- 1Department of Biotechnology, Guru Ghasidas Vishwavidyalaya, Bilaspur, Chhattisgarh, India
- 2Department of Botany, Sri Sadguru Jagjit Singh Namdhari College, Garhwa, Jharkhand, India
- 3Department of Surgical Gastroenterology, All India Institute of Medical Sciences, Raipur, Chhattisgarh, India
- 4Department of Pathology and Lab Medicine, All India Institute of Medical Sciences, Raipur, Chhattisgarh, India
- 5Trivitron Healthcare Pvt Ltd., Chennai, India
Gastrointestinal (GI) cancers account for one-fourth of the global cancer incidence and are incriminated to cause one-third of cancer-related deaths. GI cancer includes esophageal, gastric, liver, pancreatic, and colorectal cancers, mostly diagnosed at advanced stages due to a lack of accurate markers for early stages. The invasiveness of diagnostic methods like colonoscopy for solid biopsy reduces patient compliance as it cannot be frequently used to screen patients. Therefore, minimally invasive approaches like liquid biopsy may be explored for screening and early identification of gastrointestinal cancers. Liquid biopsy involves the qualitative and quantitative determination of certain cancer-specific biomarkers in body fluids such as blood, serum, saliva, and urine to predict disease progression, therapeutic tolerance, toxicities, and recurrence by evaluating minimal residual disease and its correlation with other clinical features. In this review, we deliberate upon various tumor-specific cellular and molecular entities such as circulating tumor cells (CTCs), tumor-educated platelets (TEPs), circulating tumor DNA (ctDNA), cell-free DNA (cfDNA), exosomes, and exosome-derived biomolecules and cite recent advances pertaining to their use in predicting disease progression, therapy response, or risk of relapse. We also discuss the technical challenges associated with translating liquid biopsy into clinical settings for various clinical applications in gastrointestinal cancers.
1 Introduction
Gastrointestinal (GI) cancers, encompassing colorectal, stomach, esophageal, liver, and pancreatic cancers, exhibit varying global incidences and mortality rates (Siegel et al., 2023a). A recent estimate reports that gastrointestinal cancers contribute to one in three cancer incidences and one in three cancer-related deaths globally and are expected to increase in the coming years (Sathishkumar et al., 2022; Kayali et al., 2023). Gastrointestinal tract cancers also result in the impairment of almost all quality of life (QOL) parameters, and therefore, it is essential to enhance cancer care and clinical outcomes for these cancer types (Dalhammar et al., 2022; Siddiqui et al., 2023). Among all GI tract cancers, colorectal cancer (CRC) stands as one of the most prevalent cancers worldwide, with mortality rates gradually decreasing due to improved treatments and early detection (Siegel et al., 2023b). Stomach or gastric cancer (GC), while experiencing a decrease in incidence in many regions, still presents significant mortality rates influenced by the stage of diagnosis and treatment accessibility (Iwu and Iwu-Jaja, 2023). The global incidence of esophageal cancer varies, with mortality rates often high due to late-stage detection (Joseph et al., 2022). Liver cancer (hepatocellular carcinoma, HCC) incidence is linked to factors like viral infections and lifestyle choices, resulting in high mortality rates, often due to late-stage diagnosis (Rattanasupar et al., 2021). Pancreatic cancer, which is challenging to treat and frequently diagnosed at advanced stages, registers lower survival rates among gastrointestinal cancers (Li C. et al., 2022). Efforts toward early detection through screenings, lifestyle modifications, and advancements in treatment remain pivotal in mitigating mortality rates across these cancers (Kayali et al., 2023).
Colorectal cancer is a major global health concern due to its high incidence rates and considerable fatality figures. It is rated as the third most typical cancer among those who are diagnosed in both men and women worldwide. Recent data show that CRC had a global incidence of 1.93 million and caused approximately 0.93 million deaths in 2020, which is expected to increase to 3.2 million new cases in 2040 (Siegel et al., 2023a; Siegel et al., 2023b). In addition to this, lower survival statistics describe the difficult environment that patients with colorectal cancer and clinicians must navigate. Despite improvements in medical treatment, this type of cancer frequently poses a significant barrier to increased life expectancy. Multiple variables, including late-stage detection, aggressive tumor behavior, and a lack of effective treatments for advanced patients, contribute to reduced survival rates (Siegel et al., 2023a; Siddiqui et al., 2023). The American Cancer Society reports that the colorectal cancer 5-year survival rate varies greatly depending on the stage at diagnosis. Patients with localized tumors (stages I–II) have a 5-year survival rate of nearly 90%, while those with stage III have a survival rate of nearly 72% compared to a much lower rate for those with stage IV and distant metastases, which is around 14% (Padilla-Ruiz et al., 2022; Siegel et al., 2023b). This discrepancy underlines the crucial significance of early detection using methods that can overcome difficulties presented by conventional diagnostic approaches and enhance the general prognosis for those affected by these illnesses. In the search for a minimally invasive and extremely sensitive method for detection, liquid biopsy has emerged as a new strategy in the diagnosis of many malignancies.
2 Prognostic cues captured through liquid biopsy have useful clinical importance
Liquid biopsies analyze circulating biomarkers, including DNA, RNA, proteins, and other chemicals contained in physiological fluids like blood, saliva, urine, stool, and pleural fluid, as opposed to conventional tissue biopsies, which can be invasive and difficult to collect (Mino-Kenudson, 2016; Raez et al., 2023). This method allows for the detection of genetic mutations and other biomarkers linked to tumors, such as circulating tumor cells (CTCs), circulating tumor DNA (ctDNA), cell-free DNA or RNA (cfDNA/cfRNA), tumor-educated platelets (TEPs), circulating tumor-derived endothelial cells (CTECs), exosomes, and protein molecules for early cancer detection (Vacante et al., 2020; Zhou et al., 2022). Since they offer clinicians access to real-time information about a patient’s cancer status without requiring repeated intrusive procedures, liquid biopsies are particularly useful in tracking therapy response and disease progression (Sivapalan et al., 2023) (Figure 1). Apart from capturing tumor heterogeneity, a liquid biopsy can facilitate recording broad temporal molecular footprints of the tumor, along with its changes throughout the disease progression to direct better clinical decisions (Lone et al., 2022; Shegekar et al., 2023) (Figure 1). The next section of this article discusses various components of body fluids that can be used as tools for liquid biopsy and are reported to predict disease states, survival outcomes, or treatment outcomes in various gastrointestinal cancers.
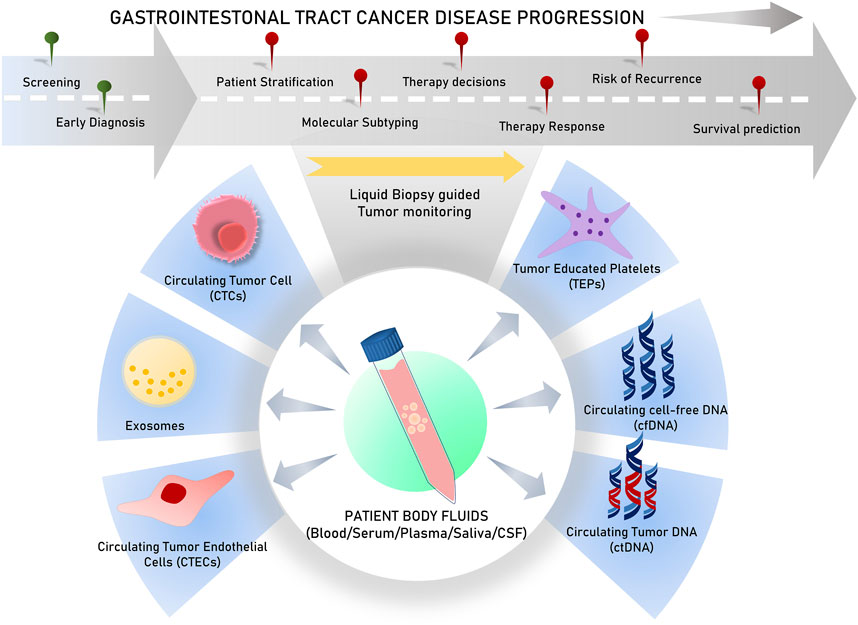
Figure 1. Schematic representation of various analytes used in liquid biopsy for varied clinical applications.
3 Circulating tumor cells
Circulating tumor cells are tumorous cells that exuviate from primary or metastatic tumors and extravasate to enter and stay in the blood and lymph circulation. These rare cells travel throughout circulation to eventually colonize a distant organ and form secondary tumors (metastasis) (Chauhan et al., 2021) (Figure 2). They exhibit considerable heterogeneity in terms of size, shape, and surface characteristics. A typical CTC is slightly larger than a white blood cell and can range from 10 to 30 um in diameter. These less frequently found cells (ranging from a few to up to hundred cells per ml of peripheral blood) can exist either as single cells or as clusters with stromal cells, platelets, or macrophages (Chen et al., 2022). Solid tumors of epithelial origin (breast, colon, prostate, etc.) usually generate CTCs, mostly expressing cytokeratins (CKs), along with epithelial cell adhesion molecules (EpCAMs). However, CTCs exhibit considerable diversity in terms of surface markers and cell types, which hinders the identification and isolation of clinically useful CTCs (Petrik et al., 2022).
CTCs, which are conventionally associated with the metastatic potential of any tumor, have potential applications in early diagnosis and screening. While considering CTCs for the early diagnosis and screening of GI tumors, it is important to consider the stage at which they manifest in a tumor (Yang C. et al., 2019). Early tumor lesions that can be detected using imaging methods, such as MRI/PET or CT scans, have approximately 109 cells and, hence, may start spreading disseminated tumor cells (DTCs) early, much before a metastatic tumor is diagnosed (Heiss et al., 1995; Friberg and Nystrom, 2015). In this regard, a study on various asymptomatic but high-risk individuals with a family history of any cancer type reported that 50% of them were positive for CTCs, while 20% developed early tumor lesions when followed up (Ried et al., 2017). Despite the theoretical and experimental rationale for considering CTCs for early diagnosis and screening, their extremely low occurrence in peripheral blood, and less sensitive technical methods to isolate them, CTC examination as a screening tool for early diagnosis in standard treatment has not been achieved, possibly because of technological and logistical limitations (Batool et al., 2023). Nevertheless, CTC detection in asymptomatic patients and patients with a family history of cancer has shown promising results in medium cohort studies, indicating its future prospects for use in screening strategies (Castro et al., 2018). However, CTCs have promising clinical applications, such as prognostic classification, metastatic monitoring, and prediction of response to therapies (Nikanjam et al., 2022) (Figure 2).
3.1 CTCs and patient groups
CTCs can also be utilized for patient stratification to provide beneficial treatment and enhance survival outcomes. In the case of CRC, CTCs are strongly associated with the stage of the disease, with more CTCs detected in advanced stages than in less advanced stages (Sastre et al., 2008; Bork et al., 2015). Notably, a study reported better diagnostic sensitivities of CTCs for early-stage CRC than serum biochemical markers, such as carcinoembryonic antigen (CEA) or CA19-9 (Yu et al., 2020). However, the CTC detection rate was not significantly associated with other clinicopathological features such as gender, location of the primary tumor (CEA), and lactate dehydrogenase (LDH) levels. However, its efficiency in detecting advanced-stage CRC remains unclear. A recent study reported that vimentin + CTCs could be used to better diagnose stage III/IV CRC (Cao et al., 2023). Moreover, research into the clinical utility of qualitative gene mutations in DNA isolated from CTCs, instead of their enumeration, has provided interesting insights. We already know that CRC-associated gene mutations with known clinical utilities, such as KRAS, APC, TP53, ERBB1/2, and FBXW7, may be evaluated in the CTC genome to predict accurate treatment options and interventions (Bidard et al., 2019) (Table 1). For instance, mutations in oncogene epithelial cell transforming sequence 2 (ECT2) were strongly associated with advanced CRCs, even more than serum CEA levels (Wang H. et al., 2019), supporting its usefulness in the prognosis of CRC (Chen et al., 2017) (Table 1). Furthermore, the expression of genes such as hTERT and MAGEA1-6 is more strongly associated with stage T3/T4 than stage T1/T2 in CRC (Kim et al., 2017).
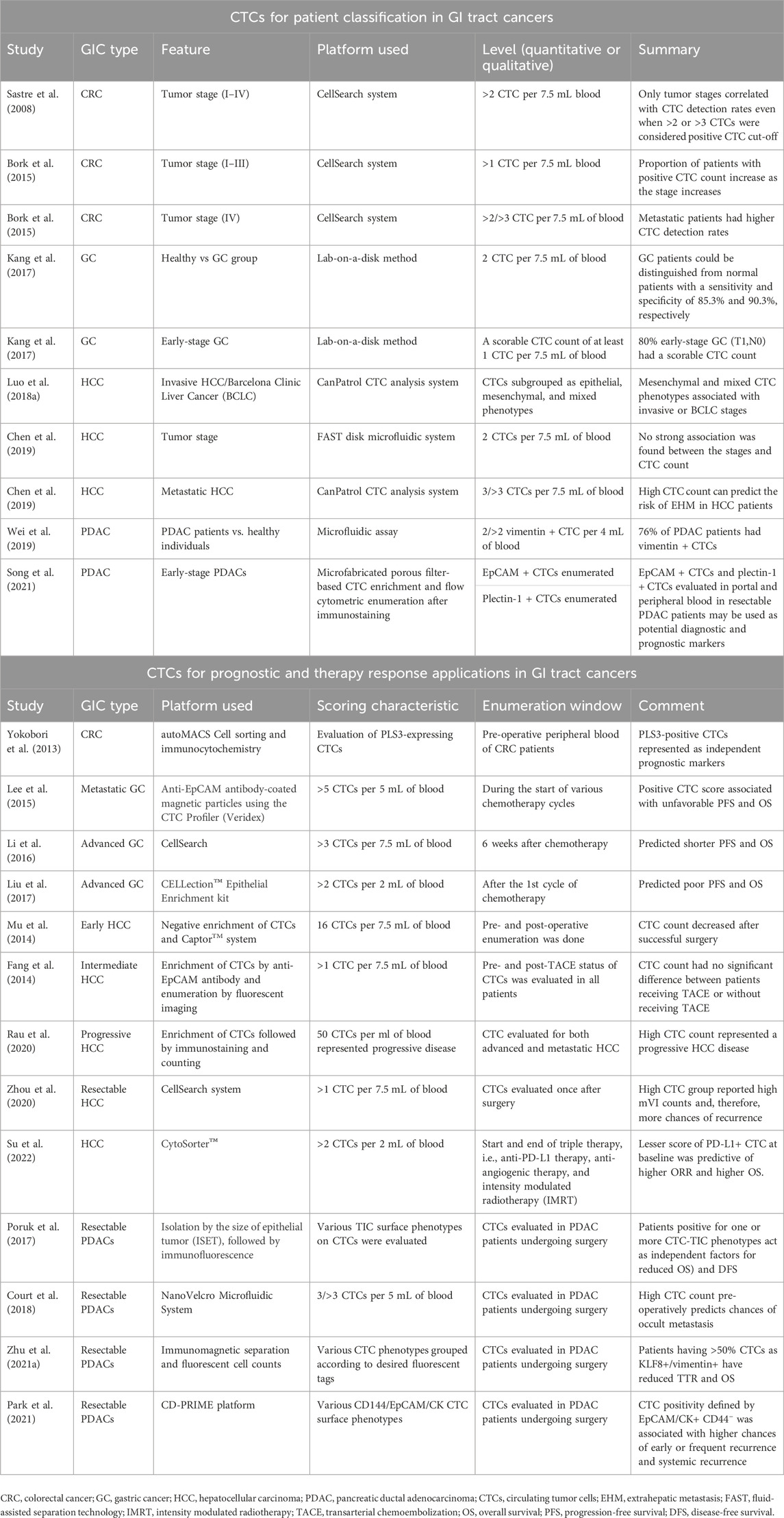
Table 1. Representative studies relating circulating tumor cell (CTC) detection for various clinical applications in gastrointestinal (GI) tract cancers.
In GC, two or more CTCs in the blood could differentiate GC patients from healthy controls, whereas a scorable CTC level could predict a high proportion of early-stage GC (T1,N0) patients (Kang et al., 2017), indicating its potential role in distinguishing patients with early-stage GC for screening and diagnosis. Interestingly, in the case of hepatocellular carcinoma (HCC), various reports indicate conflicting findings pertaining to stage-dependent associations (Chen et al., 2019; Ha et al., 2019). Few studies have reported a positive CTC count that is not associated with any clinical features, including tumor stage (Chen et al., 2019), while others have indicated that CTC levels are associated with hepatitis B, the co-existence of satellite nodules, and alanine aminotransferase levels (Ha et al., 2019). However, an interesting study that sub-grouped CTCs into epithelial/mesenchymal or mixed surface biology indicated that mesenchymal and mixed CTCs are strongly associated with a higher clinical stage and stronger invasive properties in HCC (Luo C.-L. et al., 2018). In addition, CTCs also provide valuable information for patients undergoing surgery. A post-operative CTC detection of >3 CTC per 7.5 mL of blood can distinguish patients with a risk of extrahepatic metastasis (EHM) in HCC (Sun et al., 2020). Similar to HCC, where the advanced disease stage has a mesenchymal CTC phenotype, pancreatic ductal adenocarcinoma (PDAC) also has CTCs that have the mesenchymal surface marker vimentin on their surface, which could differentiate PDAC patients from healthy patients (about 76% were positive for vimentin + CTCs) (Wei et al., 2019; Arnoletti et al., 2022). Another qualitative evaluation of plectin-1 + CTCs and EpCAMs + CTCs in the portal and peripheral blood could identify patients with early-stage resectable PDAC, which can potentially be used as an early diagnostic biomarker (Song et al., 2021). Additionally, in pancreatic cancer cases lined for surgery, pre-operative vimentin + CTC evaluation was associated with a more advanced stage of disease and metastasis (Table 1) (Wei et al., 2019). Likewise, CTC evaluation in several GI tract cancer cases can serve as a tool for patient classification to direct informed therapy prescriptions (Figure 2).
3.2 Prognosis and treatment response prediction using CTCs
CTC evaluation in cancers is strongly associated with the disease stage, along with their utility in predicting patient survival and therapy responses, which can be exploited for therapy monitoring applications and predicting outcomes in patients. In CRC patients, a study indicated that high CTC levels in the blood are significantly associated with inferior progression-free survival (PFS) and reduced overall survival (OS) (Cohen et al., 2009) (Figure 3). Moreover, a positive CTC score was closely correlated with the tumor stage in both pre- and post-operative scenarios, and interestingly, only post-operative CTC levels were positively associated with relapse-free survival (RFS) (Yang et al., 2018), indicating the clinical utility of post-operative CTC enumeration. For non-metastatic CRC (stages I–III), primary tumor features were not significantly associated with CTC detection, but a high CTC count during chemotherapy was also associated with unfavorable PFS (Wang et al., 2019b). It is already known that CTC levels are strongly associated with the TNM stage, lymphatic invasion, CEA levels, and distant metastasis (Sun et al., 2020). Several studies have, therefore, concluded that CTC scores are independent prognostic factors for predicting PFS and OS in CRC (Zhang et al., 2017; Bidard et al., 2019; Dizdar et al., 2019; Nicolazzo et al., 2019), whereas others have noted them as insignificant in some special cases, such as during pulmonary metastasectomy of metastatic CRC (Le et al., 2018). The genomic profiles of CTCs can also serve as prognostic indicators. Some genes found in CTCs play a role in the prognostic classification of CRC, including COX-2 (Cai et al., 2019), LGR-5 (Wang et al., 2018), and AKT-2, wherein positive AKT-2 expression may predict shorter median survival, PFS, and OS (Welinder et al., 2015) (Table 1). Moreover, the overexpression of plastin 3 (PLS3) on the CTC surface is an independent predictor of CRC patient prognosis, strongly associated with the DukeB and DukeC stages of CRC (Yokobori et al., 2013).
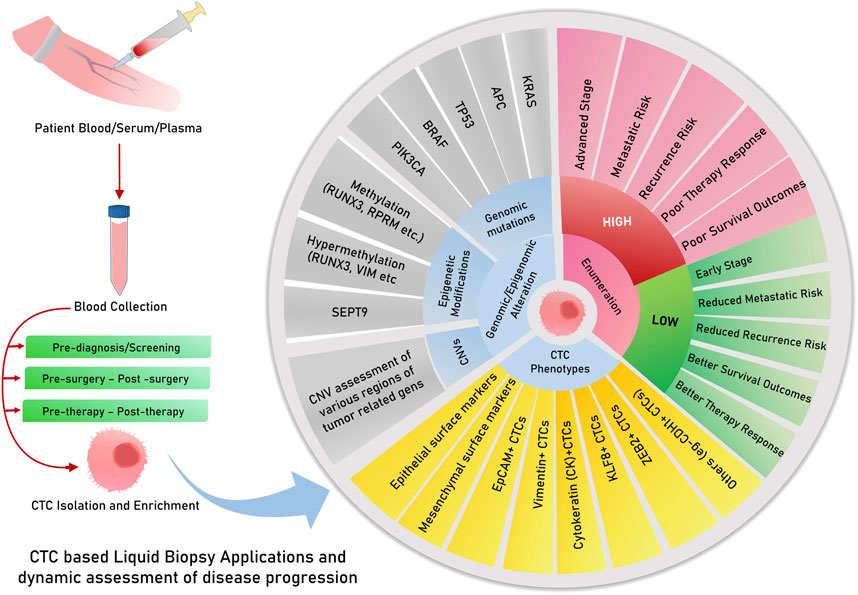
Figure 3. Circulating tumor DNA/cfDNA have multiple features and modifications, which are explored and tested for clinical decisions about risk, relapse, survival, and therapy responses. cfDNA or ctDNA is useful for dynamic monitoring, therapy responses, and risk assessment of relapse. Possibilities for the risk of assessment of CTCs for prognostic and other clinical applications.
