- 1Unit of Respiratory Medicine, Department of Experimental Medicine, University of Rome “Tor Vergata”, Rome, Italy
- 2Department of Medicine and Surgery, Respiratory Disease and Lung Function Unit, University of Parma, Parma, Italy
Asthma and chronic obstructive pulmonary disease are chronic respiratory disorders characterized by airways obstruction and chronic inflammation. Exacerbations lead to worsening of symptoms and increased airflow obstruction in both airways diseases, and they are associated with increase in local and systemic inflammation. Exosomes are cell-derived membrane vesicles containing proteins, lipids, and nucleic acids that reflect their cellular origin. Through the transfer of these molecules, exosomes act as mediators of intercellular communication. Via selective delivery of their contents to target cells, exosomes have been proved to be involved in regulation of immunity and inflammation. Although, exosomes have been extensively investigated in different diseases, little is currently known about their role in asthma and COPD pathogenesis, and particularly in exacerbations. This review aims to systemically assess the potential role of exosomes in asthma and COPD exacerbations.
Introduction
Asthma and chronic obstructive pulmonary disease (COPD) are both chronic respiratory disorders characterized by airways obstruction and chronic inflammation (Barnes, 2008). Despite substantial differences in airways inflammation between asthma and COPD, in both conditions a wide range of inflammatory cells and mediators are involved (Barnes et al., 1998; Barnes, 2004). Among these, exosomes, extracellular nanovesicles released in the airways from immune and structural cells, were found to play a potential role in the pathogenesis of asthma and COPD inflammation (Esser et al., 2010; Kesimer et al., 2009; Almqvist et al., 2008; Tan et al., 2017). Exosomes are nanosized vesicles of 30–150 nm in diameter, found in different tissues and fluids such as blood, saliva and bronchoalveolar lavage fluid (BALF) (Yu et al., 2021; Harding et al., 1983; Admyre et al., 2003). These extracellular vesicles (EVs), enclosed by a double lipid layer, are released from different cells, including immune cells, through the fusion of multivesicular endosomes with plasma membrane (Yu et al., 2021; Torregrosa Paredes et al., 2012; Théry et al., 2009a). Exosomes contain proteins, lipids, and nucleic acids (DNAs, mRNA, miRNAs and ncRNAs) which differ depending on their cellular origin (Yu et al., 2021; Caby et al., 2005). Through the transfer of these molecules to nearby cells, exosomes act as mediators of intercellular communication, inducing a modulation of the recipient cell function (Cardoso et al., 2016a; Lässer et al., 2011; Valadi et al., 2007). Moreover, exosomes have the ability to cross all the body barriers and to transfer their cargo to remote sites (Mirershadi et al., 2020). Via selective delivery of their contents to target cells, exosomes have been proved to be involved in regulation of physiological and pathological process, such as immunity and inflammation (Théry et al., 2009a; Lee et al., 2011; Beach et al., 2014).
Although, exosomes have been extensively investigated in different diseases, such as cancer and cardiovascular diseases (Rabinowits et al., 2009; Kuwabara et al., 2011), little is currently known about their role in asthma and COPD, and particularly in exacerbations. Exacerbations are characterized by worsening of symptoms in both asthma (shortness of breath, cough, wheezing or chest tightness) and COPD (dyspnea and/or cough and sputum) along with increased airflow obstruction (GOLD, 2024; GINA, 2024). In both airways diseases, exacerbations are related with increase in local and systemic inflammation (Wenzel, 2003; Celli et al., 2021).
EVs, including exosomes, have been identified as tools of intercellular communication, involved in lung homeostasis or response to pathological developments (Lo Cicero et al., 2015a). Thus, exploring exosomes involvement in immune and inflammatory process, including exacerbations, holds great potential to understand asthma and COPD pathogenesis and to identify important biomarkers for clinical application.
Therefore, the aim of this review is to systemically assess the potential role of exosomes in asthma and COPD exacerbations.
Materials and methods
Review question
The question of this systematic review was to assess whether exosomes could have a potential role in asthma and COPD exacerbations.
Search strategy
The protocol has been submitted to the international prospective register of systematic reviews (PROSPERO registration code: CRD42023483307) and performed in agreement with the Preferred Reporting Items for Systematic Reviews and Meta-Analyses Protocols (PRISMA-P) (Moher et al., 2015), with the relative flow diagram reported in Figure 1. This study satisfied all the recommended items reported by the PRISMA 2020 checklist (Page et al., 2021).
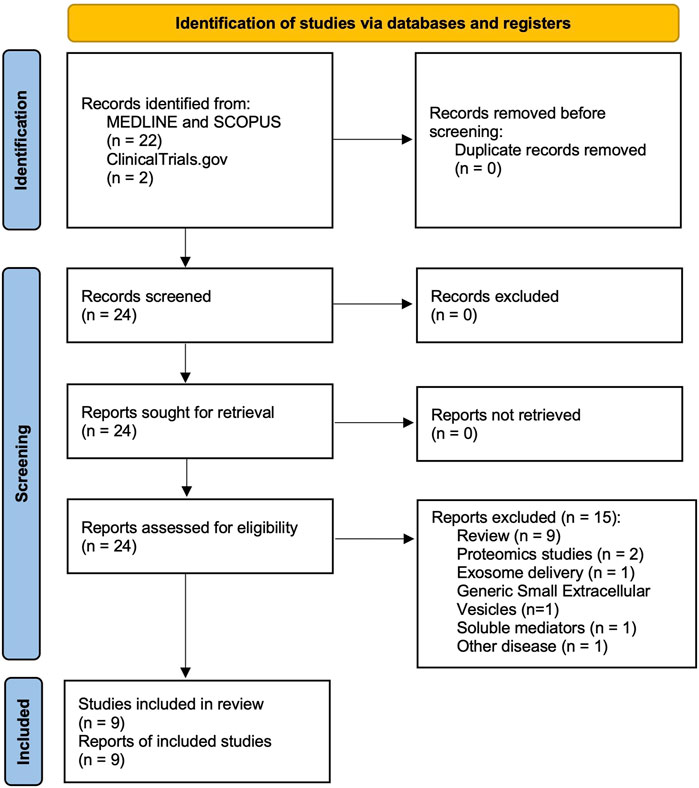
Figure 1. PRISMA 2020 flow diagram for the identification of the studies regarding the role of exosomes in exacerbations of asthma and COPD, resulting from databases (MEDLINE, SCOPUS) and registers (ClinicalTrials.gov) and included in the systematic review. COPD: chronic obstructive pulmonary disease. PRISMA: Preferred Reporting Items for Systematic Reviews and Meta-Analyses.
