, F. (2020b). Artificial Intelligence in COVID-19 Drug Repurposing. The Lancet Digital Health 2, e667–e676. doi:10.1016/S2589-7500(20)30192-8
Zhu, X., Mannar, D., Srivastava, S. S., Berezuk, A. M., Demers, J.-P., Saville, J. W., et al. (2021). Cryo-electron Microscopy Structures of the N501Y SARS-CoV-2 Spike Protein in Complex with ACE2 and 2 Potent Neutralizing Antibodies. Plos Biol. 19, e3001237. doi:10.1371/journal.pbio.3001237
Ziegler, C. G. K., Allon, S. J., Nyquist, S. K., Mbano, I. M., Miao, V. N., Tzouanas, C. N., et al. (2020). SARS-CoV-2 Receptor ACE2 Is an Interferon-Stimulated Gene in Human Airway Epithelial Cells and Is Detected in Specific Cell Subsets across Tissues. Cell 181, 1016–e19. doi:10.1016/j.cell.2020.04.035
Keywords: antibody-based biologics, artificial intelligence, clinical genome sequencing, COVID-19, FGF, reprogramming of tissue microenvironment, spatial biology, WNT
Citation: Katoh M and Katoh M (2021) Grand Challenges in Molecular Medicine for Disease Prevention and Treatment Through Cyclical Innovation. Front. Mol. Med. 1:720577. doi: 10.3389/fmmed.2021.720577
Received: 04 June 2021; Accepted: 28 June 2021;
Published: 15 July 2021.
Edited by:
Frank Emmert-Streib, Tampere University, FinlandCopyright © 2021 Katoh and Katoh. This is an open-access article distributed under the terms of the Creative Commons Attribution License (CC BY). The use, distribution or reproduction in other forums is permitted, provided the original author(s) and the copyright owner(s) are credited and that the original publication in this journal is cited, in accordance with accepted academic practice. No use, distribution or reproduction is permitted which does not comply with these terms.
*Correspondence: Masaru Katoh, bWthdG9oLWtrckB1bWluLmFjLmpw
†ORCID Masaru Katoh, http://orcid.org/0000-0003-3274-4066