In GC, a high CTC count has been associated with reduced overall survival rates in multiple studies and meta-analyses (Huang et al., 2015). Studies have also reported that an unfavorable or high CTC count after 6 weeks of chemotherapy predicts reduced PFS and OS in addition to the objective response rate (ORR) in advanced gastric cancers (Li et al., 2016). Another study employing the enrichment of the epithelial marker EpCAM, followed by immunostaining and enumeration, could predict a positive CTC count to be associated with poor PFS and OS, even in patients undergoing their first cycle of chemotherapy (Liu et al., 2017). However, in metastatic GCs, a positive CTC count was found to predict poor PFS and OS in patients undergoing various chemotherapy cycles (Lee et al., 2015) (Table 1; Figure 3). A recent meta-analysis on gastric cancer, however, identified the immunofluorescent detection of CTCs to produce a significant association between CTC-positive counts and overall survival compared to molecular detection methods (Li Q. et al., 2022). This highlights a possible reason for inconsistent CTC enumeration results due to a technical bias caused by the platform/methodology followed by various studies across the globe.
When considering HCC, CTC scores can predict responses to surgery and therapy. For instance, pre- and post-operative CTC detection by negative enrichment and the Captor™ system could efficiently report a reduction in the CTC count after successful hepatectomy (Mu et al., 2014). Studies have also reported that a positive CTC score is associated with lower survival and recurrence rates (Ha et al., 2019). More than 3 CTCs per 4 mL of blood are associated with shorter recurrence-free survival and worse overall survival in pancreatic cancer patients (Chen et al., 2019; Wei et al., 2019). In another study, a positive CTC count was associated with lower survival and a higher chance of relapse among HCC patients with low levels of alpha-fetoprotein and a co-occurrence of cirrhosis (Ha et al., 2019). CTC scoring can also be utilized to predict progressive disease (PD) in cases of HCC. For instance, a study reported that a higher CTC count is predictive of PD and that a lower CTC count could represent a stable disease (SD) or partial response (PR) in HCC. This study reported 50 CTCs per ml blood for PD and approximately 15 CTCs per ml of blood for SD and PR (Rau et al., 2020). Another risk factor assessed post-surgery is the evaluation of microvascular invasion, which can enhance the chances of recurrence in patients with HCC. Zhou et al. (2020) found that a positive CTC score is strongly associated with enhanced mVI counts, thereby increasing the chances of relapse/recurrence. The authors of this study are of the informed opinion that the CTC-positive group must have a surgical margin >1 cm from the tumor to minimize the chances of relapse and increase the overall survival of HCC patients (Zhou et al., 2020). Furthermore, intermediate-stage HCC patients who received transarterial chemoembolization (TACE) did not have significantly lower CTCs than patients who did not receive TACE, elucidating the lesser role of CTC enumeration in this patient subgroup (Fang et al., 2014) (Table 1).
Similar studies and meta-analyses in PDAC patients have found CTC detection to be useful in predicting lower overall survival and reduced progression-free survival (Han et al., 2014; Martini et al., 2019; Wang et al., 2020). Many studies in resectable PDAC patients have reported that pre-operative CTCs are associated with lower survival and chances of relapse (Poruk et al., 2017; Zhu B. et al., 2021; Hugenschmidt et al., 2021; Park et al., 2021). More recently, in a study, time to recurrence (TTR) was found to be reduced in patients with >50% CTCs as KLF8+/vimentin+, along with the perineural invasion status (Zhu P. et al., 2021). A positive CTC evaluation (EpCAM/CK+ and CD44−) in patients with resectable PDAC can also predict the chances of early, frequent, and systemic recurrence (Park et al., 2021). CTC phenotypes representing tumor-initiating markers comprised one or more markers, such as aldehyde dehydrogenase (ALDH), CD44, or CD133, indicating a tumor-initiating cell (TIC) phenotype, which could act as an independent factor for reduced OS and disease-free survival (DFS), as reported in a recent study (Poruk et al., 2017). Similarly, a few studies reported that zero or less than one CTC with EpCAM and Plectin-1 mesenchymal markers was associated with longer overall survival and can be used as a prognostic marker (Song et al., 2021). The milestone CLUSTER study was designed to evaluate CTC dynamics and found that pre-operative CTC levels were strongly associated with early recurrence in patients undergoing neoadjuvant therapy (NAT) or initial resection surgery. In the neoadjuvant group, undetectable CTCs were correlated with longer overall survival, reiterating the popular fact that the presence of CTCs is indicative of poor survival (Gemenetzis et al., 2018). However, pre-operative CTC levels could also be used to identify patients with a high chance of occult metastasis from others, as suggested by a study employing the NanoVelcro microfluidic system for CTC evaluation (Court et al., 2018). More recently, a new circulating stromal cell named cancer-associated macrophage-like cell (CAML) has been identified; a higher count of which is predictive of advanced stage and reduced progression-free survival, along with the identification of more aggressive forms of pancreatic cancer (PC) (Gardner et al., 2021) (Table 1).
Dynamic evaluation of CTC status in patients during the course of treatment would prove useful to clinically judge the response to therapies and decide the change of course if required. CTCs were observed to decrease in peripheral blood after patients received cryotherapy for liver metastasis, along with a reduction in other serum biomarkers such as CEA, CK18/19, and EpCAM (Shi et al., 2016) (Figure 3). Another study evaluating patients receiving anti-PD-L1 treatment reported high PD-1/PD-L1 expression in CTCs as a biomarker for screening patients for this therapy (Yue et al., 2018). For patients receiving the popular FOLFOX and bevacizumab treatment for mCRC, a decreasing CTC count and VEGFR positivity in CTC corresponded to better treatment responses, as reported in a study (Delgado-Ureña et al., 2018) (Table 1). Therefore, the above studies point to the important and actionable roles of CTCs and CTC-phenotypes in capturing tumor evolution during the course of therapy for the effective monitoring of treatment response and yielding better outcomes (Figure 3).
4 Tumor-educated platelets
Platelets are abundant anucleated cell types found circulating in body fluids like blood and lymph, originating from megakaryocytes. They play a well-known role in maintaining homeostasis and actively participating in the wound-healing process of thrombosis (van der Meijden and Heemskerk, 2019). Tumor-educated platelets are platelets that pick up tumor-associated biomolecules and vesicles while interacting with tumor cells (Nilsson et al., 2011) in the tumor microenvironment (TME). Platelets have a strong association with wound healing and inflammation, which are also frequent events occurring in the tumor progression pathway, indicating the formidable role of this cellular species in cancer prognosis. The first report of platelets being vehicles of biomolecular signals from tumors was reported when a study discovered their ability to attach to tumor RNA-containing vesicles (Nilsson et al., 2011). Tumor cells can directly initiate the development of circulating tumor-educated platelets by interacting with them or through various factors such as RNA/vesicles/proteins released from them to educate them to eventually emerge as TEPs (Plantureux et al., 2018). Many studies lay out the process of how tumor cells activate circulating platelets and initiate the formation of TEPs (D’Ambrosi et al., 2021; Xu et al., 2018). Across cancer types, studies stated the valuable role of TEPs in carrying tumor mutational signatures as RNA profiles and indicated their potential role fit to be used for pan-cancer diagnostic utilities (Best et al., 2015). Many studies in the scientific domain report mRNA profile changes in TEPs in various cancer types, such as non-small cell lung cancer (NSCLC) (Luo L. et al., 2018; Xue et al., 2018; Dong et al., 2021), breast cancer (Mendoza-Almanza et al., 2020), liver cancer (Best and Wurdinger, 2020; Waqar et al., 2021), and glioma (Campanella et al., 2020). Some studies have also investigated and found the role of medium-sized extracellular vesicles (EVs) (mEVs) exuviated by platelets activated by thrombins to carry prognostic clues (Contursi et al., 2023). TEPs and their role in the clinical utilities of CRC are still an under-researched area; however, their potential role in prognosis and treatment monitoring is not disputed. TEPs can, singularly or along with other diagnostic biomolecules such as CTCs, CAFs, or ctDNA, be used for clinical utilities such as early diagnosis, screening, treatment monitoring, and predicting prognosis in cancer patients.
TEPs have several clinical utilities in various cancer types apart from gastrointestinal cancers, including breast cancers (Best et al., 2015; Alimirzaie et al., 2019; Yao et al., 2019; Mendoza-Almanza et al., 2020), nasopharyngeal cancers (Wang et al., 2019c), prostate cancers (Tjon-Kon-Fat et al., 2018; Boerrigter et al., 2020), lung cancers (Sheng et al., 2018; Xing et al., 2019), glioblastoma (Campanella et al., 2020; Sol et al., 2020; Meng et al., 2021), and ovarian cancer (Mysona et al., 2019; El-Arabey et al., 2020). Most studies evaluating the role of TEPs have qualitatively reported the RNA profiles of TEPs, whereas the protein profiles of TEPs still remain considerably unexplored. For instance, a TEP RNA profiling study reported that CRC-associated signatures in RNA profiles could efficiently differentiate between CRCs and other non-cancerous colon-related diseases like ulcerative colitis (UC), Crohn’s disease, and non-cancerous polyps (Xu et al., 2022). Similarly, a study of TEP proteins concluded a strong association between CRC disease state and higher levels of platelet-derived growth factor (PDGF), platelet factor-4 (PF4), and vegetative epithelial growth factor (VEGF) in TEPs employing ELISA (Peterson et al., 2012). In addition to proteins, in CRC, the presence of a higher TIMP1 mRNA level was also reported, which could efficiently differentiate the diseased group from the healthy group, indicating its role in diagnosis (Yang L. et al., 2019). Given the biological role of PDGF and TIMP1 in angiogenesis and metastasis, a more inclusive study might indicate their role in differentiating metastatic/advanced CRC as opposed to early-stage CRC. Non-coding RNAs associated with TEPs have also been understood to have utilities in the clinical management of CRC. A study could identify high expression of four long non-coding RNAs (lncRNAs), namely, LNCAROD, TSPOAP-AS1, LINC00534, and another SNHG20, in patient serum and tumor-educated platelets isolated from CRC patients. In this, two lncRNAs, namely, LNCAROD and TSPOAP-AS1, were found to have strong associations with the primary tumor location and stage of the disease (Ye et al., 2022). Very recently, another study discovered that significant levels of the lncRNA named colon cancer-associated transcript-1 (CCAT1), usually associated with tumor TEPs, were highly expressed in the circulation of CRC patients (Tabaeian et al., 2024). Futuristic studies investigating mEVs, which were found to have distinct sizes and proteomic profiles compared to healthy individuals (Contursi et al., 2023), indicating their pro-metastatic roles, could be explored further along with other biomarkers to enhance their diagnostic and prognostic efficacies.
In pre-clinical models, pancreatic cancer cells are reported to stimulate the aggregation of platelets, a phenomenon known as tumor cell-induced platelet (TCIP) aggregation, which is followed by thrombosis; this can enhance disease progression by promoting intra-tumor communications (Mai and Inkielewicz-Stepniak, 2021). Moreover, TEPs and their role in defining the clinical characteristics of pancreatic cancers have not been well researched. However, many studies have underlined the clinical utilities of various platelet features in the range of PC characteristics like a higher mean platelet volume (Yin et al., 2018), predictive of liver-metastatic PC, and advanced stages (stages III–IV), while a decreased MPV was associated with poor prognosis and early-stage patient groups (Yagyu et al., 2021). TEPs and their RNA profiles could differentiate between KRAS wildtype and KRAS-deficient pancreatic tumors (Best et al., 2015). Proteome profiles of platelets could also differentiate between early stages (stages I–II) of the head of pancreas cancer and those of age and sex-matched healthy subjects (Sabrkhany et al., 2017). A bioinformatics investigation also revealed a possible role of TEP-associated mRNA RSL24D1 in being associated with an early pancreatic tumor disease stage compared to their healthy counterparts. New research deliberations are required in this area to bring stable biomarkers fit to be used in clinical settings and applications. As pancreatic cancer patients are at an increased risk of venous thrombosis, another possible research interest would be to investigate the association between platelets and thromboembolism-associated factors in patient prognosis as an earlier study on a pan-cancer cohort demonstrated increased levels of cancer-associated tumor thromboembolism (VTE)-associated factors such as ADAMTS-13 and VWF in patients having worse survival rates (Obermeier et al., 2019).
Platelets play an important physiological role in the liver, and therefore, in hepatic diseases such as hepatocellular carcinomas, their dysregulation is expectedly observed (Lambert, 2016). In HCC, high platelet counts were long associated with shorter survival (Schrecker et al., 2022) and may also indicate chances of early recurrence if considered along with CTCs (Lu et al., 2023). MicroRNAs isolated from TEPs also carry many cues of HCC-specific upregulations, such as enriched RhoA, SPINK1, and CTNNB1, along with a considerable upregulation of SERPIND1, IFITM3, and CD41+ levels, to demarcate HCC and cirrhosis patients (Waqar et al., 2021). Another study reported the high expression of TGF-ß, NF-KB, and VEGF in mRNA isolated from TEPs in HCC patients, indicating an advanced stage, whereas a reduced level of AKT and PIK3 could represent early-stage HCC (Asghar et al., 2020). Studies investigating microRNAs in TEPs isolated from HCC patients when computationally analyzed could identify a differentially expressed microRNA pair, i.e., miR-1293 and miR-495-3p in HCC patients, playing pathological and diagnostic roles (Zhu B. et al., 2021). These diverse utilities of TEPs underline more such investigations in larger cohorts for designing more precise prognostic models.
5 Circulating cell-free DNA
Circulating cfDNAs are DNA fragments in blood/plasma circulation (Mandel and Metais, 1948), mostly derived from healthy leukocytes and stromal cells, and found to be enriched in cancer patients (Leon et al., 1977). Their applicability in tumor diagnosis and prognosis was not recognized until studies indicated that a considerable proportion of cfDNA originates from tumors and can have tumor-associated footprints. In 1994, a remarkable study identified tumor-associated KRAS and NRAS gene mutations in cfDNA, which sparked further curiosity to unravel the diagnostic and prognostic purposes of cfDNA in various tumors (Caldas et al., 1994). In cancer patient serum and plasma, cfDNA is found at higher levels, mainly due to cellular apoptosis or necrosis of stromal and immune cells in tumors (Stejskal et al., 2023). Regarding patient diagnosis and classification, earlier studies indicated that cfDNA levels in CRC patients were enriched both in plasma and stool, paving the way for its potential use in less-invasive CRC diagnosis (Sidransky et al., 1992; Harlé, 2020). However, cfDNA elevation is also indicative of other diseased states relating to inflammatory and other physical stress conditions (Hummel et al., 2018). Therefore, its use, along with other minimally invasive markers such as tumor-specific gene mutations and their methylation status, has yielded greater prognostic and diagnostic specificity (Table 2).
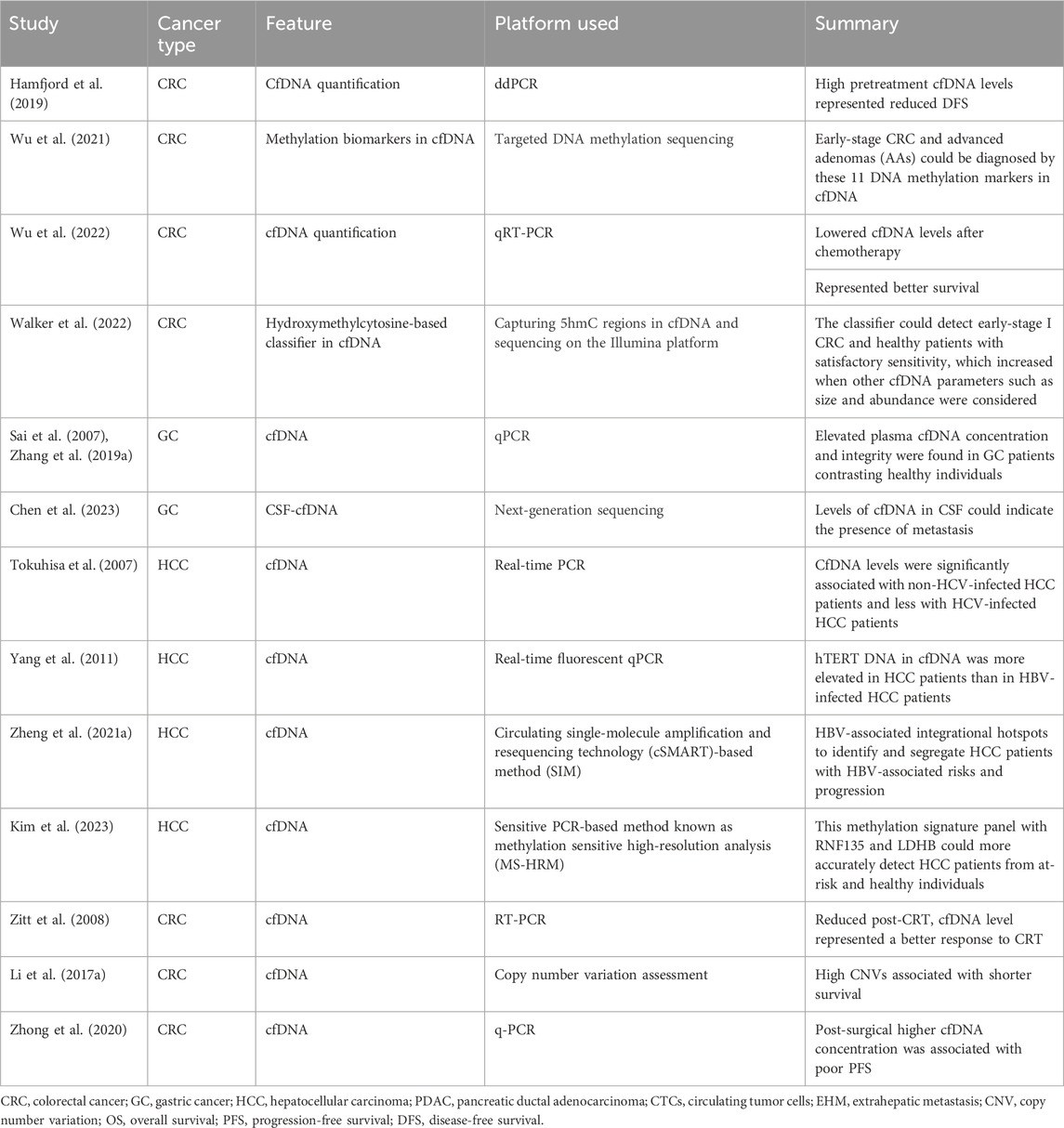
Table 2. Representative studies relating cfDNA for clinical applications in gastrointestinal tract cancers.
Tumor-specific gene mutations arise from necrotic and apoptotic tumor cells, which release their fragmented DNA in circulation, giving rise to a separate subgroup within cfDNA known as ctDNA (Leung et al., 2016). This ctDNA is a vehicle for tumor-specific gene mutations, like KRAS (Sidransky et al., 1992), BRAF (Fu et al., 2021), TP53, APC, or PIK3CA (Jauhri et al., 2017). Therefore, the detection of these mutations in cfDNA would indicate disease states, chances of recurrence, or responses to therapies in a more focused manner. However, recent investigations showed that ctDNA detection rates are dependent on the quality and quantity of input cfDNA in DNA-sequencing platforms (Bettegowda et al., 2014). An interesting study indicated that 45% of CRC-specific mutations (KRAS) in tumor tissues could also be found in their plasma cfDNA in patients and not in healthy groups (Wang et al., 2004). The concordance of CRC mutations in both tumor tissue and plasma cfDNA was also studied, which underlined the concordance of 96% KRAS and 100% BRAF mutations (Thierry et al., 2014). Similarly, many studies reported higher levels of cfDNA in plasma, which was strongly correlated with the presence of CRC in healthy individuals (Pu et al., 2021; Wu et al., 2022); on the other hand, in another study, cfDNA was found to be enriched more in colon tumors (500 ng/mL) than in rectal tumors, which had plasma levels of approximately 250 ng/mL (Frattini et al., 2008) (Table 3).