The PEO (Population, Exposure, and Outcome) framework was applied to develop the literature search strategy and question, as previously reported (Moola et al., 2015). The “Population” included asthma and COPD; the “Exposure” regarded asthma and COPD exacerbations; the assessed “Outcome” was exosomes.
A comprehensive literature search was performed for research studies, written in English, and investigating the role of exosomes in exacerbations of asthma and COPD. The search was performed in MEDLINE, Scopus, and ClinicalTrials.gov, to identify relevant studies available with no time limit up to 6 September 2023.
The string used for the search in MEDLINE, Scopus, and ClinicalTrials.gov was as follows: “exosomes AND (COPD OR asthma) AND exacerbations”.
Literature search results were uploaded to Eppi-Reviewer 4 (EPPI-Centre Software. London, United Kingdom), a web-based software program for managing and analysing data in literature reviews that facilitates collaboration among reviewers during the study selection process.
Study selection
Research studies reporting results concerning the role of exosomes in asthma and COPD exacerbations were included in the systematic review.
Two reviewers (L.C. and R.L.) independently checked the relevant studies identified from MEDLINE, Scopus, and ClinicalTrials.gov. The studies were selected in agreement with previously mentioned criteria, and any difference in opinion about eligibility was resolved by consensus.
Data extraction
Data from included studies were extracted and checked for study year and references, PMID or ClinicalTrials.gov identifier, study and subjects’ characteristics, outcomes, main results, and study quality assessment via the Jadad Score (Jadad et al., 1996).
Endpoints
The endpoint of this systematic review was to evaluate the potential role of exosomes in asthma and COPD exacerbations.
Strategy for data synthesis
Data from original papers were extracted and reported via qualitative synthesis. Statistical significance was identified for p < 0.05.
Quality score
The risk of bias for included clinical studies was analyzed via the Jadad score (Jadad et al., 1996). The Jadad score, with a scale of 1–5 (score of 5 being the best quality), was used to assess the quality of the papers concerning the likelihood of bias related with randomisation, double blinding, withdrawals, and dropouts (Jadad et al., 1996). Studies were considered of low quality at Jadad score <3, of medium quality at Jadad score = 3, and of high quality at Jadad score >3.
Two reviewers (L.C. and R.L.) independently assessed the quality of studies, and any difference in opinion about the quality score was resolved by consensus.
Results
Study characteristics
Of the 24 potentially relevant records screened in MEDLINE, Scopus, and ClinicalTrials.gov, 9 studies were deemed eligible for a qualitative analysis. This systematic review included data obtained from studies investigating the role of exosomes in asthma and COPD exacerbations.
Seven studies (Tan et al., 2017; Yu et al., 2021; Torregrosa Paredes et al., 2012; Meng et al., 2022; Jiang et al., 2023; Song et al., 2020; Wang et al., 2022) were published in full text articles, and 2 studies (ClinicalTrials.gov, 2023b; ClinicalTrials.gov, 2023a) were available only on ClinicalTrials.gov. For 3 of the included studies (Tan et al., 2017; Torregrosa Paredes et al., 2012; Wang et al., 2022) Jadad score was suitable for quality assessment. All were considered of low quality (Jadad score = 0). The main characteristics of the studies included in the systematic review are summarized in Table 1.
Role of exosomes in COPD exacerbations
In 2017, a study conducted by Tan et al. (Tan et al., 2017) described a correlation between the level of circulating exosomes and the levels of plasma biomarkers of systemic inflammation in COPD patients. Circulating exosomes, identified as CD9+ macrovesicles, and plasmatic systemic inflammation biomarkers, such as C-reactive protein (CRP), soluble tumor necrosis factor receptor-1 (sTNFR1) and interleukin (IL)-6, were quantified in patients with acute exacerbation of COPD (n = 20) or stable COPD (n = 20), and non-smoking healthy controls (n = 20). Plasma exosomes were significantly higher in patients with exacerbations (p < 0.001) and stable COPD patients (p < 0.05) compared to healthy controls. A numerical increase in plasma exosomes level was described in patients with exacerbations compared to stable COPD patients. Moreover, the level of circulating exosomes correlated with plasma levels of CRP (p < 0.001), sTNFR1 (p < 0.01), and IL-6 (p < 0.01).
Higher levels of plasma CRP and sTNFR1 were observed in patients with exacerbations compared to stable COPD patients (p < 0.01 and p < 0.05, respectively) and healthy controls (p < 0.001 for both correlation). In stable COPD patients, plasma CRP was higher than in healthy controls (p < 0.001). Also IL-6 plasma level were higher in patients with exacerbations and stable COPD patients, compared to healthy controls (p < 0.05 and p < 0.01, respectively).
Overall, the study by Tan et al. (Tan et al., 2017) suggested that circulating exosomes were elevated in both stable COPD and in patients with COPD exacerbations, and a correlation with systemic inflammatory biomarkers was demonstrated.
In 2022, Meng et al. (Meng et al., 2022) described, in an in vitro and in vivo PM2.5 exposure models, the effect of PM2.5 on exosomal hsa_circ_0005045. PM2.5, an ambient fine particulate matter, has been associated with higher prevalence of COPD among non-smoking subjects (Wang et al., 2018). The study showed that PM2.5 induced and upregulated the exosomal circRNA hsa_circ_0005045. This molecule, through the binding to the exosomal protein peroxiredoxin2 (PRDX2), induced elastase (ELANE) and tumor necrosis factor (TNF)-α release by inflammatory cells, thus exacerbating airways inflammatory response.
A cohort of 83 males and 15 females, diagnosed with stable mild-to-moderate COPD, was enrolled. A pair of blood samples, pre-exposure and post-exposure, was collected from the same subjects at 10–14 days after an air pollution episode (daily PM2.5 > 75 μg m− 3). Eight matched blood samples from this cohort were used for circRNA microarray analysis and exosomes were isolated from blood before and after PM2.5 exposure.
Healthy donors matched with sex, age, and smoking status were enrolled. Peripheral blood samples were collected during the stable phase in COPD patients and from healthy donors. Non-smoking was defined as smoking cessation for at least 1 year, and smokers were considered as subjects currently smoking or as having smoked at least 100 cigarettes in one’s lifetime.
Concerning in vivo murine model, male C57BL/6 mice treated group received PM2.5 for 2 weeks leading to COPD-like lesions after exposure. Mice control group received high-efficiency particulate air-filtered room air (FRA) at the same flow rate.
