- 1Department of Emergency Medicine, The Affiliated Hospital, Jiangsu University, Zhenjiang, Jiangsu, China
- 2Department of Critical Care Medicine, The Affiliated Hospital, Jiangsu University, Zhenjiang, Jiangsu, China
Background: The lymphocyte-to-C-reactive protein ratio (LCR) was a novel biomarker of inflammation that had been implicated in various diseases. Nevertheless, the role of LCR in the context of sepsis patients admitted to the Intensive Care Unit (ICU) had not been thoroughly elucidated. This study aimed to determine the significance of the LCR in predicting the prognosis of sepsis patients within ICU.
Methods: A sample of sepsis patients requiring ICU care was selected from the Affiliated Hospital of Jiangsu University. These patients were then segmented into four quartiles based on their LCR levels. The primary endpoint of the study was 30-day mortality and the secondary endpoint was the occurrence of Acute Kidney Injury (AKI). Survival analysis, via the Kaplan-Meier method and log-rank test, was conducted to assess survival rates. Cox proportional hazards regression and logistic regression models were employed to investigate the association between LCR and clinical outcomes. Additional subgroup analyses were conducted to evaluate the influence of other confounding factors on the relationship between LCR and patient outcomes.
Results: A total of 1,123 patients were enrolled in this study, with a median age of 75 (65–84) years, and 707 (63.0%) of them were male. The 30-day mortality rate was 28.1%, while the incidence of AKI was 45.6%. A progressive decrease in LCR levels was found to be associated with an increased cumulative incidence of 30-day mortality (log-rank P < 0.001). Multivariable Cox proportional hazards analyses demonstrated that LCR was an independent predictor of 30-day mortality [per 1-unit increase in LCR: HR (95%CI): 0.370 (0.142–0.963); P = 0.042]. Additionally, multivariable logistic regression analysis revealed a significant association between LCR and AKI occurrence [per 1-unit increase in LCR: OR (95%CI): 0.541 (0.307–0.953); P = 0.034]. Furthermore, subgroup analysis indicated a stronger correlation for patients aged over 65 years compared to those aged 65 or younger (p for interaction <0.05) in predicting 30-day mortality or AKI occurrence based on LCR.
Conclusion: A reduction in LCR was notably linked to 30-day mortality and the occurrence of AKI in sepsis patients. These findings suggested that LCR could potentially serve as a valuable tool in identifying sepsis patients at a heightened risk of adverse outcomes.
Introduction
Sepsis is a condition that can be fatal due to an malfunctioning immune response to an infection. Currently, there are no approved specific treatments for sepsis (Chen and Wei, 2021). In developed countries, the rate of sepsis cases per 100,000 individuals had been estimated to be 437 over the past decade, with an adult mortality rate of about 17 percent. Severe sepsis, which had a higher severity, had an annual incidence of 270 cases per 100,000 people and a mortality rate of 26%. Developing and less developed countries tend to have even higher incidence and mortality rates of sepsis (Fleischmann et al., 2016). Due to the serious nature of sepsis and its associated poor prognosis, researchers had focused on identifying various risk factors that can predict the outcome of patients with critical illness (Jones et al., 2009; Gilani et al., 2014). Nevertheless, despite these endeavors, the fatality rate linked to sepsis persisted at a high level. It was therefore essential to promptly recognize and forecast the likelihood of death in individuals with sepsis to assess the gravity of the condition and determine suitable treatment courses.
The development of sepsis was associated with an imbalance between pro-inflammatory and anti-inflammatory responses (Singer et al., 2016; Chousterman et al., 2017; Liu and Sun, 2019). During the early stages of sepsis, activated immune cells released large quantities of pro-inflammatory factors, leading to a hyperimmune response and the onset of a cytokine storm (Hahn et al., 2016). C-reactive protein (CRP), synthesized in the liver, served a critical function as an inflammatory agent combating bacterial infection and sepsis (Black et al., 2004). Since its discovery by Tillet in 1930, CRP had been widely used in clinical settings as an indicator of infection response (Cha et al., 2022). Numerous studies had confirmed the clinical significance of CRP in the early diagnosis of sepsis. It was deemed a significant predictor and risk element for unfavorable consequences in individuals with sepsis (Stocker et al., 2021; Wang et al., 2013). In sepsis, anti-inflammatory cytokines were also discharged into the bloodstream, inducing immunosuppression and resulting in lymphocyte apoptosis (Heffernan et al., 2012; Menges et al., 1999; Hotchkiss et al., 2005). Lymphocytes, the primary white blood cells responsible for fighting infection and disease, played a crucial role (Grossman and Paul, 2015; Mastrogiovanni et al., 2022). Several studies had reported that lymphocytopenia, a reduced lymphocyte count was frequently observed in sepsis patients and is associated with unfavorable outcomes (Boomer et al., 2012; Drewry et al., 2014; Chung et al., 2015). At present, evaluating the prognosis of sepsis by combining multiple biochemical markers was an important focus in sepsis research (Huang et al., 2020; Can et al., 2018; Zhang et al., 2022). Studies had demonstrated that the lymphocyte-to-C-reactive protein ratio (LCR), which integrated lymphocyte and C-reactive protein levels, not only reflected the inflammatory status but also indicated immune function (Yildirim and Koca, 2021; Aoyama et al., 2022; Utsumi et al., 2022). LCR showed improved consistency compared to using lymphocyte and C-reactive protein individually, thereby enhancing the sensitivity in assessing the inflammatory status. Therefore, LCR had emerged as a valuable biomarker for the early detection and prediction of severe conditions such as coronavirus disease 2019 (COVID-19) and sepsis (Ullah et al., 2020; Li et al., 2023; Liu and Mu, 2023). However, the precise role of LCR in forecasting adverse outcomes in sepsis patients had yet to be fully understood. Therefore, the aim of this study was to examine whether LCR could serve as a novel indicator of inflammation, linked to both 30-day mortality and the incidence of acute kidney injury (AKI), among sepsis patients in the intensive care unit (ICU).
Methods
Study population
This retrospective study was carried out at the Affiliated Hospital of Jiangsu University in Zhenjiang, China, spanning from January 2015 to November 2023. Data from 1,488 patients were extracted from the electronic medical record system. Sepsis was defined according to the Third International Consensus Definitions for Sepsis (Sepsis-3) (Singer et al., 2016), which specified that it was characterized by a sequential organ failure assessment (SOFA) score of ≥2 within 24 h of admission, along with at least one site of infection. Information on initial admissions for patients aged 18 years or older was gathered for this research. Additionally, the study excluded individuals who were not admitted to the ICU, those with an ICU stay of less than 24 h, individuals with a pre-existing chronic kidney disease, and those diagnosed with hepatic cirrhosis. Patients with missing data on CRP and lymphocyte on the first day of ICU admission were also excluded. Following a thorough evaluation, a total of 1,123 patients were included in the research and divided into four groups according to LCR quartiles (Figure 1). The study was designed in accordance with the Helsinki Declaration for studies involving humans. Approval for the study protocol was granted by the Affiliated Hospital of Jiangsu University (No. KY 2023K1007). Written informed consent was obtained from all participants involved.
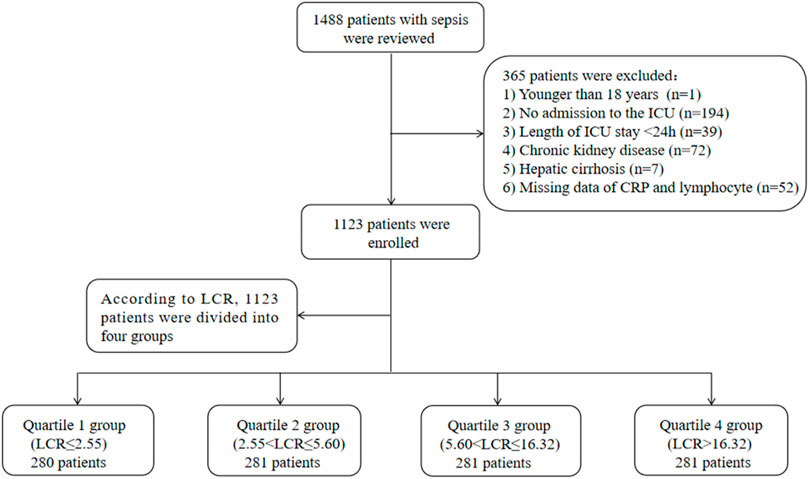
Figure 1. Flow of included patients through the trial. Abbreviations: LCR, Lymphocyte-to-C-Reactive Protein ratio; ICU, Intensive Care Unit.