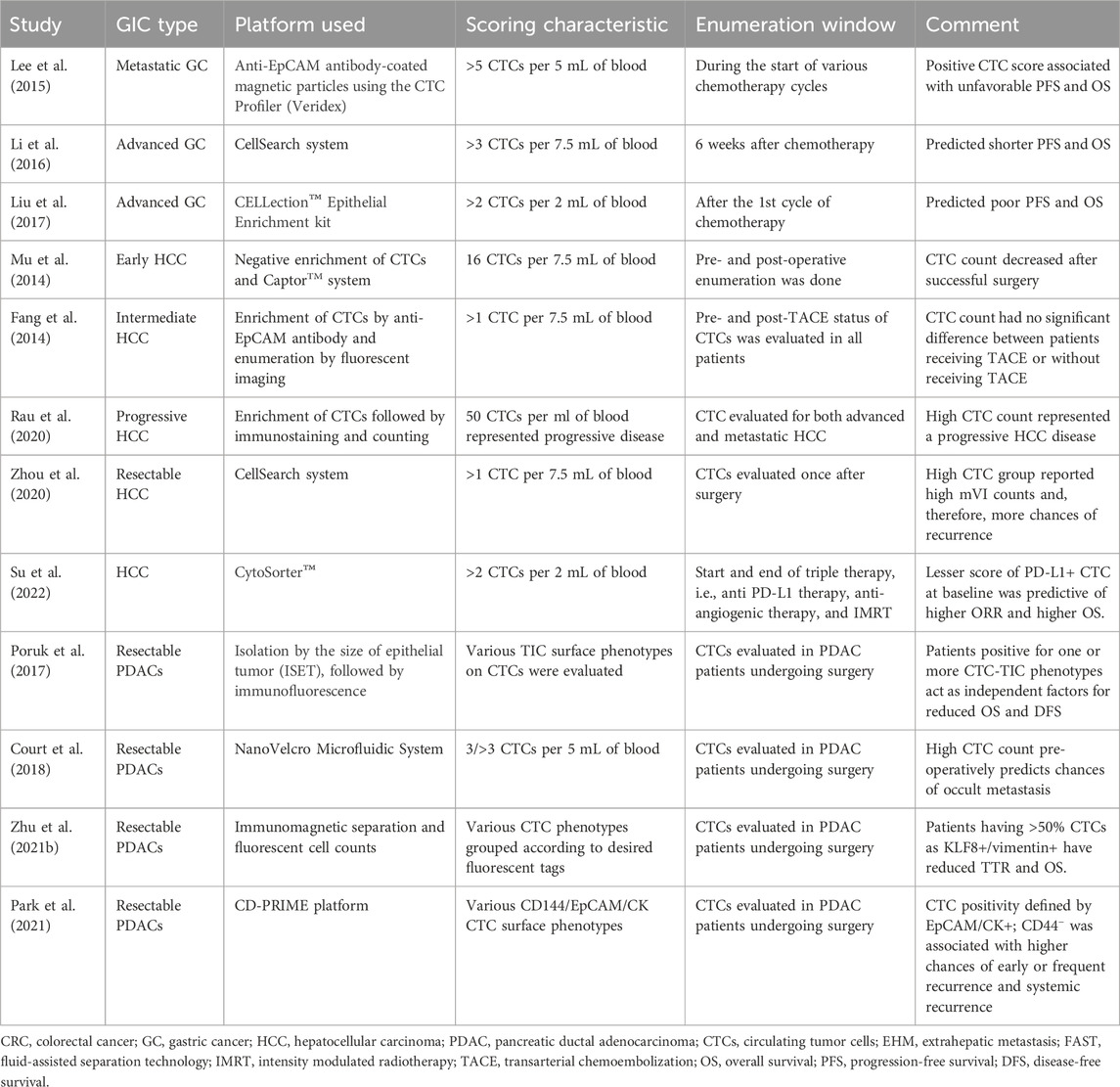
Table 3. Studies relating CTC detection and various prognostic parameters in gastrointestinal cancers.
CTC-associated parameters such as cfDNA abundance and integrity (Pu et al., 2021; Wu et al., 2022) are also stage-dependent and could be used for monitoring disease progression. However, studies have failed to show a compounded cfDNA trend across studies; instead, cfDNA abundance and growth rates at endpoints of various clinical crossroads, such as post-therapy and pre-therapy, have found evidential backing in studies. Moreover, in a recent large-scale study of 16,347 patients in stages I–III of CRC, post-operative cfDNA levels did not affect ctDNA positivity, which clearly demonstrated the better usefulness of ctDNA in driving clinical decisions (Cohen et al., 2023).
Currently, DNA methylation markers in cfDNA are being researched for more accurate patient classification exercises. In this thread, a study found that an 11-DNA methylation marker model could effectively distinguish between early-stage CRC and advanced adenomas and can help guide early diagnosis (Wu et al., 2021). Another recent finding is the role of 5-hydroxyl cytosine modifications in cfDNA. In a study, 5-hydroxymethylcytosine regions were captured and sequenced to develop a 5hmC profile of CRC and GC patients. This classifier could diagnose early-stage I and late-stage IV diseases in healthy individuals (Walker et al., 2022). The sensitivity of this method increased as it included other cfDNA features, such as the size of fragments and abundance, along with these 5hmC profiles (Walker et al., 2022), indicating a novel role of 5hmC regions of cfDNA in early CRC and GC diagnosis.
In hepatocellular carcinoma patients, cfDNA levels are approximately 20 times more enriched than those in healthy individuals (Iizuka et al., 2006; Tokuhisa et al., 2007; Huang et al., 2012). Patients undergoing hepatectomy project a longer survival rate and lesser recurrence risk if cfDNA levels reduce after surgery (Tokuhisa et al., 2007). HCC patient subgroups include those with liver cirrhosis, chronic hepatitis, or HBV-infected groups. cfDNA can be used to quantitatively recognize these subtypes. For instance, hTERT DNA in cfDNA was found to be more enriched in non-HBV-infected HCC patients than in HBV-infected HCC patients (Yang et al., 2011) and HCV carrier HCC patients (Tokuhisa et al., 2007). HBV infections damage the host DNA and are responsible for HCC progression. A study identified HBV integration hotspots in the cfDNA of HCC patients (Zheng B. et al., 2021) to be potentially used for surveillance and HBV-related risk stratification in HCC patients. The methylation of cfDNA is also predictive of HCC, such as the methylation of the cfDNA-based assay with a sensitivity of 78.5% and a specificity of 89% that could distinguish the diseased group from the healthy group (Wang C. et al., 2021). Another cfDNA-based methylation signature panel with ring finger protein 135 (RNF135) along with lactate dehydrogenase B (LDHB) studied healthy individuals, individuals at risk, and HCC patients to positively diagnose 57% of HCC patients better than the A-fetoprotein analysis (Kim et al., 2023). Recent interest lies in developing a multi-omics assay that integrates the mutational and epigenomic status in cfDNA to predict HCC in a more precise method (Wang P. et al., 2022) (Table 3).
In pancreatic cancers, cfDNA features have several associations with the disease state and stage. Apart from elevated levels of cfDNA in PDAC patients (Bronkhorst et al., 2019), cfDNA fragment lengths are also found to be shortened in individuals with such diseases (Ranucci, 2019). For the precise detection of stages, more specific genomic signatures in cfDNA (ctDNA) are required. The most frequently mutated genes in PDAC are KRAS, SMAD4, CDKN2A, and TP53, among various other genomic markers that could be assessed for differentiating the diagnosis of early and metastatic PDAC cases. A study reported that genetic alterations in cfDNA could be more specifically found in metastatic PDAC in contrast to fewer such alterations in local PDAC (Bettegowda et al., 2014).
Regarding prognosis and therapy response, as a trend, various studies have reported a decrease in cfDNA levels after chemotherapy (Wu et al., 2022). Patients who had higher cfDNA levels before first-line chemotherapy (oxaliplatin-based) showed reduced survival in terms of DFS in metastatic CRC (Hamfjord et al., 2019). On the other hand, patients who underwent surgery and had enhanced levels of cfDNA experienced lower PFS (Zhong et al., 2020). Numerous studies also record that in patients receiving various therapies, those who registered a reduction in cfDNA levels compared to pre-therapy cfDNA levels represented a better survival and therapy response group (Leon et al., 1977; Sozzi et al., 2001; Sozzi et al., 2003). Patients who received chemoradiotherapy (CRT) were reported to either have increased cfDNA levels or decreased cfDNA levels post-CRT compared to their initial pre-CRT cfDNA levels. Those patients who recorded reduced cfDNA levels after CRT were found to better respond than those with consistently high cfDNA levels (Zitt et al., 2008). Copy number variations (CNVs) are yet another feature of cfDNA that could indicate tumor progression and provide survival predictions. A positive test for enhanced CNVs in several chromosomal regions and tumor-associated genes found in cfDNA is associated with shorter survival (Li J. et al., 2017). However, more large-scale studies are needed to make strong predictions for the early diagnosis and definitive prognosis of CRC.
In gastrointestinal cancers like CRC, plasma cfDNA is found to be elevated in multiple studies (Sai et al., 2007; Zhang H. et al., 2019), both in terms of its concentration and integrity. The majority of individuals with gastroesophageal cancers (GECs) present with an advanced stage at diagnosis or collapse due to disease recurrence even after the main tumor is surgically removed, underlining the fact that GECs have high death rates. Such disease progressions can be predicted using tumor-specific genetic alterations in cfDNA isolated from plasma (Rumiato et al., 2017; Koldby et al., 2019). As already stated, in CRC, the methylation status of specific tumor-associated markers has better prognostic efficacy, which has also been noted in gastric cancers. A recent study identified the elevated status of methylated genetic markers in RUNX3, P16, RASSF1A, and RPRM in gastric cancers, unlike in healthy individuals. The study also underlines the greater role of RNX3 and RPRM in detecting early-stage (I–II) GC (Saliminejad et al., 2020).
In HCC, cfDNA assessment during the administration of systemic therapies and follow-up can capture tumor heterogeneity and actionable mutations from tumors, direct therapy, and offer progress monitoring tools (Coto-Llerena et al., 2022). Many therapy-responsive gene mutations also exist, such as CTNB1 S33C (Lin C. et al., 2021), ARIDIA R727, AXIN1 (Higuera et al., 2022), NF1 (Lyu et al., 2022), and NRAS, which can be detected well in cfDNA/ctDNA and can, therefore, direct therapy decisions in HCC patients. Higher cfDNA levels, as expected, supported reduced survival rates (Iizuka et al., 2006) and a higher risk of recurrence with elevated chances of metastasis (Huang et al., 2012).
6 Circulating tumor DNA
As discussed in the above section, ctDNA is a new and promising biomarker for appropriate, directed treatment in precision oncology using liquid biopsy. Tumor DNA, as ctDNA, can be used to analyze various tumor-related features such as prognosis, therapy responses, or heterogeneous resistance in individual patients, which are typically overlooked by tumor biopsies. The dynamics of repeated tumor biopsies have reduced patient compliance (Li W. et al., 2022) and may not be necessary after the discovery of ctDNA in many cancer types. Equivalent to next-generation sequencing (NGS) whole-genome sequencing, cfDNA and ctDNA are the most invaluable and highlighted blood biomarkers in recent years due to their predictive and prognostic importance. The foremost challenge to detecting ctDNA in the plasma is the presence of low-quantity DNA with a half-life of approximately 2 h. In contrast, ctDNA is present in higher amounts in cancer patients than in healthy individuals. The proportion of ctDNA in total plasma cfDNA increases for advanced stages (10%) while remaining very low in early stages; the exosomal prion proteins were found to be overexpressed in CRC, which could be cancer (0.1%). Less invasive procedures for testing ctDNA may help in the screening/diagnosis of early tumors, but its strong roles have been more extensively researched for the disease monitoring and molecular assessment of patient recovery, responses, and chances of relapse during and after the respective treatments. Although several studies showcase that ctDNA detection is more helpful in the advanced stages of tumors in detecting the heterogeneity of disease, a large-scale longitudinal study reported that using methylation markers in ctDNA, some major gastrointestinal tumors can be diagnosed as early as 4 years before they are detected by current standard diagnostic procedures (Chen et al., 2020a).
6.1 ctDNA and patient stratification
Circulating tumor DNA can play a role in early screening. Few studies claim that ctDNA detection can help in the pre-diagnosis of cancer even before the symptoms appear. In a recent study, ctDNA methylation was detected in the plasma of 605 individuals with no symptoms, and among them, 191 patients within 4 years were detected with various GI cancers (colorectal, esophageal, stomach, and liver cancer) (Chen et al., 2020b). However, more such studies are needed for conclusive evidence for its use in screening and early diagnosis. In addition to this, ctDNA features can harbor clues for patient stratification for precision therapy decisions. Circulating tumor DNA with a CRC-specific mutation can also be an important parameter for diagnosing clinically relevant tumor features. In metastatic CRC, ctDNA with KRAS mutations could detect diseased groups with 99.2% specificity and 87.2% sensitivity (Bettegowda et al., 2014), whereas its sensitivity to detect stage I CRC was not satisfactory. The inclusion of a more diverse gene panel of 38 genes indicated enhanced diagnostic abilities in gastric cancer, as reported by a study (Varkalaite et al., 2021). The methylation status of ctDNA could also provide discriminatory capability to distinguish between early disease and healthy individuals. A study concluded that the presence of six methylation markers in ctDNA was enriched in about 78% of stage I–III CRC patients (Mo et al., 2023) and that the methylation status of the SEPT9 gene in plasma is strongly capable of distinguishing GC patients from healthy individuals least invasively (Bergheim et al., 2018; Zhao et al., 2022) (Table 3).
In PC, a four-gene (PXDN, ADAMTS1, LRFN5, and BNC1) methylation status assessment in ctDNA could provide 90% specificity in the early detection of PCs (Ying et al., 2021). Later, an interesting study designed a sequencing data analysis tool/algorithm called methylated CpG tandem amplification and sequencing (MCTA-Seq), utilizing 153 gene methylation assessments in ctDNA, which could diagnose GCs in various stages and could also discriminate between chromosomal instability methylator phenotype (CIMP) and non-CIMP gastric cancers. This algorithm and gene panel could also differentiate between early GC from CRC or HCC samples (Ren et al., 2022). This method has been applied to various other gastrointestinal cancer types for its early diagnostic utilities in respective types such as CRC (Li et al., 2019) and HCC (Wen et al., 2015).
In gastric cancer, a separate sub-type, Epstein–Barr virus (EBV)-GC, is identified by the presence of EBV infection. This subtype can be diagnosed by qPCR methods, which detect EBV sequences in tissue DNA. However, this subtype can also be efficiently diagnosed using cfDNA, with a sensitivity of 71% and a specificity of 94%. In addition to detecting the EBV subtype, this method can also help in assessing tumor progression and therapy responses in EBV-positive patients (Shoda et al., 2017). In many cases, it has been observed that cfDNA/ctDNA integrity is reduced in various cancer types as they are reported to have many regions of ultrashort or jagged DNA. Quite recently, technologies and tools were innovated that could efficiently capture even these ultrashort or jagged DNA sequences in ctDNA. A sequencing tool called broad-range cell-free DNA-Seq (BRcfDNA-Seq) can capture these ultrashort cfDNA fragments isolated from saliva and could discriminate between gastric cancer patients and healthy individuals (Swarup et al., 2023). Few rare GC cases metastasize in the leptomeningeal region, giving rise to gastric cancer with a leptomeningeal metastasis (GCLM) subtype. Quantifying post-therapy cfDNA in the CSF of such cases provides various prognostic clues, such as this study (Chen et al., 2023), which reported that reduced cfDNA levels in the CSF indicated better PFS in GCLM patients. In such cases, identifying levels and genomic alterations in CSF ctDNA could hold promising potential to provide prognostic decisions and clinical assessment. Many ongoing clinical trials are also identifying and mapping methylomes in GC patients for potential use in the early diagnosis of GC (Han et al., 2023).
6.2 ctDNA for prognosis and therapy response
ctDNA assessment before and after treatments can also be used to predict therapy responses. A study found a difference in the ctDNA level in mCRC patients before and after the treatment with FOLFIRI, where patients were found positive for increased ctDNA levels after the first cycle of chemotherapy and showed shorter PFS and poor survival (Lyskjær et al., 2019). Studies have shown the importance of ctDNA detection in the treatment with standard chemotherapy drugs. Hence, it is suggested that ctDNA detection in the first week of FOLFIRI treatment in colorectal cancer patients could direct the progression of diseases, survival outcomes, and the indication for treatment failure in patients. KRAS and NRAS are commonly mutated genes in CRC and are also an indicator for the response to anti-EGFR-based targeted therapy using monoclonal antibodies like panitumumab and cetuximab. A study investigated the role of ctDNA in the early prediction of responses to these anti-EGFR therapies (Vidal et al., 2017). In addition to this, the presence of CRC-specific mutations such as KRAS, APC, and p53 in ctDNA is associated with a higher chance of metastasis/recurrence in patients not harboring these mutations (Wang et al., 2004).
However, another recent study also underlined the relevance of scoring ctDNA positivity in cfDNA using a wide range of CRC-specific gene panels that can be used to predict recurrence risk in post-operative stage II/III patients and can guide the administration of adjuvant radiotherapy (ART). Here, the study reported post-operative ctDNA positivity with an enhanced risk of recurrence (Chen et al., 2021) and can help in the selection of high-risk patients for ARTs. A ctDNA methylation panel of 6 markers revealed 17 times more chances of relapse in ctDNA-positive patients when evaluated after 1 month of surgery in stage I–III CRC (Mo et al., 2023). The same study followed patients who received adjuvant chemotherapy and found that ctDNA positivity led to shorter recurrence-free survival (Mo et al., 2023), highlighting that continuous ctDNA monitoring can help in accessing disease progression and therapy response. In patients prescribed for ACT, post-operative ctDNA positivity is associated with a risk of relapse, compared to patients responding as negative for ctDNA during ACT cycles (Henriksen et al., 2022). The serial assessment of ctDNA followed through post-operative and ACT cycles indicated better chances of survival and a reduced risk of relapse in the reduced ctDNA growth rate over time (Henriksen et al., 2022). On the completion of definitive therapy (surgery or adjuvant therapy) in stage I–IV CRC patients, the assessment of ctDNA for minimal residual disease (MRD) helped in predicting a 100% recurrence in the ctDNA-positive group versus 24% in those without any detectable ctDNA. This method of ctDNA assessment over 1-year follow-up after definitive therapy represented 55% sensitivity, which was enriched to 69% when genomic alteration and epigenomic markers were considered (Parikh et al., 2021). The above study also showcases the importance of epigenomic markers of ctDNA having more sensitivity for recurrence prediction than plasma CEA, reiterating the importance of consistent longitudinal surveillance using an inclusive biomarker panel of ctDNA, epigenomic, and genomic mutational markers (Figure 4).
In another milestone innovation, a blood-based assay, ColonAiQ, was developed after narrowing down 108 methylation markers to only 6 strong predictive markers in ctDNA using a specialized algorithm and designing these 6 biomarkers as an assay. This assay could predict relapse in 85% of cases that had a positive ctDNA methylation status after definitive therapies (surgery or adjuvant therapies), which persisted throughout follow-up (Cai et al., 2021). In the case of gastric cancer, a 38-gene panel to detect somatic mutations in ctDNA was found to predict an increased risk of recurrence and poor survival in patients found positive for these mutations (Varkalaite et al., 2021). More recently, various ctDNA-related features, such as blood tumor mutational burden (bTMB) and CNVs, have been investigated for clinical utilities. In a similar study with GC patients receiving nivolumab monotherapy, a higher blood tumor mutational burden (>6 mt/mb) was associated with better overall survival and longer PFS, whereas a reduced bTMB was found to confer a better disease control rate (DCR). However, the study also noted that the best survival among the cohort was registered by those with higher TMB and a negative CNV in ctDNA assessments (Inagaki et al., 2023).
In an ongoing study, the identification of novel genomic alterations in cfDNA could hold actionable therapeutic interest, such as ERBB2, FGFR2, and TP53 in 4.9%, 6.2%, and 38.3%, respectively. The identification of these mutations could distinguish GC cases from others (Kim et al., 2024). In PDAC patients, the Microsatellite Instability (MSI)-high status is usually assessed for therapy using immune checkpoint inhibitor drugs like pembrolizumab, nivolumab, or those administered with ipilimumab. The use of liquid biopsy to assess this MSI-H detection in cfDNA expectedly predicted better outcomes in immune checkpoint inhibitors as with conventional testing using tumor tissues (Chakrabarti et al., 2022). In resectable PDAC cases, the assessment of KRAS mutations greatly affects treatment outcomes. A multiplex KRAS detection tool based on cfDNA could find a strong association between the mutational concentration of KRAS and its fractional abundance to effectively determine progression-free survival (Kim et al., 2018). Detectable ctDNA is associated with shorter progression-free survival, whereas KRAS mutant allele fractions detected in ctDNA and exosomal DNA were found to be strong predictors of overall survival and PFS (Bernard et al., 2019). In unresectable PDACs that are registered for first-line chemotherapy, the positive assessment of KRAS mutations in ctDNA had unfavorable responses to chemotherapy and, thus, could help in the early detection of patients not expected to gain favorable treatment outcomes (Watanabe et al., 2023). However, it is imperative to continue developing more sensitive methods that can improve the identification of ctDNA originating from tumors and also increase its prognostic prediction accuracy through the inclusion of epigenetic, genomic, and other blood-based biomarkers (Cao et al., 2020).
7 Exosomes and exosome-derived biomolecules
Exosomes are released by all cells of the body. They are extracellular vesicles of approximately 149 nm in size, which represents the phenotypic characteristics of the cells from where they were generated; hence, they are prominently heterogeneous (Kalluri and LeBleu, 2020). Like other sub-cellular components, exosomes are also built from the lipid bilayer and also possess nucleic acids such as DNA, RNA, miRNAs, lncRNAs, tRNAs, snRNAs, and circRNAs, which can act as useful biomarkers. An average healthy human blood sample contains ∼1,900 trillion exosomes, whereas patients with cancer have ∼3,800 trillion exosomes (Doyle and Wang, 2019). The reason for the increase in exosome diversity is not well known; however, it is assumed that due to reformed cellular physiology, exosome populations increase in density and diversity (Zhang X. et al., 2019; Gurung et al., 2021; Dilsiz, 2022). Exosomes are considered more promising biomarkers and targets for therapy as they are found in greater numbers and remain stable in circulation. Exosomes have a dual nature in tumor progression and were shown to possess antitumor functions to prevent disease progression (Zhang Y. et al., 2019; Doyle and Wang, 2019). Exosomes can be detected in many biological secretions, like blood, urine, saliva, tears, breast milk, amniotic fluid, and cerebrospinal fluid. The frequent identification of exosomes in secretion makes it efficient for the diagnosis of malignancies. It is also majorly found in pancreatic, ovarian, and breast cancer patients (Zhang H. et al., 2019; Osaki and Okada, 2019; Paskeh et al., 2022), among others.