CircRNAs microarray analysis showed that the levels of hsa_circ_0005045 in the plasma of COPD patients were significantly elevated compared to healthy controls. From circRNAs microarray analysis, performed on plasma samples of non-smoking COPD patients who experienced exacerbation after PM2.5 ambient exposure, 111 upregulated circRNAs and 69 downregulated circRNAs were detected. Quantitative Reverse Transcription Polymerase Chain Reaction (qRT-PCR) was performed to confirm the upregulation of 6 specific circRNAs, including hsa_circ_0005045. Four of these circRNAs were associated with the “enrichment of extracellular exosomes” category, suggesting a correlation between circRNAs and exosomes. Analysis of the expression levels of 4 candidate exosomal circRNAs in 98 matched samples revealed that only hsa_circ_0005045 showed a significant upregulation in exosomes following exposure to PM2.5. hsa_circ_0005045 potentially interacted in a complex with PRDX2 and neutrophil ELANE within exosomes. Both, PRDX2 and ELANE exhibited elevated plasma concentrations following PM2.5 exposure, and these changes correlated with the levels of hsa_circ_0005045. Furthermore, hsa_circ_0005045 expression increased in exosomes derived from both bronchial and alveolar epithelial cells after in vitro exposure to PM2.5.
A second cohort of 327 retirees diagnosed with COPD and divided in ABCD group according to GOLD 2017 (Barnes, 2017), was recruited to predict the risk of COPD acute exacerbations, by using a machine learning model. This model showed that COPD patients sensitive to PM2.5 exposure were non-smoking, group C, and more likely to express higher levels of exosomal hsa_circ_0005045.
The function of hsa_circ_0005045 was further investigated in vivo, in a murine model with COPD-like lesions induced by PM2.5 inhalation. The levels of a circRNA homologous to hsa_circ_0005045 were consistently and significantly elevated after 7, 14, and 28 days of PM2.5 inhalation in COPD-like murine BALF and plasma-derived exosomes. Moreover, these exosomes, isolated from PM2.5-inhaled mice, induced hallmarks of COPD and increased plasma levels of hsa_circ_0005045 homologous in healthy murine lungs. After PM2.5 exposure, a significantly increase of PRDX2, inflammatory cells, and TNF-α was observed in lung tissues of both exposed and control mice, suggesting a potential contribution of the hsa_circ_0005045 homologous enriched exosomes to induce hallmarks of COPD after PM2.5 exposure.
Recently, Jiang et al. (Jiang et al., 2023) conducted a preclinical study, in a mouse model of COPD, with the aim to investigate the role of serum exosome-derived miR-7 in the pathogenesis of COPD. miR-7 is a MicroRNAs considered a COPD biomarker, significantly upregulated in the serum of COPD patients (Akbas et al., 2012). The study showed that the level of miR-7 was significantly (p < 0.0001) increased in exosomes derived from COPD mice and in lung tissue macrophages (p < 0.01). Furthermore, COPD-exosomes induced an inflammatory response in the lungs of control mice. Lung tissue macrophages were found to be increased and showed elevated inducible nitric oxide synthase (iNOS) and decreased Arg1 levels, indicating a shift towards M1 macrophage polarization (iNOS, p < 0.0001; Arg1, p < 0.001). Moreover, pro-inflammatory cytokine levels secreted by macrophages showed significant increases (IL-6, p < 0.0001; TNF-α, p < 0.0001). The study also tested the effect of miR-7 inhibitor that blocked M1 polarization of macrophages and reduced IL-6 and TNF-α secretion, confirming miR-7 involvement in macrophage activation and differentiation.
Overall, the study showed that elevated miR-7 levels in the serum of COPD mice could play a role in COPD exacerbation by promoting M1 polarization in lung macrophages.
A prospective cohort study, available only in ClinicalTrials.gov (NCT04183530), aimed to perform a comprehensive characterization of COPD, through multidimensional data, including exosomes evaluation (ClinicalTrials.gov, 2023b). However, no results are still available.
Role of exosomes in asthma exacerbations
In a study by Torregrosa Paredes et al. (Torregrosa Paredes et al., 2012), phenotypical and functional characteristics of BALF exosomes were investigated in asthmatic (n = 12) and healthy (n = 13) subjects. BALF exosomes were collected from mild allergic asthmatic patients, with birch pollen specific IgE (>2 kU/l), before and 24 h after birch allergen provocation. BALF exosomes from asthmatics showed an altered phenotypic profile, compared to healthy controls, even before allergen challenge. More specifically, higher levels of the surface molecules, such as tetraspanins CD81 and CD63, and HLA-DR were observed in BALF exosomes from asthmatic patients, compared to healthy controls. The scavenger receptor CD36, known to have a function in bacterial recognition (Baranova et al., 2008), and potentially implicated in asthma exacerbations in response to bacterial infections, was higher expressed by BALF exosomes from asthmatics, compared to healthy controls. No phenotypic changes were induced by allergen provocation, except for MUC1 that decreased after challenge. Both BALF exosomes from healthy controls and asthmatics expressed leukotriene A4 hydrolase (LTA4H), leukotriene C4 synthase (LTC4S), FLAP, and 15-LO-1 that are responsible of LTA4 conversion to LTB4 and LTC4. Furthermore, after 48 h incubation, BALF exosomes of asthmatics induced significantly higher production of LTs and IL-8 in bronchial epithelial cells (BEC), compared to healthy controls, with no difference after allergen challenge. Only a modest increase in IL-6 production in BEC was observed, but with no significant differences between asthmatics and healthy controls.
The evidence of a pro-inflammatory altered exosome profile led to hypothesize a potential role of BALF exosomes in asthmatic inflammation.
In a preclinical mice model of asthma, Yu et al. (Yu et al., 2021) observed that ovalbumin (OVA) challenge of airways epithelial cells (AECs) induced the release of a major number of exosomes, compared to PBS-challenged AECs. OVA-challenged AEC-derived exosomes (OAEs) presented a different protein composition compared to PBS-treated AEC-derived exosomes (PAEs). More specifically, PLXNB2, a CD100 ligand, was the most expressed protein in OAEs and was found to be increased in BALF exosomes from asthmatic mice compared to controls. The proteolytic cleavage of macrophages CD100, mediated by OAEs MMP14, promoted pro-inflammatory responses in the airways, suggesting a potential mechanism of OAE-mediated asthma exacerbations. Interaction between CD100 and PLXNB2 allowed to internalize OAEs, representing a trigger to the transcription of pro-inflammatory chemokines and cytokines. Thus, OAEs were found to be able to increase airways hyper-responsiveness (AHR) and to induce the infiltration or activation of macrophages, neutrophils, and eosinophils in the airways. These observations highlighted the complex interaction between AECs and innate immune cells, powered by exosomes, in the pathogenesis of asthma.
