- 1Department of Hepatic Surgery, Second Affiliated Hospital of Harbin Medical University, Harbin, China
- 2Department of Pathology, Second Affiliated Hospital of Harbin Medical University, Harbin, China
Exosomes are small lipid nanovesicles with a diameter of 30–150 nm. They are present in all body fluids and are actively secreted by the majority of cells through the process of exocytosis. Exosomes play an essential role in intercellular communication and act as significant molecular carriers in regulating various physiological and pathological processes, such as the emergence of drug resistance in tumors. Tumor-associated exosomes transfer drug resistance to other tumor cells by releasing substances such as multidrug resistance proteins and miRNAs through exosomes. These substances change the cell phenotype, making it resistant to drugs. Tumor-associated exosomes also play a role in impacting drug resistance in other cells, like immune cells and stromal cells. Exosomes alter the behavior and function of these cells to help tumor cells evade immune surveillance and form a tumor niche. In addition, exosomes also export substances such as tumoricidal drugs and neutralizing antibody drugs to help tumor cells resist drug therapy. In this review, we summarize the mechanisms of exosomes in promoting drug resistance by delivering cargo in the context of the tumor microenvironment (TME).
1 Introduction
All structures that the cell releases into the external environment are known as extracellular vesicles (EVs) (Jia et al., 2022; Vergani et al., 2022; Jeppesen et al., 2023). Exosomes, the smallest subgroup of EVs, were originally thought to be the pathway by which cells excrete waste (Johnstone et al., 1991; Clancy and D’Souza-Schorey, 2023). Tumor-associated exosomes can transport their cargo to destination cells and cause associated phenotypic changes when released into the environment (Krylova and Feng, 2023). The generation of drug resistance in tumors involves many complicated processes, such as epigenetic modifications, changes in cell signal transduction pathways, drug metabolism, and excretion. Exosomes have the function of promoting drug resistance through material transfer (Antonarakis et al., 2014). For instance, exosomes carry drug-resistant genes and transfer them from resistant tumor cells to sensitive cells. Some molecules in exosomes (such as miRNA and lncRNA) protect drug-resistant tumor cells from treatment-induced cytotoxicity by inhibiting the apoptosis pathway, increasing tumor tolerance to chemotherapeutic drugs. Multiple exosomes were found to be upregulated in the plasma of patients with drug-resistant tumors, which suggests that different elements of exosomes influence tumor invasion and progression (Antonarakis et al., 2014). In this review, we focus on exosomes in tumor drug resistance research.
2 Exosomes mediate drug resistance transmission
Through horizontal transfer of genetic material, drug-resistant tumor cells can act as paracrine regulators and are abundant sources of exosomes. Exosomes can mediate the drug resistance of tumor cells by delivering a variety of cargoes like RNA (Antonarakis et al., 2014), DNA (Sansone et al., 2017), multidrug resistance (MDR)-associated proteins, and molecular metabolites such as prostaglandin E2 (PGE2) and TGF-β (Xiang et al., 2009). Exosomes play a role in transferring these cargoes with well-defined resistance phenotypes into sensitive cancer cells to alter cell growth and induce anti-preapoptotic pathways (Milman et al., 2019; Li et al., 2020a).
2.1 Transfer of the transmembrane protein
Chloride intracellular channel 1 (CLIC1) is an ion channel that is significantly related to drug resistance in gastric cancer cells (Ma et al., 2012). By isolating exosomes from the supernatant, Zhao et al. (2019) found that CLIC1 expression was higher in vincristine-resistant cell lines than in common GC cell lines. This proves that exosome-mediated transfer of CLIC1 can induce the development of vincristine resistance.
P-glycoprotein (P-gp) is a drug efflux pump reliant on energy regulated by the human ABCB1 gene and genes such as MDR1 (Lv et al., 2014). To make the drug effective, anti-tumor medications must reach the cytoplasm or nucleus. Tumor cells are able to encapsulate drugs as exosome cargo or secrete them out of the cell using P-gp. Blocking P-gp with calcium ion blockers can effectively prevent the efflux of anti-tumor drugs, thereby increasing the concentration of intracellular tumor drugs (Luo et al., 2013). Lv et al. (2014) found that the P-gp content of drug-resistant MCF-7/DOC was significantly higher than that of sensitive MCF-7/S, which indicated that tumor cells could induce chemotherapy-sensitive tumor cells to tolerate drugs by transferring P-gp.
2.2 Changing cytokines
LncARSR is an RNA activated in sunitinib-resistant renal cell carcinoma (RCC). It induces the expression of c-MET and AXL in RCC, which facilitates the resistance of Sunitinib by binding to miR-34/miR-449 competitively. Sunitinib resistance can be propagated by incorporating LncARSR into exosomes and delivering them to sensitive cells. Targeted nucleic acid therapy of sunitinib-resistant RCC against lncARSR or AXL/c-MET inhibitors restores the sunitinib response (Sun et al., 2018). The exosome-mediated circVMP1 delivery system has a similar effect. CircVMP1 targets the miR-524-5p-METTL3/SOX2 axis to promote cisplatin resistance in tumor cells, transmitting malignant features and cisplatin resistance to cisplatin-sensitive non-small cell lung cancer (NSCLC) (Xie et al., 2022). Analogously, microRNA-301b-3p from mesenchymal stem cells (MSCs) is delivered to chemotherapy-sensitive cells via exosomes to promote gastric cancer (GC) resistance by targeting TXNIP (Zhu et al., 2023). Wang et al. suggested that the knockout of the adipocyte-associated exosome LOC606724 or SNHG1 in adipocytes promotes the apoptotic effects of chemotherapy agents in multiple myeloma (MM) cells. MM cells facilitate LncRNA incorporation into adipocyte exosomes via methylation of LncRNA m6A mediated by METTL7A, leading to further resistance to MM (Wang et al., 2022a). In another experiment, exosomes from oxaliplatin-resistant colorectal cancer (CRC) cells delivered ciRS-122 to sensitive cells. Upregulation of miR-122 sponge and PKM2 promotes glycolysis and drug resistance. Moreover, miR-122 can target pyruvate kinase, thereby reducing the drug sensitivity of chemotherapy-sensitive cells (Wang et al., 2020a). Similarly, exosomes associated with oral squamous cell carcinoma (OSCC) induce drug resistance in tumor cells by upregulating the expression of mir-155 (Kirave et al., 2020). Liver cancer-related extracellular vesicles mediate drug efflux, thereby increasing chemotherapy drug resistance in hepatocellular carcinoma (HCC) by translocating Rab7402B (Li et al., 2020b). By downregulating miR-579-3p, circpar3-loaded exosomes induce resistance to cisplatin in the lateral population of nasopharyngeal cancer cells (Ai et al., 2023).
Since exosomes from cancer cells express cancer-associated cell surface proteins that sequester the substance away from the target cell, they obstruct antibody and medication therapy. For example, B-cell lymphoma cell-associated exosomes carry CD20, which binds to therapeutic anti-CD20 antibodies to deplete complement. This process protects lymphoma cells from antibody attack (Aung et al., 2011). Additionally, tumor-reactive antibodies are bound and sequestered by tumor exosomes to reduce their binding to breast cancer cells (Battke et al., 2011; Bach et al., 2017) (Figure 1).