7.1 Exosome miRNAs for the clinical management of GI cancers
miRNAs are mainly short-length RNA species with strand lengths of approximately 19–21 nucleotides, which are found to regulate tumor pathogenesis and progression as tumor suppressors or initiators through several mechanisms (O’Brien et al., 2018). miRNAs play a major role in cancer progression by interacting with tumor-to-stromal interactions, immune invasion, angiogenesis, and the tumor microenvironment (Peng and Croce, 2016; O’Brien et al., 2018). These miRNAs have multiple clinical utilities, from being used as a tool for molecular diagnosis to a method for the prognosis assessment of several cancers (Galvão-Lima et al., 2021; Mondal et al., 2023). These miRNAs can become associated with exosomes as miRNAs shredded from tissue damage or programmed cell death can enter the bloodstream via micro-vesicles and exosomes by binding to HDL, AGO2, and LDL proteins (Li Z. et al., 2022). Exosomal miRNAs play a crucial role in all three aspects of identifying potential markers for diagnosis, prognosis, and predicting OS and DFS and the therapy for chemosensitive or resistant tumors (Cao et al., 2022). Certain miRNAs have high expression in the serum exosomes of CRC patients, such as miR-21, miR-1229, miR-150, let-7a, miR-223, miR-23a, and miR-1246. These findings suggest the origin of tumors in colonic tissue and can help in the differential diagnosis of CRC (Ogata-Kawata et al., 2014). Exosome miRNAs were also reported to have improved the diagnosis of early-stage CRC. For instance, miR-125a-3p, when combined with the CEA marker, suggests early-stage CRC with an AUC curve of 0.855 (Wang et al., 2017). CRC stages I–II were reported to have elevated levels of exo-miR-1246, especially a noted expression in stage II, underlining its early-stage association compared to healthy individuals (Ogata-Kawata et al., 2014; Wang et al., 2017). An elevated level of miR-21 is reported to persist in colonic adenoma throughout advanced CRC stages, indicating its diagnostic importance (Uratani et al., 2016). However, the lone use of a single miRNA would be less specific for diagnosing any cancer type as miRNAs are also elevated in other malignancies. Therefore, the use of a panel of exosome-associated miRNAs has yielded better diagnostic and predictive potential (Uratani et al., 2016).
A tested miRNA panel consisting of miR-21 and miR-1246 was combined with a few other miRNAs (miR-23a, miR-1229, miR-150, miR-223, and let-7a) for increasing diagnostic efficacy for various CRC stages (Uratani et al., 2016). Exosomal levels of this miRNA panel can help in cancer surveillance as their concentration is reported to reduce after resectional surgery (Ogata-Kawata et al., 2014). Another study demonstrated that the enrichment of miRNA panels in exosomes is better than that of circulating miRNAs. Particular exosomal miRNA panels consisting of exo-miR-23, exo-miR-16, and exo-let-7 were strongly enhanced in the CRC patient group compared to healthy individuals (Dohmen et al., 2022). Some miRNAs can have clear expressional differences in various stages of disease, which can be exploited for stage-specific diagnosis. Another miRNA, miR-320b, was found to be enhanced in stage IV CRC and not in stages I–II CRC. Similarly, miR-320d is also found to be highly expressed in metastatic CRC (Tang et al., 2019). In gastric cancer as well, exo-miR-590-5p had elevated expression in the lower stages (stage I/II) and reduced expression in the advanced stages (stage IV) and metastatic CRC (Zheng G.-D. et al., 2021).
Some exosome miRNAs can also predict survival chances, recurrence, and therapy response. Among these, a reduced level of miR-422-3p is related to higher chances of recurrence in stage I/II CRC individuals (Liu C. et al., 2016). However, its role in determining the FOLFOX adjuvant therapy response in progressive CRC stages remains elusive. In gastric cancer, elevated levels of exosomal miRNA miR-590-5p were found to be responsible for decreased overall survival (Zheng B. et al., 2021). Yet another exosomal miRNA exo-miR-92a derived from GC patients was found to play a multifaceted role where its high levels could signify shorter survival, metastatic state, and poor survival outcomes (Yang et al., 2020). A meta-analysis of multiple such studies on miR-92a underlines its important role in prognosis (Guo et al., 2021). Poor survival and recurrence risk (peritoneal recurrence-free survival) increase with high expression of exosome-encapsulated exo-miR-21 in GC cases, as reported in a study (Soeda et al., 2019). Likewise, a range of exo-miRNAs are found to have prognostic and therapeutic importance. An elevated exo-miR-215-5p level is associated with poor DFS and has prognostic utilities in HCC. For instance, exo-miR-10b-5p can be used as a biomarker to identify the early stages of this disease (Cho et al., 2020). miR-720 was found to be consistently elevated in HCC patients compared to patients with other liver diseases. Moreover, exo-miRNAs are also closely associated with small HCCs and intrahepatic tumor stage progression (Jang et al., 2022). Tumor-suppressing exo-miR-199a-3p is found to reverse and control resistance to chemotherapeutic drugs like cisplatin when externally administered (Lou et al., 2020; Zhang et al., 2020). Similarly, many oncogenic miRNAs, such as miR-155 and miR-21, in plasma have been associated with poor survival and progression (Ratnasari et al., 2022), but the roles of their exosomal variants in HCC remain to be explored (Zhou et al., 2018). In the diagnosis and prognosis of pancreatic cancer, exo-miRNAs might be employed, such as exo-miR-1226, which is usually downregulated in PDAC cases compared to benign lesions of the pancreas. Its elevated levels in PDAC patients may signify higher invasiveness and a higher risk of metastasis and recurrence of this disease (Wang F.-W. et al., 2021). On the other hand, exo-miRNAs enriched in portal blood rather than peripheral blood, such as exo-miR-21, exo-miR-451a, and exo-miR-4525, can be employed for identifying PDAC cases undergoing resectable surgery. These exo-miRNAs can be utilized for assessing cases with a high risk of recurrence and poor survival (Kawamura et al., 2019). When considering an efficient exo-miRNA panel, a study identified an exosome miRNA signature having six exo-miRNAs (miR-1273f, miR-1229-3p, miR-432-5p, miR-195-5p, miR-133a-3p, and miR-130b-5p), a higher risk score of which could predict higher chances of recurrence and poor survival outcomes in PDAC cases (Nishiwada et al., 2022).
7.2 Exosome lncRNAs
Long non-coding RNAs (lncRNAs) are non-protein-encoding RNA transcripts, usually longer than 200 nucleotides and are sometimes termed “mRNA-like” because of their plausibility to acquire RNA-like modifications such as polyadenylation, 7-methylguanosine capping, or splicing (Mattick et al., 2023). Tumor-suppressor lncRNAs and oncogenic lncRNAs are the two types of lncRNAs that can be distinguished based on their roles in tumors (Guzel et al., 2020). Moreover, in recent years, studies have identified lncRNAs linked to cancer in blood or other bodily fluids that are comparatively stable (Beylerli et al., 2022). lncRNAs found in plasma or serum may be employed as possible biomarkers for various tumor types (Le et al., 2021; Beylerli et al., 2022). Few studies have examined exosomal lncRNA expression as a possible minimally invasive diagnostic biomarker in various gastrointestinal cancers, such as colorectal cancer, gastric cancer, and pancreatic cancer (Gao et al., 2020; Kumar et al., 2022). A growing body of research has revealed that circulating lncRNAs are implicated in various gastrointestinal diseases and conditions (Liu et al., 2022). These include lncRNAs upregulated in hepatocellular carcinoma (highly upregulated in liver cancer, HULC) or in colorectal cancer (colon cancer-associated transcripts, CCATs) and various others (Zhang X. et al., 2019; Liu et al., 2022; Liau et al., 2023). Some of the lncRNAs that are upregulated in CRC are LNCV6/116109, LNCV6/98602, LNCV6/98390, LNCV6/84003, LNCV6/38772, and LNCV/108266 (Chen and Shen, 2020; Preethi et al., 2022).
In CRC management, the expression of exosome miRNAs such as HOTTIP (Oehme et al., 2019), SPINT1-AS1 (Li et al., 2018), and RPPH1 (Liang et al., 2019) can be determined to assess various survival parameters. Few other exosome miRNAs, such as GAS5 (Xu J. et al., 2020), H19 (Ren et al., 2018), 91H (Gao et al., 2018), and RPPH1 (Liang et al., 2019), correlate with the TNM stage, whereas others, such as HOTTIP (Oehme et al., 2019), CRNDE-h/p (Liu T. et al., 2016; Yu et al., 2017), APC1 (Wang J. et al., 2021), and CCAT2 (Wang S. et al., 2019), are reported to influence distance or lymph node metastasis. In gastric cancers, similar dysregulated exosomal lncRNAs could provide clinically valuable clues. Like an exosomal lncRNA, FRLnc1 is reported to signify poor survival and chances of recurrence and can also represent lymph node and distant metastasis (Kurnick et al., 2019; Zhang et al., 2021). There are also a wide range of exosomal miRNAs that potentially have diagnostic and prognostic applications in various gastrointestinal cancers (Gao et al., 2018; Roshani et al., 2022).
Moreover, exosomal lncRNAs have been shown in recent research to facilitate cell-to-cell communication within the TME, which aids in the growth and chemoresistance of cancer cells. For instance, by triggering the Wnt pathway, the overexpression of CAF-derived exosomal H19 can improve chemoresistance and encourage the stemness of CRC cells (Ren et al., 2018). Furthermore, exosomal colorectal cancer-associated lncRNA (CCAL) has been demonstrated to directly connect with the mRNA-stabilizing protein human antigen R (HuR), thereby enhancing CRC cell resistance to oxaliplatin (Deng et al., 2020). Consequently, as they might be crucial to the development of tumors, it is worth investigating the new lncRNAs that are abundant in exosomes created by endothelial cells that surround the TME. Exosomal lncRNA-UCA1 has been shown by Yang et al. to be capable of transmitting cetuximab resistance to susceptible CRC cells, and its expression is strongly associated with cetuximab treatment in CRC patients (Luan et al., 2020). Chen et al. (2020a) found that exosomal HOTTIP is significantly expressed in CRC cells that are resistant to mitomycin and can enhance CRC resistance to mitomycin by preventing miR-214 from degrading KPNA3. Therefore, one intriguing strategy for treating chemoresistance in CRC may be to target exosomal lncRNAs.
7.3 Exosomal circRNAs
The size of cirRNAs in exosomes ranges from 201 to 599 bp. circRNAs are a part of endogenous lncRNAs that have closed loops and are stable with longer half-lives (Wang Y. et al., 2019). One of the important functions of cirRNAs is to bind with RNA or proteins to regulate alternative splicing and transcription (Wang H. et al., 2019; Xu Y. et al., 2020). Dysregulated circRNAs and their contribution to influencing various malignancies have been well researched, with potential for clinical applications (Li et al., 2015; Zhang et al., 2023). Exosome-encapsulated circRNAs are found to be more stable in the serum/plasma of patients and, hence, more likely to indicate accurate lncRNA-associated clinical insights than other free circRNA species (Li et al., 2015). These molecular species can also be exploited for critical clinical information pertaining to prognosis and therapy responses (Yi et al., 2023). In CRC patients, exosomal has_circ_0004771 is upregulated compared to the serum of CRC post-operative patients, thereby showing potential as an early-stage detection biomarker for CRC (Pan et al., 2019). One such circulating exosome, circ-FBXW7, in combination with miR-128-3p, contributes to chemoresistance for oxaliplatin in CRC, thus suggesting an important therapeutic marker for CRC patients (Xu et al., 2021; Xu et al., 2024). Additionally, many exosomal circRNAs were found to play an important role in determining the resistance to chemotherapy in many cancer types, like gastric, esophageal, colorectal, pancreatic, and non-small lung cancers (Xu et al., 2024). However, a thorough mechanism remains unknown due to limited studies.
7.4 Exosomal proteins
Exosomal proteins are gaining interest in the detection of plasma and serum circulation. The transfer of oncoproteins from intercellular exosomes helps in the progression of tumors (Li W. et al., 2017), and thus, these exosomal proteins may carry tumor-specific biomarkers into circulation and can be used for diagnostic and prognostic applications (Wang X. et al., 2022; Liu et al., 2023). These proteins reflect the cellular origin of exosomes and also contribute to the detection of cancer. In pancreatic cancer, exosome proteins are reported to be in an elevated form as macrophage migration inhibitory factor (MIF) compared with healthy subjects (Chang and Pauklin, 2021). Moreover, the cell surface proteoglycan glypican-1 (GPC-1) is also found in exosomes extracted from pancreatic and breast cancer patients (Lorenzon and Blandino, 2016; Wang et al., 2019b), which can also be detected in the early and late stages of pancreatic cancer compared to the serum of healthy controls. In CRC patients, low CPNE3 exosomal proteins have shown better OS and DFS than higher CPNE3 exosomal protein levels in healthy individuals (Sun et al., 2019; Sun et al., 2019). In contrast, the QSOX1 exosomal protein is found to be low in the plasma of CRC patients compared to controls, potentially revealing diagnostic biomarkers with an ROC curve (AUC of 0.904) (Ganig et al., 2021). Furthermore, a possible therapeutic strategy for CRC would be to target the exosomal prion proteins found to be overexpressed in CRC. Drugs targeting these prion proteins, when administered along with 5-Fu, yielded better suppression of CRC progression (Yun et al., 2021). Moreover, exosomal PD-L1 is expressed in higher concentrations by cancer cells than that by healthy cells, and hence, its presence could indicate poorer prognosis (Lawler et al., 2020; Hu et al., 2023) (Table 3).
8 Technical challenges regarding the translation of liquid biopsy
Many clinical trials testing the efficiency of liquid biopsy components in the diagnosis of GI tract cancers have been conducted globally (Table 4). Despite that, the translation of liquid biopsy for clinical applications in cancer will require multiple challenges to be addressed. Among these, a major role is played by improving cellular and sub-cellular analyte isolation efficiency using better enrichment protocols (Li et al., 2020). Usually, many sequencing technologies are used to detect ctDNA, aiming to detect rare mutations and compare them with wildtype sequences. The most important recent techniques for the analysis of liquid biopsy are PCR-based and NGS technology. The digital droplet PCR (ddPCR) can identify tumor-specific mutations with high accuracy. ddPCR is a sensitive and rapid technology suitable for detecting mutations, and many such assays are commercialized, but it is limited by the restricted number of probes for each reaction. However, these limitations can be overcome with NGS platforms, which provide whole-genome sequencing of even new and rare molecular alterations without any prior knowledge (Lin S. Y. et al., 2021). NGS is also used to determine somatic single-nucleotide variants, allelic frequencies, copy number variations, and DNA methylation for ctDNA in plasma (Lin C. et al., 2021). Targeted sequencing of desired regions can also be performed using NGS platforms, but it requires a high ctDNA concentration for definitive data, indicating genome-wide changes specific to the tumor. Therefore, targeted sequencing has low specificity (Glenn, 2011), which can be improved by adapting to several methods to reduce background error rates (Snyder et al., 2016). Furthermore, bioinformatics-based approaches can be used to improvise the method and reduce error rates (Ma et al., 2019). One such tool used is the base position error rate (BPER), which is an algorithm used to detect the mean of all types of errors caused by the sequencing environment (Pécuchet et al., 2016). Another drawback of isolating and analyzing cfDNA/ctDNA from cancer patients is its disturbed integrity due to the presence of numerous ultrashort and jagged DNA fragments within ctDNA. This problem has been addressed by using a modified NGS pipeline tool known as BRcfDNA-Seq, which allows the faithful analysis of such low-integrity ctDNA in body fluids (Swarup et al., 2023).
Another such liquid biopsy analyte that emerged as a strong candidate to reveal disease status in cancer patients is exosomes and exosome-derived biomolecules. There are many methods for exosome isolation, each with its own limitations and advantages. The most common methods are ultracentrifugation and precipitation. High-speed centrifugation separates the molecules based on size and density differences. This technique has the potential for the isolation of exosomes. However, the instrument cost, low yield, stability, and large sample requirement make it inappropriate for use in clinical applications. Density gradient centrifugation can also be used with UC to purify exosomes. However, methods such as precipitation can be utilized, which reduces the solubility of exosomes in addition to its lower sample volume requirements and is comparatively inexpensive for clinical utilities. Moreover, new methods of enhanced exosome isolation assisted by microfluidics (Contreras-Naranjo et al., 2017) or nanomaterials (Fang et al., 2022) can be explored for higher isolation efficacies. In addition to the above, another technical challenge would be to reduce the false-positive scoring of analytes due to the presence of other conditions, such as stress, inflammatory disease, infectious disease, and other co-morbidities that are unrelated to tumors, so as to score only tumor-associated molecular or cellular footprints using liquid biopsy.
9 Conclusion
Liquid biopsy utilizes a range of tumor-related cellular, sub-cellular, and molecular analytes. These analytes are enumerated, qualified, quantified, and scored using varied parameters for use in several cancer types. These parameters are recorded using many technical and analytical tools on many platforms, ranging from flow cytometry, PCR, ddPCR, and immunofluorescent imaging to next-generation sequencing platforms. These techniques capture these analytes at different levels of sensitivities, given that the proportions of these disease-associated analytes are rare and comparatively limited in the bodily fluids from which they are isolated. Therefore, one of the major challenges to scoring these analytes before translating them into clinical settings would be to standardize them in large cohort studies. Moreover, another challenge of limited sensitivities and specificities of an analyte can be addressed by using a multi-analyte study design comprising a panel of biomarkers or a combination of various liquid biopsy analytes. For instance, a study observed an increase in the sensitivity of a singular miRNA-based test when it was co-analyzed with a serum biochemical marker CEA in detecting early-stage CRC (Wang et al., 2017). Similar analyses using top-scoring analytes could yield better and more focused sensitivities to diagnose a disease condition. Another consideration could be to ensure enhanced repeatability and dependability of outcomes through standardized protocols and processes that are required across various research and clinical settings across the world so that results can be compared without any false conclusions. Moreover, the detection of rare mutations and methylation markers or CNVs in molecular species such as ctDNA or cfDNA are better indicators of tumor progression and therapy response and, hence, must be further explored (Wu et al., 2021; Mo et al., 2023). Apart from this, liquid biopsy can have immense potential to emerge as a screening tool to identify early occurrences of cancer in asymptomatic and at-risk patients. This would require large-scale multi-center studies with consideration for multi-analyte investigations to decrease statistical biases with patient and biomarker diversity.
Author contributions
DM: conceptualization, methodology, writing–original draft, visualization, and writing–review and editing. SS: conceptualization, writing–original draft, and writing–review and editing. VS: writing–review and editing. VD: supervision and writing–review and editing. SP: conceptualization, supervision, validation, and writing–review and editing. RK: conceptualization, supervision, and writing–review and editing. ST: conceptualization, supervision, and writing–review and editing. NV: conceptualization, supervision, and writing–review and editing. DS: conceptualization, resources, supervision, visualization, and writing–review and editing.
Funding
The author(s) declare that no financial support was received for the research, authorship, and/or publication of this article.
Acknowledgments
The authors acknowledge the institutional and administrative support of the Department of Biotechnology, Guru Ghasidas Vishwavidyalaya (GGV). Collaborative and research support of clinicians of the All India Institute of Medical Sciences (AIIMS), Raipur, is appreciated, along with the courage and support of patients with cancer. Their sacrifice and contributions to this body of knowledge are appreciated. The authors also acknowledge research support of all our collaborators. The co-authors Sapnita Shinde (SS) and Vibha Sinha (VS) received the Senior Research Fellowship by Indian Council of Medical Research (ICMR), New Delhi.
Conflict of interest
Author ST was employed by Trivitron Healthcare Pvt Ltd.
The remaining authors declare that the research was conducted in the absence of any commercial or financial relationships that could be construed as a potential conflict of interest.
Publisher’s note
All claims expressed in this article are solely those of the authors and do not necessarily represent those of their affiliated organizations, or those of the publisher, the editors, and the reviewers. Any product that may be evaluated in this article, or claim that may be made by its manufacturer, is not guaranteed or endorsed by the publisher.