Conversely, a preclinical study conducted by Song et al. (Song et al., 2020), evidenced the contribution of mesenchymal stem cell (MSC)-derived exosomes in the inhibition of chronic allergic inflammation, airways remodeling, and epithelial-mesenchymal transition (EMT) of airways epithelium in an asthma rat model. MSCs and MSC-derived exosomes significantly reduced inflammatory cells, such as lymphocytes, eosinophils, and neutrophils in OVA-sensitized and challenged rats’ airways. Moreover, treatment with MSCs and MSC-derived exosomes significantly reduced the proliferation of goblet cells and collagen deposition. All these effects were supposed to be mediated by exosomes highly expressed miRNAs affecting the expression of key proteins of the Wnt/β-catenin signaling pathway, implicated in EMT and airways remodeling. Indeed, treatment of rat model with BML-284, a small molecule agonist of the Wnt/β-catenin signaling pathway, reversed the downregulation of this pathway in airways epithelium, induced by MSCs and MSC-derived exosomes, exacerbating airways remodeling.
Recently, Wang et al. (Wang et al., 2022) conducted a longitudinal bi-phasic case-control study to characterize the profile of circulating exosomal microRNAs (ExoMiRNAs) in mild asthmatic patients and healthy controls, after in vivo rhinovirus (RV) challenge. RV is a common-cold-causing respiratory virus, notably cause of asthma exacerbations (Gern and Busse, 1999). Mild allergic asthmatic patients (n = 12) and matched non atopic healthy controls (n = 12) were recruited. Serum samples were collected before and after RV challenge. Inflammatory markers were also evaluated, including cytokines, chemokines, eosinophils, and neutrophils in nasal lavage. Furthermore, fractional exhaled nitric oxide (FeNO) and pulmonary functions parameters were measured. At baseline, no difference in miRNA expression was observed between asthmatics and healthy controls. After the RV challenge, a total of 26 ExoMiRNAs were differentially expressed (DE) between asthmatics and healthy controls. Expression of these 26 DE ExoMiRNAs not only was different before and after RV challenge in asthmatics, but it was differentiated also from miRNA expression in healthy controls, after RV challenge. Among these miRNAs, two clusters were identified. The Upregulated Cluster miRNAs, that significantly correlated with Th1 and regulatory cytokine, and Downregulated Cluster miRNAs, that significantly correlated with pulmonary function measurements, inflammatory biomarkers, and Th2 and Th17 cytokine groups. Overall, the study described the regulatory roles of ExoMiRNAs in cytokine-mediated immune response, in RV-exacerbated asthma.
A randomized study, available only in ClinicalTrials.gov (NCT04542902), was designed to perform a characterization of eosinophils derived exosomes in asthmatic and severe asthmatic patients, with the aim to investigate the role of different eosinophils subtypes in asthma pathogenesis and to further delineate asthma phenotypes (ClinicalTrials.gov, 2023a). To date, no results are still available.
Discussion
Exosomes are extracellular nanosized vesicles released from different cell types, following the fusion of multivesicular endosomes with plasma membrane (Théry et al., 2009b). They are physiologically released but also during cellular activation, senescence, and apoptosis (Lo Cicero et al., 2015a). These cell-derived membrane vesicles are enclosed by a lipid bilayer and contain proteins, lipids, and nucleic acids. Through the transfer of these macromolecules, exosomes act as mediators of intercellular communication (Cardoso et al., 2016a). Via the activation of different signaling cascades, exosomes also play a pivotal role in inflammation process (Console et al., 2019). Exosomes are likely to be involved in modulation of inflammation also in asthma and COPD (Esser et al., 2010; Kesimer et al., 2009; Almqvist et al., 2008; Tan et al., 2017) (Figure 2). Evidence that exosomes participate to the modulation of airways inflammatory process through the release of exosomal molecules, such as nucleic acids, arise mostly from preclinical studies in asthma and COPD models. More specifically, a study conducted using both in vitro and in vivo PM2.5 exposure models demonstrated that PM2.5 induced and upregulated the circRNA hsa_circ_0005045 in exosomes derived from plasma and from bronchial and alveolar epithelial cells of COPD patients. Moreover, in a murine COPD model, the homologous of exosomal circRNA hsa_circ_0005045, binding to exosomal PRDX2, caused the release of TNF-α by inflammatory cells in lung tissue (Meng et al., 2022).
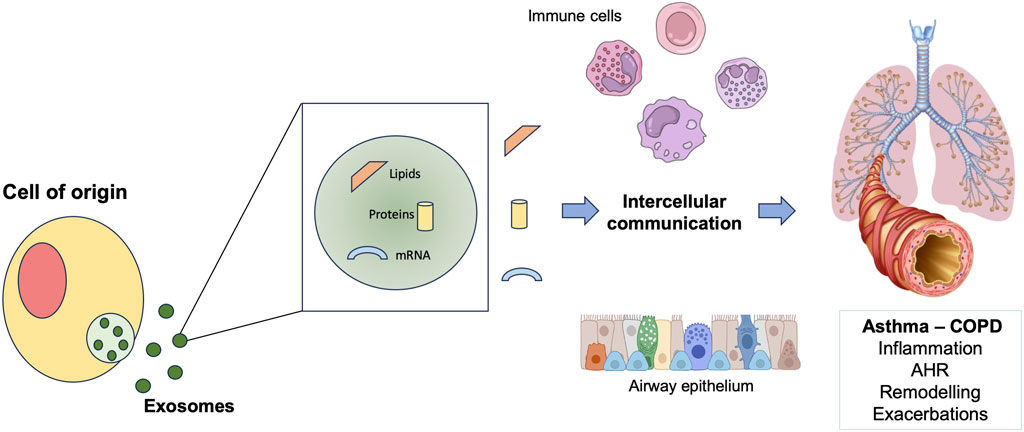
Figure 2. Schematic representation of the role of exosomes in exacerbations of asthma and COPD. COPD: chronic obstructive pulmonary disease; AHR: airway hyperresponsiveness.
A further preclinical study, in a mouse model of COPD, proved that exosome-derived miR-7 was significantly increased in exosomes from COPD mice and was found to be involved in macrophage activation and differentiation in mice lung tissue, leading to exacerbation of local inflammatory response (Jiang et al., 2023).