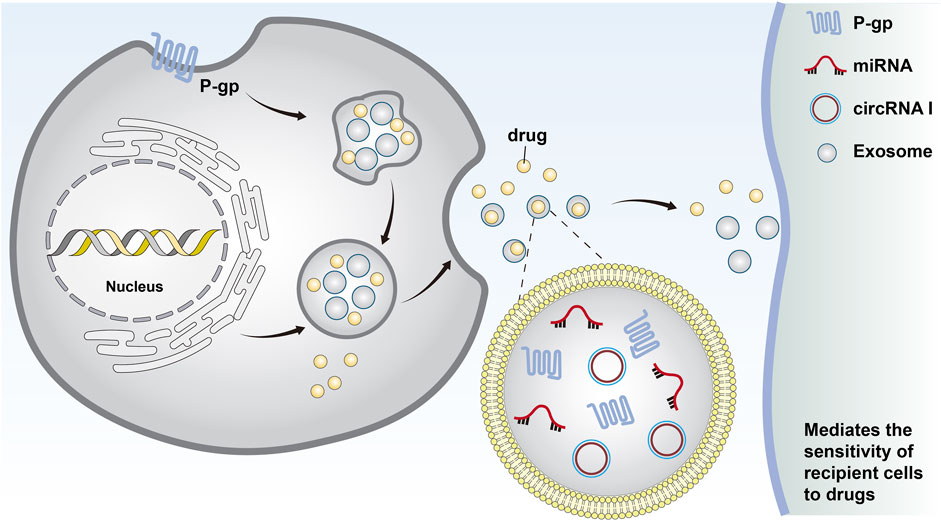
Figure 1. Cells form early endosomes through endocytosis. These early endosomes form the invagination of late endosomes through the plasma membrane and eventually become intracellular multi-vesticular bodies. The vesicles between 30 and 140 nm in diameter were identified as exosomes. Chemotherapeutic drugs can be excreted from cells through exosomes, and miRNAs and MDR-associated proteins can be secreted by exosomes to transfer drug resistance.
2.3 Mediating ferroptosis
The generation of harmful lipid peroxides in an iron-dependent way is the cause of ferroptosis (Lei et al., 2022; Tong et al., 2022; Zhao et al., 2022). Anti-tumor drugs such as erastin block the entry of extracellular cystine into cells by inhibiting system Xc activity. This process blocks the synthesis of glutathione (GSH) in cells, weakens the oxidation resistance of cells, and eventually leads to ferroptosis (Chen et al., 2021a). Exosomes derived from NSCLC cells transfer miR-4443 to susceptible cells, conferring cisplatin resistance. Cisplatin-induced FSP1-mediated ferroptosis is inhibited by the overexpression of miR-4443 (Song et al., 2021). The researchers highlighted that the lung cancer-associated exosome circRNA101093 (cir93) regulates arachidonic acid (AA) by maintaining an increase in cir93 in lung adenocarcinoma (LUAD) cells. cir93 interacts with and increases fatty acid-binding protein 3 (FABP3). FABP3 transports AA and enhances its response to taurine, leading to a reduction in AA and the induction of chemotherapy drug tolerance (Zhang et al., 2022a). Through Zhang et al.’s investigation, these tests revealed that exosome miR-522 secreted by GC-related fibroblasts can lead to the inhibition of ALOX15. It might lessen the buildup of lipids in tumor cells to prevent ferroptosis of tumor cells, finally leading to reduced chemotherapy sensitivity (Zhang et al., 2020). Cancer-associated fibroblasts (CAFs) were found to inhibit ferroptosis and promote the chemoresistance of pancreatic ductal adenocarcinoma (PDAC) cells after gemcitabine (GEM) treatment by secreting exosomes miR-3173-5p (Qi et al., 2023). Ferroptosis provides a new direction for antagonizing tumor drug resistance. Du et al. (2021) constructed exosomes functionalized with CD47. The researchers used ultrasound to effectively load siderosis inducers into exosomes to accelerate HCC’s ferroptosis process and provide anti-tumor effects (Du et al., 2021).
3 Mediating immune escape
Immune escape is a significant factor in tumor drug resistance. The primary defense against cancer originates from innate immune cells, which engage in interactions with adaptive immune cells and exchange exosomes with one another (Neviani et al., 2019). Tumors exert control over pathways such as differentiation, polarization, cytokine recruitment, and others, thereby either promoting or suppressing immune-associated exosomes.
The exosomes of the cerebrospinal fluid of gliomas contain an exclusive protein called LGALS9 ligand. The ligand attaches itself to the dendritic cells’ TIM3 receptor in the cerebrospinal fluid, further interferes with the identification of dendritic cells, inhibits antigen presentation, and leads to the inactivation of cytotoxic T cell-mediated immune responses against tumors (Wang et al., 2020b). NK cells contribute to the process of immune surveillance and immune clearance of tumor cells. Lucia found that NKG2D ligands were significantly upregulated in the supernatant of exosomes derived from epithelial ovarian cancer cells. NKG2D ligands can impair the cytotoxic function of NK cells by depleting NKG2D. This suggests that exosomes containing NKG2D ligands can be released by tumors to exert cytotoxic effects (Mincheva-Nilsson and Baranov, 2014; Labani-Motlagh et al., 2016). The exosomes miR-21-5p and miR-155-5p secreted by M2 macrophages regulate the migration, growth, and invasion of glioma cells and the formation of new blood vessels (Guo et al., 2023a). Other cell populations can regulate innate immune cells through the endocrine and paracrine effects of exosomes. For instance, exosomes of adipose-derived MSCs regulate the polarization of M2 macrophages by upregulating M2 markers such as CD163, Arg1, and CD206 and activating MafB and STAT6 (Heo et al., 2019). In addition, EVs secreted by MSCs before hypoxia stimulation downregulated PTEN through miR-21-5p delivery and induced M2 polarization (Ren et al., 2019a). Wu et al. (2021a) found that exosomes produced from M2 macrophages transferred CD11b/CD18 to HCC cells. Matrix metalloproteinase-9 (MMP-9) is activated, which greatly accelerates tumor drug resistance (Wu et al., 2021a). Exosomes derived from GL261 cells significantly inhibited the amount of CD8+ T cells in spleen cells by blocking the breakdown of IFN-γ and granzyme B (Liu et al., 2013). Likewise, exosomes associated with NSCLC cells can inhibit CD8+T cells from secreting IFN-γ, TNF-α, and other immune cells. In addition, by binding miR-934 and upregulating the expression of protein tyrosine phosphatase 2 (SHP2), which has the Src homology 2 (SH2) domain, circUSP7 suppresses CD8+T cells (Chen et al., 2021b). Tumor cells affect the production and migration of corresponding immune cells through exosomes and reconstruct the immune microenvironment to mediate the generation of drug resistance (Table 1).
4 Tumor microenvironment
Tumor-associated exosomes mediate TME changes through endocrine effects (Attaran and Bissell, 2022), such as hypoxia (He et al., 2022), starvation, and acidosis (Xia et al., 2021; Zhang et al., 2021). These stressful environments further increase the release of tumor-associated exosomes, forming a feedback loop and ultimately promoting tumor drug resistance (Mashouri et al., 2019; Han et al., 2022).
4.1 Hypoxia
One study showed that miR-182-5p was markedly upregulated in the exosomes of glioblastoma in a hypoxic environment. VEGFR accumulates as a result of direct inhibition of its target Kruppel-like factors 2 and 4 by exosome miR-182-5p. It induces tumor angiogenesis. The structure of the newly formed vessels is unstable, and blood flow may be rapid, which makes it difficult for the drug to effectively cross the tumor vascular network, thereby limiting the drug’s reach to the tumor tissue and reducing its concentration within the tumor (Li et al., 2020c). At the transcriptional level, circPDK1 secreted by pancreatic cancer-associated exosome packaging can be activated by HIF1A, and the BPTF/c-myc axis is activated by miR-628-3p (Lin et al., 2022). Additionally, researchers discovered that the stronger oncogenic proteins STAT3 and FAS are present in exosomes of ovarian cancer cell lines grown in hypoxic environments, which dramatically increases in vitro cell motility and invasion as well as chemotherapy resistance (Dorayappan et al., 2018). Similar responses have been shown in breast cancer (BC) (King et al., 2012; Jung et al., 2018; Hannafon et al., 2019; Tian et al., 2021; Xi et al., 2021), HCC (Matsuura et al., 2019; Yu et al., 2019; Wang et al., 2022b; Yu et al., 2022; Jia et al., 2023), pancreatic cancer (Patton et al., 2020; Chen et al., 2022; Lin et al., 2022), GC (Du et al., 2020; Xia et al., 2020), CRC (Ren et al., 2019b; Li et al., 2021; Sun et al., 2021; Wu et al., 2023), and prostate cancer (PC) (Deep et al., 2020).