References
Alimirzaie, S., Bagherzadeh, M., and Akbari, M. R. (2019). Liquid biopsy in breast cancer: a comprehensive review. Clin. Genet. 95 (6), 643–660. doi:10.1111/cge.13514
Alunni-Fabbroni, M., Rönsch, K., Huber, T., Cyran, C. C., Seidensticker, M., Mayerle, J., et al. (2019). Circulating DNA as prognostic biomarker in patients with advanced hepatocellular carcinoma: a translational exploratory study from the SORAMIC trial. J. Transl. Med. 17 (1), 328. doi:10.1186/s12967-019-2079-9
Arnoletti, J. P., Reza, J., Rosales, A., Monreal, A., Fanaian, N., Whisner, S., et al. (2022). Pancreatic Ductal Adenocarcinoma (PDAC) circulating tumor cells influence myeloid cell differentiation to support their survival and immunoresistance in portal vein circulation. PLOS ONE 17 (3), e0265725. doi:10.1371/journal.pone.0265725
Asghar, S., Waqar, W., Umar, M., and Manzoor, S. (2020). Tumor educated platelets, a promising source for early detection of hepatocellular carcinoma: liquid biopsy an alternative approach to tissue biopsy. Clin. Res. Hepatology Gastroenterology 44 (6), 836–844. doi:10.1016/j.clinre.2020.03.023
Batool, S. M., Yekula, A., Khanna, P., Hsia, T., Gamblin, A. S., Ekanayake, E., et al. (2023). The Liquid Biopsy Consortium: challenges and opportunities for early cancer detection and monitoring. Cell Rep. Med. 4 (10), 101198. doi:10.1016/j.xcrm.2023.101198
Bergheim, J., Semaan, A., Gevensleben, H., Groening, S., Knoblich, A., Dietrich, J., et al. (2018). Potential of quantitative SEPT9 and SHOX2 methylation in plasmatic circulating cell-free DNA as auxiliary staging parameter in colorectal cancer: a prospective observational cohort study. Br. J. Cancer 118 (9), 1217–1228. doi:10.1038/s41416-018-0035-8
Bernard, V., Kim, D. U., San Lucas, F. A., Castillo, J., Allenson, K., Mulu, F. C., et al. (2019). Circulating nucleic acids are associated with outcomes of patients with pancreatic cancer. Gastroenterology 156 (1), 108–118. doi:10.1053/j.gastro.2018.09.022
Best, M. G., Sol, N., Kooi, I., Tannous, J., Westerman, B. A., Rustenburg, F., et al. (2015). RNA-seq of tumor-educated platelets enables blood-based pan-cancer, multiclass, and molecular pathway cancer diagnostics. Cancer Cell 28 (5), 666–676. doi:10.1016/j.ccell.2015.09.018
Best, M. G., and Wurdinger, T. (2020). Tumor-educated platelets for the earlier detection of hepatocellular carcinoma. Clin. Res. hepatology gastroenterology 44 (6), 794–795. doi:10.1016/j.clinre.2020.03.028
Bettegowda, C., Sausen, M., Leary, R. J., Kinde, I., Wang, Y., Agrawal, N., et al. (2014). Detection of circulating tumor DNA in early- and late-stage human malignancies. Sci. Transl. Med. 6 (224), 224ra24. doi:10.1126/scitranslmed.3007094
Beylerli, O., Gareev, I., Sufianov, A., Ilyasova, T., and Guang, Y. (2022). Long noncoding RNAs as promising biomarkers in cancer. Non-Coding RNA Res. 7 (2), 66–70. doi:10.1016/j.ncrna.2022.02.004
Bidard, F.-C., Kiavue, N., Ychou, M., Cabel, L., Stern, M.-H., Madic, J., et al. (2019). Circulating tumor cells and circulating tumor DNA detection in potentially resectable metastatic colorectal cancer: a prospective ancillary study to the unicancer prodige-14 trial. Cells 8 (6), 516. doi:10.3390/cells8060516
Boerrigter, E., Groen, L. N., Van Erp, N. P., Verhaegh, G. W., and Schalken, J. A. (2020). Clinical utility of emerging biomarkers in prostate cancer liquid biopsies. Expert Rev. Mol. Diagnostics 20 (2), 219–230. doi:10.1080/14737159.2019.1675515
Bork, U., Rahbari, N. N., Schölch, S., Reissfelder, C., Kahlert, C., Büchler, M. W., et al. (2015). Circulating tumour cells and outcome in non-metastatic colorectal cancer: a prospective study. Br. J. Cancer 112 (8), 1306–1313. doi:10.1038/bjc.2015.88
Bronkhorst, A. J., Ungerer, V., and Holdenrieder, S. (2019). The emerging role of cell-free DNA as a molecular marker for cancer management. Biomol. Detect. Quantification 17, 100087. doi:10.1016/j.bdq.2019.100087
Cai, G., Cai, M., Feng, Z., Liu, R., Liang, L., Zhou, P., et al. (2021). A multilocus blood-based assay targeting circulating tumor DNA methylation enables early detection and early relapse prediction of colorectal cancer. Gastroenterology 161 (6), 2053–2056.e2. doi:10.1053/j.gastro.2021.08.054
Cai, J., Huang, L., Huang, J., Kang, L., Lin, H., Huang, P., et al. (2019). Associations between the cyclooxygenase-2 expression in circulating tumor cells and the clinicopathological features of patients with colorectal cancer. J. Cell. Biochem. 120 (4), 4935–4941. doi:10.1002/jcb.27768
Caldas, C., Hahn, S. A., Hruban, R. H., Redston, M. S., Yeo, C. J., and Kern, S. E. (1994). Detection of K-ras mutations in the stool of patients with pancreatic adenocarcinoma and pancreatic ductal hyperplasia. Cancer Res. 54 (13), 3568–3573.
Campanella, R., Guarnaccia, L., Cordiglieri, C., Trombetta, E., Caroli, M., Carrabba, G., et al. (2020). Tumor-educated platelets and angiogenesis in glioblastoma: another brick in the wall for novel prognostic and targetable biomarkers, changing the vision from a localized tumor to a systemic pathology. Cells 9 (2), 294. doi:10.3390/cells9020294
Cao, D., Cao, X., Jiang, Y., Xu, J., Zheng, Y., Kang, D., et al. (2022). Circulating exosomal microRNAs as diagnostic and prognostic biomarkers in patients with diffuse large B-cell lymphoma. Hematol. Oncol. 40 (2), 172–180. doi:10.1002/hon.2956
Cao, F., Wei, A., Hu, X., He, Y., Zhang, J., Xia, L., et al. (2020). Integrated epigenetic biomarkers in circulating cell-free DNA as a robust classifier for pancreatic cancer. Clin. Epigenetics 12 (1), 112. doi:10.1186/s13148-020-00898-2
Cao, Y., Yang, M., Peng, T., Liu, Y., and Yu, J. (2023). Evaluation of cell surface vimentin positive circulating tumor cells as a prognostic biomarker for stage III/IV colorectal cancer. Sci. Rep. 13 (1), 18791. doi:10.1038/s41598-023-45951-1
Castro, J., Sanchez, L., Nuñez, M. T., Lu, M., Castro, T., Sharifi, H. R., et al. (2018). Screening circulating tumor cells as a noninvasive cancer test in 3388 individuals from high-risk groups (ICELLATE2). Dis. Markers 2018, 4653109. doi:10.1155/2018/4653109
Chakrabarti, S., Bucheit, L., Starr, J. S., Innis-Shelton, R., Shergill, A., Dada, H., et al. (2022). Detection of microsatellite instability-high (MSI-H) by liquid biopsy predicts robust and durable response to immunotherapy in patients with pancreatic cancer. J. Immunother. Cancer 10 (6), e004485. doi:10.1136/jitc-2021-004485
Chang, C.-H., and Pauklin, S. (2021). Extracellular vesicles in pancreatic cancer progression and therapies. Cell Death Dis. 12 (11), 973. doi:10.1038/s41419-021-04258-7
Chauhan, A., Kaur, R., Ghoshal, S., and Pal, A. (2021). Exploration of circulating tumour cell (CTC) biology: a paradigm shift in liquid biopsy. Indian J. Clin. Biochem. 36 (2), 131–142. doi:10.1007/s12291-020-00923-4
Chen, C.-J., Sung, W.-W., Chen, H.-C., Chern, Y.-J., Hsu, H.-T., Lin, Y.-M., et al. (2017). Early assessment of colorectal cancer by quantifying circulating tumor cells in peripheral blood: ECT2 in diagnosis of colorectal cancer. Int. J. Mol. Sci. 18 (4), 743. doi:10.3390/ijms18040743
Chen, G., Peng, J., Xiao, Q., Wu, H.-X., Wu, X., Wang, F., et al. (2021). Postoperative circulating tumor DNA as markers of recurrence risk in stages II to III colorectal cancer. J. Hematol. Oncol. 14 (1), 80. doi:10.1186/s13045-021-01089-z
Chen, Q., Zou, J., He, Y., Pan, Y., Yang, G., Zhao, H., et al. (2022). A narrative review of circulating tumor cells clusters: a key morphology of cancer cells in circulation promote hematogenous metastasis. Front. Oncol. 12, 944487. doi:10.3389/fonc.2022.944487
Chen, S., and Shen, X. (2020). Long noncoding RNAs: functions and mechanisms in colon cancer. Mol. Cancer 19 (1), 167. doi:10.1186/s12943-020-01287-2
Chen, X., Bai, K., Zhang, Y., Xu, Y., Huo, Y., Wang, S., et al. (2023). Genomic alterations of cerebrospinal fluid cell-free DNA in leptomeningeal metastases of gastric cancer. J. Transl. Med. 21 (1), 296. doi:10.1186/s12967-023-04077-8
Chen, X., Gole, J., Gore, A., He, Q., Lu, M., Min, J., et al. (2020a). Non-invasive early detection of cancer four years before conventional diagnosis using a blood test. Nat. Commun. 11 (1), 3475. doi:10.1038/s41467-020-17316-z
Chen, X., Liu, Y., Zhang, Q., Liu, B., Cheng, Y., Zhang, Y., et al. (2020b). Exosomal long non-coding RNA HOTTIP increases resistance of colorectal cancer cells to mitomycin via impairing MiR-214-mediated degradation of KPNA3. Front. Cell Dev. Biol. 8, 582723. doi:10.3389/fcell.2020.582723
Chen, Y., Li, S., Li, W., Yang, R., Zhang, X., Ye, Y., et al. (2019). Circulating tumor cells undergoing EMT are poorly correlated with clinical stages or predictive of recurrence in hepatocellular carcinoma. Sci. Rep. 9 (1), 7084. doi:10.1038/s41598-019-43572-1
Cho, H. J., Eun, J. W., Baek, G. O., Seo, C. W., Ahn, H. R., Kim, S. S., et al. (2020). Serum exosomal MicroRNA, miR-10b-5p, as a potential diagnostic biomarker for early-stage hepatocellular carcinoma. J. Clin. Med. 9 (1), 281. doi:10.3390/jcm9010281
Cohen, S. A., Kasi, P. M., Aushev, V. N., Hanna, D. L., Botta, G. P., Sharif, S., et al. (2023). Kinetics of postoperative circulating cell-free DNA and impact on minimal residual disease detection rates in patients with resected stage I-III colorectal cancer. J. Clin. Oncol. 41 (4_Suppl. l), 5. doi:10.1200/JCO.2023.41.4_suppl.5
Cohen, S. J., Punt, C. J. A., Iannotti, N., Saidman, B. H., Sabbath, K. D., Gabrail, N. Y., et al. (2009). Prognostic significance of circulating tumor cells in patients with metastatic colorectal cancer. Ann. Oncol. Official J. Eur. Soc. Med. Oncol. 20 (7), 1223–1229. doi:10.1093/annonc/mdn786
Contreras-Naranjo, J. C., Wu, H.-J., and Ugaz, V. M. (2017). Microfluidics for exosome isolation and analysis: enabling liquid biopsy for personalized medicine. Lab a Chip 17 (21), 3558–3577. doi:10.1039/c7lc00592j
Contursi, A., Fullone, R., Szklanna-Koszalinska, P., Marcone, S., Lanuti, P., Taus, F., et al. (2023). Tumor-educated platelet extracellular vesicles: proteomic profiling and crosstalk with colorectal cancer cells. Cancers 15 (2), 350. doi:10.3390/cancers15020350
Coto-Llerena, M., Benjak, A., Gallon, J., Meier, M.-A., Boldanova, T., Terracciano, L. M., et al. (2022). Circulating cell-free DNA captures the intratumor heterogeneity in multinodular hepatocellular carcinoma. JCO Precis. Oncol. 6, e2100335. doi:10.1200/PO.21.00335
Court, C. M., Ankeny, J. S., Sho, S., Winograd, P., Hou, S., Song, M., et al. (2018). Circulating tumor cells predict occult metastatic disease and prognosis in pancreatic cancer. Ann. Surg. Oncol. 25 (4), 1000–1008. doi:10.1245/s10434-017-6290-8
Dalhammar, K., Kristensson, J., Falkenback, D., Rasmussen, B. H., and Malmström, M. (2022). Symptoms, problems and quality of life in patients newly diagnosed with oesophageal and gastric cancer – a comparative study of treatment strategy. BMC Cancer 22 (1), 434. doi:10.1186/s12885-022-09536-x
D’Ambrosi, S., Nilsson, R. J., and Wurdinger, T. (2021). Platelets and tumor-associated RNA transfer. Blood 137 (23), 3181–3191. doi:10.1182/blood.2019003978
Delgado-Ureña, M., Ortega, F. G., de Miguel-Pérez, D., Rodriguez-Martínez, A., García-Puche, J. L., Ilyine, H., et al. (2018). Circulating tumor cells criteria (CyCAR) versus standard RECIST criteria for treatment response assessment in metastatic colorectal cancer patients. J. Transl. Med. 16 (1), 251. doi:10.1186/s12967-018-1624-2
Deng, X., Ruan, H., Zhang, X., Xu, X., Zhu, Y., Peng, H., et al. (2020). Long noncoding RNA CCAL transferred from fibroblasts by exosomes promotes chemoresistance of colorectal cancer cells. Int. J. Cancer 146 (6), 1700–1716. doi:10.1002/ijc.32608
Dizdar, L., Fluegen, G., van Dalum, G., Honisch, E., Neves, R. P., Niederacher, D., et al. (2019). Detection of circulating tumor cells in colorectal cancer patients using the GILUPI CellCollector: results from a prospective, single-center study. Mol. Oncol. 13 (7), 1548–1558. doi:10.1002/1878-0261.12507
Dohmen, J., Semaan, A., Kobilay, M., Zaleski, M., Branchi, V., Schlierf, A., et al. (2022). Diagnostic potential of exosomal microRNAs in colorectal cancer. Diagn. Basel, Switz. 12 (6), 1413. doi:10.3390/diagnostics12061413
Dong, X., Song, X., Ding, S., Yu, M., Shang, X., Wang, K., et al. (2021). Tumor-educated platelet SNORD55 as a potential biomarker for the early diagnosis of non-small cell lung cancer. Thorac. Cancer 12 (5), 659–666. doi:10.1111/1759-7714.13823
Doyle, L. M., and Wang, M. Z. (2019). Overview of extracellular vesicles, their origin, composition, purpose, and methods for exosome isolation and analysis. Cells 8 (7), 727. doi:10.3390/cells8070727
El-Arabey, A. A., Abdalla, M., and Abd-Allah, A. R. (2020). GATA3 and stemness of high-grade serous ovarian carcinoma: novel hope for the deadliest type of ovarian cancer. Hum. Cell 33 (3), 904–906. doi:10.1007/s13577-020-00368-0
Fang, X., Wang, Y., Wang, S., and Liu, B. (2022). Nanomaterials assisted exosomes isolation and analysis towards liquid biopsy. Mater. Today Bio 16, 100371. doi:10.1016/j.mtbio.2022.100371
Fang, Z.-T., Zhang, W., Wang, G.-Z., Zhou, B., Yang, G.-W., Qu, X.-D., et al. (2014). Circulating tumor cells in the central and peripheral venous compartment – assessing hematogenous dissemination after transarterial chemoembolization of hepatocellular carcinoma. OncoTargets Ther. 7 (null), 1311–1318. doi:10.2147/OTT.S62605
Frattini, M., Gallino, G., Signoroni, S., Balestra, D., Lusa, L., Battaglia, L., et al. (2008). Quantitative and qualitative characterization of plasma DNA identifies primary and recurrent colorectal cancer. Cancer Lett. 263 (2), 170–181. doi:10.1016/j.canlet.2008.03.021
Friberg, S., and Nystrom, A. (2015). Cancer metastases: early dissemination and late recurrences. Cancer Growth Metastasis 8, 43–49. doi:10.4137/CGM.S31244
Fu, Y., Ye, Y., Liu, X., Zhu, G., Xu, Y., Sun, J., et al. (2021). Analyzing microsatellite instability and gene mutation in circulating cell-free DNA to monitor colorectal cancer progression. Transl. Cancer Res. 10 (6), 2812–2821. doi:10.21037/tcr-20-2762
Galvão-Lima, L. J., Morais, A. H. F., Valentim, R. A. M., and Barreto, E. J. S. S. (2021). miRNAs as biomarkers for early cancer detection and their application in the development of new diagnostic tools. Biomed. Eng. OnLine 20 (1), 21. doi:10.1186/s12938-021-00857-9
Ganig, N., Baenke, F., Thepkaysone, M.-L., Lin, K., Rao, V. S., Wong, F. C., et al. (2021). Proteomic analyses of fibroblast- and serum-derived exosomes identify QSOX1 as a marker for non-invasive detection of colorectal cancer. Cancers 13 (6), 1351. doi:10.3390/cancers13061351
Gao, T., Liu, X., He, B., Nie, Z., Zhu, C., Zhang, P., et al. (2018). Exosomal lncRNA 91H is associated with poor development in colorectal cancer by modifying HNRNPK expression. Cancer Cell Int. 18, 11. doi:10.1186/s12935-018-0506-2
Gao, Y., Wang, J.-W., Ren, J.-Y., Guo, M., Guo, C.-W., Ning, S.-W., et al. (2020). Long noncoding RNAs in gastric cancer: from molecular dissection to clinical application. World J. Gastroenterology 26 (24), 3401–3412. doi:10.3748/wjg.v26.i24.3401
Gardner, K. P., Aldakkak, M., Tang, C.-M., Tsai, S., and Adams, D. L. (2021). Circulating stromal cells in resectable pancreatic cancer correlates to pathological stage and predicts for poor clinical outcomes. NPJ Precis. Oncol. 5 (1), 25. doi:10.1038/s41698-021-00161-8
Gemenetzis, G., Groot, V. P., Yu, J., Ding, D., Teinor, J. A., Javed, A. A., et al. (2018). Circulating tumor cells dynamics in pancreatic adenocarcinoma correlate with disease status: results of the prospective CLUSTER study. Ann. Surg. 268 (3), 408–420. doi:10.1097/SLA.0000000000002925
Glenn, T. C. (2011). Field guide to next-generation DNA sequencers. Mol. Ecol. Resour. 11 (5), 759–769. doi:10.1111/j.1755-0998.2011.03024.x
Guo, H., Wang, Y., Wang, Z., Wang, Z., and Xue, S. (2021). The diagnostic and prognostic value of miR-92a in gastric cancer: a systematic review and meta-analysis. Open Med. Wars. Pol. 16 (1), 1386–1394. doi:10.1515/med-2021-0347
Gurung, S., Perocheau, D., Touramanidou, L., and Baruteau, J. (2021). The exosome journey: from biogenesis to uptake and intracellular signalling. Cell Commun. Signal. CCS 19 (1), 47. doi:10.1186/s12964-021-00730-1
Guzel, E., Okyay, T. M., Yalcinkaya, B., Karacaoglu, S., Gocmen, M., and Akcakuyu, M. H. (2020). Tumor suppressor and oncogenic role of long non-coding RNAs in cancer. North. Clin. Istanbul 7 (1), 81–86. doi:10.14744/nci.2019.46873
Ha, Y., Kim, T. H., Shim, J. E., Yoon, S., Jun, M. J., Cho, Y.-H., et al. (2019). Circulating tumor cells are associated with poor outcomes in early-stage hepatocellular carcinoma: a prospective study. Hepatol. Int. 13 (6), 726–735. doi:10.1007/s12072-019-09994-9
Hamfjord, J., Guren, T. K., Dajani, O., Johansen, J. S., Glimelius, B., Sorbye, H., et al. (2019). Total circulating cell-free DNA as a prognostic biomarker in metastatic colorectal cancer before first-line oxaliplatin-based chemotherapy. Ann. Oncol. 30 (7), 1088–1095. doi:10.1093/annonc/mdz139
Han, L., Chen, W., and Zhao, Q. (2014). Prognostic value of circulating tumor cells in patients with pancreatic cancer: a meta-analysis. Tumour Biol. J. Int. Soc. Oncodevelopmental Biol. Med. 35 (3), 2473–2480. doi:10.1007/s13277-013-1327-5
Han, Y., Wei, J., Wang, W., Gao, R., Shen, N., Song, X., et al. (2023). Multidimensional analysis of a cell-free DNA whole methylome sequencing assay for early detection of gastric cancer: protocol for an observational case-control study. JMIR Res. Protoc. 12, e48247. doi:10.2196/48247
Harlé, A. (2020). Cell-free DNA in the management of colorectal cancer. Progres. Dans Les. Rech. Sur Le. Cancer 215, 253–261. doi:10.1007/978-3-030-26439-0_13
Heiss, M. M., Allgayer, H., Gruetzner, K. U., Funke, I., Babic, R., Jauch, K. W., et al. (1995). Individual development and uPA-receptor expression of disseminated tumour cells in bone marrow: a reference to early systemic disease in solid cancer. Nat. Med. 1 (10), 1035–1039. doi:10.1038/nm1095-1035
Henriksen, T. V., Tarazona, N., Frydendahl, A., Reinert, T., Gimeno-Valiente, F., Carbonell-Asins, J. A., et al. (2022). Circulating tumor DNA in stage III colorectal cancer, beyond minimal residual disease detection, toward assessment of adjuvant therapy efficacy and clinical behavior of recurrences. Clin. Cancer Res. 28 (3), 507–517. doi:10.1158/1078-0432.CCR-21-2404
Higuera, M., Vargas-Accarino, E., Torrens, M., Gregori, J., Salcedo, M. T., Martínez-Campreciós, J., et al. (2022). Ultra deep sequencing of circulating cell-free DNA as a potential tool for hepatocellular carcinoma management. Cancers 14 (16), 3875. doi:10.3390/cancers14163875
Hu, R., Jahan, M. S., and Tang, L. (2023). ExoPD-L1: an assistant for tumor progression and potential diagnostic marker. Front. Oncol. 13, 1194180. doi:10.3389/fonc.2023.1194180
Huang, X., Gao, P., Sun, J., Chen, X., Song, Y., Zhao, J., et al. (2015). Clinicopathological and prognostic significance of circulating tumor cells in patients with gastric cancer: a meta-analysis. Int. J. Cancer 136 (1), 21–33. doi:10.1002/ijc.28954
Huang, Z., Hua, D., Hu, Y., Cheng, Z., Zhou, X., Xie, Q., et al. (2012). Quantitation of plasma circulating DNA using quantitative PCR for the detection of hepatocellular carcinoma. Pathology Oncol. Res. POR 18 (2), 271–276. doi:10.1007/s12253-011-9438-z
Hugenschmidt, H., Labori, K. J., Borgen, E., Brunborg, C., Schirmer, C. B., Seeberg, L. T., et al. (2021). Preoperative CTC-detection by CellSearch(®) is associated with early distant metastasis and impaired survival in resected pancreatic cancer. Cancers 13 (3), 485. doi:10.3390/cancers13030485
Hummel, E. M., Hessas, E., Müller, S., Beiter, T., Fisch, M., Eibl, A., et al. (2018). Cell-free DNA release under psychosocial and physical stress conditions. Transl. Psychiatry 8 (1), 236. doi:10.1038/s41398-018-0264-x
Hussung, S., Akhoundova, D., Hipp, J., Follo, M., Klar, R. F. U., Philipp, U., et al. (2021). Longitudinal analysis of cell-free mutated KRAS and CA 19-9 predicts survival following curative resection of pancreatic cancer. BMC cancer 21 (1), 49. doi:10.1186/s12885-020-07736-x
Iizuka, N., Sakaida, I., Moribe, T., Fujita, N., Miura, T., Stark, M., et al. (2006). Elevated levels of circulating cell-free DNA in the blood of patients with hepatitis C virus-associated hepatocellular carcinoma. Anticancer Res. 26 (6C), 4713–4719.