Phenotypical and functional exosomes characteristics were described both in asthmatic models and patients, confirming exosomes implication in the modulation of airways inflammation in asthma, including a potential mechanism of exosomes-mediated asthma exacerbations. In a preclinical mice model of asthma, AECs released a higher number of exosomes with a peculiar protein composition, after OVA challenge. Internalization of OAEs, after CD100- PLXNB2 interaction, and proteolytic CD100 cleavage, mediated by OAEs via MMP14, triggered pro-inflammatory responses in the airways, promoting infiltration of inflammatory cells, transcription of pro-inflammatory chemokines and cytokines, and increased AHR (Yu et al., 2021). Phenotypic characteristics of asthmatics BALF exosomes were further investigated before and after allergen provocation. Asthmatics BALF exosomes showed higher levels of tetraspanins CD81 and CD63, of HLA-DR, and CD36, a scavenger receptor with a potential role in asthma exacerbations in response to bacterial infections, compared to healthy controls. Allergen provocation did not induce any exosomes phenotypic changes. Moreover, asthmatics BALF exosomes induced significantly higher production of LTs and IL-8 in BEC, compared to healthy controls (Torregrosa Paredes et al., 2012).
Conversely, in an asthma rat model, MSC-derived exosomes inhibited chronic allergic inflammation, airways remodeling, and EMT of airways epithelium. These exosomes showed high levels of miRNAs affecting the Wnt/β-catenin signaling pathway, known to be implicated in EMT and airways remodeling (Song et al., 2020).
Two clinical studies dealt specifically with the potential role of exosomes in asthma and COPD exacerbations. A clinical trial in COPD patients showed that higher levels of circulating exosomes were detectable in patients with COPD exacerbations and in stable COPD patients, compared to healthy controls. A numerical increase in plasma exosomes level was described in patients with exacerbations compared to stable COPD patients. Besides, a correlation between circulating exosomes and systemic inflammatory biomarkers was highlighted (Tan et al., 2017).
Recently, an observational, longitudinal bi-phasic case-control study evaluated the profile of circulating exosomal MiRNAs (ExoMiRNAs) in mild asthmatic patients and healthy controls, after in vivo RV challenge, providing evidence on regulatory roles of ExoMiRNAs in cytokine-mediated immune response, in RV-exacerbated asthma (Wang et al., 2022) (Figure 3).
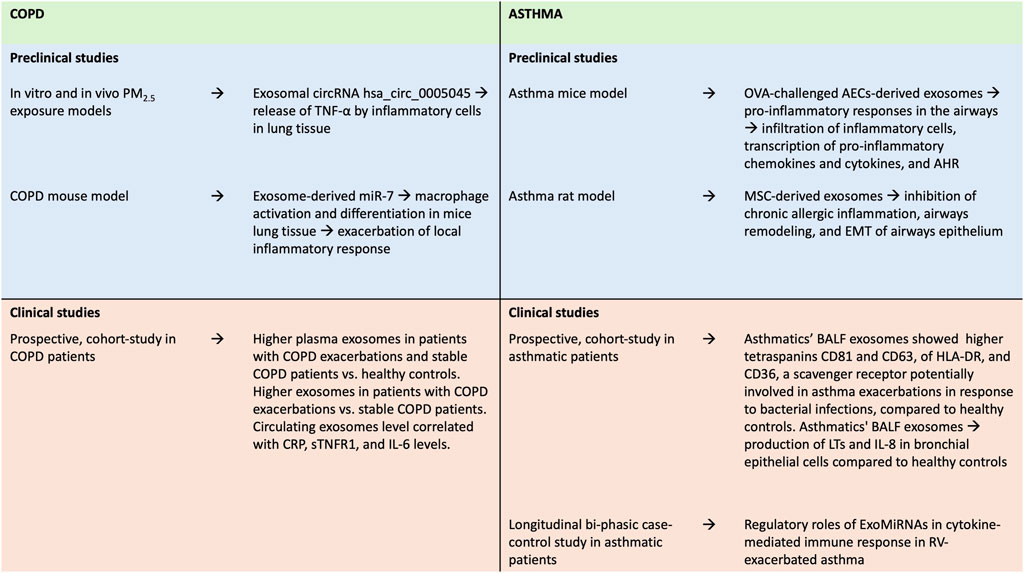
Figure 3. Summary of findings from preclinical and clinical studies on the role of exosomes in asthma and COPD. AECs, airways epithelial cells; AHR, airway hyperresponsiveness; BALF, bronchoalveolar lavage fluid; COPD, Chronic Obstructive Pulmonary Disease; CRP, C-reactive protein; EMT, epithelial-mesenchymal transition; IL-6, Interleukin-6; IL-8, Interleukin-8; LTs, leukotrienes; MSC: mesenchymal stem cell; OVA, ovalbumin; RV, rhinovirus; sTNFR, soluble Tumor Necrosis Factor Receptor-1; TNF-α, Tumor Necrosis Factor-α.
Overall, this evidence suggests that, depending on their origin, exosomes promote inflammation via regulating the function of immune cells through their recruitment, activation, or differentiation (Engeroff and Vogel, 2022). Conversely, exosomes derived from MSCs showed regenerative proprieties in lung tissues, leading to hypothesize their potential role in attenuate remodeling in chronic lung diseases (Lener et al., 2015). Thus, cellular origin and cargo induce exosomes’ specific activity under pathological conditions (Chaput et al., 2006; Kowal and Tkach, 2019). However, current knowledge is still lacking and the process inducing pro-inflammatory or anti-inflammatory exosomes activity are under investigations (Rajabi et al., 2022).
Because of the origin from different cells type and the multitude of molecules expressed, exosomes are largely studied as an emerging class of easily accessible biomarkers (Yáñez-Mó et al., 2015; Srinivasan et al., 2016). Indeed, exosomes could provide information about their origin microenvironment, potentially useful to the diagnosis and prognosis of the diseases (Xu et al., 2016). One of the most attractive aspects of exosomes, and particularly of sputum and BALF derived exosomes, is to exploit the longitudinal sampling to monitor diseases progression (Kalluri and LeBleu, 2020). Moreover, due to expression of molecules closely linked to the pathogenesis and phenotype of specific disorders, exosomes represent a promising tool for early disease diagnosis and personalized therapy (Purghè et al., 2021).
Exosomes are characterized by considerable stability in extracellular environment, because of the lipidic bilayer, suggesting their potential application, not only as biomarkers but also as therapeutics agents (Mathis et al., 2021; Kadota et al., 2016). Engineered exosomes showed low immunogenicity and toxicity and could be able to deliver both lipophilic and hydrophilic drugs, with a preserved activity, to target cells (Raimondo et al., 2019).