Critical processes in the formation of exosomes, such as vesicle budding and cargo sorting, are adversely impacted in an anoxic environment (Bister et al., 2020; He et al., 2022). Furthermore, hypoxia can affect ubiquitination proteins and ubiquitination-related enzymes, affecting the sorting of exosomal proteins. Ubiquitin proteins can accumulate through the ubiquitin-binding domains of ESCRT-0 and ESCRT-II within the multi-vesicular endosome microdomain. This process limits the number of membranes available for exosome formation, which affects exosome volume (van Niel et al., 2018; Jiang et al., 2022). In a study of PC patients, plasma from tumor patients showed higher levels of exosomes and lower volumes of exosomes. Smaller exosomes are readily able to pass through physiological barriers and enter more cells. Smaller exosomes may travel more readily through the bloodstream to the site of metastasis with this variation, which weakens the effect of drug therapy (Logozzi et al., 2021). Studies have shown that the sugar mixture on the surface of tumor-associated exosomes is highly specific (Zheng et al., 2020; Zhang et al., 2022b). The endocytosis mechanism of exosomes is sensitive to hypoxia. More exosomes can be absorbed by hypoxic cells reliant on proteoglycans (Cerezo-Magaña et al., 2021). In conclusion, modifications to plasma membrane glycoproteins brought about by hypoxia may enhance the drug resistance of tumor cells.
4.2 Epithelial mesenchymal transformation
Epithelial–mesenchymal transformation (EMT) of tumor tissues promotes drug-resistant cell survival. Cancer cells’ enhanced plasticity enables non-genetic adaptation to drug tolerance. Under tyrosine kinase inhibition (TKI), EMT is connected to the survival of drug-resistant cells in EGFR-mutated NSCLC (Remon et al., 2018). Exosome miR-663b secreted by cervical cancer cells directly targets the 3′-untranslated region (3′-UTR) of monoacylglycerol acyltransferase 3 (MGAT3) and participates in the process of EMT transformation. Exosome miR-663b-induced metastasis of ovarian cancer cells is inhibited by MGAT3 overexpression. At the same time, exosome miR-663b can be enucleated by cervical cancer cells, which affects the survival of drug-resistant cells (You et al., 2021). The GC-associated exosome circ133 acts on the miR-133a and RhoA axes. Circ133 promotes cancer by disinhibiting its target SIX1. Interestingly, the target SIX1 reduced the sensitivity to chemotherapy in BC cells by increasing the TGFβ signaling pathway (Micalizzi et al., 2009). Moreover, GC-associated exosomes induce the transcription of ZEB1 to regulate the differentiation of CRC cells and induce drug resistance (Ono et al., 2012). CRC-associated exosomes promote EMT and increase the intracellular accumulation of oxaliplatin by delivering miR-128-3p. They have the potential to improve drug sensitivity and reduce drug resistance, serving as novel biomarkers (Liu et al., 2022a).
4.3 Proangiogenic effect
Exosomes promote tumor angiogenesis through various mechanisms that have antagonistic effects on anti-angiogenic drugs and eventually lead to drug resistance. Exosome miR-204-3p induces vascular endothelial cells to form tubular forms via the ATXN1/STAT3 pathway. TAK-981 has a function in inhibiting SUMOylation. It prevents tumor growth and angiogenesis by blocking miR-204-3p′s exosome sorting process. Glioma cells can accelerate angiogenesis by upregulating SUMOylation and eliminating the inhibitory factor miR-204-3p under hypoxia (Guo et al., 2023b). OSCC cells have upregulated miRNA-210-3p to promote tumor growth by increasing tumor grade and microvascular density. Ephrin A3 expression is mechanically downregulated by the exosomal miRNA-210-3p to activate the PI3K/Akt axis. The process increases angiogenesis and advances OSCC (Wang et al., 2020c). Analogously, the high migratory potential of nasopharyngeal carcinoma cells is associated with angiogenesis. Exosome miRNA-23a inhibits the production of TSGA10 by binding to its 3′-UTR. MiRNA-23a leads to angiogenesis and increases metastasis in nasopharyngeal carcinoma (Bao et al., 2018). Exosome lncRNA may also play an essential role in regulating angiogenesis. For example, exosomal miRNA-29-3p downregulates the expression of PTEN, and the inactivated PTEN signaling pathway promotes the phosphorylation of PI3K/Akt, which promotes angiogenesis in lung cancer (Cheng et al., 2019). Angiogenin-2 (ANGPT2) is believed to promote angiogenesis by disrupting vascular stability. HCC cells can secrete exosomes containing ANGPT2. These exosomes are introduced into umbilical vein endothelial cells by intracellular action, and elevated expression of ANGPT2 causes angiogenesis and encourages drug resistance (Xie et al., 2020) (Figure 2).
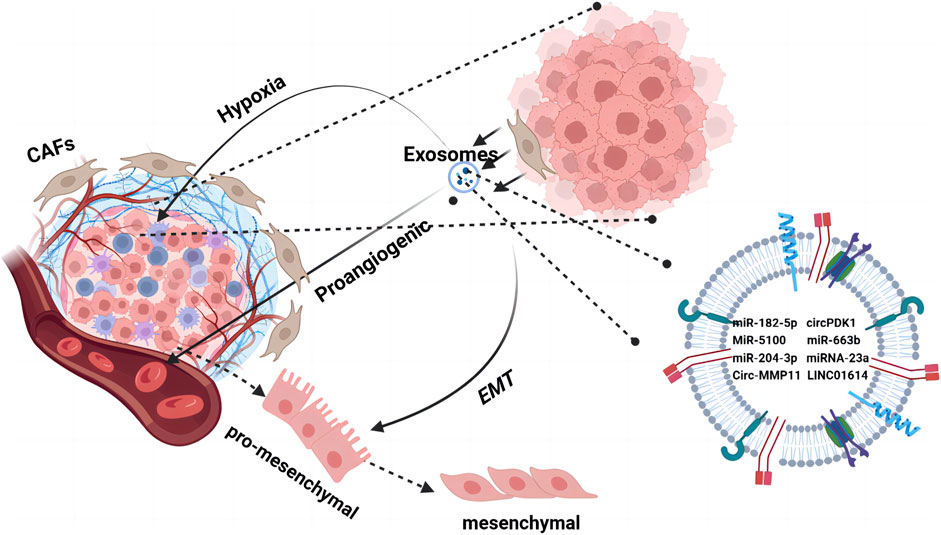
Figure 2. The TME orchestrates cell–cell interactions, such as paracrine and near-paracrine interactions, through tumor-associated exosome networks. It is composed of a variety of cells embedded in the extracellular matrix, including endothelial cells and tumor-associated fibroblasts. Tumor cells secrete cytokines through exosomes to promote changes in the TME such as hypoxia, angiogenesis, and EMT. Tumor cells use the regulatory mechanisms of microsystems to form a drug-resistant state.
4.4 CAF-related exosomes
Wu et al. measured RNA levels in lapatinib-resistant BC specimens and then highlighted that exosomes may be a means of delivering Circ-MMP11. The knockdown of circ-MMP11 promotes lapatinib sensitivity by inhibiting cell survival and invasion while promoting apoptosis in lapatinib-resistant BC cells (Wu et al., 2021b). In Liu’s study, cancer-associated fibroblasts of LUAD secreted a long non-coding RNA, LINC01614, by exosome packaging. This RNA directly interacts with ANXA2 and p65 to promote NF-κB activation, with the glutamine transporters SLC38A2 and SLC7A5 being overexpressed, eventually enhancing glutamine influx in cancer cells. Alterations in glutamine metabolism increase the antioxidant capacity of cells and reduce their susceptibility to drug-induced apoptosis (Liu et al., 2022b).