Inagaki, C., Kawakami, H., Maeda, D., Sakai, D., Urakawa, S., Nishida, K., et al. (2023). The potential clinical utility of cell-free DNA for gastric cancer patients treated with nivolumab monotherapy. Sci. Rep. 13 (1), 5652. doi:10.1038/s41598-023-32645-x
Iwu, C. D., and Iwu-Jaja, C. J. (2023). Gastric cancer epidemiology: current trend and future direction. Hygiene 3 (3), 256–268. doi:10.3390/hygiene3030019
Jang, J. W., Kim, J. M., Kim, H. S., Kim, J. S., Han, J. W., Lee, S. K., et al. (2022). Diagnostic performance of serum exosomal miRNA-720 in hepatocellular carcinoma. J. Liver Cancer 22 (1), 30–39. doi:10.17998/jlc.2022.02.25
Jauhri, M., Bhatnagar, A., Gupta, S., Bp, M., Minhas, S., Shokeen, Y., et al. (2017). Prevalence and coexistence of KRAS, BRAF, PIK3CA, NRAS, TP53, and APC mutations in Indian colorectal cancer patients: next-generation sequencing-based cohort study. Tumour Biol. J. Int. Soc. Oncodevelopmental Biol. Med. 39 (2), 1010428317692265. doi:10.1177/1010428317692265
Joseph, A., Raja, S., Kamath, S., Jang, S., Allende, D., McNamara, M., et al. (2022). Esophageal adenocarcinoma: a dire need for early detection and treatment. Clevel. Clin. J. Med. 89 (5), 269–279. doi:10.3949/ccjm.89a.21053
Kalluri, R., and LeBleu, V. S. (2020). The biology, function, and biomedical applications of exosomes. Sci. (New York, N.Y.) 367 (6478). doi:10.1126/science.aau6977
Kang, H. M., Kim, G. H., Jeon, H. K., Kim, D. H., Jeon, T. Y., Park, D. Y., et al. (2017). Circulating tumor cells detected by lab-on-a-disc: role in early diagnosis of gastric cancer. PloS One 12 (6), e0180251. doi:10.1371/journal.pone.0180251
Kawamura, S., Iinuma, H., Wada, K., Takahashi, K., Minezaki, S., Kainuma, M., et al. (2019). Exosome-encapsulated microRNA-4525, microRNA-451a and microRNA-21 in portal vein blood is a high-sensitive liquid biomarker for the selection of high-risk pancreatic ductal adenocarcinoma patients. J. Hepato-Biliary-Pancreatic Sci. 26 (2), 63–72. doi:10.1002/jhbp.601
Kayali, S., Marabotto, E., and Giannini, E. (2023). Gastrointestinal tract cancers, an increasing burden of the modern era: epidemiology and prevention. Cancers 15 (18), 4634. doi:10.3390/cancers15184634
Khan, K. H., Cunningham, D., Werner, B., Vlachogiannis, G., Spiteri, I., Heide, T., et al. (2018). Longitudinal liquid biopsy and mathematical modeling of clonal evolution forecast time to treatment failure in the prospect-c phase II colorectal cancer clinical trial. Cancer Discov. 8 (10), 1270–1285. doi:10.1158/2159-8290.CD-17-0891
Kim, B., Kim, Y., Cho, J. Y., and Lee, K.-A. (2024). Identification of potential genomic alterations using pan-cancer cell-free DNA next-generation sequencing in patients with gastric cancer. Ann. Laboratory Med. 44 (2), 164–173. doi:10.3343/alm.2023.0187
Kim, D.-D., Yang, C.-S., Chae, H.-D., Kwak, S.-G., and Jeon, C.-H. (2017). Melanoma antigen-encoding gene family member A1-6 and hTERT in the detection of circulating tumor cells following CD45(-) depletion and RNA extraction. Oncol. Lett. 14 (1), 837–843. doi:10.3892/ol.2017.6226
Kim, M. K., Woo, S. M., Park, B., Yoon, K.-A., Kim, Y.-H., Joo, J., et al. (2018). Prognostic implications of multiplex detection of KRAS mutations in cell-free DNA from patients with pancreatic ductal adenocarcinoma. Clin. Chem. 64 (4), 726–734. doi:10.1373/clinchem.2017.283721
Kim, S.-C., Kim, D.-W., Cho, E. J., Lee, J.-Y., Kim, J., Kwon, C., et al. (2023). A circulating cell-free DNA methylation signature for the detection of hepatocellular carcinoma. Mol. cancer 22 (Issue 1), 164. doi:10.1186/s12943-023-01872-1
Koldby, K. M., Mortensen, M. B., Detlefsen, S., Pfeiffer, P., Thomassen, M., and Kruse, T. A. (2019). Tumor-specific genetic aberrations in cell-free DNA of gastroesophageal cancer patients. J. Gastroenterology 54 (2), 108–121. doi:10.1007/s00535-018-1508-5
Kumar, D. P., Manu, K. A., and Macha, M. A. (2022). Editorial: the role of non-coding RNAs in gastrointestinal cancer. Front. Oncol. 12, 1056897. doi:10.3389/fonc.2022.1056897
Kurnick, S. A., Ge, Z., Dzink-Fox, J., Muthupalani, S., and Fox, J. G. (2019). Mo1784–the role of Foxm1 in helicobacter pylori-associated gastric cancer. Gastroenterology 156 (6), S–837. doi:10.1016/s0016-5085(19)39051-1
Lambert, M. P. (2016). Platelets in liver and renal disease. Hematol. Am. Soc. Hematol. Educ. Program 2016 (1), 251–255. doi:10.1182/asheducation-2016.1.251
Lawler, S. E., Nowicki, M. O., Ricklefs, F. L., and Chiocca, E. A. (2020). Immune escape mediated by exosomal PD-L1 in cancer. Adv. Biosyst. 4 (12), e2000017. doi:10.1002/adbi.202000017
Le, P., Romano, G., Nana-Sinkam, P., and Acunzo, M. (2021). Non-coding RNAs in cancer diagnosis and therapy: focus on lung cancer. Cancers 13 (6), 1372. doi:10.3390/cancers13061372
Le, U.-T., Bronsert, P., Picardo, F., Riethdorf, S., Haager, B., Rylski, B., et al. (2018). Intraoperative detection of circulating tumor cells in pulmonary venous blood during metastasectomy for colorectal lung metastases. Sci. Rep. 8 (1), 8751. doi:10.1038/s41598-018-26410-8
Lee, S. J., Lee, J., Kim, S. T., Park, S. H., Park, J. O., Park, Y. S., et al. (2015). Circulating tumor cells are predictive of poor response to chemotherapy in metastatic gastric cancer. Int. J. Biol. Markers 30 (4), e382–e386. doi:10.5301/jbm.5000151
Leon, S. A., Shapiro, B., Sklaroff, D. M., and Yaros, M. J. (1977). Free DNA in the serum of cancer patients and the effect of therapy. Cancer Res. 37 (3), 646–650.
Leung, F., Kulasingam, V., Diamandis, E. P., Hoon, D. S. B., Kinzler, K., Pantel, K., et al. (2016). Circulating tumor DNA as a cancer biomarker: fact or fiction? Clin. Chem. 62 (8), 1054–1060. doi:10.1373/clinchem.2016.260331
Li, C., Li, W., Zhang, Y., Zhang, X., Liu, T., Zhang, Y., et al. (2018). Increased expression of antisense lncRNA SPINT1-AS1 predicts a poor prognosis in colorectal cancer and is negatively correlated with its sense transcript. OncoTargets Ther. 11, 3969–3978. doi:10.2147/OTT.S163883
Li, C., Zhou, T., Chen, J., Li, R., Chen, H., Luo, S., et al. (2022a). The role of Exosomal miRNAs in cancer. J. Transl. Med. 20 (1), 6. doi:10.1186/s12967-021-03215-4
Li, J., Dittmar, R. L., Xia, S., Zhang, H., Du, M., Huang, C.-C., et al. (2017a). Cell-free DNA copy number variations in plasma from colorectal cancer patients. Mol. Oncol. 11 (8), 1099–1111. doi:10.1002/1878-0261.12077
Li, J., Zhou, X., Liu, X., Ren, J., Wang, J., Wang, W., et al. (2019). Detection of colorectal cancer in circulating cell-free DNA by methylated CpG tandem amplification and sequencing. Clin. Chem. 65 (7), 916–926. doi:10.1373/clinchem.2019.301804
Li, Q., Feng, Z., Miao, R., Liu, X., Liu, C., and Liu, Z. (2022b). Prognosis and survival analysis of patients with pancreatic cancer: retrospective experience of a single institution. World J. Surg. Oncol. 20 (1), 11. doi:10.1186/s12957-021-02478-x
Li, W., Li, C., Zhou, T., Liu, X., Liu, X., Li, X., et al. (2017b). Role of exosomal proteins in cancer diagnosis. Mol. Cancer 16 (1), 145. doi:10.1186/s12943-017-0706-8
Li, W., Liu, J.-B., Hou, L.-K., Yu, F., Zhang, J., Wu, W., et al. (2022c). Liquid biopsy in lung cancer: significance in diagnostics, prediction, and treatment monitoring. Mol. Cancer 21 (1), 25. doi:10.1186/s12943-022-01505-z
Li, X., Li, Y., Shao, W., Li, Z., Zhao, R., and Ye, Z. (2020). Strategies for enrichment of circulating tumor cells. Transl. Cancer Res. 9 (3), 2012–2025. doi:10.21037/tcr.2020.01.17
Li, Y., Gong, J., Zhang, Q., Lu, Z., Gao, J., Li, Y., et al. (2016). Dynamic monitoring of circulating tumour cells to evaluate therapeutic efficacy in advanced gastric cancer. Br. J. Cancer 114 (2), 138–145. doi:10.1038/bjc.2015.417
Li, Y., Zheng, Q., Bao, C., Li, S., Guo, W., Zhao, J., et al. (2015). Circular RNA is enriched and stable in exosomes: a promising biomarker for cancer diagnosis. Cell Res. 25 (8), 981–984. doi:10.1038/cr.2015.82
Li, Z., Song, M., Han, S., Jin, C., and Yang, J. (2022d). The prognostic role of circulating tumor cells in gastric cancer: a meta-analysis. Front. Oncol. 12, 963091. doi:10.3389/fonc.2022.963091
Liang, Z.-X., Liu, H.-S., Wang, F.-W., Xiong, L., Zhou, C., Hu, T., et al. (2019). LncRNA RPPH1 promotes colorectal cancer metastasis by interacting with TUBB3 and by promoting exosomes-mediated macrophage M2 polarization. Cell Death Dis. 10 (11), 829. doi:10.1038/s41419-019-2077-0
Liau, X. L., Salvamani, S., Gunasekaran, B., Chellappan, D. K., Rhodes, A., Ulaganathan, V., et al. (2023). CCAT 1- A pivotal oncogenic long non-coding RNA in colorectal cancer. Br. J. Biomed. Sci. 80, 11103. doi:10.3389/bjbs.2023.11103
Lin, C., Liu, X., Zheng, B., Ke, R., and Tzeng, C.-M. (2021a). Liquid biopsy, ctDNA diagnosis through NGS. Life Basel, Switz. 11 (9), 890. doi:10.3390/life11090890
Lin, S. Y., Chang, T.-T., Steffen, J. D., Chen, S., Jain, S., Song, W., et al. (2021b). Detection of CTNNB1 hotspot mutations in cell-free DNA from the urine of hepatocellular carcinoma patients. Diagn. Basel, Switz. 11 (8), 1475. doi:10.3390/diagnostics11081475
Liu, C., Eng, C., Shen, J., Lu, Y., Takata, Y., Mehdizadeh, A., et al. (2016a). Serum exosomal miR-4772-3p is a predictor of tumor recurrence in stage II and III colon cancer. Oncotarget 7 (46), 76250–76260. doi:10.18632/oncotarget.12841
Liu, M., Lai, Z., Yuan, X., Jin, Q., Shen, H., Rao, D., et al. (2023). Role of exosomes in the development, diagnosis, prognosis and treatment of hepatocellular carcinoma. Mol. Med. 29 (1), 136. doi:10.1186/s10020-023-00731-5
Liu, T., Zhang, X., Gao, S., Jing, F., Yang, Y., Du, L., et al. (2016b). Exosomal long noncoding RNA CRNDE-h as a novel serum-based biomarker for diagnosis and prognosis of colorectal cancer. Oncotarget 7 (51), 85551–85563. doi:10.18632/oncotarget.13465
Liu, Y., Ao, X., Wang, Y., Li, X., and Wang, J. (2022). Long non-coding RNA in gastric cancer: mechanisms and clinical implications for drug resistance. Front. Oncol. 12, 841411. doi:10.3389/fonc.2022.841411
Liu, Y., Ling, Y., Qi, Q., Lan, F., Zhu, M., Zhang, Y., et al. (2017). Prognostic value of circulating tumor cells in advanced gastric cancer patients receiving chemotherapy. Mol. Clin. Oncol. 6 (2), 235–242. doi:10.3892/mco.2017.1125
Lone, S. N., Nisar, S., Masoodi, T., Singh, M., Rizwan, A., Hashem, S., et al. (2022). Liquid biopsy: a step closer to transform diagnosis, prognosis and future of cancer treatments. Mol. Cancer 21 (1), 79. doi:10.1186/s12943-022-01543-7
Lorenzon, L., and Blandino, G. (2016). Glypican-1 exosomes: do they initiate a new era for early pancreatic cancer diagnosis? Transl. Gastroenterology Hepatology 1, 8. doi:10.21037/tgh.2016.01.07
Lou, G., Chen, L., Xia, C., Wang, W., Qi, J., Li, A., et al. (2020). MiR-199a-modified exosomes from adipose tissue-derived mesenchymal stem cells improve hepatocellular carcinoma chemosensitivity through mTOR pathway. J. Exp. Clin. Cancer Res. 39, 4–9. doi:10.1186/s13046-019-1512-5
Lu, Z., Huang, Y., Huang, J., Ni, H. H., Luo, T., Wei, X., et al. (2023). High platelet count is a potential prognostic factor of the early recurrence of hepatocellular carcinoma in the presence of circulating tumor cells. J. Hepatocell. Carcinoma 10, 57–68. doi:10.2147/JHC.S398591
Luan, Y., Li, X., Luan, Y., Zhao, R., Li, Y., Liu, L., et al. (2020). Circulating lncRNA UCA1 promotes malignancy of colorectal cancer via the miR-143/MYO6 Axis. Nucleic Acids 19, 790–803. doi:10.1016/j.omtn.2019.12.009
Luo, C.-L., Xu, Z.-G., Chen, H., Ji, J., Wang, Y.-H., Hu, W., et al. (2018a). LncRNAs and EGFRvIII sequestered in TEPs enable blood-based NSCLC diagnosis. Cancer Manag. Res. 10, 1449–1459. doi:10.2147/CMAR.S164227
Luo, L., Peng, B., Jiang, Z., He, G., Cheng, Y., Gao, Y., et al. (2018b). Correlative factors of the deterioration of necrotizing enterocolitis in small for gestational age newborns. Chin. J. Hepatobiliary Surg. 8, 13–17. doi:10.1038/s41598-017-18467-8
Lyskjær, I., Kronborg, C. S., Rasmussen, M. H., Sørensen, B. S., Demuth, C., Rosenkilde, M., et al. (2019). Correlation between early dynamics in circulating tumour DNA and outcome from FOLFIRI treatment in metastatic colorectal cancer. Sci. Rep. 9 (1), 11542. doi:10.1038/s41598-019-47708-1
Lyu, X., Tsui, Y.-M., Ho, D. W.-H., and Ng, I. O.-L. (2022). Liquid biopsy using cell-free or circulating tumor DNA in the management of hepatocellular carcinoma. Cell. Mol. Gastroenterology Hepatology 13 (6), 1611–1624. doi:10.1016/j.jcmgh.2022.02.008
Ma, X., Shao, Y., Tian, L., Flasch, D. A., Mulder, H. L., Edmonson, M. N., et al. (2019). Analysis of error profiles in deep next-generation sequencing data. Genome Biol. 20 (1), 50. doi:10.1186/s13059-019-1659-6
Mai, S., and Inkielewicz-Stepniak, I. (2021). Pancreatic cancer and platelets crosstalk: a potential biomarker and target. Front. Cell Dev. Biol. 9, 749689. doi:10.3389/fcell.2021.749689
Mandel, P., and Metais, P. (1948). Nuclear acids in human blood plasma. Comptes Rendus Des Seances de La Soc. de Biol. de Ses Fil. 142 (3–4), 241–243.
Martini, V., Timme-Bronsert, S., Fichtner-Feigl, S., Hoeppner, J., and Kulemann, B. (2019). Circulating tumor cells in pancreatic cancer: current perspectives. Cancers 11 (11), 1659. doi:10.3390/cancers11111659
Mattick, J. S., Amaral, P. P., Carninci, P., Carpenter, S., Chang, H. Y., Chen, L.-L., et al. (2023). Long non-coding RNAs: definitions, functions, challenges and recommendations. Nat. Rev. Mol. Cell Biol. 24 (6), 430–447. doi:10.1038/s41580-022-00566-8
Mendoza-Almanza, G., Burciaga-Hernández, L., Maldonado, V., Melendez-Zajgla, J., and Olmos, J. (2020). Role of platelets and breast cancer stem cells in metastasis. World J. Stem Cells 12 (11), 1237–1254. doi:10.4252/wjsc.v12.i11.1237
Meng, Y., Sun, J., Zheng, Y., Zhang, G., Yu, T., and Piao, H. (2021). Platelets: the emerging clinical diagnostics and therapy selection of cancer liquid biopsies. OncoTargets Ther. 14, 3417–3428. doi:10.2147/OTT.S311907
Mino-Kenudson, M. (2016). Cons: can liquid biopsy replace tissue biopsy? the US experience. Transl. lung cancer Res. 5 (Issue 4), 424–427. doi:10.21037/tlcr.2016.08.01
Mo, S., Ye, L., Wang, D., Han, L., Zhou, S., Wang, H., et al. (2023). Early detection of molecular residual disease and risk stratification for stage I to III colorectal cancer via circulating tumor DNA methylation. JAMA Oncol. 9 (6), 770–778. doi:10.1001/jamaoncol.2023.0425
Mondal, D., Shinde, S., Paul, S., Thakur, S., Velu, G. S. K., Tiwari, A. K., et al. (2023). Diagnostic significance of dysregulated miRNAs in T-cell malignancies and their metabolic roles. Front. Oncol. 13, 1230273. doi:10.3389/fonc.2023.1230273
Mu, H., Lin, K., Zhao, H., Li, C., Sun, Y., Cai, J., et al. (2014). Detection of circulating tumor cells in patients with hepatocellular carcinoma. Zhonghua Zhong Liu Za Zhi Chin. J. Oncol. 36 (4), 276–281.