Exosomes miRNA expression profile is also under investigation for diagnostic and therapeutic purposes in inflammatory respiratory disease, and targeting exosomes derived miRNA could represent a potential therapeutic strategy (Gon et al., 2020).
However, clinical application of exosomes is limited by several factors, including insufficient knowledge regarding dosage, route, timing of administration, and potential side effects. Furthermore, methods for exosomes’ isolation and purification have not been standardized, resulting in heterogeneity in exosome populations and content (Rezabakhsh et al., 2021). Currently available technologies are not able to identify exosomes secreted by specific cells within the vast number of exosomes present in body fluids. Moreover, the mechanisms of interaction between exosomes and recipient cells are not fully understood, representing a risk for potential side effects (Kadota et al., 2016). Also, the ability of exosomes to cross natural barriers (Mirershadi et al., 2020) could represent a potential risk for the uncontrolled dissemination of their contents.
An intrinsic limitation of the present systematic review is linked to limited research on the topic, therefore primary studies included in the review were heterogeneous and evidences emerged mainly from preclinical trials. Only 3 of the studies included in the present systematic review were clinical trials and were considered of low quality according to the Jadad score (Jadad score = 0).
Overall, while the biological impact of exosomes in the pathogenesis of inflammation in respiratory diseases has been widely addressed, their role in asthma and COPD exacerbations is still under investigations.
Future challenges include to investigate the cellular origin of circulating exosomes in respiratory diseases and exploring their proteomics and metabolomics content to better understand their role in acute flare-up. Randomized controlled trials, recruiting selected population, are needed to better understand the role of exosomes in exacerbations of chronic obstructive diseases.
Author contributions
RL: Data curation, Investigation, Methodology, Visualization, Writing–original draft, Writing–review and editing. LC: Conceptualization, Data curation, Funding acquisition, Investigation, Methodology, Project administration, Resources, Software, Supervision, Validation, Visualization, Writing–original draft, Writing–review and editing. EM: Writing–original draft, Writing–review and editing. EP: Writing–original draft, Writing–review and editing. PR: Conceptualization, Data curation, Funding acquisition, Investigation, Methodology, Project administration, Resources, Software, Supervision, Validation, Visualization, Writing–original draft, Writing–review and editing.
Funding
The author(s) declare that no financial support was received for the research, authorship, and/or publication of this article.
Conflict of interest
The authors declare that the research was conducted in the absence of any commercial or financial relationships that could be construed as a potential conflict of interest.
Publisher’s note
All claims expressed in this article are solely those of the authors and do not necessarily represent those of their affiliated organizations, or those of the publisher, the editors and the reviewers. Any product that may be evaluated in this article, or claim that may be made by its manufacturer, is not guaranteed or endorsed by the publisher.
References
Admyre, C., Grunewald, J., Thyberg, J., Gripenbäck, S., Tornling, G., Eklund, A., et al. (2003). Exosomes with major histocompatibility complex class II and co-stimulatory molecules are present in human BAL fluid. Eur. Respir. J. 22 (4), 578–583. doi:10.1183/09031936.03.00041703
Akbas, F., Coskunpinar, E., Aynaci, E., Müsteri Oltulu, Y., and Yildiz, P. (2012). Analysis of serum micro-RNAs as potential biomarker in chronic obstructive pulmonary disease. Exp. Lung Res. 38 (6), 286–294. doi:10.3109/01902148.2012.689088
Almqvist, N., Lönnqvist, A., Hultkrantz, S., Rask, C., and Telemo, E. (2008). Serum-derived exosomes from antigen-fed mice prevent allergic sensitization in a model of allergic asthma. Immunology 125 (1), 21–27. doi:10.1111/j.1365-2567.2008.02812.x
Baranova, I. N., Kurlander, R., Bocharov, A. V., Vishnyakova, T. G., Chen, Z., Remaley, A. T., et al. (2008). Role of human CD36 in bacterial recognition, phagocytosis, and pathogen-induced JNK-mediated signaling. J. Immunol. 181 (10), 7147–7156. doi:10.4049/JIMMUNOL.181.10.7147
Barnes, P. J. (2004). Mediators of chronic obstructive pulmonary disease. Pharmacol. Rev. 56 (4), 515–548. doi:10.1124/pr.56.4.2
Barnes, P. J. (2008). Immunology of asthma and chronic obstructive pulmonary disease. Nat. Rev. Immunol. 8 (3), 183–192. doi:10.1038/nri2254
Barnes, P. J. (2017). GOLD 2017: a new report. Chest 151 (2), 245–246. doi:10.1016/j.chest.2016.11.042
Barnes, P. J., Fan Chung, K., and Page, C. P. (1998). Inflammatory mediators of asthma: an update. Pharmacol. Rev. 50 (4), 515–596.
Beach, A., Zhang, H. G., Ratajczak, M. Z., and Kakar, S. S. (2014). Exosomes: an overview of biogenesis, composition and role in ovarian cancer. J. Ovarian Res. 7 (1), 14. doi:10.1186/1757-2215-7-14
Caby, M. P., Lankar, D., Vincendeau-Scherrer, C., Raposo, G., and Bonnerot, C. (2005). Exosomal-like vesicles are present in human blood plasma. Int. Immunol. 17 (7), 879–887. doi:10.1093/intimm/dxh267
Cardoso, A. L., Guedes, J. R., and De Lima, M. C. P. (2016a). Role of microRNAs in the regulation of innate immune cells under neuroinflammatory conditions. Curr. Opin. Pharmacol. 26, 1–9. doi:10.1016/j.coph.2015.09.001
Celli, B. R., Fabbri, L. M., Aaron, S. D., Agusti, A., Brook, R., Criner, G. J., et al. (2021). An updated definition and severity classification of chronic obstructive pulmonary disease exacerbations: the rome proposal. Am. J. Respir. Crit. Care Med. 204 (11), 1251–1258. doi:10.1164/rccm.202108-1819PP
Chaput, N., Flament, C., Viaud, S., Taieb, J., Roux, S., Spatz, A., et al. (2006). Dendritic cell derived-exosomes: biology and clinical implementations. J. Leukoc. Biol. 80 (3), 471–478. doi:10.1189/JLB.0206094
ClinicalTrials.gov (2023a). Study details | non-coding RNAs analysis of eosinophil subtypes in asthma. Available at: https://clinicaltrials.gov/study/NCT04542902?cond=Asthma%20OR%20COPD&term=exosome%20and&rank=2.