In previous studies, researchers have suggested that cancer stem cells (CSCs) are inherently resistant to chemotherapy-induced cell death. After chemotherapy treatment, fibroblast-derived conditioned medium (CM) promoted the proportion of CSCs (CD133+ and TOP-GFP+) and tumor viability. Further studies have shown that exosomes isolated from CM have similar effects described above (Ren et al., 2018; Hu et al., 2019). Correspondingly, through the METTL3/miR-181d-5p axis, METTL3 decreases the susceptibility of CRC cells to 5-fururacil (5-FU) and enhances exosome miR-181b-5p in CAFs (Pan et al., 2022).
5 Therapeutic strategies
Because of their role in promoting drug resistance in tumor cells, exosomes have great potential to be a therapeutic target for inhibiting tumor drug resistance. Limiting the biogenesis and release of exosomes or downregulating the uptake of exosomes by target cells has functions in restoring the sensitivity of tumors to chemotherapeutic drugs. For ESCFRT-dependent cells, downregulation of hepatocyte growth factor-regulated tyrosine kinase substrate (HRS) and signal transducer adapter molecule 1 (STAM1) can limit the secretion of exosomes and limit the efflux of drugs from tumor cells (Colombo et al., 2013). In addition, antagonizing drug efflux and changing the TME can indirectly compensate for the drug resistance caused by exosomes to a certain extent. For example, Diannexin can impair the angiogenesis of A431 squamous carcinoma in SCID mice by downregulating exosomes (Al-Nedawi et al., 2009). Exosomes can also induce drug killing in other ways. Autophagy and exosomes can compensate for each other in different environments to promote the export of signaling molecules (Deng et al., 2019). The combined use of chemotherapeutic drugs and autophagy inhibitors such as chloroquine or hydroxychloroquine can significantly enhance the sensitivity of chemotherapeutic drugs (Singh et al., 2018). RNAs from exosomes could compete with PD-L1 to modulate DNA damage responses, thereby enhancing chemotherapy sensitivity (Tu et al., 2019). Key concepts of therapy methods using exosomes to mediate drug effects are summarized in Table 2.
6 Conclusion
The diversity of exosomes confers significant advantages as biomarkers, and their stable structure effectively transfers the genetic information of the parent cells. Tumor-derived exosomes also profoundly affect the process of drug resistance. In tumor patients, exosomes generated from tumors transfer RNA and proteins to mediate drug resistance. At the same time, tumor cells influence the TME through exosomes, causing a series of changes, such as hypoxia, to induce drug resistance. This means that the use of exosomes can reverse the drug resistance of tumor cells and increase the effectiveness of drug therapy. Since exosomes are multi-purpose carriers of communication between multiple organs, restoring their normal secretion plays a particularly important role in the treatment of patients with tumors (Dixson et al., 2023). Specific components in exosomes, such as miRNAs or proteins, may become biomarkers of tumor resistance. By examining the expression levels of specific components in extracellular vesicles secreted from patient samples, we can help predict patient response to drug therapy and guide an individualized treatment plans (Fitts et al., 2019; Hoshino et al., 2020). Targeting specific signaling pathways or related molecules involved in the generation and release of extracellular vesicles could inhibit tumor drug resistance (Pu and Ji, 2022). Exosomes are expected to be useful indicators for tumor therapy as they regulate many aspects of heterotypic cell-to-cell interactions in the TME. Plenty of exosomes have been discovered and uploaded to the Vesiclepedia online compendium. Researchers can review the identified RNA molecules and proteins in exosomes (Kalra et al., 2012). A deeper understanding of the biogenesis of tumor-associated exosomes is of great significance for further exploration of the information exchange between tumor and normal cells and for utilizing their properties to guide combined clinical therapy (Debbi et al., 2022; Rezaie et al., 2022).
Author contributions
BR: writing–review and editing and writing–original draft. XL: writing–review and editing and writing–original draft. ZZ: writing–review and editing. ST: writing–review and editing, supervision, and conceptualization. SY: writing–review and editing, supervision, and conceptualization.
Funding
The author(s) declare that financial support was received for the research, authorship, and/or publication of this article. This study was supported by 2023 Basic scientific research expenses scientific research projects of universities of Heilongjiang province (NO. 2023-KYYWF-0182).
Acknowledgments
The authors thank all who made contributions to the manuscript.
Conflict of interest
The authors declare that the research was conducted in the absence of any commercial or financial relationships that could be construed as a potential conflict of interest.
Publisher’s note
All claims expressed in this article are solely those of the authors and do not necessarily represent those of their affiliated organizations, or those of the publisher, the editors, and the reviewers. Any product that may be evaluated in this article, or claim that may be made by its manufacturer, is not guaranteed or endorsed by the publisher.
References
Ai, J., Tan, G., Li, W., Liu, H., Li, T., Zhang, G., et al. (2023). Exosomes loaded with circPARD3 promotes EBV-miR-BART4-induced stemness and cisplatin resistance in nasopharyngeal carcinoma side population cells through the miR-579-3p/SIRT1/SSRP1 axis. Cell. Biol. Toxicol. 39 (2), 537–556. doi:10.1007/s10565-022-09738-w
Al-Nedawi, K., Meehan, B., Kerbel, R. S., Allison, A. C., and Rak, J. (2009). Endothelial expression of autocrine VEGF upon the uptake of tumor-derived microvesicles containing oncogenic EGFR. Proc. Natl. Acad. Sci. U. S. A. 106 (10), 3794–3799. doi:10.1073/pnas.0804543106
Antonarakis, E. S., Lu, C., Wang, H., Luber, B., Nakazawa, M., Roeser, J. C., et al. (2014). AR-V7 and resistance to enzalutamide and abiraterone in prostate cancer. N. Engl. J. Med. 371 (11), 1028–1038. doi:10.1056/NEJMoa1315815
Attaran, S., and Bissell, M. J. (2022). The role of tumor microenvironment and exosomes in dormancy and relapse. Semin. Cancer Biol. 78, 35–44. doi:10.1016/j.semcancer.2021.09.008