Variable extraction
The potential variables for this study were extracted from the electronic medical record system and can be categorized into six main groups: demographic information, comorbidities, infection pathogens, laboratory indicators, severity of illness scores, and treatments. A comprehensive list of the extracted variables was included in Table 1. Patient follow-up began upon admission and ended upon death or discharge. All laboratory indicators and illness severity scores were documented on the first day in the ICU. To avoid possible bias, variables were excluded if they had more than 20% missing values. Variables with missing data less than 20% were processed by multiple imputation using a random forest algorithm (Blazek et al., 2021; Austin et al., 2021). The value of LCR was calculated as [1,000*lymphocyte count (×109 cells/L)]/[CRP (mg/L)]. Subsequently, patients were divided into four groups based on their LCR quartile ranges: Q1 (LCR ≤ 2.55, ≤ 25th percentile), Q2 (2.55 < LCR ≤ 5.60, 25th–50th percentile), Q3 (5.60 < LCR ≤ 16.32, 50th–75th percentile), and Q4 (LCR > 16.32, > 75th percentile).
Primary endpoint and secondary endpoint
The primary endpoint of the current study was 30-day mortality, while the secondary endpoint was the occurrence of AKI in the ICU. AKI was defined according to the 2012 Kidney Disease (Kellum et al., 2013): Improving Global Outcomes Clinical Practice Guidelines (KDIGO) as an increase in serum creatinine of 0.3 mg/dL within 48 h, a rise of at least 1.5 times the baseline level within the preceding 7 days, or a decrease in urine output to less than 0.5 mL/kg/h for more than 6 h. A diagnosis of AKI can be made if one or more of these criteria were met.
Statistical analysis
The PASS software was used to calculate the test’s effectiveness. A significance level (α) of 0.05 was set, with a total sample size of 1,123, resulting in a power of 92.6% to analyze the relationship between LCR and patient outcomes. The statistical analyses were performed with SPSS software version 26.0, and the figures were created using GraphPad Prism 10.0. Data that had a normal distribution were expressed as means ± standard deviations and analyzed using independent t-tests or one-way analysis of variance. Non-normally distributed data were presented as medians and interquartile ranges and were analyzed using the Mann-Whitney U-test. Categorical variables were represented as percentages and were assessed using chi-square tests. Kaplan-Meier survival analysis was utilized to examine the connection between the clinical outcomes and LCR, both as a continuous variable and in quartiles, to determine the incidence rate of clinical outcome events among groups with varying LCR levels. Differences among groups were evaluated using the log-rank test. In addition, we employed receiver operating characteristic (ROC) analysis to estimate the predictive value of the LCR for 30-day mortality and the incidence of AKI. To estimate the hazard ratio (HR) and 95% confidence interval (CI) for the risk of LCR on outcomes, Cox proportional hazards and logistic regression models were employed. The LCR was incorporated into the models as either a continuous variable or a categorical variable. Further stratified analyses were performed according to age, gender, hypertension, diabetes, smoking, and lactate level to assess the consistency of the prognostic impact of LCR on outcomes. The interaction between LCR and the stratified variables was also tested. A significance level of P < 0.05 (two-sided) was deemed statistically significant for all analyses.
Results
Study population
The study included 1,123 patients diagnosed with sepsis. Table 2 provided an overview of the initial characteristics of these patients. The median age of the enrolled patients was 75 (IQR: 65–84) years. Among the patients, 707 (63.0%) were male, and the median BMI was 22.49 (IQR: 20.08–25.21) kg/m2. In terms of comorbidities, 579 (51.3%) patients had hypertension, 309 (27.5%) had diabetes, 161 (14.3%) had cerebral infarction, 116 (10.3%) had coronary artery disease, and 87 (7.7%) had COPD. Upon admission to the ICU, the median levels of Lym and CRP were 0.6 (IQR: 0.3–0.9) * 109/L and 104.2 (IQR: 42.0–163.2) mg/L, respectively. The median LCR was 5.62 (IQR: 2.56–16.35). The mortality rate within 30 days was 28.1%, while the incidence of AKI was 45.6%. Please refer to Table 2 for additional variables and details.
Baseline characteristics
In Table 2, the baseline characteristics were divided into quartiles according to the LCR. The median values of LCR for each quartile were as follows: 1.67 (IQR: 1.14–2.08), 3.91 (IQR: 3.09–4.65), 9.16 (IQR: 7.21–11.51), and 64.71 (IQR: 29.01–197.56). Patients with higher LCR demonstrated a lower prevalence of smoking, Gram-positive bacterial and fungal infections, lower levels of WBC, Neu, CRP, Tbil, AST, glucose, creatinine, BUN, uric acid, and lactate. On the other hand, they exhibited higher levels of Lym, Mon, Hb, PLT, and albumin, as well as a higher severity of SOFA score. Additionally, patients in the higher LCR group had a lower proportion of vasoactive drug usage compared to the lower LCR group. With an increase in LCR, there was a gradual decrease in the following: 30-day mortality rate (36.8% vs. 33.1% vs. 26.3% vs. 16.4%, P < 0.001), occurrence of AKI (58.9% vs. 50.2% vs. 41.6% vs. 31.7%, P < 0.001), length of stay in the ICU (5 days vs. 6 days vs. 7 days vs. 6 days, P < 0.001), 60-day mortality rate (42.1% vs. 38.4% vs. 32.4% vs. 20.6%, P < 0.001), ICU mortality rate (40.7% vs. 36.7% vs. 30.6% vs. 19.6%, P < 0.001), and hospital mortality rate (42.9% vs. 38.8% vs. 32.4% vs. 21.0%, P < 0.001). Due to the link between the Q4 group and low 30-day mortality, a further comparison was conducted between Q4 and the combined first to third quartiles (Q1-3). This analysis revealed that different grouping approaches yielded similar results (Supplementary Table S1).
Table 3 presented the variances in baseline characteristics between survivors and non-survivors over their 30-day hospital stay. Non-survivors tended to be older and had a lower BMI. They also had a higher prevalence of coronary artery disease, COPD, cerebral infarction, fungal and viral infections. In terms of laboratory values, Non-survivors had higher counts of WBC, Neu, CRP, Tbil, ALT, AST, creatinine, BUN, uric acid, D-dimer, and potassium. Additionally, they had elevated levels of lactate and lower levels of Lym, Hb, PLT, and albumin. Non-survivors also had higher APACHE II scores and a greater likelihood of receiving CRRT, vasoactive drugs, and invasive ventilation. Significantly, the levels of LCR were higher in the Non-survivor group compared to the Survivor group (6.84 vs. 4.09, P < 0.001). Figure 2A depicted the distribution of LCR categorized by the 30-day in-hospital mortality status.
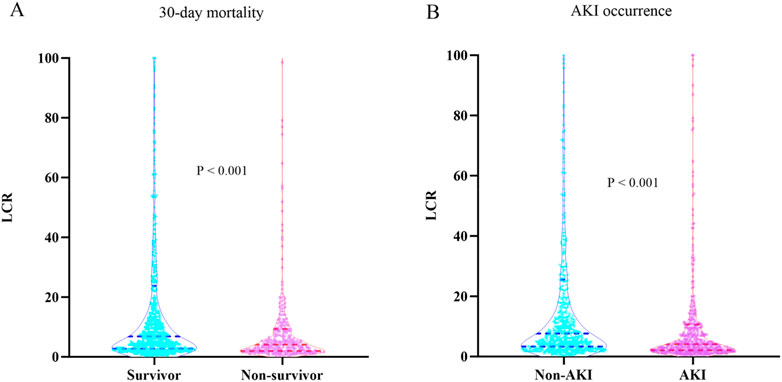
Figure 2. (A) Boxplots of the LCR showing the distribution in the Survivor group and Non-survivor group at 30 days. (B) Boxplots of the LCR showing the distribution in the Non-AKI group and AKI group. Abbreviations: LCR, Lymphocyte-to-C-Reactive Protein ratio; AKI, Acute kidney injury.