Mysona, D., Pyrzak, A., Purohit, S., Zhi, W., Sharma, A., Tran, L., et al. (2019). A combined score of clinical factors and serum proteins can predict time to recurrence in high grade serous ovarian cancer. Gynecol. Oncol. 152 (3), 574–580. doi:10.1016/j.ygyno.2018.12.015
Nicolazzo, C., Raimondi, C., Gradilone, A., Emiliani, A., Zeuner, A., Francescangeli, F., et al. (2019). Circulating tumor cells in right- and left-sided colorectal cancer. Cancers 11 (8), 1042. doi:10.3390/cancers11081042
Nikanjam, M., Kato, S., and Kurzrock, R. (2022). Liquid biopsy: current technology and clinical applications. J. Hematol. Oncol. 15 (1), 131. doi:10.1186/s13045-022-01351-y
Nilsson, R. J. A., Balaj, L., Hulleman, E., van Rijn, S., Pegtel, D. M., Walraven, M., et al. (2011). Blood platelets contain tumor-derived RNA biomarkers. Blood 118 (13), 3680–3683. doi:10.1182/blood-2011-03-344408
Nishiwada, S., Cui, Y., Sho, M., Jun, E., Akahori, T., Nakamura, K., et al. (2022). Transcriptomic profiling identifies an exosomal microRNA signature for predicting recurrence following surgery in patients with pancreatic ductal adenocarcinoma. Ann. Surg. 276 (6), e876–e885. doi:10.1097/SLA.0000000000004993
Normanno, N., Esposito Abate, R., Lambiase, M., Forgione, L., Cardone, C., Iannaccone, A., et al. (2018). RAS testing of liquid biopsy correlates with the outcome of metastatic colorectal cancer patients treated with first-line FOLFIRI plus cetuximab in the CAPRI-GOIM trial. Ann. Oncol. 29 (1), 112–118. doi:10.1093/annonc/mdx417
Obermeier, H. L., Riedl, J., Ay, C., Koder, S., Quehenberger, P., Bartsch, R., et al. (2019). The role of ADAMTS-13 and von Willebrand factor in cancer patients: Results from the Vienna Cancer and Thrombosis Study. Res. Pract. Thrombosis Haemostasis 3 (3), 503–514. doi:10.1002/rth2.12197
O’Brien, J., Hayder, H., Zayed, Y., and Peng, C. (2018). Overview of MicroRNA biogenesis, mechanisms of actions, and circulation. Front. Endocrinol. 9, 402. doi:10.3389/fendo.2018.00402
Oehme, F., Krahl, S., Gyorffy, B., Muessle, B., Rao, V., Greif, H., et al. (2019). Low level of exosomal long non-coding RNA HOTTIP is a prognostic biomarker in colorectal cancer. RNA Biol. 16 (10), 1339–1345. doi:10.1080/15476286.2019.1637697
Ogata-Kawata, H., Izumiya, M., Kurioka, D., Honma, Y., Yamada, Y., Furuta, K., et al. (2014). Circulating exosomal microRNAs as biomarkers of colon cancer. PloS One 9 (4), e92921. doi:10.1371/journal.pone.0092921
Osaki, M., and Okada, F. (2019). Exosomes and their role in cancer progression. Yonago Acta Medica 62 (2), 182–190. doi:10.33160/yam.2019.06.002
Padilla-Ruiz, M., Morales-Suárez-Varela, M., Rivas-Ruiz, F., Alcaide, J., Varela-Moreno, E., Zarcos-Pedrinaci, I., et al. (2022). Influence of diagnostic delay on survival rates for patients with colorectal cancer. Int. J. Environ. Res. Public Health 19 (6), 3626. doi:10.3390/ijerph19063626
Pan, B., Qin, J., Liu, X., He, B., Wang, X., Pan, Y., et al. (2019). Identification of serum exosomal hsa-circ-0004771 as a novel diagnostic biomarker of colorectal cancer. Front. Genet. 10, 1096. doi:10.3389/fgene.2019.01096
Parikh, A. R., Van Seventer, E. E., Siravegna, G., Hartwig, A. V., Jaimovich, A., He, Y., et al. (2021). Minimal residual disease detection using a plasma-only circulating tumor DNA assay in patients with colorectal cancer. Clin. Cancer Res. An Official J. Am. Assoc. Cancer Res. 27 (20), 5586–5594. doi:10.1158/1078-0432.CCR-21-0410
Park, Y., Jun, H. R., Choi, H. W., Hwang, D. W., Lee, J. H., Song, K. B., et al. (2021). Circulating tumour cells as an indicator of early and systemic recurrence after surgical resection in pancreatic ductal adenocarcinoma. Sci. Rep. 11 (1), 1644. doi:10.1038/s41598-020-80383-1
Paskeh, M. D. A., Entezari, M., Mirzaei, S., Zabolian, A., Saleki, H., Naghdi, M. J., et al. (2022). Emerging role of exosomes in cancer progression and tumor microenvironment remodeling. J. Hematol. Oncol. 15 (1), 83. doi:10.1186/s13045-022-01305-4
Pécuchet, N., Zonta, E., Didelot, A., Combe, P., Thibault, C., Gibault, L., et al. (2016). Base-position error rate analysis of next-generation sequencing applied to circulating tumor DNA in non-small cell lung cancer: a prospective study. PLoS Med. 13 (12), e1002199. doi:10.1371/journal.pmed.1002199
Peng, Y., and Croce, C. M. (2016). The role of MicroRNAs in human cancer. Signal Transduct. Target. Ther. 1 (1), 15004. doi:10.1038/sigtrans.2015.4
Peterson, J. E., Zurakowski, D., Italiano, J. E. J., Michel, L. V., Connors, S., Oenick, M., et al. (2012). VEGF, PF4 and PDGF are elevated in platelets of colorectal cancer patients. Angiogenesis 15 (2), 265–273. doi:10.1007/s10456-012-9259-z
Petrik, J., Verbanac, D., Fabijanec, M., Hulina-Tomašković, A., Čeri, A., Somborac-Bačura, A., et al. (2022). Circulating tumor cells in colorectal cancer: detection systems and clinical utility. Int. J. Mol. Sci. 23 (21), 13582. doi:10.3390/ijms232113582
Plantureux, L., Crescence, L., Dignat-George, F., Panicot-Dubois, L., and Dubois, C. (2018). Effects of platelets on cancer progression. Thrombosis Res. 164, S40–S47. doi:10.1016/j.thromres.2018.01.035
Poruk, K. E., Blackford, A. L., Weiss, M. J., Cameron, J. L., He, J., Goggins, M., et al. (2017). Circulating tumor cells expressing markers of tumor-initiating cells predict poor survival and cancer recurrence in patients with pancreatic ductal adenocarcinoma. Clin. Cancer Res. An Official J. Am. Assoc. Cancer Res. 23 (11), 2681–2690. doi:10.1158/1078-0432.CCR-16-1467
Preethi, K. A., Selvakumar, S. C., Ross, K., Jayaraman, S., Tusubira, D., and Sekar, D. (2022). Liquid biopsy: exosomal microRNAs as novel diagnostic and prognostic biomarkers in cancer. Mol. Cancer 21 (1), 54. doi:10.1186/s12943-022-01525-9
Pu, W., Xiao, L., Zhou, C., Zhong, F., Wu, Y., Gong, W., et al. (2021). Cancer stage-dependent alterations in cell-free DNA in patients with colorectal cancer. J. B.U.ON, Official J. Balkan Union Oncol. 26 (1), 109–115.
Raez, L. E., Brice, K., Dumais, K., Lopez-Cohen, A., Wietecha, D., Izquierdo, P. A., et al. (2023). Liquid biopsy versus tissue biopsy to determine front line therapy in metastatic non-small cell lung cancer (NSCLC). Clin. Lung Cancer 24 (2), 120–129. doi:10.1016/j.cllc.2022.11.007
Ranucci, R. (2019). Cell-free DNA: applications in different diseases. Methods Mol. Biol. Clift. N.J. 1909, 3–12. doi:10.1007/978-1-4939-8973-7_1
Ratnasari, N., Lestari, P., Renovaldi, D., Raditya Ningsih, J., Qoriansas, N., Wardana, T., et al. (2022). Potential plasma biomarkers: miRNA-29c, miRNA-21, and miRNA-155 in clinical progression of Hepatocellular Carcinoma patients. PloS One 17 (2), e0263298. doi:10.1371/journal.pone.0263298
Rattanasupar, A., Chartleeraha, S., Akarapatima, K., and Chang, A. (2021). Factors that affect the surveillance and late-stage detection of a newly diagnosed hepatocellular carcinoma. Asian Pac. J. Cancer Prev. APJCP 22 (10), 3293–3298. doi:10.31557/APJCP.2021.22.10.3293
Rau, K.-M., Liu, C.-T., Hsiao, Y.-C., Hsiao, K.-Y., Wang, T.-M., Hung, W.-S., et al. (2020). Sequential circulating tumor cell counts in patients with locally advanced or metastatic hepatocellular carcinoma: monitoring the treatment response. J. Clin. Med. 9 (Issue 1), 188. doi:10.3390/jcm9010188
Ren, J., Ding, L., Zhang, D., Shi, G., Xu, Q., Shen, S., et al. (2018). Carcinoma-associated fibroblasts promote the stemness and chemoresistance of colorectal cancer by transferring exosomal lncRNA H19. Theranostics 8 (14), 3932–3948. doi:10.7150/thno.25541
Ren, J., Lu, P., Zhou, X., Liao, Y., Liu, X., Li, J., et al. (2022). Genome-scale methylation analysis of circulating cell-free DNA in gastric cancer patients. Clin. Chem. 68 (2), 354–364. doi:10.1093/clinchem/hvab204
Ried, K., Eng, P., and Sali, A. (2017). Screening for circulating tumour cells allows early detection of cancer and monitoring of treatment effectiveness: an observational study. Asian Pac. J. Cancer Prev. APJCP 18 (8), 2275–2285. doi:10.22034/APJCP.2017.18.8.2275
Roshani, M., Baniebrahimi, G., Mousavi, M., Zare, N., Sadeghi, R., Salarinia, R., et al. (2022). Exosomal long non-coding RNAs: novel molecules in gastrointestinal cancers’ progression and diagnosis. Front. Oncol. 12, 1014949. doi:10.3389/fonc.2022.1014949
Rumiato, E., Boldrin, E., Malacrida, S., Realdon, S., Fassan, M., Morbin, T., et al. (2017). Detection of genetic alterations in cfDNA as a possible strategy to monitor the neoplastic progression of Barrett’s esophagus. Transl. Res. 190, 16–24. doi:10.1016/j.trsl.2017.09.004
Sabrkhany, S., Kuijpers, M. J. E., van Kuijk, S. M. J., Sanders, L., Pineda, S., Olde Damink, S. W. M., et al. (2017). A combination of platelet features allows detection of early-stage cancer. Eur. J. Cancer (Oxford, Engl. 1990) 80, 5–13. doi:10.1016/j.ejca.2017.04.010
Sai, S., Ichikawa, D., Tomita, H., Ikoma, D., Tani, N., Ikoma, H., et al. (2007). Quantification of plasma cell-free DNA in patients with gastric cancer. Anticancer Res. 27 (4C), 2747–2751.
Saliminejad, K., Soleymani Fard, S., Khorram Khorshid, H. R., Yaghmaie, M., Mahmoodzadeh, H., Mousavi, S. A., et al. (2020). Methylation analysis of P16, RASSF1A, RPRM, and RUNX3 in circulating cell-free DNA for detection of gastric cancer: a validation study. Avicenna J. Med. Biotechnol. 12 (2), 99–106. doi:10.18502/ajmb.v14i4.10482
Sastre, J., Maestro, M. L., Puente, J., Veganzones, S., Alfonso, R., Rafael, S., et al. (2008). Circulating tumor cells in colorectal cancer: correlation with clinical and pathological variables. Ann. Oncol. Official J. Eur. Soc. Med. Oncol. 19 (5), 935–938. doi:10.1093/annonc/mdm583
Sathishkumar, K., Chaturvedi, M., Das, P., Stephen, S., and Mathur, P. (2022). Cancer incidence estimates for 2022 and projection for 2025: result from national cancer registry programme, India. Indian J. Med. Res. 156 (4and5), 598–607. doi:10.4103/ijmr.ijmr_1821_22
Schrecker, C., Waidmann, O., El Youzouri, H., Trojan, J., Schnitzbauer, A. A., Bechstein, W. O., et al. (2022). Low platelet count predicts reduced survival in potentially resectable hepatocellular carcinoma. Curr. Oncol. Tor. Ont. 29 (3), 1475–1487. doi:10.3390/curroncol29030124
Shegekar, T., Vodithala, S., and Juganavar, A. (2023). The emerging role of liquid biopsies in revolutionising cancer diagnosis and therapy. Cureus 15 (8), e43650. doi:10.7759/cureus.43650
Sheng, M., Dong, Z., and Xie, Y. (2018). Identification of tumor-educated platelet biomarkers of non-small-cell lung cancer. OncoTargets Ther. 11, 8143–8151. doi:10.2147/OTT.S177384
Shi, J., Li, Y., Liang, S., Zeng, J., Liu, G., Mu, F., et al. (2016). Analysis of circulating tumor cells in colorectal cancer liver metastasis patients before and after cryosurgery. Cancer Biol. Ther. 17 (9), 935–942. doi:10.1080/15384047.2016.1210731
Shoda, K., Ichikawa, D., Fujita, Y., Masuda, K., Hiramoto, H., Hamada, J., et al. (2017). Clinical utility of circulating cell-free Epstein-Barr virus DNA in patients with gastric cancer. Oncotarget 8 (17), 28796–28804. doi:10.18632/oncotarget.15675
Siddiqui, M. T. H., Khan, M. R., Jawaid, A., Shaukat, F., and Zahid, N. (2023). Evaluation of quality of life of gastrointestinal cancer patients presenting to a tertiary care hospital in Pakistan. Ecancermedicalscience 17, 1527. doi:10.3332/ecancer.2023.1527
Sidransky, D., Tokino, T., Hamilton, S. R., Kinzler, K. W., Levin, B., Frost, P., et al. (1992). Identification of ras oncogene mutations in the stool of patients with curable colorectal tumors. Sci. (New York, N.Y.) 256 (5053), 102–105. doi:10.1126/science.1566048
Siegel, R. L., Miller, K. D., Wagle, N. S., and Jemal, A. (2023a). Cancer statistics, 2023. CA A Cancer J. Clin. 73 (1), 17–48. doi:10.3322/caac.21763
Siegel, R. L., Wagle, N. S., Cercek, A., Smith, R. A., and Jemal, A. (2023b). Colorectal cancer statistics, 2023. CA A Cancer J. Clin. 73 (3), 233–254. doi:10.3322/caac.21772
Sivapalan, L., Murray, J. C., Canzoniero, J. V., Landon, B., Jackson, J., Scott, S., et al. (2023). Liquid biopsy approaches to capture tumor evolution and clinical outcomes during cancer immunotherapy. J. Immunother. Cancer 11 (1), e005924. doi:10.1136/jitc-2022-005924
Snyder, M. W., Kircher, M., Hill, A. J., Daza, R. M., and Shendure, J. (2016). Cell-free DNA comprises an in vivo nucleosome footprint that informs its tissues-of-origin. Cell 164 (1), 57–68. doi:10.1016/j.cell.2015.11.050
Soeda, N., Iinuma, H., Suzuki, Y., Tsukahara, D., Midorikawa, H., Igarashi, Y., et al. (2019). Plasma exosome-encapsulated microRNA-21 and microRNA-92a are promising biomarkers for the prediction of peritoneal recurrence in patients with gastric cancer. Oncol. Lett. 18 (5), 4467–4480. doi:10.3892/ol.2019.10807
Sol, N., Gjg, S., Vancura, A., Tjerkstra, M., Leurs, C., Rustenburg, F., et al. (2020). Tumor-educated platelet RNA for the detection and (pseudo) progression monitoring of glioblastoma. Cell Rep. Med. 1 (7), 100101. doi:10.1016/j.xcrm.2020.100101
Song, B. G., Kwon, W., Kim, H., Lee, E. M., Han, Y. M., Kim, H., et al. (2021). Detection of circulating tumor cells in resectable pancreatic ductal adenocarcinoma: a prospective evaluation as a prognostic marker. Front. Oncol. 10, 616440. doi:10.3389/fonc.2020.616440
Sozzi, G., Conte, D., Leon, M., Ciricione, R., Roz, L., Ratcliffe, C., et al. (2003). Quantification of free circulating DNA as a diagnostic marker in lung cancer. J. Clin. Oncol. Official J. Am. Soc. Clin. Oncol. 21 (21), 3902–3908. doi:10.1200/JCO.2003.02.006
Sozzi, G., Conte, D., Mariani, L., Lo Vullo, S., Roz, L., Lombardo, C., et al. (2001). Analysis of circulating tumor DNA in plasma at diagnosis and during follow-up of lung cancer patients. Cancer Res. 61 (12), 4675–4678.