Aung, T., Chapuy, B., Vogel, D., Wenzel, D., Oppermann, M., Lahmann, M., et al. (2011). Exosomal evasion of humoral immunotherapy in aggressive B-cell lymphoma modulated by ATP-binding cassette transporter A3. Proc. Natl. Acad. Sci. U. S. A. 108 (37), 15336–15341. doi:10.1073/pnas.1102855108
Bach, D. H., Hong, J. Y., Park, H. J., and Lee, S. K. (2017). The role of exosomes and miRNAs in drug-resistance of cancer cells. Int. J. Cancer 141 (2), 220–230. doi:10.1002/ijc.30669
Bao, L., You, B., Shi, S., Shan, Y., Zhang, Q., Yue, H., et al. (2018). Metastasis-associated miR-23a from nasopharyngeal carcinoma-derived exosomes mediates angiogenesis by repressing a novel target gene TSGA10. Oncogene 37 (21), 2873–2889. doi:10.1038/s41388-018-0183-6
Battke, C., Ruiss, R., Welsch, U., Wimberger, P., Lang, S., Jochum, S., et al. (2011). Tumour exosomes inhibit binding of tumour-reactive antibodies to tumour cells and reduce ADCC. Cancer Immunol. Immunother. 60 (5), 639–648. doi:10.1007/s00262-011-0979-5
Bister, N., Pistono, C., Huremagic, B., Jolkkonen, J., Giugno, R., and Malm, T. (2020). Hypoxia and extracellular vesicles: a review on methods, vesicular cargo and functions. J. Extracell. Vesicles 10 (1), e12002. doi:10.1002/jev2.12002
Cerezo-Magaña, M., Christianson, H. C., van Kuppevelt, T. H., Forsberg-Nilsson, K., and Belting, M. (2021). Hypoxic induction of exosome uptake through proteoglycan-dependent endocytosis fuels the lipid droplet phenotype in glioma. Mol. Cancer Res. 19 (3), 528–540. doi:10.1158/1541-7786.MCR-20-0560
Chen, K., Wang, Q., Liu, X., Wang, F., Yang, Y., and Tian, X. (2022). Hypoxic pancreatic cancer derived exosomal miR-30b-5p promotes tumor angiogenesis by inhibiting GJA1 expression. Int. J. Biol. Sci. 18 (3), 1220–1237. doi:10.7150/ijbs.67675
Chen, S. W., Zhu, S. Q., Pei, X., Qiu, B. Q., Xiong, D., Long, X., et al. (2021b). Cancer cell-derived exosomal circUSP7 induces CD8(+) T cell dysfunction and anti-PD1 resistance by regulating the miR-934/SHP2 axis in NSCLC. Mol. Cancer 20 (1), 144. doi:10.1186/s12943-021-01448-x
Chen, X., Kang, R., Kroemer, G., and Tang, D. (2021a). Broadening horizons: the role of ferroptosis in cancer. Nat. Rev. Clin. Oncol. 18 (5), 280–296. doi:10.1038/s41571-020-00462-0
Cheng, Y., Dai, X., Yang, T., Zhang, N., Liu, Z., and Jiang, Y. (2019). Low long noncoding RNA growth arrest-specific transcript 5 expression in the exosomes of lung cancer cells promotes tumor angiogenesis. J. Oncol. 2019, 2476175. doi:10.1155/2019/2476175
Clancy, J. W., and D’Souza-Schorey, C. (2023). Tumor-derived extracellular vesicles: multifunctional entities in the tumor microenvironment. Annu. Rev. Pathol. 18, 205–229. doi:10.1146/annurev-pathmechdis-031521-022116
Colombo, M., Moita, C., van Niel, G., Kowal, J., Vigneron, J., Benaroch, P., et al. (2013). Analysis of ESCRT functions in exosome biogenesis, composition and secretion highlights the heterogeneity of extracellular vesicles. J. Cell. Sci. 126 (Pt 24), 5553–5565. doi:10.1242/jcs.128868
Debbi, L., Guo, S., Safina, D., and Levenberg, S. (2022). Boosting extracellular vesicle secretion. Biotechnol. Adv. 59, 107983. doi:10.1016/j.biotechadv.2022.107983
Deep, G., Jain, A., Kumar, A., Agarwal, C., Kim, S., Leevy, W. M., et al. (2020). Exosomes secreted by prostate cancer cells under hypoxia promote matrix metalloproteinases activity at pre-metastatic niches. Mol. Carcinog. 59 (3), 323–332. doi:10.1002/mc.23157
Deng, S., Shanmugam, M. K., Kumar, A. P., Yap, C. T., Sethi, G., and Bishayee, A. (2019). Targeting autophagy using natural compounds for cancer prevention and therapy. Cancer 125 (8), 1228–1246. doi:10.1002/cncr.31978
Dixson, A. C., Dawson, T. R., Di Vizio, D., and Weaver, A. M. (2023). Context-specific regulation of extracellular vesicle biogenesis and cargo selection. Nat. Rev. Mol. Cell. Biol. 24 (7), 454–476. doi:10.1038/s41580-023-00576-0
Dorayappan, K. D. P., Wanner, R., Wallbillich, J. J., Saini, U., Zingarelli, R., Suarez, A. A., et al. (2018). Hypoxia-induced exosomes contribute to a more aggressive and chemoresistant ovarian cancer phenotype: a novel mechanism linking STAT3/Rab proteins. Oncogene 37 (28), 3806–3821. doi:10.1038/s41388-018-0189-0
Du, J., Liang, Y., Li, J., Zhao, J. M., Wang, Z. N., and Lin, X. Y. (2020). Gastric cancer cell-derived exosomal microRNA-23a promotes angiogenesis by targeting PTEN. Front. Oncol. 10, 326. doi:10.3389/fonc.2020.00326
Du, J., Wan, Z., Wang, C., Lu, F., Wei, M., Wang, D., et al. (2021). Designer exosomes for targeted and efficient ferroptosis induction in cancer via chemo-photodynamic therapy. Theranostics 11 (17), 8185–8196. doi:10.7150/thno.59121
Fitts, C. A., Ji, N., Li, Y., and Tan, C. (2019). Exploiting exosomes in cancer liquid biopsies and drug delivery. Adv. Healthc. Mater 8 (6), e1801268. doi:10.1002/adhm.201801268
Guo, Q., Fan, Y., Wang, Q., Li, B., Qiu, W., Qi, Y., et al. (2023b). Glioblastoma upregulates SUMOylation of hnRNP A2/B1 to eliminate the tumor suppressor miR-204-3p, accelerating angiogenesis under hypoxia. Cell. Death Dis. 14 (2), 147. doi:10.1038/s41419-023-05663-w
Guo, X., Sui, R., and Piao, H. (2023a). Exosomes-mediated crosstalk between glioma and immune cells in the tumor microenvironment. CNS Neurosci. Ther. 29 (8), 2074–2085. doi:10.1111/cns.14239
Han, Q. F., Li, W. J., Hu, K. S., Gao, J., Zhai, W. L., Yang, J. H., et al. (2022). Exosome biogenesis: machinery, regulation, and therapeutic implications in cancer. Mol. Cancer 21 (1), 207. doi:10.1186/s12943-022-01671-0
Hannafon, B. N., Xu, Y. F., Bruns, M., Calloway, C. L., and Ding, W. Q. (2019). Metastasis-associated protein 1 (MTA1) is transferred by exosomes and contributes to the regulation of hypoxia and estrogen signaling in breast cancer cells. Cell. Commun. Signal 17 (1), 13. doi:10.1186/s12964-019-0325-7
He, G., Peng, X., Wei, S., Yang, S., Li, X., Huang, M., et al. (2022). Exosomes in the hypoxic TME: from release, uptake and biofunctions to clinical applications. Mol. Cancer 21 (1), 19. doi:10.1186/s12943-021-01440-5
Heo, J. S., Choi, Y., and Kim, H. O. (2019). Adipose-derived mesenchymal stem cells promote M2 macrophage phenotype through exosomes. Stem Cells Int. 2019, 7921760. doi:10.1155/2019/7921760
Hoshino, A., Kim, H. S., Bojmar, L., Gyan, K. E., Cioffi, M., Hernandez, J., et al. (2020). Extracellular vesicle and particle biomarkers define multiple human cancers. Cell. 182 (4), 1044–1061. doi:10.1016/j.cell.2020.07.009
