- 1Research Institute of Medical and Health Sciences, University of Sharjah, Sharjah, United Arab Emirates
- 2College of Medicine, University of Sharjah, Sharjah, United Arab Emirates
- 3College of Medicine, Mohammed Bin Rashid University of Medicine and Health Sciences, Dubai, United Arab Emirates
- 4Faculty of Medicine, Alexandria University, Alexandria, Egypt
- 5College of Dental Medicine, University of Sharjah, Sharjah, United Arab Emirates
- 6Division of Surgery and Interventional Science, University College London, London, United Kingdom
Prostate cancer (PCa) is considered one of the most common cancers worldwide. Despite advances in patient diagnosis, management, and risk stratification, 10%–20% of patients progress to castration-resistant disease. Our previous report highlighted a protective role of Dickkopf-3 (DKK3) in PCa stroma. This role was proposed to be mediated through opposing extracellular matrix protein 1 (ECM-1) and TGF-β signalling activity. However, a detailed analysis of the prognostic value of DKK3, ECM-1 and members of the TGF-β signalling pathway in PCa was not thoroughly investigated. In this study, we explored the prognostic value of DKK3, ECM-1 and TGFB1 using a bioinformatical approach through analysis of large publicly available datasets from The Cancer Genome Atlas Program (TGCA) and Pan-Cancer Atlas databases. Our results showed a significant gradual loss of DKK3 expression with PCa progression (p < 0.0001) associated with increased DNA methylation in its promoter region (p < 1.63E-12). In contrast, patients with metastatic lesions showed significantly higher levels of TGFB1 expression compared to primary tumours (p < 0.00001). Our results also showed a marginal association between more advanced tumour stage presented as positive lymph node involvement and low DKK3 mRNA expression (p = 0.082). However, while ECM1 showed no association with tumour stage (p = 0.773), high TGFB1 expression showed a significant association with more advanced stage presented as advanced T3 stage compared to patients with low TGFB1 mRNA expression (p < 0.001). Interestingly, while ECM1 showed no significant association with patient outcome, patients with high DKK3 mRNA expression showed a significant association with favourable outcomes presented as prolonged disease-specific (p = 0.0266), progression-free survival (p = 0.047) and disease-free (p = 0.05). In contrast, high TGFB1 mRNA expression showed a significant association with poor patient outcomes presented as shortened progression-free (p = 0.00032) and disease-free survival (p = 0.0433). Moreover, DKK3, TGFB1 and ECM1 have acted as immune-associated genes in the PCa tumour microenvironment. In conclusion, our findings showed a distinct prognostic value for this three-gene signature in PCa. While both DKK3 and TGFB1 showed a potential role as a clinical marker for PCa stratification, ECM1 showed no significant association with the majority of clinicopathological parameters, which reduce its clinical significance as a reliable prognostic marker.
Introduction
Prostate cancer (PCa) is still one of the most common male cancers (Greenberg et al., 2013) and one of the top five leading causes of death (Rawla, 2019; Barsouk et al., 2020). Moreover, many reports have highlighted an increase in the number of PCa cases diagnosed annually with the prediction that those numbers might increase in the near future (Mistry et al., 2011). An important element in determining the optimal management plan for patients with PCa is risk stratification (Greenberg et al., 2013; Yamazaki et al., 2021). Gleason score, PSA levels and clinical stage are considered important elements in risk stratification and prediction of tumour progression as well as recurrence (Yamazaki et al., 2021). The Gleason score is one of the main components of PCa prognosis and a risk stratification tool and is mainly based on the assessment of architectural features and glandular de-differentiation (Chen and Zhou, 2016; Tagai et al., 2019; van Leenders et al., 2020).
PCa patients may develop resistance to initial hormone therapy (10%–20%) and progress to a more advanced stage known as a castration-resistant disease (Vellky and Ricke, 2020; Harris et al., 2009; Yamazaki et al., 2021). This presents a clinical challenge, and there is a need to discover new prognostic biomarkers that can predict tumour progression and recurrence accurately (Frantzi et al., 2020).
Many reports highlighted the important role of cancer cell interactions with the reactive stroma in determining tumour behaviour, spread and progression. Some factors in the tumour microenvironment have tumour-inhibitory effects that might improve patient prognosis (Baghban et al., 2020), despite the stromal compartment in many cancers being pro-tumourigenic (Lopes-Coelho et al., 2018; Valkenburg et al., 2018). Recently, we revealed a protective role of stromal Dickkopf-3 (DKK3) in PCa (Al Shareef et al., 2018). Moreover, we also observed an inverse correlation between DKK3 and transforming growth Factor Beta Induced 1 (TGFB1) expression and that the activity of DKK3 was affected in different ways by TGFB1 and ECM-1 (Al Shareef et al., 2018; Niehrs, 2006; Kikuchi et al., 2021).