Association between the 30-day mortality and LCR
Based on the Kaplan-Meier survival analysis curves, it was observed that patients with higher LCR levels had a reduced risk of 30-day mortality (Figure 3). Additionally, Figure 4A indicated a decreasing trend in mortality rates with higher LCR. Furthermore, our analysis demonstrated that the LCR had a higher predictive power for 30-day mortality (AUC: 0.620: 95% CI: 0.585–0.656; P < 0.001) compared to the other indicators, such as WBC, Neu, lymphocyte, CRP, albumin, APACHE II score, and SOFA score (Supplementary Table S2). Supplementary Table S3 displayed the outcomes of the univariate COX regression analysis for the risk of 30-day mortality in sepsis patients. Significant variables from the univariate analysis (P < 0.05) were included, along with factors recommended by clinicians based on their expertise, as independent variables for the COX regression analysis. The influential factors that were identified included LCR, age, BMI, Neu, Hb, lactate, APACHE II score, and invasive ventilation. To investigate the association between LCR and 30-day mortality, multivariate Cox proportional risk analysis was conducted. The results indicated that LCR was a notable risk factor in the unadjusted model [HR (95%CI): 0.351 (0.131–0.938); P = 0.037], partly adjusted model [HR (95%CI): 0.354 (0.130–0.969); P = 0.043], and fully adjusted model [HR (95%CI): 0.370 (0.142–0.963); P = 0.042] when LCR was treated as a continuous variable. When LCR was considered as a categorical variable, patients in the higher quartile of LCR had a substantially lower risk of 30-day mortality compared to those in the lowest quartile, as observed in the three established Cox proportional hazards models: unadjusted model [HR (95%CI): 0.446 (0.315–0.631); P < 0.001], partly adjusted model [HR (95%CI): 0.443 (0.311–0.631); P < 0.001], and fully adjusted model [HR (95%CI): 0.480 (0.333–0.692); P < 0.001] (Table 4). Additionally, there was a downward tendency for the risk of 30-day mortality to increase with the LCR, as shown in Figure 5A.
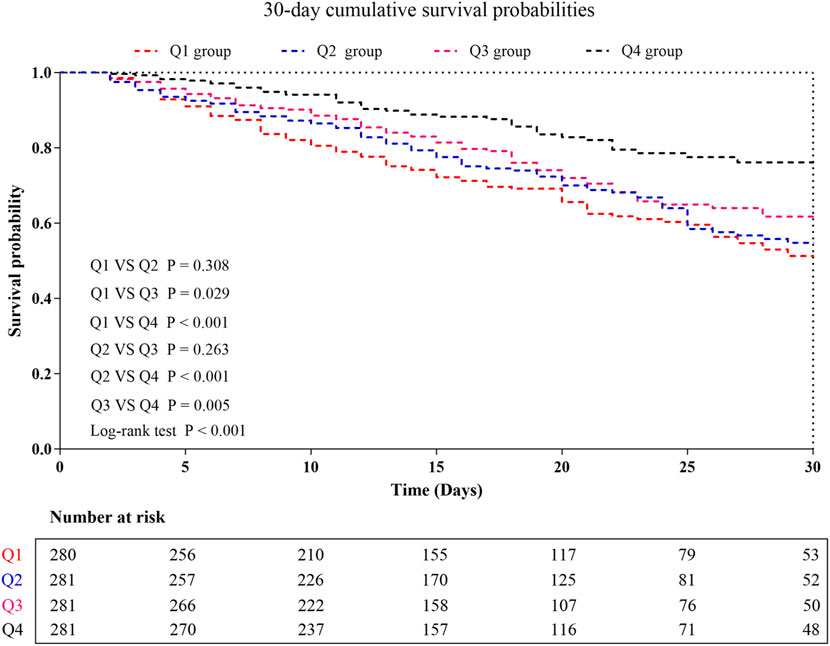
Figure 3. Kaplan–Meier curves showing cumulative probability of 30-day mortality. LCR quartiles: Q1 group (LCR ≤ 2.55); Q2 group (2.55 < LCR ≤ 5.60); Q3 group (5.60 < LCR ≤ 16.32); Q4 group (LCR > 16.32).
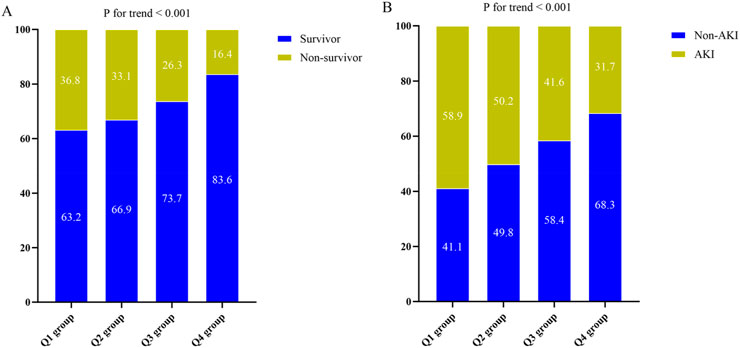
Figure 4. (A) The prevalence of 30-day mortality ratio among different quartiles of LCR. (B) The prevalence of AKI occurrence ratio among different quartiles of LCR. LCR quartiles: Q1 group (LCR ≤ 2.55); Q2 group (2.55 < LCR ≤ 5.60); Q3 group (5.60 < LCR ≤ 16.32); Q4 group (LCR > 16.32). Abbreviations: LCR, Lymphocyte-to-C-Reactive Protein ratio; AKI, Acute kidney injury.
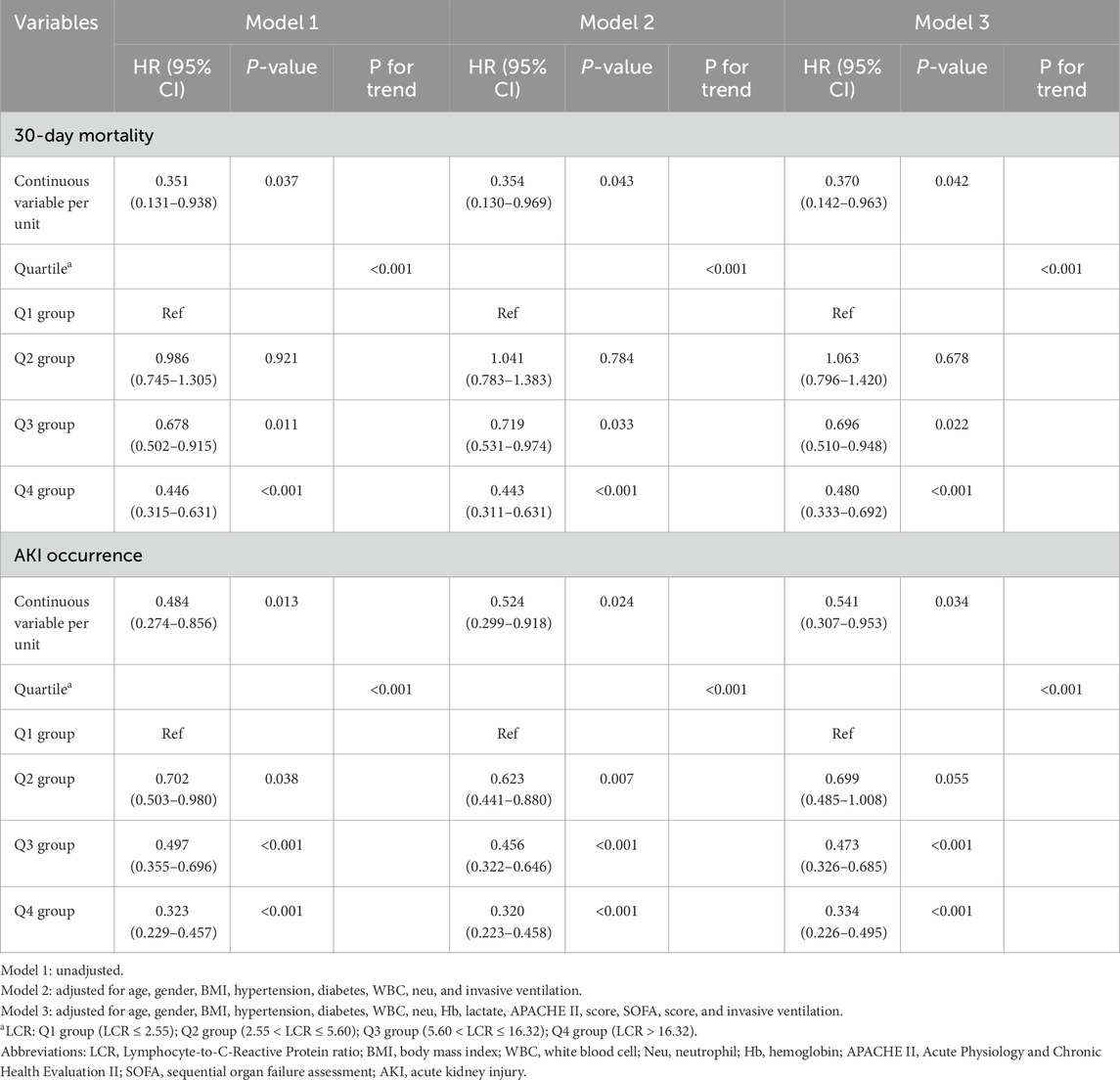
Table 4. Cox proportional hazards and logistic regression models for 30-day mortality and AKI occurrence.