Hu, Y. B., Yan, C., Mu, L., Mi, Y. L., Zhao, H., Hu, H., et al. (2019). Exosomal Wnt-induced dedifferentiation of colorectal cancer cells contributes to chemotherapy resistance. Oncogene 38 (11), 1951–1965. doi:10.1038/s41388-018-0557-9
Jeppesen, D. K., Zhang, Q., Franklin, J. L., and Coffey, R. J. (2023). Extracellular vesicles and nanoparticles: emerging complexities. Trends Cell. Biol. 33 (8), 667–681. doi:10.1016/j.tcb.2023.01.002
Jia, W., Liang, S., Lin, W., Li, S., Yuan, J., Jin, M., et al. (2023). Hypoxia-induced exosomes facilitate lung pre-metastatic niche formation in hepatocellular carcinoma through the miR-4508-RFX1-IL17A-p38 MAPK-NF-κB pathway. Int. J. Biol. Sci. 19 (15), 4744–4762. doi:10.7150/ijbs.86767
Jia, Y., Yu, L., Ma, T., Xu, W., Qian, H., Sun, Y., et al. (2022). Small extracellular vesicles isolation and separation: current techniques, pending questions and clinical applications. Theranostics 12 (15), 6548–6575. doi:10.7150/thno.74305
Jiang, H., Zhao, H., Zhang, M., He, Y., Li, X., Xu, Y., et al. (2022). Hypoxia induced changes of exosome cargo and subsequent biological effects. Front. Immunol. 13, 824188. doi:10.3389/fimmu.2022.824188
Johnstone, R. M., Mathew, A., Mason, A. B., and Teng, K. (1991). Exosome formation during maturation of mammalian and avian reticulocytes: evidence that exosome release is a major route for externalization of obsolete membrane proteins. J. Cell. Physiol. 147 (1), 27–36. doi:10.1002/jcp.1041470105
Jung, K. O., Jo, H., Yu, J. H., Gambhir, S. S., and Pratx, G. (2018). Development and MPI tracking of novel hypoxia-targeted theranostic exosomes. Biomaterials 177, 139–148. doi:10.1016/j.biomaterials.2018.05.048
Kalra, H., Simpson, R. J., Ji, H., Aikawa, E., Altevogt, P., Askenase, P., et al. (2012). Vesiclepedia: a compendium for extracellular vesicles with continuous community annotation. PLoS Biol. 10 (12), e1001450. doi:10.1371/journal.pbio.1001450
King, H. W., Michael, M. Z., and Gleadle, J. M. (2012). Hypoxic enhancement of exosome release by breast cancer cells. BMC Cancer 12, 421. doi:10.1186/1471-2407-12-421
Kirave, P., Gondaliya, P., Kulkarni, B., Rawal, R., Garg, R., Jain, A., et al. (2020). Exosome mediated miR-155 delivery confers cisplatin chemoresistance in oral cancer cells via epithelial-mesenchymal transition. Oncotarget 11 (13), 1157–1171. doi:10.18632/oncotarget.27531
Krylova, S. V., and Feng, D. (2023). The machinery of exosomes: biogenesis, release, and uptake. Int. J. Mol. Sci. 24 (2), 1337. doi:10.3390/ijms24021337
Labani-Motlagh, A., Israelsson, P., Ottander, U., Lundin, E., Nagaev, I., Nagaeva, O., et al. (2016). Differential expression of ligands for NKG2D and DNAM-1 receptors by epithelial ovarian cancer-derived exosomes and its influence on NK cell cytotoxicity. Tumour Biol. 37 (4), 5455–5466. doi:10.1007/s13277-015-4313-2
Lei, G., Zhuang, L., and Gan, B. (2022). Targeting ferroptosis as a vulnerability in cancer. Nat. Rev. Cancer 22 (7), 381–396. doi:10.1038/s41568-022-00459-0
Li, J., Yang, P., Chen, F., Tan, Y., Huang, C., Shen, H., et al. (2021). Hypoxic colorectal cancer-derived extracellular vesicles deliver microRNA-361-3p to facilitate cell proliferation by targeting TRAF3 via the noncanonical NF-κB pathways. Clin. Transl. Med. 11 (3), e349. doi:10.1002/ctm2.349
Li, J., Yuan, H., Xu, H., Zhao, H., and Xiong, N. (2020c). Hypoxic cancer-secreted exosomal miR-182-5p promotes glioblastoma angiogenesis by targeting kruppel-like factor 2 and 4. Mol. Cancer Res. 18 (8), 1218–1231. doi:10.1158/1541-7786.MCR-19-0725
Li, R., Dong, C., Jiang, K., Sun, R., Zhou, Y., Yin, Z., et al. (2020b). Rab27B enhances drug resistance in hepatocellular carcinoma by promoting exosome-mediated drug efflux. Carcinogenesis 41 (11), 1583–1591. doi:10.1093/carcin/bgaa029
Li, S., Yi, M., Dong, B., Jiao, Y., Luo, S., and Wu, K. (2020a). The roles of exosomes in cancer drug resistance and its therapeutic application. Clin. Transl. Med. 10 (8), e257. doi:10.1002/ctm2.257
Lin, J., Wang, X., Zhai, S., Shi, M., Peng, C., Deng, X., et al. (2022). Hypoxia-induced exosomal circPDK1 promotes pancreatic cancer glycolysis via c-myc activation by modulating miR-628-3p/BPTF axis and degrading BIN1. J. Hematol. Oncol. 15 (1), 128. doi:10.1186/s13045-022-01348-7
Liu, T., Fang, P., Ma, Z., Wang, X., Chen, H., et al. (2022b). Cancer-associated fibroblast-specific lncRNA LINC01614 enhances glutamine uptake in lung adenocarcinoma. J. Hematol. Oncol. 15 (1), 141. doi:10.1186/s13045-022-01359-4
Liu, T., Zhang, X., Du, L., Wang, Y., Liu, X., Tian, H., et al. (2022a). Retraction Note: exosome-transmitted miR-128-3p increase chemosensitivity of oxaliplatin-resistant colorectal cancer. Mol. Cancer 21 (1), 157. doi:10.1186/s12943-022-01627-4
Liu, Z. M., Wang, Y. B., and Yuan, X. H. (2013). Exosomes from murine-derived GL26 cells promote glioblastoma tumor growth by reducing number and function of CD8+T cells. Asian Pac J. Cancer Prev. 14 (1), 309–314. doi:10.7314/apjcp.2013.14.1.309
Logozzi, M., Mizzoni, D., Di Raimo, R., Giuliani, A., Maggi, M., Sciarra, A., et al. (2021). Plasmatic exosome number and size distinguish prostate cancer patients from healthy individuals: a prospective clinical study. Front. Oncol. 11, 727317. doi:10.3389/fonc.2021.727317
Luo, L., Sun, Y. J., Yang, L., Huang, S., and Wu, Y. J. (2013). Avermectin induces P-glycoprotein expression in S2 cells via the calcium/calmodulin/NF-κB pathway. Chem. Biol. Interact. 203 (2), 430–439. doi:10.1016/j.cbi.2013.03.009
Lv, M. M., Zhu, X. y., Chen, W. x., Zhong, S. l., Hu, Q., Ma, T. f., et al. (2014). Exosomes mediate drug resistance transfer in MCF-7 breast cancer cells and a probable mechanism is delivery of P-glycoprotein. Tumour Biol. 35 (11), 10773–10779. doi:10.1007/s13277-014-2377-z
Ma, P. F., Chen, J. Q., Wang, Z., Liu, J. L., and Li, B. P. (2012). Function of chloride intracellular channel 1 in gastric cancer cells. World J. Gastroenterol. 18 (24), 3070–3080. doi:10.3748/wjg.v18.i24.3070
Mashouri, L., Yousefi, H., Aref, A. R., Ahadi, A. M., Molaei, F., and Alahari, S. K. (2019). Exosomes: composition, biogenesis, and mechanisms in cancer metastasis and drug resistance. Mol. Cancer 18 (1), 75. doi:10.1186/s12943-019-0991-5
Matsuura, Y., Wada, H., Eguchi, H., Gotoh, K., Kobayashi, S., Kinoshita, M., et al. (2019). Exosomal miR-155 derived from hepatocellular carcinoma cells under hypoxia promotes angiogenesis in endothelial cells. Dig. Dis. Sci. 64 (3), 792–802. doi:10.1007/s10620-018-5380-1