DKK3 is a member of the DKK family, secreted glycoproteins that negatively regulate Wnt signalling, although in the case of DKK3, this must be through a different mechanism (Veeck and Dahl, 2012). This might be attributed to the distinct amino acid sequence of DKK3, compared to other DKK family members, in the region of the LRP6-binding site (Niehrs, 2006; Kikuchi et al., 2021). Reports suggest that the tumour suppressive activity of DKK3 is related to its negative regulation of the β-catenin activity (Lee et al., 2020).
Previous reports highlighted a reduction in DKK3 expression in aggressive human cancer cells including basal breast cancer, melanoma, and hepatocellular carcinoma (HCC) through the promotor hypermethylation (Veeck and Dahl, 2012). Moreover, ectopic overexpression of DKK3 in some cancer cell lines inhibits their proliferation or induces apoptosis. Together, these studies suggest DKK3 has a tumour-suppressive function in various cancers (Hsieh et al., 2004; Kuphal et al., 2006; Lorsy et al., 2016). In contrast, some other reports highlight a tumour-promoting role of DKK3, for example, in oesophageal adenocarcinoma and oral squamous cell carcinoma (SCC), where it increases tumour cell proliferation and migration (Katase et al., 2013; Wang et al., 2015).
In the current study, we performed a comprehensive analysis of the prognostic value of DKK3, TGFB1 and ECM-1 as a multiple-gene prognostic signature in PCa using bioinformatics approaches.
Materials and methods
Gene expression evaluation in PCa nad normal samples
The publicly available application TNM plot (https://tnmplot.com/analysis/) was used to evaluate the mRNA expression levels of the genes of interest in normal (n = 106), tumour (n = 283) and metastatic (n = 6) samples (Bartha and Gyorffy, 2021). For statistical significance, the Kruskal–Wallis test was used to compare the gene expression levels among normal, tumour and metastatic tissue with p < 0.01 used as a cut-off value for statistical significance. In addition, using cBioPortal (cbioportal.org), we retrieved and analysed mRNA data (RNA SeqV2) of 493 patients with prostate adenocarcinoma from the Cancer Genome Atlas (TGCA) and Pan-Cancer Atlas. Log-rank test p-values were used to evaluate the statistical significance between the low and the high expression level (classified according to above and below mean mRNA expression of each biomarker. The Chi-squared test was used to evaluate the association between each biomarker and clinicopathological parameters including tumour stage as well as patient outcome. Moreover, the DNA methylation profiles retrieved from the TCGA database were assessed in order to evaluate the DKK3 promoter DNA methylation levels using the UALCAN portal. DNA methylation analysis was carried out using normalized beta values from 502 PCa patients and 50 healthy controls considering beta value cut-offs of hyper-methylation [beta value: 0.7–0.5] or hypo-methylation [beta-value: 0.3–0.25].
Evaluation of the prognostic capacity
To evaluate the prognostic capacity of each marker of interest, we used the CANCERTOOL database, (http://web.bioinformatics.cicbiogune.es/CANCERTOOL), which is a comprehensive portal that aims to investigate different genes and their association with clinical data including disease progression, pathologic, and molecular characteristics, to evaluate the expression levels of our markers in normal versus malignant tissues.
Correlation analysis of gene expression and immune infiltration in PCa tumour microenvironment
The relationship between DKK3, TGFB1 and ECM1 and immune cell infiltration in the in PCa microenvironment was explored using the Tumour IMmune Estimation Resource (TIMER) (https://cistrome.shinyapps.io/timer/), which consists of deconvolution and comprehensive analysis of tumour-infiltrating immune cells in various types of cancer. The association between DKK3, TGFB1 and ECM1 expression and the abundances of immune infiltrates including B cells, CD4+ T cells, CD8+ T cells, neutrophils, macrophages, and dendritic cells in the PCa tumour microenvironment was assessed, using Spearman’s test (p < 0.05).
Construction of gene-gene interaction network and functional enrichment analysis
To assess the functional association between the studied genes DKK3, TGFB1 and ECM1 and their related connected genes, the gene-gene interaction network was constructed based on a large data of functional-related data sets including genetic and protein interactions, physical interaction, co-expression, co-localization, and common pathway, using GeneMANIA bioinformatics tool with defaults parameters (Warde-Farley et al., 2010). Genes can be linked by the interacted network based on variable attributes. The network includes nodes that represent genes while edges represent connections. Besides, functional enrichment analysis on Gene Ontology terms including biological process (BP), cellular component (CC), and molecular function (MF) categories was conducted using the Enrichr database (Xie et al., 2021) to explore the biological significance and common pathways between the DKK3, TGFB1 and ECM1 genes. The hypergeometric distribution cut-off for the functional enrichment analyses was a p-value of <0.05.