ClinicalTrials.gov (2023b). Study details | the individualized accurate diagnosis and treatment of chronic objective pulmonary disease(COPD) patients based on multidimensional data. Available at: https://clinicaltrials.gov/study/NCT04183530?cond=Asthma%20OR%20COPD&term=exosome%20and&rank=1.
Console, L., Scalise, M., and Indiveri, C. (2019). Exosomes in inflammation and role as biomarkers. Clin. Chim. Acta 488, 165–171. doi:10.1016/J.CCA.2018.11.009
Engeroff, P., and Vogel, M. (2022). The potential of exosomes in allergy immunotherapy. Vaccines (Basel) 10 (1), 133. doi:10.3390/VACCINES10010133
Esser, J., Gehrmann, U., D'Alexandri, F. L., Hidalgo-Estévez, A. M., Wheelock, C. E., Scheynius, A., et al. (2010). Exosomes from human macrophages and dendritic cells contain enzymes for leukotriene biosynthesis and promote granulocyte migration. J. Allergy Clin. Immunol. 126 (5), 1032–1040. doi:10.1016/j.jaci.2010.06.039
Gern, J. E., and Busse, W. W. (1999). Association of rhinovirus infections with asthma. Clin. Microbiol. Rev. 12 (1), 9–18. doi:10.1128/CMR.12.1.9
GINA (2024). GINA main report - global initiative for asthma - GINA. Available at: https://ginasthma.org/wp-content/uploads/2024/05/GINA-2024-Strategy-Report-24_05_22_WMS.pdf (Accessed June 06, 2024).
GOLD (2024). GOLD report - global initiative for chronic obstructive lung disease - GOLD. Available at: https://goldcopd.org/2024-gold-report/, https://goldcopd.org/wp-content/uploads/2024/02/GOLD-2024_v1.2-11Jan24_WMV.pdf (Accessed June 06, 2024).
Gon, Y., Shimizu, T., Mizumura, K., Maruoka, S., and Hikichi, M. (2020). Molecular techniques for respiratory diseases: MicroRNA and extracellular vesicles. Respirology 25 (2), 149–160. doi:10.1111/RESP.13756
Harding, C., Heuser, J., and Stahl, P. (1983). Receptor-mediated endocytosis of transferrin and recycling of the transferrin receptor in rat reticulocytes. J. Cell Biol. 97 (2), 329–339. doi:10.1083/jcb.97.2.329
Jadad, A. R., Moore, R. A., Carroll, D., Jenkinson, C., Reynolds, D. J., Gavaghan, D. J., et al. (1996). Assessing the quality of reports of randomized clinical trials: is blinding necessary? Control Clin. Trials 17 (1), 1–12. doi:10.1016/0197-2456(95)00134-4
Jiang, Y., Wang, J., Zhang, H., Min, Y., and Gu, T. (2023). Serum exosome-derived MiR-7 exacerbates chronic obstructive pulmonary disease by regulating macrophage differentiation. Iran. J. Public Health 52 (3), 563–574. doi:10.18502/IJPH.V52I3.12139
Kadota, T., Fujita, Y., Yoshioka, Y., Araya, J., Kuwano, K., and Ochiya, T. (2016). Extracellular vesicles in chronic obstructive pulmonary disease. Int. J. Mol. Sci. 17 (11), 1801. doi:10.3390/IJMS17111801
Kalluri, R., and LeBleu, V. S. (2020). The biology, function, and biomedical applications of exosomes. Science 367 (6478), eaau6977. doi:10.1126/SCIENCE.AAU6977
Kesimer, M., Scull, M., Brighton, B., DeMaria, G., Burns, K., O'Neal, W., et al. (2009). Characterization of exosome-like vesicles released from human tracheobronchial ciliated epithelium: a possible role in innate defense. FASEB J. 23 (6), 1858–1868. doi:10.1096/fj.08-119131
Kowal, J., and Tkach, M. (2019). Dendritic cell extracellular vesicles. Int. Rev. Cell Mol. Biol. 349, 213–249. doi:10.1016/BS.IRCMB.2019.08.005
Kuwabara, Y., Ono, K., Horie, T., Nishi, H., Nagao, K., Kinoshita, M., et al. (2011). Increased microRNA-1 and microRNA-133a levels in serum of patients with cardiovascular disease indicate myocardial damage. Circ. Cardiovasc Genet. 4 (4), 446–454. doi:10.1161/CIRCGENETICS.110.958975
Lässer, C., Alikhani, V. S., Ekström, K., Eldh, M., Paredes, P. T., Bossios, A., et al. (2011). Human saliva, plasma and breast milk exosomes contain RNA: uptake by macrophages. J. Transl. Med. 9, 9. doi:10.1186/1479-5876-9-9
Lee, T. H., D’Asti, E., Magnus, N., Al-Nedawi, K., Meehan, B., and Rak, J. (2011). Microvesicles as mediators of intercellular communication in cancer--the emerging science of cellular debris. Seminars Immunopathol. 33 (5), 455–467. doi:10.1007/s00281-011-0250-3
Lener, T., Gimona, M., Aigner, L., Börger, V., Buzas, E., Camussi, G., et al. (2015). Applying extracellular vesicles based therapeutics in clinical trials - an ISEV position paper. J. Extracell. Vesicles 4 (1), 30087. doi:10.3402/JEV.V4.30087
Lo Cicero, A., Stahl, P. D., and Raposo, G. (2015a). Extracellular vesicles shuffling intercellular messages: for good or for bad. Curr. Opin. Cell Biol. 35, 69–77. doi:10.1016/J.CEB.2015.04.013
Mathis, B. J., Kusumoto, M., Zaboronok, A., and Hiramatsu, Y. (2021). Packaging and delivery of asthma therapeutics. Pharmaceutics 14 (1), 92. doi:10.3390/PHARMACEUTICS14010092
Meng, Q., Wang, J., Cui, J., Li, B., Wu, S., Yun, J., et al. (2022). Prediction of COPD acute exacerbation in response to air pollution using exosomal circRNA profile and Machine learning. Environ. Int. 168, 107469. doi:10.1016/j.envint.2022.107469
Mirershadi, F., Ahmadi, M., Rezabakhsh, A., Rajabi, H., Rahbarghazi, R., and Keyhanmanesh, R. (2020). Unraveling the therapeutic effects of mesenchymal stem cells in asthma. Stem Cell Res. Ther. 11 (1), 400. doi:10.1186/S13287-020-01921-2
Moher, D., Shamseer, L., Clarke, M., Ghersi, D., Liberati, A., Petticrew, M., et al. (2015). Preferred reporting items for systematic review and meta-analysis protocols (PRISMA-P) 2015 statement. Syst. Rev. 4, 1. doi:10.1186/2046-4053-4-1