Micalizzi, D. S., Christensen, K. L., Jedlicka, P., Coletta, R. D., Barón, A. E., Harrell, J. C., et al. (2009). The Six1 homeoprotein induces human mammary carcinoma cells to undergo epithelial-mesenchymal transition and metastasis in mice through increasing TGF-beta signaling. J. Clin. Investig. 119 (9), 2678–2690. doi:10.1172/JCI37815
Milman, N., Ginini, L., and Gil, Z. (2019). Exosomes and their role in tumorigenesis and anticancer drug resistance. Drug Resist Updat 45, 1–12. doi:10.1016/j.drup.2019.07.003
Mincheva-Nilsson, L., and Baranov, V. (2014). Cancer exosomes and NKG2D receptor-ligand interactions: impairing NKG2D-mediated cytotoxicity and anti-tumour immune surveillance. Semin. Cancer Biol. 28, 24–30. doi:10.1016/j.semcancer.2014.02.010
Neviani, P., Wise, P. M., Murtadha, M., Liu, C. W., Wu, C. H., Jong, A. Y., et al. (2019). Natural killer-derived exosomal miR-186 inhibits neuroblastoma growth and immune escape mechanisms. Cancer Res. 79 (6), 1151–1164. doi:10.1158/0008-5472.CAN-18-0779
Ono, H., Imoto, I., Kozaki, K., Tsuda, H., Matsui, T., Kurasawa, Y., et al. (2012). SIX1 promotes epithelial-mesenchymal transition in colorectal cancer through ZEB1 activation. Oncogene 31 (47), 4923–4934. doi:10.1038/onc.2011.646
Pan, S., Deng, Y., Fu, J., Zhang, Y., Zhang, Z., and Qin, X. (2022). N6-methyladenosine upregulates miR-181d-5p in exosomes derived from cancer-associated fibroblasts to inhibit 5-FU sensitivity by targeting NCALD in colorectal cancer. Int. J. Oncol. 60 (2), 14. doi:10.3892/ijo.2022.5304
Patton, M. C., Zubair, H., Khan, M. A., Singh, S., and Singh, A. P. (2020). Hypoxia alters the release and size distribution of extracellular vesicles in pancreatic cancer cells to support their adaptive survival. J. Cell. Biochem. 121 (1), 828–839. doi:10.1002/jcb.29328
Pu, Y., and Ji, Q. (2022). Tumor-associated macrophages regulate PD-1/PD-L1 immunosuppression. Front. Immunol. 13, 874589. doi:10.3389/fimmu.2022.874589
Qi, R., Bai, Y., Li, K., Liu, N., Xu, Y., Dal, E., et al. (2023). Cancer-associated fibroblasts suppress ferroptosis and induce gemcitabine resistance in pancreatic cancer cells by secreting exosome-derived ACSL4-targeting miRNAs. Drug Resist Updat 68, 100960. doi:10.1016/j.drup.2023.100960
Remon, J., Steuer, C. E., Ramalingam, S. S., and Felip, E. (2018). Osimertinib and other third-generation EGFR TKI in EGFR-mutant NSCLC patients. Ann. Oncol. 29 (suppl_1), i20–i27. doi:10.1093/annonc/mdx704
Ren, J., Ding, L., Zhang, D., Shi, G., Xu, Q., Shen, S., et al. (2018). Carcinoma-associated fibroblasts promote the stemness and chemoresistance of colorectal cancer by transferring exosomal lncRNA H19. Theranostics 8 (14), 3932–3948. doi:10.7150/thno.25541
Ren, R., Sun, H., Ma, C., Liu, J., and Wang, H. (2019b). Colon cancer cells secrete exosomes to promote self-proliferation by shortening mitosis duration and activation of STAT3 in a hypoxic environment. Cell. Biosci. 9, 62. doi:10.1186/s13578-019-0325-8
Ren, W., Hou, J., Yang, C., Wang, H., Wu, S., Wu, Y., et al. (2019a). Extracellular vesicles secreted by hypoxia pre-challenged mesenchymal stem cells promote non-small cell lung cancer cell growth and mobility as well as macrophage M2 polarization via miR-21-5p delivery. J. Exp. Clin. Cancer Res. 38 (1), 62. doi:10.1186/s13046-019-1027-0
Rezaie, J., Feghhi, M., and Etemadi, T. (2022). A review on exosomes application in clinical trials: perspective, questions, and challenges. Cell. Commun. Signal 20 (1), 145. doi:10.1186/s12964-022-00959-4
Sansone, P., Savini, C., Kurelac, I., Chang, Q., Amato, L. B., Strillacci, A., et al. (2017). Packaging and transfer of mitochondrial DNA via exosomes regulate escape from dormancy in hormonal therapy-resistant breast cancer. Proc. Natl. Acad. Sci. U. S. A. 114 (43), E9066–e9075. doi:10.1073/pnas.1704862114
Singh, S. S., Vats, S., Chia, A. Y. Q., Tan, T. Z., Deng, S., Ong, M. S., et al. (2018). Dual role of autophagy in hallmarks of cancer. Oncogene 37 (9), 1142–1158. doi:10.1038/s41388-017-0046-6
Song, Z., Jia, G., Ma, P., and Cang, S. (2021). Exosomal miR-4443 promotes cisplatin resistance in non-small cell lung carcinoma by regulating FSP1 m6A modification-mediated ferroptosis. Life Sci. 276, 119399. doi:10.1016/j.lfs.2021.119399
Sun, H., Meng, Q., Shi, C., Yang, H., Li, X., Wu, S., et al. (2021). Hypoxia-inducible exosomes facilitate liver-tropic premetastatic niche in colorectal cancer. Hepatology 74 (5), 2633–2651. doi:10.1002/hep.32009
Sun, Z., Yang, S., Zhou, Q., Wang, G., Song, J., Li, Z., et al. (2018). Emerging role of exosome-derived long non-coding RNAs in tumor microenvironment. Mol. Cancer 17 (1), 82. doi:10.1186/s12943-018-0831-z
Tian, T., Han, J., Huang, J., Li, S., and Pang, H. (2021). Hypoxia-induced intracellular and extracellular heat shock protein gp96 increases paclitaxel-resistance and facilitates immune evasion in breast cancer. Front. Oncol. 11, 784777. doi:10.3389/fonc.2021.784777
Tong, X., Tang, R., Xiao, M., Xu, J., Wang, W., Zhang, B., et al. (2022). Targeting cell death pathways for cancer therapy: recent developments in necroptosis, pyroptosis, ferroptosis, and cuproptosis research. J. Hematol. Oncol. 15 (1), 174. doi:10.1186/s13045-022-01392-3
Tu, X., Qin, B., Zhang, Y., Zhang, C., Kahila, M., Nowsheen, S., et al. (2019). PD-L1 (B7-H1) competes with the RNA exosome to regulate the DNA damage response and can Be targeted to sensitize to radiation or chemotherapy. Mol. Cell. 74 (6), 1215–1226. doi:10.1016/j.molcel.2019.04.005
van Niel, G., D’Angelo, G., and Raposo, G. (2018). Shedding light on the cell biology of extracellular vesicles. Nat. Rev. Mol. Cell. Biol. 19 (4), 213–228. doi:10.1038/nrm.2017.125
Vergani, E., Daveri, E., Vallacchi, V., Bergamaschi, L., Lalli, L., Castelli, C., et al. (2022). Extracellular vesicles in anti-tumor immunity. Semin. Cancer Biol. 86 (1), 64–79. doi:10.1016/j.semcancer.2021.09.004
Wang, H., Wang, L., Zhou, X., Luo, X., Liu, K., Jiang, E., et al. (2020c). OSCC exosomes regulate miR-210-3p targeting EFNA3 to promote oral cancer angiogenesis through the PI3K/AKT pathway. Biomed. Res. Int. 2020, 2125656. doi:10.1155/2020/2125656
Wang, M., Cai, Y., Peng, Y., Xu, B., Hui, W., and Jiang, Y. (2020b). Exosomal LGALS9 in the cerebrospinal fluid of glioblastoma patients suppressed dendritic cell antigen presentation and cytotoxic T-cell immunity. Cell. Death Dis. 11 (10), 896. doi:10.1038/s41419-020-03042-3