Results
DKK3 is downregulated in PCa samples compared to normal tissue
To improve our understanding of the role of DKK3, TGFB1 and ECM-1 genes in tumourigenesis, we initially investigated the expression levels of DKK3 in PCa samples compared to their normal counterparts using the CANCERTOOL database. Different datasets were used including Glinksy et al., Grasso et al., Lapointe et al., Taylor et al., TCGA (RNA-seq), Tomlins et al., and Varambally et al. (Figure 1). Our results showed a significant lower level of DKK3 in PCa samples compared to samples obtained from their normal counterparts. Similarly, TGFB1 showed also significant downregulation in PCa samples compared to normal counterparts. In contrast, ECM-1 levels showed no significant differences between the normal and PCa samples (Supplementary Figure S1).
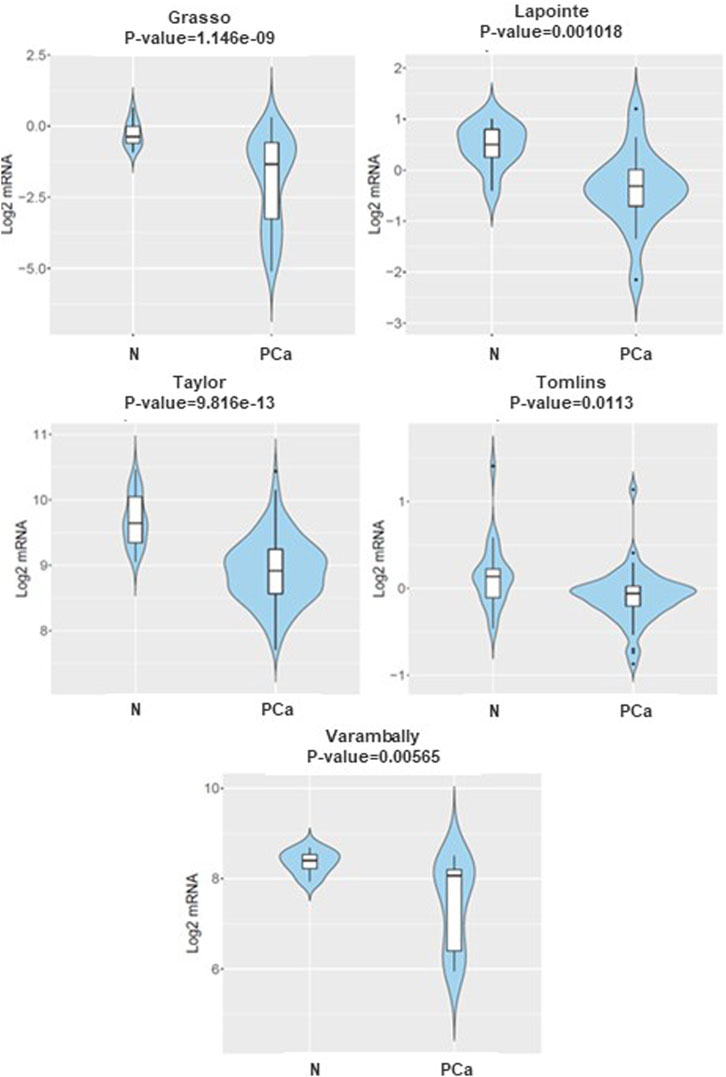
Figure 1. The mRNA expression of DKK3 in normal versus prostate cancer samples CANCERTOOL database. Violin plots show the expression of DKK3 in non-tumoral tissue (N) and primary tumours (PT) in various datasets of the CANCERTOOL database.
Moreover, the evaluation of DNA methylation levels in the promoter region of DKK3 using TCGA publicly available methylome data showed significant DNA methylation differences between primary PCa and normal samples (p < 1.62 E-12). Indeed, the DKK3 promoter was highly hypermethylated among PCa cases (n = 502), while it was hypomethylated in the normal samples (n = 50). Our findings indicate that there is a notable decrease in the expression of DKK3 in PCa samples from various datasets, indicating that the promoter region of the DKK3 gene may be affected by DNA methylation, leading to its silencing (Figure 2).