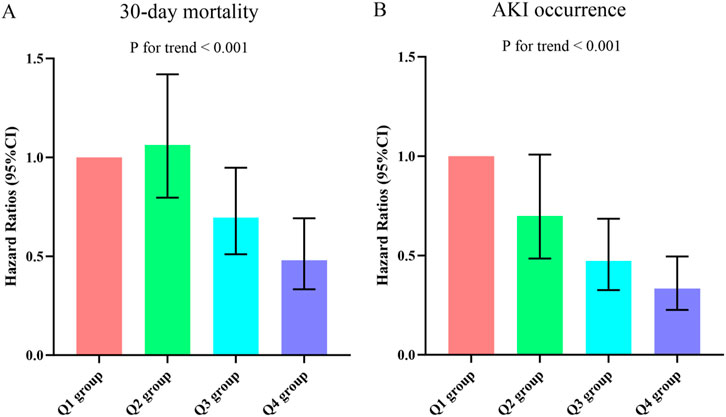
Figure 5. Hazard ratios (95% CIs) for 30-day mortality (A) and AKI occurrence (B) according to LCR quartiles after adjusting for age, gender, BMI, hypertension, diabetes, WBC, Neu, Hb, lactate, APACHE II score, SOFA score, and invasive ventilation. Error bars indicate 95% CIs. The first quartile is the reference. LCR quartiles: Q1 group (LCR ≤ 2.55); Q2 group (2.55 < LCR ≤ 5.60); Q3 group (5.60 < LCR ≤ 16.32); Q4 group (LCR > 16.32). LCR, Lymphocyte-to-C-Reactive Protein ratio; BMI, body mass index; WBC, white blood cell; Neu, neutrophil; Hb, hemoglobin; APACHE II, Acute Physiology and Chronic Health Evaluation II; SOFA, Sequential Organ Failure Assessment; AKI, Acute kidney injury.
Additionally, we conducted a further analysis of the risk stratification value of LCR for 30-day mortality in various subgroups of the enrolled patients, including age, gender, hypertension, diabetes, smoking, and lactate level. Among sepsis patients, the LCR was found to be significantly associated with a higher risk of 30-day mortality in two subgroups: those aged over 65 years [HR (95%CI): 0.289 (0.094–0.884); P = 0.030] and non-smokers [HR (95%CI): 0.253 (0.070–0.905); P = 0.035]. Interestingly, it was observed that the predictive value of LCR appeared to be more pronounced in patients aged > 65 years compared to those aged ≤ 65 years [HR (95%CI) aged > 65 years 0.289 (0.094–0.884) vs. Aged ≤ 65 years 0.092 (0.001–16.284); P for interaction = 0.028] (Figure 6).
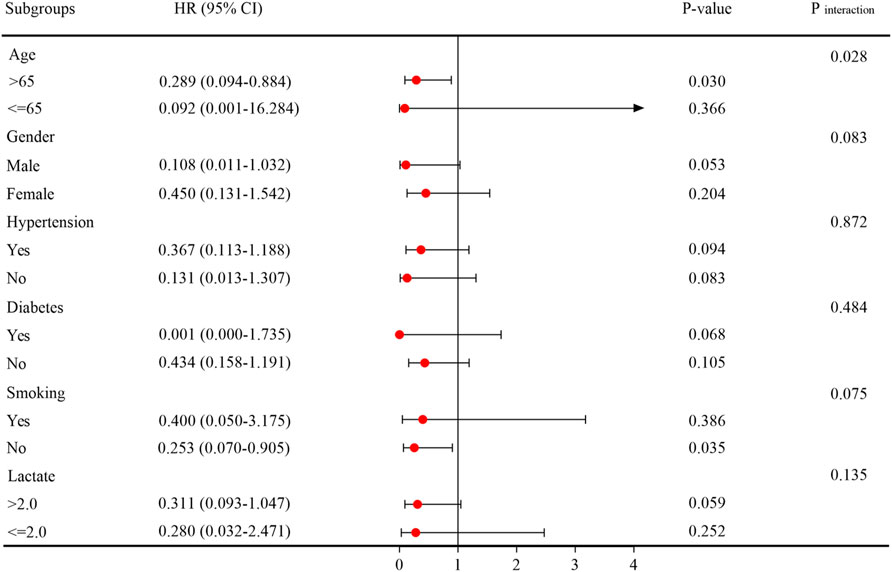
Figure 6. Subgroup analysis regarding the influence of different LCR in the 30-day mortality. Abbreviations: LCR, Lymphocyte-to-C-Reactive Protein ratio.
Relationship between LCR and AKI occurrence
The incidence of AKI varied significantly across the LCR groups (Q1: 58.9% vs. Q2: 50.2% vs. Q3: 41.6% vs. Q4: 31.7%, P < 0.001) (Table 2), suggesting a decreasing trend in AKI occurrence with higher LCR levels (Figure 4B). Additionally, the AUC for the LCR in discriminating AKI occurrence was 0.625 (95%CI: 0.592–0.657, P < 0.001). The cut-off value for the LCR to predict AKI occurrence was determined to be 4.49 (Supplementary Table S2). Table 3 displayed the differences in baseline characteristics between patients without AKI and those who developed AKI during their ICU stay. The AKI group had a higher prevalence of diabetes, coronary artery disease, Gram-negative bacteria, fungus, and virus infections. Furthermore, they exhibited elevated levels of WBC, Neu, CRP, LCR, Tbil, ALT, AST, glucose, creatinine, uric acid, D-dimer, and potassium, as well as lower levels of Lym, Hb, PLT, and albumin. Additionally, the AKI group had higher severity scores such as APACHE II score and SOFA score and a higher proportion of patients receiving CRRT, vasoactive drugs, and invasive ventilation. Compared to individuals without AKI, those with AKI had higher rates of 30-day mortality, 60-day mortality, ICU mortality, hospital mortality, longer ICU stays, and shorter hospital stays. Figure 2B illustrateed the distribution of the LCR according to AKI occurrence.
Supplementary Table S4 presented the findings of a binary logistic regression analysis that examined the association between the occurrence of AKI in sepsis patients and the LCR variable. In the analysis, which included variables from the univariate analysis, the results showed a significant association between LCR and AKI occurrence across all models. When LCR was considered as a continuous variable, the unadjusted model indicated a HR of 0.484 (95% CI: 0.274–0.856) with a P-value of 0.013. Similarly, the partially adjusted model yielded a HR of 0.524 (95% CI: 0.299–0.918) with a p-value of 0.024, and the fully adjusted model showed a HR of 0.541 (95% CI: 0.307–0.953) with a p-value of 0.034. When LCR was analyzed as a nominal variable, the risk of AKI occurrence displayed a decreasing trend with increasing LCR quartiles. Specifically, compared to the Q1 group, the risk of AKI occurrence was lower in the Q2 group, Q3 group, and Q4 group. The HR (95% CI) values for Q2 group, Q3 group, and Q4 group were 0.699 (0.485–1.008), 0.473 (0.326–0.685), and 0.334 (0.226–0.495) respectively (Table 4). This trend was found to be statistically significant, with a P-value for trend <0.001 (Figure 5B).
Additionally, we conducted stratified analyses to explore the relationship between LCR and AKI occurrence based on potential modifiers, including age, gender, hypertension, diabetes, smoking, and lactate level. These findings were presented in Figure 7. We found a significant association between LCR and an increased risk of AKI in a subgroup of those aged > 65 years [HR (95%CI): 0.331 (0.151–0.727); P = 0.006], those without hypertension [HR (95%CI): 0.213 (0.046–0.979); P = 0.047], those without diabetes [HR (95%CI): 0.416 (0.188–0.923); P = 0.031], those without smoking [HR (95%CI): 0.494 (0.268–0.907); P = 0.023], and those with lactate level > 2.0 mmol/L [HR (95%CI): 0.338 (0.142–0.802); P = 0.014]. Interestingly, the impact of LCR on AKI risk appeared more significant in patients aged > 65 years compared to those aged ≤ 65 years, as indicated by the HR of 0.331 (95%CI: 0.151–0.727) in the former group and 0.916 (95%CI: 0.412–2.036) in the latter group. This interaction was statistically significant, with a P-value for interaction of 0.045.