Stejskal, P., Goodarzi, H., Srovnal, J., Hajdúch, M., van ’t Veer, L. J., and Magbanua, M. J. M. (2023). Circulating tumor nucleic acids: biology, release mechanisms, and clinical relevance. Mol. Cancer 22 (1), 15. doi:10.1186/s12943-022-01710-w
Su, K., Guo, L., He, K., Rao, M., Zhang, J., Yang, X., et al. (2022). PD-L1 expression on circulating tumor cells can be a predictive biomarker to PD-1 inhibitors combined with radiotherapy and antiangiogenic therapy in advanced hepatocellular carcinoma. Front. oncol. 12, 873830. doi:10.3389/fonc.2022.873830
Sun, B., Li, Y., Zhou, Y., Ng, T. K., Zhao, C., Gan, Q., et al. (2019). Circulating exosomal CPNE3 as a diagnostic and prognostic biomarker for colorectal cancer. J. Cell. Physiology 234 (2), 1416–1425. doi:10.1002/jcp.26936
Sun, Y.-F., Wang, P.-X., Cheng, J.-W., Gong, Z.-J., Huang, A., Zhou, K.-Q., et al. (2020). Postoperative circulating tumor cells: an early predictor of extrahepatic metastases in patients with hepatocellular carcinoma undergoing curative surgical resection. Cancer Cytopathol. 128 (10), 733–745. doi:10.1002/cncy.22304
Sunakawa, Y., Satake, H., Usher, J., Jaimes, Y., Miyamoto, Y., Nakamura, M., et al. (2022). Dynamic changes in RAS gene status in circulating tumour DNA: a phase II trial of first-line FOLFOXIRI plus bevacizumab for RAS-mutant metastatic colorectal cancer (JACCRO CC-11). ESMO open 7 (3), 100512. doi:10.1016/j.esmoop.2022.100512
Swarup, N., Cheng, J., Choi, I., Heo, Y. J., Kordi, M., Aziz, M., et al. (2023). Multi-faceted attributes of salivary cell-free DNA as liquid biopsy biomarkers for gastric cancer detection. Biomark. Res. 11 (1), 90. doi:10.1186/s40364-023-00524-2
Tabaeian, S. P., Eshkiki, Z. S., Dana, F., Fayyaz, F., Baniasadi, M., Agah, S., et al. (2024). Evaluation of tumor-educated platelet long non-coding RNAs (lncRNAs) as potential diagnostic biomarkers for colorectal cancer. J. Cancer Res. Ther. 24, 1212–1222. doi:10.4103/jcrt.jcrt_1212_22
Tang, Y., Zhao, Y., Song, X., Song, X., Niu, L., and Xie, L. (2019). Tumor-derived exosomal miRNA-320d as a biomarker for metastatic colorectal cancer. J. Clin. Laboratory Analysis 33 (9), e23004. doi:10.1002/jcla.23004
Thierry, A. R., Mouliere, F., El Messaoudi, S., Mollevi, C., Lopez-Crapez, E., Rolet, F., et al. (2014). Clinical validation of the detection of KRAS and BRAF mutations from circulating tumor DNA. Nat. Med. 20 (4), 430–435. doi:10.1038/nm.3511
Tjon-Kon-Fat, L., Lundholm, M., Schröder, M., Wurdinger, T., Thellenberg-Karlsson, C., Widmark, A., et al. (2018). Platelets harbor prostate cancer biomarkers and the ability to predict therapeutic response to abiraterone in castration resistant patients. Prostate 78 (1), 48–53. doi:10.1002/pros.23443
Tokuhisa, Y., Iizuka, N., Sakaida, I., Moribe, T., Fujita, N., Miura, T., et al. (2007). Circulating cell-free DNA as a predictive marker for distant metastasis of hepatitis C virus-related hepatocellular carcinoma. Br. J. Cancer 97 (10), 1399–1403. doi:10.1038/sj.bjc.6604034
Unseld, M., Belic, J., Pierer, K., Zhou, Q., Moser, T., Bauer, R., et al. (2021). A higher ctDNA fraction decreases survival in regorafenib-treated metastatic colorectal cancer patients. Results from the regorafenib’s liquid biopsy translational biomarker phase II pilot study. Int. J. Cancer Res. 148 (6), 1452–1461. doi:10.1002/ijc.33303
Uratani, R., Toiyama, Y., Kitajima, T., Kawamura, M., Hiro, J., Kobayashi, M., et al. (2016). Diagnostic potential of cell-free and exosomal MicroRNAs in the identification of patients with high-risk colorectal adenomas. PloS One 11 (10), e0160722. doi:10.1371/journal.pone.0160722
Vacante, M., Ciuni, R., Basile, F., and Biondi, A. (2020). The liquid biopsy in the management of colorectal cancer: an overview. Biomedicines 8 (9), 308. doi:10.3390/biomedicines8090308
van der Meijden, P. E. J., and Heemskerk, J. W. M. (2019). Platelet biology and functions: new concepts and clinical perspectives. Nat. Rev. Cardiol. 16 (3), 166–179. doi:10.1038/s41569-018-0110-0
van ’t Erve, I., Medina, J. E., Leal, A., Papp, E., Phallen, J., Adleff, V., et al. (2023). Metastatic colorectal cancer treatment response evaluation by ultra-deep sequencing of cell-free dna and matched white blood cells. Clin. Cancer Res. 29 (5), 899–909. doi:10.1158/1078-0432.CCR-22-2538
Varkalaite, G., Forster, M., Franke, A., Kupcinskas, J., and Skieceviciene, J. (2021). Liquid biopsy in gastric cancer: analysis of somatic cancer tissue mutations in plasma cell-free DNA for predicting disease state and patient survival. Clin. Transl. Gastroenterology 12 (9), e00403. doi:10.14309/ctg.0000000000000403
Vidal, J., Muinelo, L., Dalmases, A., Jones, F., Edelstein, D., Iglesias, M., et al. (2017). Plasma ctDNA RAS mutation analysis for the diagnosis and treatment monitoring of metastatic colorectal cancer patients. Ann. Oncol. 28 (6), 1325–1332. doi:10.1093/annonc/mdx125
Walker, N. J., Rashid, M., Yu, S., Bignell, H., Lumby, C. K., Livi, C. M., et al. (2022). Hydroxymethylation profile of cell-free DNA is a biomarker for early colorectal cancer. Sci. Rep. 12 (1), 16566. doi:10.1038/s41598-022-20975-1
Wang, C., Wang, J., Cui, W., Liu, Y., Zhou, H., Wang, Y., et al. (2021a). Serum exosomal miRNA-1226 as potential biomarker of pancreatic ductal adenocarcinoma. OncoTargets Ther. 14, 1441–1451. doi:10.2147/OTT.S296816
Wang, F.-W., Cao, C.-H., Han, K., Zhao, Y.-X., Cai, M.-Y., Xiang, Z.-C., et al. (2021b). APC-activated long noncoding RNA inhibits colorectal carcinoma pathogenesis through reduction of exosome production. J. Clin. Investigation 131 (7), e149666. doi:10.1172/JCI149666
Wang, H., Wei, X., Wu, B., Su, J., Tan, W., and Yang, K. (2019a). Tumor-educated platelet miR-34c-3p and miR-18a-5p as potential liquid biopsy biomarkers for nasopharyngeal carcinoma diagnosis. Cancer Manag. Res. 11, 3351–3360. doi:10.2147/CMAR.S195654
Wang, J., Yan, F., Zhao, Q., Zhan, F., Wang, R., Wang, L., et al. (2017). Circulating exosomal miR-125a-3p as a novel biomarker for early-stage colon cancer. Sci. Rep. 7 (1), 4150. doi:10.1038/s41598-017-04386-1
Wang, J., Yang, L., Diao, Y., Liu, J., Li, J., Li, R., et al. (2021c). Circulating tumour DNA methylation in hepatocellular carcinoma diagnosis using digital droplet PCR. J. Int. Med. Res. 49 (3), 300060521992962. doi:10.1177/0300060521992962
Wang, J.-Y., Hsieh, J.-S., Chang, M.-Y., Huang, T.-J., Chen, F.-M., Cheng, T.-L., et al. (2004). Molecular detection of APC, K- ras, and p53 mutations in the serum of colorectal cancer patients as circulating biomarkers. World J. Surg. 28 (7), 721–726. doi:10.1007/s00268-004-7366-8
Wang, L., Duan, W., Yan, S., Xie, Y., and Wang, C. (2019b). Circulating long non-coding RNA colon cancer-associated transcript 2 protected by exosome as a potential biomarker for colorectal cancer. Biomed. Pharmacother. = Biomedecine Pharmacother. 113, 108758. doi:10.1016/j.biopha.2019.108758
Wang, L., Zhou, S., Zhang, W., Wang, J., Wang, M., Hu, X., et al. (2019c). Circulating tumor cells as an independent prognostic factor in advanced colorectal cancer: a retrospective study in 121 patients. Int. J. Colorectal Dis. 34 (4), 589–597. doi:10.1007/s00384-018-03223-9
Wang, P., Song, Q., Ren, J., Zhang, W., Wang, Y., Zhou, L., et al. (2022a). Simultaneous analysis of mutations and methylations in circulating cell-free DNA for hepatocellular carcinoma detection. Sci. Transl. Med. 14 (672), eabp8704. doi:10.1126/scitranslmed.abp8704
Wang, S., Qiu, Y., and Bai, B. (2019d). The expression, regulation, and biomarker potential of glypican-1 in cancer. Front. Oncol. 9, 614. doi:10.3389/fonc.2019.00614
Wang, W., Wan, L., Wu, S., Yang, J., Zhou, Y., Liu, F., et al. (2018). Mesenchymal marker and LGR5 expression levels in circulating tumor cells correlate with colorectal cancer prognosis. Cell. Oncol. Dordr. 41 (5), 495–504. doi:10.1007/s13402-018-0386-4
Wang, X., Huang, J., Chen, W., Li, G., Li, Z., and Lei, J. (2022b). The updated role of exosomal proteins in the diagnosis, prognosis, and treatment of cancer. Exp. Mol. Med. 54 (9), 1390–1400. doi:10.1038/s12276-022-00855-4
Wang, Y., Liu, J., Ma, J., Sun, T., Zhou, Q., Wang, W., et al. (2019e). Exosomal circRNAs: biogenesis, effect and application in human diseases. Mol. Cancer 18 (1), 116. doi:10.1186/s12943-019-1041-z
Wang, Y., Yu, X., Hartmann, D., and Zhou, J. (2020). Circulating tumor cells in peripheral blood of pancreatic cancer patients and their prognostic role: a systematic review and meta-analysis. HPB Official J. Int. Hepato Pancreato Biliary Assoc. 22 (5), 660–669. doi:10.1016/j.hpb.2019.11.003
Waqar, W., Asghar, S., and Manzoor, S. (2021). Platelets’ RNA as biomarker trove for differentiation of early-stage hepatocellular carcinoma from underlying cirrhotic nodules. PloS One 16 (9), e0256739. doi:10.1371/journal.pone.0256739
Watanabe, F., Suzuki, K., Aizawa, H., Endo, Y., Takayama, Y., Kakizawa, N., et al. (2023). Circulating tumor DNA in molecular assessment feasibly predicts early progression of pancreatic cancer that cannot be identified via initial imaging. Sci. Rep. 13 (1), 4809. doi:10.1038/s41598-023-31051-7
Wei, T., Zhang, X., Zhang, Q., Yang, J., Chen, Q., Wang, J., et al. (2019). Vimentin-positive circulating tumor cells as a biomarker for diagnosis and treatment monitoring in patients with pancreatic cancer. Cancer Lett. 452, 237–243. doi:10.1016/j.canlet.2019.03.009
Welinder, C., Jansson, B., Lindell, G., and Wenner, J. (2015). Cytokeratin 20 improves the detection of circulating tumor cells in patients with colorectal cancer. Cancer Lett. 358 (1), 43–46. doi:10.1016/j.canlet.2014.12.024
Wen, L., Li, J., Guo, H., Liu, X., Zheng, S., Zhang, D., et al. (2015). Genome-scale detection of hypermethylated CpG islands in circulating cell-free DNA of hepatocellular carcinoma patients. Cell Res. 25 (11), 1250–1264. doi:10.1038/cr.2015.126
Wu, X., Zhang, Y., Hu, T., He, X., Zou, Y., Deng, Q., et al. (2021). A novel cell-free DNA methylation-based model improves the early detection of colorectal cancer. Mol. Oncol. 15 (10), 2702–2714. doi:10.1002/1878-0261.12942
Wu, Z., Yu, L., Hou, J., Cui, L., Huang, Y., Chen, Q., et al. (2022). Plasma cfDNA for the diagnosis and prognosis of colorectal cancer. J. Oncol. 2022, 9538384. doi:10.1155/2022/9538384
Xing, S., Zeng, T., Xue, N., He, Y., Lai, Y., Li, H., et al. (2019). Development and validation of tumor-educated blood platelets integrin alpha 2b (ITGA2B) RNA for diagnosis and prognosis of non-small-cell lung cancer through RNA-seq. Int. J. Biol. Sci. 15 (9), 1977–1992. doi:10.7150/ijbs.36284
Xu, J., Xiao, Y., Liu, B., Pan, S., Liu, Q., Shan, Y., et al. (2020a). Exosomal MALAT1 sponges miR-26a/26b to promote the invasion and metastasis of colorectal cancer via FUT4 enhanced fucosylation and PI3K/Akt pathway. J. Exp. Clin. Cancer Res. CR 39 (1), 54. doi:10.1186/s13046-020-01562-6
Xu, L., Li, X., Li, X., Wang, X., Ma, Q., She, D., et al. (2022). RNA profiling of blood platelets noninvasively differentiates colorectal cancer from healthy donors and noncancerous intestinal diseases: a retrospective cohort study. Genome Med. 14 (1), 26. doi:10.1186/s13073-022-01033-x
Xu, X. R., Yousef, G. M., and Ni, H. (2018). Cancer and platelet crosstalk: opportunities and challenges for aspirin and other antiplatelet agents. Blood 131 (16), 1777–1789. doi:10.1182/blood-2017-05-743187
Xu, Y., Han, J., Zhang, X., Zhang, X., Song, J., Gao, Z., et al. (2024). Exosomal circRNAs in gastrointestinal cancer: role in occurrence, development, diagnosis and clinical application (Review). Oncol. Rep. 51 (2), 19. doi:10.3892/or.2023.8678
Xu, Y., Kong, S., Qin, S., Shen, X., and Ju, S. (2020b). Exosomal circRNAs: sorting mechanisms, roles and clinical applications in tumors. Front. Cell Dev. Biol. 8, 581558. doi:10.3389/fcell.2020.581558
Xu, Y., Qiu, A., Peng, F., Tan, X., Wang, J., and Gong, X. (2021). Exosomal transfer of circular RNA FBXW7 ameliorates the chemoresistance to oxaliplatin in colorectal cancer by sponging miR-18b-5p. Neoplasma 68 (1), 108–118. doi:10.4149/neo_2020_200417N414
Xue, L., Xie, L., Song, X., and Song, X. (2018). Identification of potential tumor-educated platelets RNA biomarkers in non-small-cell lung cancer by integrated bioinformatical analysis. J. Clin. Laboratory Analysis 32 (7), e22450. doi:10.1002/jcla.22450
Yagyu, T., Saito, H., Sakamoto, T., Uchinaka, E., Morimoto, M., Hanaki, T., et al. (2021). Decreased mean platelet volume predicts poor prognosis in patients with pancreatic cancer. BMC Surg. 21 (1), 8. doi:10.1186/s12893-020-00976-5
Yang, B., Feng, X., Liu, H., Tong, R., Wu, J., Li, C., et al. (2020). High-metastatic cancer cells derived exosomal miR92a-3p promotes epithelial-mesenchymal transition and metastasis of low-metastatic cancer cells by regulating PTEN/Akt pathway in hepatocellular carcinoma. Oncogene 39 (42), 6529–6543. doi:10.1038/s41388-020-01450-5
Yang, C., Chen, F., Wang, S., and Xiong, B. (2019a). Circulating tumor cells in gastrointestinal cancers: current status and future perspectives. Front. Oncol. 9, 1427. doi:10.3389/fonc.2019.01427
Yang, C., Shi, D., Wang, S., Wei, C., Zhang, C., and Xiong, B. (2018). Prognostic value of pre- and post-operative circulating tumor cells detection in colorectal cancer patients treated with curative resection: a prospective cohort study based on ISET device. Cancer Manag. Res. 10, 4135–4144. doi:10.2147/CMAR.S176575
Yang, L., Jiang, Q., Li, D.-Z., Zhou, X., Yu, D.-S., and Zhong, J. (2019b). TIMP1 mRNA in tumor-educated platelets is diagnostic biomarker for colorectal cancer. Aging (Albany NY) 11 (20), 8998–9012. doi:10.18632/aging.102366
Yang, Y.-J., Chen, H., Huang, P., Li, C.-H., Dong, Z.-H., and Hou, Y.-L. (2011). Quantification of plasma hTERT DNA in hepatocellular carcinoma patients by quantitative fluorescent polymerase chain reaction. Clin. Investigative Med. 34 (4), E238. doi:10.25011/cim.v34i4.15366
Yao, B., Qu, S., Hu, R., Gao, W., Jin, S., Ju, J., et al. (2019). Delivery of platelet TPM3 mRNA into breast cancer cells via microvesicles enhances metastasis. FEBS Open Bio 9 (12), 2159–2169. doi:10.1002/2211-5463.12759
Ye, B., Li, F., Chen, M., Weng, Y., Qi, C., Xie, Y., et al. (2022). A panel of platelet-associated circulating long non-coding RNAs as potential biomarkers for colorectal cancer. Genomics 114 (1), 31–37. doi:10.1016/j.ygeno.2021.11.026
Yi, Q., Yue, J., Liu, Y., Shi, H., Sun, W., Feng, J., et al. (2023). Recent advances of exosomal circRNAs in cancer and their potential clinical applications. J. Transl. Med. 21 (1), 516. doi:10.1186/s12967-023-04348-4
Yin, J.-B., Wang, X., Zhang, X., Liu, L., and Wang, R.-T. (2018). Mean platelet volume predicts survival in pancreatic cancer patients with synchronous liver metastases. Sci. Rep. 8 (1), 6014. doi:10.1038/s41598-018-24539-0
Ying, L., Sharma, A., Chhoda, A., Ruzgar, N., Hasan, N., Kwak, R., et al. (2021). Methylation-based cell-free DNA signature for early detection of pancreatic cancer. Pancreas 50 (9), 1267–1273. doi:10.1097/MPA.0000000000001919
Yokobori, T., Iinuma, H., Shimamura, T., Imoto, S., Sugimachi, K., Ishii, H., et al. (2013). Plastin3 is a novel marker for circulating tumor cells undergoing the epithelial-mesenchymal transition and is associated with colorectal cancer prognosis. Cancer Res. 73 (7), 2059–2069. doi:10.1158/0008-5472.CAN-12-0326
Yu, B., Du, Q., Li, H., Liu, H.-Y., Ye, X., Zhu, B., et al. (2017). Diagnostic potential of serum exosomal colorectal neoplasia differentially expressed long non-coding RNA (CRNDE-p) and microRNA-217 expression in colorectal carcinoma. Oncotarget 8 (48), 83745–83753. doi:10.18632/oncotarget.19407
Yu, H., Ma, L., Zhu, Y., Li, W., Ding, L., and Gao, H. (2020). Significant diagnostic value of circulating tumour cells in colorectal cancer. Oncol. Lett. 20 (1), 317–325. doi:10.3892/ol.2020.11537
Yue, C., Jiang, Y., Li, P., Wang, Y., Xue, J., Li, N., et al. (2018). Dynamic change of PD-L1 expression on circulating tumor cells in advanced solid tumor patients undergoing PD-1 blockade therapy. Oncoimmunology 7 (7), e1438111. doi:10.1080/2162402X.2018.1438111
Yun, C.-W., Lee, J.-H., Go, G., Jeon, J., Yoon, S., and Lee, S.-H. (2021). Prion protein of extracellular vesicle regulates the progression of colorectal cancer. Cancers 13 (9), 2144. doi:10.3390/cancers13092144
Zhang, D., Zhao, L., Zhou, P., Ma, H., Huang, F., Jin, M., et al. (2017). Circulating tumor microemboli (CTM) and vimentin+ circulating tumor cells (CTCs) detected by a size-based platform predict worse prognosis in advanced colorectal cancer patients during chemotherapy. Cancer Cell Int. 17, 6. doi:10.1186/s12935-016-0373-7
Zhang, F., Jiang, J., Qian, H., Yan, Y., and Xu, W. (2023). Exosomal circRNA: emerging insights into cancer progression and clinical application potential. J. Hematol. Oncol. 16 (1), 67. doi:10.1186/s13045-023-01452-2
Zhang, H., Liao, Z., Liu, F., Su, C., Zhu, H., Li, Y., et al. (2019a). Long noncoding RNA HULC promotes hepatocellular carcinoma progression. Aging 11 (20), 9111–9127. doi:10.18632/aging.102378
Zhang, K., Shao, C., Zhu, J., Lv, X., Tu, C., Jiang, C., et al. (2020). Exosomes function as nanoparticles to transfer miR-199a-3p to reverse chemoresistance to cisplatin in hepatocellular carcinoma. Biosci. Rep. 40 (7), BSR20194026. doi:10.1042/BSR20194026
Zhang, X., Wu, Z., Shen, Q., Li, R., Jiang, X., Wu, J., et al. (2019b). Clinical significance of cell-free DNA concentration and integrity in serum of gastric cancer patients before and after surgery. Cell. Mol. Biol. (Noisy-Le-Grand, France) 65 (7), 111–117. doi:10.14715/cmb/2019.65.7.19
Zhang, Y., Chen, L., Ye, X., Wu, Z., Zhang, Z., Sun, B., et al. (2021). Expression and mechanism of exosome-mediated A FOXM1 related long noncoding RNA in gastric cancer. J. Nanobiotechnology 19 (1), 133. doi:10.1186/s12951-021-00873-w
Zhang, Y., Liu, Y., Liu, H., and Tang, W. H. (2019c). Exosomes: biogenesis, biologic function and clinical potential. Cell and Biosci. 9, 19. doi:10.1186/s13578-019-0282-2
Zhao, L., Li, M., Zhang, S., and Liu, Y. (2022). Plasma-methylated SEPT9 for the noninvasive diagnosis of gastric cancer. J. Clin. Med. 11 (21), 6399. doi:10.3390/jcm11216399
Zheng, B., Liu, X.-L., Fan, R., Bai, J., Wen, H., Du, L.-T., et al. (2021a). The landscape of cell-free HBV integrations and mutations in cirrhosis and hepatocellular carcinoma patients. Clin. Cancer Res. An Official J. Am. Assoc. Cancer Res. 27 (13), 3772–3783. doi:10.1158/1078-0432.CCR-21-0002
Zheng, G.-D., Xu, Z.-Y., Hu, C., Lv, H., Xie, H.-X., Huang, T., et al. (2021b). Exosomal miR-590-5p in serum as a biomarker for the diagnosis and prognosis of gastric cancer. Front. Mol. Biosci. 8, 636566. doi:10.3389/fmolb.2021.636566
Zhong, Y., Zhou, Q., Zhang, Y., Zhou, S., Zhang, G., Jiang, C., et al. (2020). Cell-free DNA as a biomarker for colorectal cancer: a retrospective analysis in patients before and after surgery. Cell. Mol. Biol. (Noisy-Le-Grand, France) 66 (2), 135–141. doi:10.14715/cmb/2020.66.2.22
Zhou, H., Zhu, L., Song, J., Wang, G., Li, P., Li, W., et al. (2022). Liquid biopsy at the frontier of detection, prognosis and progression monitoring in colorectal cancer. Mol. Cancer 21 (1), 86. doi:10.1186/s12943-022-01556-2
Zhou, K.-Q., Sun, Y.-F., Cheng, J.-W., Du, M., Ji, Y., Wang, P.-X., et al. (2020). Effect of surgical margin on recurrence based on preoperative circulating tumor cell status in hepatocellular carcinoma. EBioMedicine 62, 103107. doi:10.1016/j.ebiom.2020.103107
Zhou, Y., Ren, H., Dai, B., Li, J., Shang, L., Huang, J., et al. (2018). Hepatocellular carcinoma-derived exosomal miRNA-21 contributes to tumor progression by converting hepatocyte stellate cells to cancer-associated fibroblasts. J. Exp. Clin. Cancer Res. 37 (1), 324. doi:10.1186/s13046-018-0965-2
Zhu, B., Gu, S., Wu, X., He, W., and Zhou, H. (2021a). Bioinformatics analysis of tumor-educated platelet microRNAs in patients with hepatocellular carcinoma. Biosci. Rep. 41 (12). doi:10.1042/BSR20211420
Zhu, P., Liu, H.-Y., Liu, F.-C., Gu, F.-M., Yuan, S.-X., Huang, J., et al. (2021b). Circulating tumor cells expressing krüppel-like factor 8 and vimentin as predictors of poor prognosis in pancreatic cancer patients. Cancer Control 28, 10732748211027163. doi:10.1177/10732748211027163
Zitt, M., Müller, H. M., Rochel, M., Schwendinger, V., Zitt, M., Goebel, G., et al. (2008). Circulating cell-free DNA in plasma of locally advanced rectal cancer patients undergoing preoperative chemoradiation: a potential diagnostic tool for therapy monitoring. Dis. Markers 25 (3), 159–165. doi:10.1155/2008/598071
Keywords: colorectal cancer, gastrointestinal cancers, liquid biopsy, prognosis, circulating tumor cells, clinical applications
Citation: Mondal D, Shinde S, Sinha V, Dixit V, Paul S, Gupta RK, Thakur S, Vishvakarma NK and Shukla D (2024) Prospects of liquid biopsy in the prognosis and clinical management of gastrointestinal cancers. Front. Mol. Biosci. 11:1385238. doi: 10.3389/fmolb.2024.1385238
Received: 12 February 2024; Accepted: 08 April 2024;
Published: 06 May 2024.
Edited by:
Ashok Kumar, All India Institute of Medical Sciences, IndiaReviewed by:
Taha Resid Özdemir, Health Sciences University, TürkiyeAsha Thomas, Case Western Reserve University, United States
Copyright © 2024 Mondal, Shinde, Sinha, Dixit, Paul, Gupta, Thakur, Vishvakarma and Shukla. This is an open-access article distributed under the terms of the Creative Commons Attribution License (CC BY). The use, distribution or reproduction in other forums is permitted, provided the original author(s) and the copyright owner(s) are credited and that the original publication in this journal is cited, in accordance with accepted academic practice. No use, distribution or reproduction is permitted which does not comply with these terms.
*Correspondence: Dhananjay Shukla, sdhannu@gmail.com