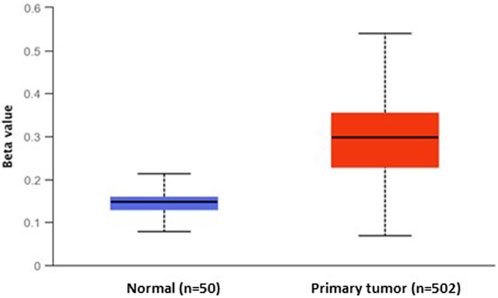
Figure 2. The differential DNA methylation levels of DKK3 promoter between normal and primary prostate tumours.
The value of DKK3 expression as a marker of tumour progression in PCa samples
To improve our understanding of the role of DKK3, ECM-1 and TGF-β in PCa tumourigenesis, we evaluated their expression in samples representing different stages of PCa progression (Figure 3). To achieve this, we analysed DKK3, ECM-1 and TGFB1 expression in normal prostate, primary tumours and samples obtained from more advanced diseases presented as patients with tumour metastases. Interestingly, our results showed a gradual and significant downregulation of DKK3 expression during tumour progression. Indeed, the expression levels of DKK3 showed significant downregulation in tumour samples compared to normal. Further downregulation was observed between tumour samples and samples obtained from patients with metastatic tumours (p < 0.0001). In contrast, while TGFB1 expression was comparable between normal and tumour samples, metastatic lesions showed a significantly higher level of TGFB1, compared to both primary tumour and normal samples (p = 0.04). Moreover, while ECM1 expression levels were higher in tumour samples compared to normal tissue, ECM1 expression was significantly lower in metastatic samples compared to tumour, as well as normal samples (p < 0.0001). As a reference, we also investigated the expression levels of the classical marker PSA (KLK3) in the same samples. Our findings showed that while PSA levels initially increased in the tumour samples compared to normal, those levels were significantly lower in metastatic samples, compared to tumours (p < 0.0001). In summary, our results highlight the possible benefits of DKK3 expression as a marker of PCa progression, owing to its gradual loss during tumourigenesis.
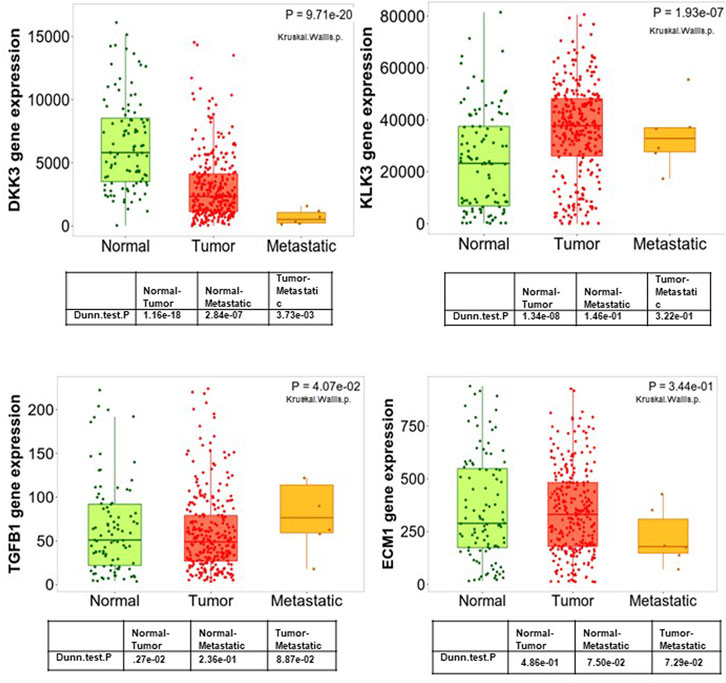
Figure 3. Boxplots of the mRNA expression of DKK3, ECM1, TGFB1 and PSA (KLK3) in a large patient cohort using the TNM plot database.
The association between DKK3, ECM-1 and TGFB1 mRNA expression and tumour staging and grade in a large patient cohort
Next, we evaluated the mRNA expression of the three genes in association with the tumour stage using the prostate adenocarcinoma (TCGA, PanCancer Atlas) cohort (Figure 4). Our results showed a marginal significance (p = 0.082) between DKK3 mRNA expression and LN involvement (Figure 4A). Patients with higher DKK3 mRNA expression showed less chance of positive lymph node involvement (N1) compared to patients with lower DKK3 mRNA expression. In comparison, while ECM1 showed no association with tumour stage (p = 0.773), TGFB1 showed a significant association with tumour stage (Figure 4B). Patients with high TGFB1 mRNA expression presented with the more advanced T3A (tumour spread outside the prostate, but not invading seminal vesicles) and T3B (tumour spread outside prostate with seminal vesicles invasion) stages, compared to patients with low TGFB1 mRNA expression (p < 0.001).