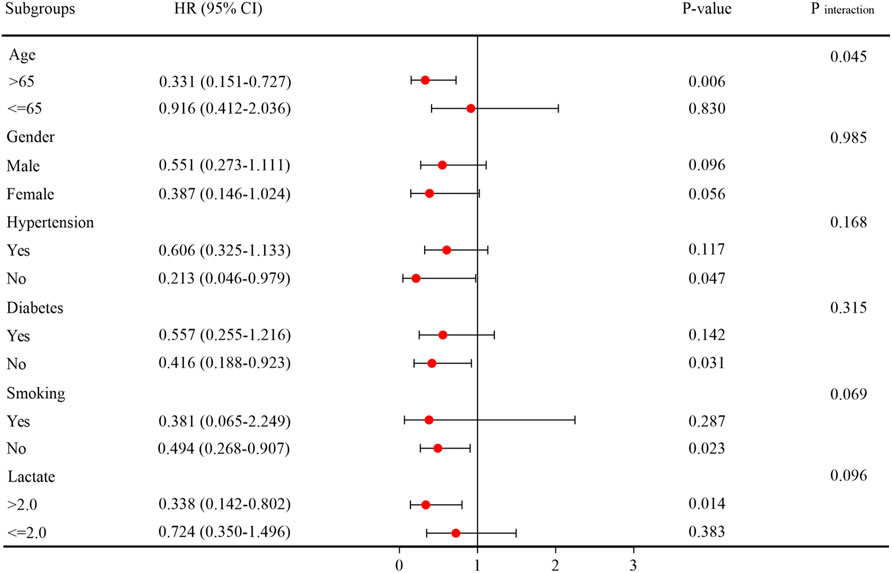
Figure 7. Subgroup analysis regarding the influence of different LCR in the AKI occurrence. Abbreviations: LCR, Lymphocyte-to-C-Reactive Protein ratio; AKI, Acute kidney injury.
Discussion
In this study, we had identified a specific correlation between the LCR and adverse outcomes in ICU patients with sepsis. Our findings indicated that a decreased LCR served as a strong and independent predictor of both 30-day mortality and the occurrence of AKI in ICU patients with sepsis. Notably, this association remained significant even after considering a wide range of clinical and laboratory variables. The results indicated that a lower LCR can be considered a novel indicator of poorer prognosis. Consequently, the LCR showed potential as a valuable tool for clinicians in making decisions and may be an independent risk factor for ICU patients with sepsis.
Sepsis had always posed a significant challenge in the clinical treatment of patients with severe infections (Grande et al., 2019). Clinical studies had demonstrated that early detection of sepsis and prompt targeted treatment of prognostic risk factors can greatly reduce mortality rates (Rhodes et al., 2017; Yin et al., 2018). Previous research had focused on examining the predictive accuracy of multiple biomarkers in assessing the prognosis of sepsis patients. These biomarkers included the neutrophil-to-lymphocyte ratio (NLR), plasma 7-ketocholesterol (7-KC), C-reactive protein-to-albumin ratio (CAR), serum S100 calcium-binding protein B (S100B), milk fat globule epidermal growth factor 8 (MFG-E8), and red blood cell distribution width (Huang et al., 2020; Liu et al., 2023a; Hu Z. D. et al., 2020; Zhang et al., 2023; Hu et al., 2023; Wu et al., 2022). Notably, the LCR had recently emerged as a novel prognostic indicator for various cancers and the severity assessment of COVID-19 (Ullah et al., 2020; Iseda et al., 2021; Takeuchi et al., 2021; Okugawa et al., 2020; Angin et al., 2021). However, the potential prognostic value of LCR in sepsis patients remained unexplored in existing literature.
Sepsis was characterized by a systemic inflammatory response triggered by infection (Singer et al., 2016). In addition to the inflammatory responses, compensatory anti-inflammatory responses also occured, resulting in the suppression of the host’s immune function (de Pablo et al., 2014). One prominent aspect of immunosuppression observed in experimental sepsis was lymphocyte apoptosis. This process further contributed to immunosuppression, making the host more vulnerable to invading pathogens (Hotchkiss et al., 2005; Lang and Matute-Bello, 2009; Chu et al., 2021). Sepsis patients often experienced a significant decrease in lymphocyte count, which can be attributed to lymphocyte marginalization, increased apoptosis, and cell redistribution (Felmet et al., 2005). Lymphocytopenia, a common condition observed in hospitalized patients with sepsis, had been linked to elevated mortality rates (Venet et al., 2010; Adrie et al., 2017). A meta-analysis indicated that individuals experiencing lymphocytopenia faced a threefold higher risk of developing severe sepsis (Zhao et al., 2020). A retrospective study conducted in Spain revealed that sepsis patients with concurrent lymphocytopenia exhibited increased rates of ICU admission and higher mortality rates (Cilloniz et al., 2021). Additionally, a study by Sheikh Motahar Vahedi et al. (2019) concluded that lymphopenia independently predicted 28-day mortality in sepsis patients. Even sepsis individuals with low-normal lymphocyte counts were found to have higher short-term mortality rates compared to those with higher lymphocyte counts.
Currently, the prevailing understanding of sepsis pathophysiology was based on the balance theory of host response. According to this theory, sepsis occured when there was an imbalance in immune responses, leading to an exaggerated inflammatory reaction characterized by the release of inflammatory factors. This systemic inflammation can then trigger multiple organ failure. Consequently, inflammatory factors served as prominent markers throughout the progression of sepsis (Chousterman et al., 2017). CRP, an acute-phase protein synthesized by the liver, exhibited increased levels in response to inflammation or infection (Black et al., 2004). Higher levels of CRP were found to be indicative of a more severe disease status and a poorer prognosis in patients with sepsis (Stocker et al., 2021; Gülcher et al., 2016). A study by Cha et al. (2022) involving 2,291 elderly patients diagnosed with sepsis and admitted to the emergency department found that combining CRP levels with other inflammatory biomarkers could predict 28-day mortality. Koozi et al. (2020) discovered that an admission CRP level greater than 100 mg/L was associated with 30-day mortality in sepsis patients. Furthermore, a prospective study involving 349 patients identified CRP as an independent predictive factor for short-term mortality in sepsis (Huang et al., 2022). That being said, it was worth noting that some studies had compared CRP levels between ICU survivors and non-survivors with sepsis but did not find any significant differences (Silvestre et al., 2009; Pettilä et al., 2002; Ryoo et al., 2019). This suggested that CRP alone may not be the sole determinant of sepsis prognosis, and additional factors should be considered in predicting outcomes.
Recently, there had been a growing interest in combining lymphocyte count and CRP level to enhance their predictive value as individual markers. The LCR, an index obtained by dividing the lymphocyte count by the CRP level, had emerged as a promising marker for systemic inflammation and had garnered increased attention. In a retrospective cohort study focusing on incarcerated hernias, it was found that a low preoperative LCR level could potentially serve as a biomarker for estimating intestinal ischemia (Yildirim et al., 2021). Liu et al. (2023b) suggested that preoperative LCR served as an innovative and valuable prognostic indicator for predicting the incidence of major adverse cardiovascular events (MACEs) during hospitalization and in the long term following primary percutaneous coronary intervention in patients diagnosed with ST-segment elevation myocardial infarction (STEMI). Yildirim and Koca (2021) investigated LCR as a prognostic biomarker in colorectal cancer, and discovered that LCR outperformed other prognostic factors in predicting postoperative complications in patients undergoing colorectal cancer surgery on day 5. Hu H. et al. (2020) also demonstrated that preoperative LCR could be used as an effective and independent prognostic indicator for patients with osteosarcoma. Moreover, elevated LCR had shown prognostic value in patients with esophageal cancer, gastric cancer, and hepatocellular carcinoma (Iseda et al., 2021; Takeuchi et al., 2021; Angin et al., 2021). Additionally, LCR had been associated with infectious diseases. A meta-analysis conducted by Lagunas-Rangel (2020) based on six studies revealed a potential link between decreased LCR and the severity of COVID-19. Ullah et al. (2020) reported that a lower LCR could serve as a predictive marker for in-hospital complications and mortality in COVID-19 patients. Given its potential, LCR could be valuable in identifying and anticipating the severity and fatality of COVID-19. In a recent study involving 1035 COVID-19 patients in 2023, the authors observed that LCR was a predictive marker for severe forms of COVID-19 upon emergency department admission, suggesting its utility in identifying high-risk patients (Abensur Vuillaume et al., 2023). These findings collectively highlighted LCR as an easily accessible and objective hematological biomarker for systemic inflammation. However, limited attention had been given to the prognostic relationship between LCR and sepsis patients in the ICU in published research. As far as we know, this was the initial investigation exploring the potential impact of LCR in predicting the clinical outcomes of sepsis in patients. Our data indicated that a decreased LCR level in sepsis patients was linked to an increased risk of 30-day mortality and the occurrence of AKI. These results implied that an early reduction in LCR could function as a prospective indicator of unfavorable outcomes in sepsis patients.