Moola, S., Munn, Z., Sears, K., Sfetcu, R., Currie, M., Lisy, K., et al. (2015). Conducting systematic reviews of association (etiology): the Joanna Briggs Institute’s approach. Int. J. Evid. Based Healthc. 13 (3), 163–169. doi:10.1097/XEB.0000000000000064
Page, M. J., McKenzie, J. E., Bossuyt, P. M., Boutron, I., Hoffmann, T. C., Mulrow, C. D., et al. (2021). The PRISMA 2020 statement: an updated guideline for reporting systematic reviews. BMJ 372 (Mar), n71. doi:10.1136/BMJ.N71
Purghè, B., Manfredi, M., Ragnoli, B., Baldanzi, G., and Malerba, M. (2021). Exosomes in chronic respiratory diseases. Biomed. Pharmacother. 144 (Dec), 112270. doi:10.1016/J.BIOPHA.2021.112270
Rabinowits, G., Gerçel-Taylor, C., Day, J. M., Taylor, D. D., and Kloecker, G. H. (2009). Exosomal microRNA: a diagnostic marker for lung cancer. Clin. Lung Cancer 10 (1), 42–46. doi:10.3816/CLC.2009.n.006
Raimondo, S., Giavaresi, G., Lorico, A., and Alessandro, R. (2019). Extracellular vesicles as biological shuttles for targeted therapies. Int. J. Mol. Sci. 20 (8), 1848. doi:10.3390/IJMS20081848
Rajabi, H., Konyalilar, N., Erkan, S., Mortazavi, D., Korkunc, S. K., Kayalar, O., et al. (2022). Emerging role of exosomes in the pathology of chronic obstructive pulmonary diseases; destructive and therapeutic properties. Stem Cell Res. Ther. 13 (1), 144. doi:10.1186/S13287-022-02820-4
Rezabakhsh, A., Sokullu, E., and Rahbarghazi, R. (2021). Applications, challenges and prospects of mesenchymal stem cell exosomes in regenerative medicine. Stem Cell Res. Ther. 12 (1), 521. doi:10.1186/S13287-021-02596-Z
Song, J., Zhu, X. M., and Wei, Q. Y. (2020). MSCs reduce airway remodeling in the lungs of asthmatic rats through the Wnt/β-catenin signaling pathway. Eur. Rev. Med. Pharmacol. Sci. 24 (21), 11199–11211. doi:10.26355/eurrev_202011_23608
Srinivasan, S., Vannberg, F. O., and Dixon, J. B. (2016). Lymphatic transport of exosomes as a rapid route of information dissemination to the lymph node. Sci. Rep. 6, 24436. doi:10.1038/SREP24436
Tan, D. B. A., Armitage, J., Teo, T. H., Ong, N. E., Shin, H., and Moodley, Y. P. (2017). Elevated levels of circulating exosome in COPD patients are associated with systemic inflammation. Respir. Med. 132, 261–264. doi:10.1016/J.RMED.2017.04.014
Théry, C., Ostrowski, M., and Segura, E. (2009a). Membrane vesicles as conveyors of immune responses. Nat. Rev. Immunol. 9 (8), 581–593. doi:10.1038/nri2567
Théry, C., Zitvogel, L., and Amigorena, S. (2002). Exosomes: composition, biogenesis and function. Nat. Rev. Immunol. 2 (8), 569–579. doi:10.1038/nri855
Torregrosa Paredes, P., Esser, J., Admyre, C., Nord, M., Rahman, Q. K., Lukic, A., et al. (2012). Bronchoalveolar lavage fluid exosomes contribute to cytokine and leukotriene production in allergic asthma. Allergy Eur. J. Allergy Clin. Immunol. 67 (7), 911–919. doi:10.1111/j.1398-9995.2012.02835.x
Valadi, H., Ekström, K., Bossios, A., Sjöstrand, M., Lee, J. J., and Lötvall, J. O. (2007). Exosome-mediated transfer of mRNAs and microRNAs is a novel mechanism of genetic exchange between cells. Nat. Cell Biol. 9 (6), 654–659. doi:10.1038/ncb1596
Wang, C., Xu, J., Yang, L., Xu, Y., Zhang, X., Bai, C., et al. (2018). Prevalence and risk factors of chronic obstructive pulmonary disease in China (the China Pulmonary Health [CPH] study): a national cross-sectional study. Lancet 391, 1706–1717. doi:10.1016/S0140-6736(18)30841-9
Wang, W., Sinha, A., Lutter, R., Yang, J., Ascoli, C., Sterk, P. J., et al. (2022). Analysis of exosomal MicroRNA dynamics in response to rhinovirus challenge in a longitudinal case-control study of asthma. Viruses 14 (11), 2444. doi:10.3390/V14112444
Xu, R., Greening, D. W., Zhu, H. J., Takahashi, N., and Simpson, R. J. (2016). Extracellular vesicle isolation and characterization: toward clinical application. J. Clin. Invest. 126 (4), 1152–1162. doi:10.1172/JCI81129
Yáñez-Mó, M., Siljander, P. R. M., Andreu, Z., Zavec, A. B., Borràs, F. E., Buzas, E. I., et al. (2015). Biological properties of extracellular vesicles and their physiological functions. J. Extracell. Vesicles 4, 27066–27160. doi:10.3402/JEV.V4.27066
Keywords: asthma, COPD, exacerbation, exosomes, systematic review
Citation: Laitano R, Calzetta L, Motta E, Puxeddu E and Rogliani P (2024) Role of exosomes in exacerbations of asthma and COPD: a systematic review. Front. Mol. Biosci. 11:1356328. doi: 10.3389/fmolb.2024.1356328
Received: 15 December 2023; Accepted: 27 May 2024;
Published: 18 June 2024.
Edited by:
Jianbin Xu, Zhejiang University, ChinaReviewed by:
Dwijendra K. Gupta, Allahabad University, IndiaSurjendu Maity, Duke University, United States
Copyright © 2024 Laitano, Calzetta, Motta, Puxeddu and Rogliani. This is an open-access article distributed under the terms of the Creative Commons Attribution License (CC BY). The use, distribution or reproduction in other forums is permitted, provided the original author(s) and the copyright owner(s) are credited and that the original publication in this journal is cited, in accordance with accepted academic practice. No use, distribution or reproduction is permitted which does not comply with these terms.
*Correspondence: Luigino Calzetta, luigino.calzetta@unipr.it
†These authors have contributed equally to this work