Wang, X., Zhang, H., Yang, H., Bai, M., Ning, T., Deng, T., et al. (2020a). Exosome-delivered circRNA promotes glycolysis to induce chemoresistance through the miR-122-PKM2 axis in colorectal cancer. Mol. Oncol. 14 (3), 539–555. doi:10.1002/1878-0261.12629
Wang, X., Zhou, Y., Dong, K., Zhang, H., Gong, J., and Wang, S. (2022b). Exosomal lncRNA HMMR-AS1 mediates macrophage polarization through miR-147a/ARID3A axis under hypoxia and affects the progression of hepatocellular carcinoma. Environ. Toxicol. 37 (6), 1357–1372. doi:10.1002/tox.23489
Wang, Z., He, J., Bach, D. H., Huang, Y. H., Li, Z., Liu, H., et al. (2022a). Induction of m(6)A methylation in adipocyte exosomal LncRNAs mediates myeloma drug resistance. J. Exp. Clin. Cancer Res. 41 (1), 4. doi:10.1186/s13046-021-02209-w
Wu, J., Gao, W., Tang, Q., Yu, Y., You, W., Wu, Z., et al. (2021a). M2 macrophage-derived exosomes facilitate HCC metastasis by transferring α(M) β(2) integrin to tumor cells. Hepatology 73 (4), 1365–1380. doi:10.1002/hep.31432
Wu, L., Xue, M., Lai, S., Chen, J., Lin, Y., Ding, N., et al. (2023). Hypoxia derived exosomes promote the proliferation and metastasis of colorectal cancer through the regulation of HIF-1α/miR-4299/ZBTB4. Life Sci. 329, 121872. doi:10.1016/j.lfs.2023.121872
Wu, X., Ren, Y., Yao, R., Zhou, L., and Fan, R. (2021b). Circular RNA circ-MMP11 contributes to lapatinib resistance of breast cancer cells by regulating the miR-153-3p/ANLN Axis. Front. Oncol. 11, 639961. doi:10.3389/fonc.2021.639961
Xi, L., Peng, M., Liu, S., Liu, Y., Wan, X., Hou, Y., et al. (2021). Hypoxia-stimulated ATM activation regulates autophagy-associated exosome release from cancer-associated fibroblasts to promote cancer cell invasion. J. Extracell. Vesicles 10 (11), e12146. doi:10.1002/jev2.12146
Xia, L., Oyang, L., Lin, J., Tan, S., Han, Y., Wu, N., et al. (2021). The cancer metabolic reprogramming and immune response. Mol. Cancer 20 (1), 28. doi:10.1186/s12943-021-01316-8
Xia, X., Wang, S., Ni, B., Xing, S., Cao, H., Zhang, Z., et al. (2020). Hypoxic gastric cancer-derived exosomes promote progression and metastasis via MiR-301a-3p/PHD3/HIF-1α positive feedback loop. Oncogene 39 (39), 6231–6244. doi:10.1038/s41388-020-01425-6
Xiang, X., Poliakov, A., Liu, C., Liu, Y., Deng, Z. b., Wang, J., et al. (2009). Induction of myeloid-derived suppressor cells by tumor exosomes. Int. J. Cancer 124 (11), 2621–2633. doi:10.1002/ijc.24249
Xie, H., Yao, J., Wang, Y., and Ni, B. (2022). Exosome-transmitted circVMP1 facilitates the progression and cisplatin resistance of non-small cell lung cancer by targeting miR-524-5p-METTL3/SOX2 axis. Drug Deliv. 29 (1), 1257–1271. doi:10.1080/10717544.2022.2057617
Xie, J. Y., Wei, J. X., Lv, L. H., Han, Q. F., Yang, W. B., Li, G. L., et al. (2020). Angiopoietin-2 induces angiogenesis via exosomes in human hepatocellular carcinoma. Cell. Commun. Signal 18 (1), 46. doi:10.1186/s12964-020-00535-8
You, X., Wang, Y., Meng, J., Han, S., Liu, L., Sun, Y., et al. (2021). Exosomal miR-663b exposed to TGF-β1 promotes cervical cancer metastasis and epithelial-mesenchymal transition by targeting MGAT3. Oncol. Rep. 45 (4), 12. doi:10.3892/or.2021.7963
Yu, S., Zhou, L., Fu, J., Xu, L., Liu, B., Zhao, Y., et al. (2022). H-TEX-mediated signaling between hepatocellular carcinoma cells and macrophages and exosome-targeted therapy for hepatocellular carcinoma. Front. Immunol. 13, 997726. doi:10.3389/fimmu.2022.997726
Yu, Y., Min, Z., Zhou, Z., Linhong, M., Tao, R., Yan, L., et al. (2019). Hypoxia-induced exosomes promote hepatocellular carcinoma proliferation and metastasis via miR-1273f transfer. Exp. Cell. Res. 385 (1), 111649. doi:10.1016/j.yexcr.2019.111649
Zhang, A., Xu, Y., Xu, H., Ren, J., Meng, T., Ni, Y., et al. (2021). Lactate-induced M2 polarization of tumor-associated macrophages promotes the invasion of pituitary adenoma by secreting CCL17. Theranostics 11 (8), 3839–3852. doi:10.7150/thno.53749
Zhang, H., Deng, T., Liu, R., Ning, T., Yang, H., Liu, D., et al. (2020). CAF secreted miR-522 suppresses ferroptosis and promotes acquired chemo-resistance in gastric cancer. Mol. Cancer 19 (1), 43. doi:10.1186/s12943-020-01168-8
Zhang, J., Qin, Y., Jiang, Q., Li, F., Jing, X., Cao, L., et al. (2022b). Glycopattern alteration of glycoproteins in gastrointestinal cancer cell lines and their cell-derived exosomes. J. Proteome Res. 21 (8), 1876–1893. doi:10.1021/acs.jproteome.2c00159
Zhang, X., Xu, Y., Ma, L., Yu, K., Niu, Y., Xu, X., et al. (2022a). Essential roles of exosome and circRNA_101093 on ferroptosis desensitization in lung adenocarcinoma. Cancer Commun. (Lond) 42 (4), 287–313. doi:10.1002/cac2.12275
Zhao, K., Wang, Z., Li, X., Liu, J. L., Tian, L., and Chen, J. Q. (2019). Exosome-mediated transfer of CLIC1 contributes to the vincristine-resistance in gastric cancer. Mol. Cell. Biochem. 462 (1-2), 97–105. doi:10.1007/s11010-019-03613-9
Zhao, L., Zhou, X., Xie, F., Zhang, L., Yan, H., Huang, J., et al. (2022). Ferroptosis in cancer and cancer immunotherapy. Cancer Commun. (Lond) 42 (2), 88–116. doi:10.1002/cac2.12250
Zheng, H., Guan, S., Wang, X., Zhao, J., Gao, M., and Zhang, X. (2020). Deconstruction of heterogeneity of size-dependent exosome subpopulations from human urine by profiling N-glycoproteomics and phosphoproteomics simultaneously. Anal. Chem. 92 (13), 9239–9246. doi:10.1021/acs.analchem.0c01572
Keywords: exosomes, drug resistance, tumor, immune escape, tumor environment
Citation: Ren B, Li X, Zhang Z, Tai S and Yu S (2024) Exosomes: a significant medium for regulating drug resistance through cargo delivery. Front. Mol. Biosci. 11:1379822. doi: 10.3389/fmolb.2024.1379822
Received: 31 January 2024; Accepted: 17 June 2024;
Published: 29 July 2024.
Edited by:
Yanwei Luo, Central South University, ChinaReviewed by:
Dwijendra K. Gupta, Allahabad University, IndiaMithlesh Kumar Temre, National Institute on Aging (NIH), United States
Copyright © 2024 Ren, Li, Zhang, Tai and Yu. This is an open-access article distributed under the terms of the Creative Commons Attribution License (CC BY). The use, distribution or reproduction in other forums is permitted, provided the original author(s) and the copyright owner(s) are credited and that the original publication in this journal is cited, in accordance with accepted academic practice. No use, distribution or reproduction is permitted which does not comply with these terms.
*Correspondence: Sheng Tai, dGFpc2hlbmcxOTczQDE2My5jb20=; Shan Yu, eXVzaGFuQGhyYm11LmVkdS5jbg==
†These authors have contributed equally to this work.