Additionally, this study conducted a subgroup analysis to further analyze the risk stratification in different patient groups. The subgroup analysis results showed that the predictive significance of LCR for 30-day mortality and AKI occurrence remained stable across male and female patients. Nonetheless, there was no noteworthy correlation observed between LCR and either 30-day mortality or AKI incidence among patients with hypertension and diabetes who were part of this study. This could be attributed to reverse causality, where individuals with these underlying conditions were more inclined to receive suitable treatment or embrace healthier lifestyle choices, potentially impacting the connection between LCR and the outcomes. Another important finding of our study was the observation that patients with lower LCR values tended to be older, and the association between LCR and 30-day mortality or AKI occurrence appeared to be more pronounced in this older patient group. It was imperative for healthcare providers to give heightened consideration to older patients due to their potentially higher prevalence of comorbidities. However, our study underscored the necessity of providing equal focus on younger patients as well, considering that they may still face a high mortality rate despite their relatively younger age.
The study presented in this article had a significant strength in demonstrating the correlation between a decrease in LCR and poor outcomes in sepsis patients, establishing LCR as an important independent risk factor. However, there were several limitations that require attention. Firstly, it was important to note that this research was a single-center study with a relatively small sample size. In order to validate the accuracy of our findings, conducting multicenter studies with larger sample sizes was necessary. Secondly, because of the retrospective nature of the study, selection bias and missing data cannot be entirely ruled out. Although we established strict and clear inclusion and exclusion criteria, as well as unified data collection methods, prospective studies are still needed to confirm the prognostic value of the LCR in real-time clinical decision-making. Thirdly, despite efforts made to address confounding variables through adjustments for multiple factors and subgroup analyses, there may still be undetermined factors affecting the prognosis, such as the use of antibiotics, fluid resuscitation, corticosteroids, and organ dysfunction, which were not taken into account due to lack of available data. We will consider the importance of these research variables in future studies to ensure comprehensive results. Fourthly, all participants were exclusively Chinese patients, warranting further investigation into the association between LCR and short-term outcomes within different populations. Moreover, this analysis focused solely on the prognostic value of baseline LCR, leaving uncertainty regarding the predictive value of LCR changes during follow-up. Therefore, the predictive power of the LCR change is also needed to be evaluated in future research.
Conclusion
Our study findings highlighted the importance of LCR as a valuable indicator for predicting 30-day mortality and AKI occurrence in sepsis patients. Therefore, incorporating LCR measurement into the assessment of risk and prognosis for this patient population could prove beneficial. Furthermore, future research should concentrate on exploring whether interventions aimed at the LCR could improve clinical outcomes in these patients.
Data availability statement
The original contributions presented in the study are included in the article/Supplementary Material, further inquiries can be directed to the corresponding author.
Ethics statement
The studies involving humans were approved by the Afffliated Hospital of Jiangsu University (Approval No. KY 2023K1007). The studies were conducted in accordance with the local legislation and institutional requirements. The participants provided their written informed consent to participate in this study.
Author contributions
CS: Writing–review and editing, Writing–original draft, Supervision, Conceptualization. ZH: Writing–review and editing, Conceptualization. JZ: Writing–original draft, Supervision, Formal Analysis, Data curation, Writing–review and editing, Conceptualization.
Funding
The author(s) declare that no financial support was received for the research, authorship, and/or publication of this article.
Conflict of interest
The authors declare that the research was conducted in the absence of any commercial or financial relationships that could be construed as a potential conflict of interest.
Publisher’s note
All claims expressed in this article are solely those of the authors and do not necessarily represent those of their affiliated organizations, or those of the publisher, the editors and the reviewers. Any product that may be evaluated in this article, or claim that may be made by its manufacturer, is not guaranteed or endorsed by the publisher.
Supplementary material
The Supplementary Material for this article can be found online at: https://www.frontiersin.org/articles/10.3389/fmolb.2024.1429372/full#supplementary-material
References
Abensur Vuillaume, L., Lefebvre, F., Benhamed, A., Schnee, A., Hoffmann, M., Godoy Falcao, F., et al. (2023). Lymphocyte-to-C-Reactive protein (LCR) ratio is not accurate to predict severity and mortality in patients with COVID-19 admitted to the ED. Int. J. Mol. Sci. 24 (6), 5996. doi:10.3390/ijms24065996
Adrie, C., Lugosi, M., Sonneville, R., Souweine, B., Ruckly, S., Cartier, J. C., et al. (2017). Persistent lymphopenia is a risk factor for ICU-acquired infections and for death in ICU patients with sustained hypotension at admission. Ann. Intensive Care 7 (1), 30. doi:10.1186/s13613-017-0242-0
Angin, Y. S., Yildirim, M., Dasiran, F., and Okan, I. (2021). Could lymphocyte to C-reactive protein ratio predict the prognosis in patients with gastric cancer? ANZ J. Surg. 91 (7-8), 1521–1527. doi:10.1111/ans.16913
Aoyama, T., Nakazano, M., Nagasawa, S., Hara, K., Komori, K., Tamagawa, H., et al. (2022). The association of the lymphocyte-to-C-Reactive-Protein ratio with gastric cancer patients who receive curative treatment. Vivo 36 (1), 482–489. doi:10.21873/invivo.12728
Austin, P. C., White, I. R., Lee, D. S., and van Buuren, S. (2021). Missing data in clinical research: a tutorial on multiple imputation. Can. J. Cardiol. 37 (9), 1322–1331. doi:10.1016/j.cjca.2020.11.010
Black, S., Kushner, I., and Samols, D. (2004). C-Reactive protein. J. Biol. Chem. 279 (47), 48487–48490. doi:10.1074/jbc.R400025200
Blazek, K., van Zwieten, A., Saglimbene, V., and Teixeira-Pinto, A. (2021). A practical guide to multiple imputation of missing data in nephrology. Kidney Int. 99 (1), 68–74. doi:10.1016/j.kint.2020.07.035
Boomer, J. S., Shuherk-Shaffer, J., Hotchkiss, R. S., and Green, J. M. (2012). A prospective analysis of lymphocyte phenotype and function over the course of acute sepsis. Crit. Care 16 (3), R112. doi:10.1186/cc11404
Can, E., Hamilcikan, Ş., and Can, C. (2018). The value of neutrophil to lymphocyte ratio and platelet to lymphocyte ratio for detecting early-onset neonatal sepsis. J. Pediatr. Hematol. Oncol. 40 (4), e229–e232. doi:10.1097/MPH.0000000000001059
Cha, K., Choi, S. P., Kim, S. H., and Oh, S. H. (2022). Prognostic value of ambulation ability with albumin and C-reactive protein to predict 28-day mortality in elderly sepsis patients: a retrospective multicentre registry-based study. BMC Geriatr. 22 (1), 661. doi:10.1186/s12877-022-03339-2
Chen, J., and Wei, H. (2021). Immune intervention in sepsis. Front. Pharmacol. 12, 718089. doi:10.3389/fphar.2021.718089
Chousterman, B. G., Swirski, F. K., and Weber, G. F. (2017). Cytokine storm and sepsis disease pathogenesis. Semin. Immunopathol. 39 (5), 517–528. doi:10.1007/s00281-017-0639-8
Chu, C. M., Chiu, L. C., Yu, C. C., Chuang, L. P., Kao, K. C., Li, L. F., et al. (2021). Increased death of peripheral blood mononuclear cells after TLR4 inhibition in sepsis is not via TNF/TNF receptor-mediated apoptotic pathway. Mediat. Inflamm. 2021, 2255017. doi:10.1155/2021/2255017
Chung, K. P., Chang, H. T., Lo, S. C., Chang, L. Y., Lin, S. Y., Cheng, A., et al. (2015). Severe lymphopenia is associated with elevated plasma interleukin-15 levels and increased mortality during severe sepsis. Shock 43 (6), 569–575. doi:10.1097/SHK.0000000000000347
Cilloniz, C., Peroni, H. J., Gabarrús, A., García-Vidal, C., Pericàs, J. M., Bermejo-Martin, J., et al. (2021). Lymphopenia is associated with poor outcomes of patients with community-acquired pneumonia and sepsis. Open Forum Infect. Dis. 8 (6), ofab169. doi:10.1093/ofid/ofab169
de Pablo, R., Monserrat, J., Prieto, A., and Alvarez-Mon, M. (2014). Role of circulating lymphocytes in patients with sepsis. Biomed. Res. Int. 2014, 671087. doi:10.1155/2014/671087
Drewry, A. M., Samra, N., Skrupky, L. P., Fuller, B. M., Compton, S. M., and Hotchkiss, R. S. (2014). Persistent lymphopenia after diagnosis of sepsis predicts mortality. Shock 42 (5), 383–391. doi:10.1097/SHK.0000000000000234
Felmet, K. A., Hall, M. W., Clark, R. S., Jaffe, R., and Carcillo, J. A. (2005). Prolonged lymphopenia, lymphoid depletion, and hypoprolactinemia in children with nosocomial sepsis and multiple organ failure. J. Immunol. 174 (6), 3765–3772. doi:10.4049/jimmunol.174.6.3765
Fleischmann, C., Scherag, A., Adhikari, N. K., Hartog, C. S., Tsaganos, T., Schlattmann, P., et al. (2016). Assessment of global incidence and mortality of hospital-treated sepsis. Current estimates and limitations. Am. J. Respir. Crit. Care Med. 193 (3), 259–272. doi:10.1164/rccm.201504-0781OC
Gilani, M. T., Razavi, M., and Azad, A. M. (2014). A comparison of simplified acute Physiology score II, acute Physiology and chronic Health evaluation II and acute Physiology and chronic Health evaluation III scoring system in predicting mortality and length of stay at surgical intensive care unit. Niger. Med. J. 55 (2), 144–147. doi:10.4103/0300-1652.129651
Grande, E., Grippo, F., Frova, L., Pantosti, A., Pezzotti, P., and Fedeli, U. (2019). The increase of sepsis-related mortality in Italy: a nationwide study, 2003-2015. Eur. J. Clin. Microbiol. Infect. Dis. 38 (9), 1701–1708. doi:10.1007/s10096-019-03601-3
Grossman, Z., and Paul, W. E. (2015). Dynamic tuning of lymphocytes: physiological basis, mechanisms, and function. Annu. Rev. Immunol. 33, 677–713. doi:10.1146/annurev-immunol-032712-100027
Gülcher, S. S., Bruins, N. A., Kingma, W. P., and Boerma, E. C. (2016). Elevated C-reactive protein levels at ICU discharge as a predictor of ICU outcome: a retrospective cohort study. Ann. Intensive Care 6 (1), 5. doi:10.1186/s13613-016-0105-0
Hahn, W. O., Mikacenic, C., Price, B. L., Harju-Baker, S., Katz, R., Himmelfarb, J., et al. (2016). Host derived biomarkers of inflammation, apoptosis, and endothelial activation are associated with clinical outcomes in patients with bacteremia and sepsis regardless of microbial etiology. Virulence 7 (4), 387–394. doi:10.1080/21505594.2016.1144003
Heffernan, D. S., Monaghan, S. F., Thakkar, R. K., Machan, J. T., Cioffi, W. G., and Ayala, A. (2012). Failure to normalize lymphopenia following trauma is associated with increased mortality, independent of the leukocytosis pattern. Crit. Care 16 (1), R12. doi:10.1186/cc11157
Hotchkiss, R. S., Osmon, S. B., Chang, K. C., Wagner, T. H., Coopersmith, C. M., and Karl, I. E. (2005). Accelerated lymphocyte death in sepsis occurs by both the death receptor and mitochondrial pathways. J. Immunol. 174 (8), 5110–5118. doi:10.4049/jimmunol.174.8.5110
Hu, H., Deng, X., Song, Q., Lv, H., Chen, W., Xing, X., et al. (2020b). Prognostic value of the preoperative lymphocyte-to-C-reactive protein ratio and albumin-to-globulin ratio in patients with osteosarcoma. Onco Targets Ther. 13, 12673–12681. doi:10.2147/OTT.S287192
Hu, J., Xie, S., Li, W., and Zhang, L. (2023). Diagnostic and prognostic value of serum S100B in sepsis-associated encephalopathy: a systematic review and meta-analysis. Front. Immunol. 14, 1102126. doi:10.3389/fimmu.2023.1102126
Hu, Z. D., Lippi, G., and Montagnana, M. (2020a). Diagnostic and prognostic value of red blood cell distribution width in sepsis: a narrative review. Clin. Biochem. 77, 1–6. doi:10.1016/j.clinbiochem.2020.01.001
Huang, N., Chen, J., Wei, Y., Liu, Y., Yuan, K., Chen, J., et al. (2022). Multi-marker approach using C-reactive protein, procalcitonin, neutrophil CD64 index for the prognosis of sepsis in intensive care unit: a retrospective cohort study. BMC Infect. Dis. 22 (1), 662. doi:10.1186/s12879-022-07650-6
Huang, Z., Fu, Z., Huang, W., and Huang, K. (2020). Prognostic value of neutrophil-to-lymphocyte ratio in sepsis: a meta-analysis. Am. J. Emerg. Med. 38 (3), 641–647. doi:10.1016/j.ajem.2019.10.023
Iseda, N., Itoh, S., Yoshizumi, T., Tomiyama, T., Morinaga, A., Shimagaki, T., et al. (2021). Lymphocyte-to-C-reactive protein ratio as a prognostic factor for hepatocellular carcinoma. Int. J. Clin. Oncol. 26 (10), 1890–1900. doi:10.1007/s10147-021-01985-x
Jones, A. E., Trzeciak, S., and Kline, J. A. (2009). The Sequential Organ Failure Assessment score for predicting outcome in patients with severe sepsis and evidence of hypoperfusion at the time of emergency department presentation. Crit. Care Med. 37 (5), 1649–1654. doi:10.1097/CCM.0b013e31819def97
Kellum, J. A., and Lameire, N.KDIGO AKI Guideline Work Group (2013). Diagnosis, evaluation, and management of acute kidney injury: a KDIGO summary (Part 1). Crit. Care 17 (1), 204. doi:10.1186/cc11454
Koozi, H., Lengquist, M., and Frigyesi, A. (2020). C-reactive protein as a prognostic factor in intensive care admissions for sepsis: a Swedish multicenter study. J. Crit. Care 56, 73–79. doi:10.1016/j.jcrc.2019.12.009
Lagunas-Rangel, F. A. (2020). Neutrophil-to-lymphocyte ratio and lymphocyte-to-C-reactive protein ratio in patients with severe coronavirus disease 2019 (COVID-19): a meta-analysis. J. Med. Virol. 92 (10), 1733–1734. doi:10.1002/jmv.25819
Lang, J. D., and Matute-Bello, G. (2009). Lymphocytes, apoptosis and sepsis: making the jump from mice to humans. Crit. Care 13 (1), 109. doi:10.1186/cc7144
Li, X., Wei, Y., Xu, Z., Li, T., Dong, G., Liu, X., et al. (2023). Lymphocyte-to-C-Reactive protein ratio as an early sepsis biomarker for neonates with suspected sepsis. Mediat. Inflamm. 2023, 9077787. doi:10.1155/2023/9077787
Liu, L., and Sun, B. (2019). Neutrophil pyroptosis: new perspectives on sepsis. Cell Mol. Life Sci. 76 (11), 2031–2042. doi:10.1007/s00018-019-03060-1
Liu, X., and Mu, Y. (2023). Lymphocyte to C-reactive protein ratio as an early biomarker to distinguish sepsis from pneumonia in neonates. J. Inflamm. Res. 16, 3509–3517. doi:10.2147/JIR.S424897
Liu, Y., Gao, Y., Liang, B., and Liang, Z. (2023a). The prognostic value of C-reactive protein to albumin ratio in patients with sepsis: a systematic review and meta-analysis. Aging Male 26 (1), 2261540. doi:10.1080/13685538.2023.2261540
Liu, Y., Ye, T., Chen, L., Xu, B., Wu, G., and Zong, G. (2023b). Preoperative lymphocyte to C-reactive protein ratio: a new prognostic indicator of post-primary percutaneous coronary intervention in patients with ST-segment elevation myocardial infarction. Int. Immunopharmacol. 114, 109594. doi:10.1016/j.intimp.2022.109594
Mastrogiovanni, M., Di Bartolo, V., and Alcover, A. (2022). Cell polarity regulators, multifunctional organizers of lymphocyte activation and function. Biomed. J. 45 (2), 299–309. doi:10.1016/j.bj.2021.10.002
Menges, T., Engel, J., Welters, I., Wagner, R. M., Little, S., Ruwoldt, R., et al. (1999). Changes in blood lymphocyte populations after multiple trauma: association with posttraumatic complications. Crit. Care Med. 27 (4), 733–740. doi:10.1097/00003246-199904000-00026
Okugawa, Y., Toiyama, Y., Yamamoto, A., Shigemori, T., Ide, S., Kitajima, T., et al. (2020). Lymphocyte-C-reactive protein ratio as promising new marker for predicting surgical and oncological outcomes in colorectal cancer. Ann. Surg. 272 (2), 342–351. doi:10.1097/SLA.0000000000003239
Pettilä, V., Pentti, J., Pettilä, M., Takkunen, O., and Jousela, I. (2002). Predictive value of antithrombin III and serum C-reactive protein concentration in critically ill patients with suspected sepsis. Crit. Care Med. 30 (2), 271–275. doi:10.1097/00003246-200202000-00001
Rhodes, A., Evans, L. E., Alhazzani, W., Levy, M. M., Antonelli, M., Ferrer, R., et al. (2017). Surviving sepsis campaign: international Guidelines for management of sepsis and septic shock: 2016. Intensive Care Med. 43 (3), 304–377. doi:10.1007/s00134-017-4683-6
Ryoo, S. M., Han, K. S., Ahn, S., Shin, T. G., Hwang, S. Y., Chung, S. P., et al. (2019). The usefulness of C-reactive protein and procalcitonin to predict prognosis in septic shock patients: a multicenter prospective registry-based observational study. Sci. Rep. 9 (1), 6579. doi:10.1038/s41598-019-42972-7
Sheikh Motahar Vahedi, H., Bagheri, A., Jahanshir, A., Seyedhosseini, J., and Vahidi, E. (2019). Association of lymphopenia with short term outcomes of sepsis patients; a brief report. Arch. Acad. Emerg. Med. 7 (1), e14.
Silvestre, J., Póvoa, P., Coelho, L., Almeida, E., Moreira, P., Fernandes, A., et al. (2009). Is C-reactive protein a good prognostic marker in septic patients? Intensive Care Med. 35 (5), 909–913. doi:10.1007/s00134-009-1402-y
Singer, M., Deutschman, C. S., Seymour, C. W., Shankar-Hari, M., Annane, D., Bauer, M., et al. (2016). The third international Consensus Definitions for sepsis and septic shock (Sepsis-3). JAMA 315 (8), 801–810. doi:10.1001/jama.2016.0287
Stocker, M., van Herk, W., El Helou, S., Dutta, S., Schuerman, FABA, van den Tooren-de Groot, R. K., et al. (2021). C-reactive protein, procalcitonin, and white blood count to rule out neonatal early-onset sepsis within 36 hours: a secondary analysis of the neonatal procalcitonin intervention study. Clin. Infect. Dis. 73 (2), e383–e390. doi:10.1093/cid/ciaa876
Takeuchi, M., Kawakubo, H., Hoshino, S., Matsuda, S., Mayanagi, S., Irino, T., et al. (2021). Lymphocyte-to-C-Reactive protein ratio as a novel marker for predicting oncological outcomes in patients with esophageal cancer. World J. Surg. 45 (11), 3370–3377. doi:10.1007/s00268-021-06269-z
Ullah, W., Basyal, B., Tariq, S., Almas, T., Saeed, R., Roomi, S., et al. (2020). Lymphocyte-to-C-Reactive protein ratio: a novel predictor of adverse outcomes in COVID-19. J. Clin. Med. Res. 12 (7), 415–422. doi:10.14740/jocmr4227
Utsumi, M., Inagaki, M., Kitada, K., Tokunaga, N., Kondo, M., Sakurai, Y., et al. (2022). Preoperative lymphocyte-to-C-reactive protein ratio predicts hepatocellular carcinoma recurrence after surgery. Ann. Surg. Treat. Res. 103 (2), 72–80. doi:10.4174/astr.2022.103.2.72
Venet, F., Davin, F., Guignant, C., Larue, A., Cazalis, M. A., Darbon, R., et al. (2010). Early assessment of leukocyte alterations at diagnosis of septic shock. Shock 34 (4), 358–363. doi:10.1097/SHK.0b013e3181dc0977
Wang, H. E., Shapiro, N. I., Safford, M. M., Griffin, R., Judd, S., Rodgers, J. B., et al. (2013). High-sensitivity C-reactive protein and risk of sepsis. PLoS One 8 (7), e69232. doi:10.1371/journal.pone.0069232
Wu, W., Wang, J., Chen, J., Lu, J., Lao, Y., Huang, K., et al. (2022). MFG-E8 has guiding significance for the prognosis and treatment of sepsis. Sci. Rep. 12 (1), 20916. doi:10.1038/s41598-022-25601-8
Yildirim, M., Dasiran, F., Angin, Y. S., and Okan, I. (2021). Lymphocyte-C-reactive protein ratio: a putative predictive factor for intestinal ischemia in strangulated abdominal wall hernias. Hernia 25 (3), 733–739. doi:10.1007/s10029-020-02174-x
Yildirim, M., and Koca, B. (2021). Lymphocyte C-reactive protein ratio: a new biomarker to predict early complications after gastrointestinal oncologic surgery. Cancer Biomark. 31 (4), 409–417. doi:10.3233/CBM-210251
Yin, M., Si, L., Qin, W., Li, C., Zhang, J., Yang, H., et al. (2018). Predictive value of serum albumin level for the prognosis of severe sepsis without exogenous human albumin administration: a prospective cohort study. J. Intensive Care Med. 33 (12), 687–694. doi:10.1177/0885066616685300
Zhang, S., Zhang, W., Luan, X., and Jin, Z. (2022). Diagnostic value of neutrophil/lymphocyte ratio and platelet/lymphocyte ratio in premature rupture of membranes complicated by sepsis. J. Coll. Physicians Surg. Pak 32 (5), 602–605. doi:10.29271/jcpsp.2022.05.602
Zhang, Y., Xu, S., Xu, J., Xu, F., Lu, G., Zhou, J., et al. (2023). Prognostic value of plasma 7-ketocholesterol in sepsis. Clin. Chim. Acta 548, 117467. doi:10.1016/j.cca.2023.117467
Keywords: sepsis, lymphocyte-to-C-reactive protein ratio, acute kidney injury, inflammation, immune
Citation: Song C, Hu Z and Zhang J (2024) The value of lymphocyte-to-C-reactive protein ratio for predicting clinical outcomes in patients with sepsis in intensive care unit: a retrospective single-center study. Front. Mol. Biosci. 11:1429372. doi: 10.3389/fmolb.2024.1429372
Received: 24 May 2024; Accepted: 30 August 2024;
Published: 13 September 2024.
Edited by:
Artur Slomka, Nicolaus Copernicus University in Toruń, PolandReviewed by:
Sonal Datta, Maharishi Markandeshwar University, Mullana, IndiaXinyong Liu, Zhejiang University School of Medicine, China
Copyright © 2024 Song, Hu and Zhang. This is an open-access article distributed under the terms of the Creative Commons Attribution License (CC BY). The use, distribution or reproduction in other forums is permitted, provided the original author(s) and the copyright owner(s) are credited and that the original publication in this journal is cited, in accordance with accepted academic practice. No use, distribution or reproduction is permitted which does not comply with these terms.
*Correspondence: Jinhui Zhang, ODk0MDgwNDIzQHFxLmNvbQ==