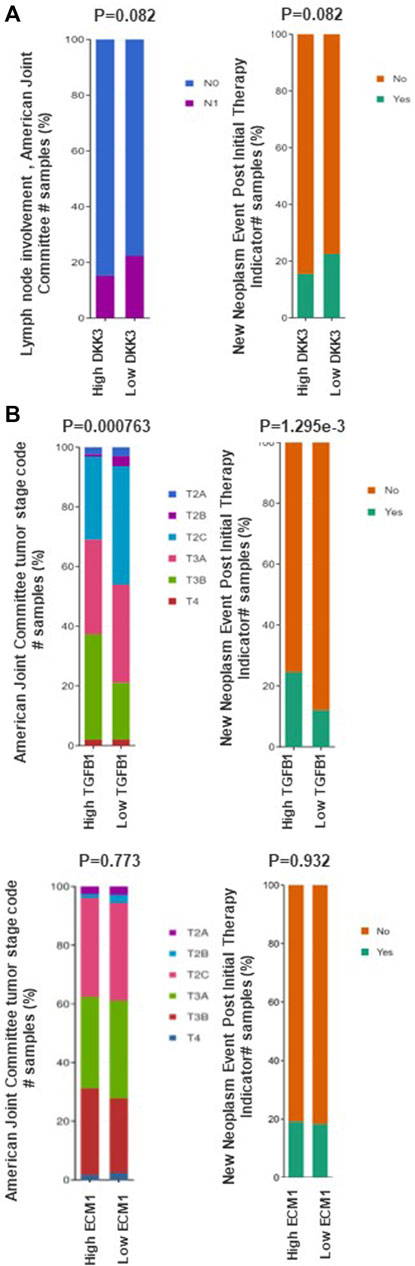
Figure 4. The association between DKK3, ECM1 and TGFB1 mRNA expression and clinicopathological features of prostate cancer. (A) The association between DKK3 and prostate cancer clinicopathological characteristics using data from prostate adenocarcinoma (TCGA) through cBioPortal tool. (B) The association between ECM1 and TGFB1 mRNA expression prostate cancer clinicopathological characteristics using data from prostate adenocarcinoma (TCGA) through cBioPortal tool.
Interestingly, while patients with high DKK3 mRNA expression showed marginally significant lower chance of having recurrence presented as new neoplastic events post initial therapy (p = 0.082), Patients with higher TGFB1 mRNA expression showed a significant higher chance of recurrence presented as new neoplastic events post initial therapy compared with patients with low TGFB1 mRNA expression (p < 0.001). In contrast, ECM1 mRNA expression showed no significant association with new neoplastic events post initial therapy (p = 0.932) (Figure 4).
The analysis between the mRNA expression of the three markers with tumor grade showed that while both DKK3 and ECM1 showed no significant variation in samples from different Gleason grade, TGFB1 mRNA expression was significantly higher in patients presented with higher gleason score (G8,9&10) in the TCGA dataset (p = 0.0001) (Supplementary Figure S2).
The correlation between DKK3 mRNA expression and both TGFB1 and ECM1 in prostate cancer samples
Further analysis was performed to investigate the correlation between DKK3 expression and TGFB1 and ECM1 in prostate cancer samples. Indeed, this might better demonstrate the interplay between DKK3 and these markers. While our analysis showed no significant correlation between ECM1 and DKK3 mRNA levels (R = 0.07, p = 0.23), our results showed a significant and negative correlation between DKK3 mRNA expression levels and TGFB1 (R = -0.17, p < 0.0001). Additional analysis was performed to investigate the association between DKK3 and KLK3, which is most relevant marker for PCa diagnosis and management, and its expression is regulated by the upstream markers, such as AR. Our analysis showed no significant correlation between DKK3 mRNA expression levels and KLK3 expression (R = -0.09, p = 0.14) (Supplementary Figure S3).
The association between DKK3, ECM1 and TGFB1 and patient outcome
Next, we evaluated the association between our markers and patient outcome presented as progression-free survival (Figure 5). While ECM-1 showed no significant association with patient outcome progression-free survival (p = 0.986), disease specific (p = 0.776) and disease free survival (p = 0.167). Both DKK3 and TGFB1 showed a significant and contrasting association with patient outcomes. While patients with high DKK3 mRNA expression showed a significant association with favourable outcomes presented as prolonged progression-free survival (p = 0.0470), disease specific (p = 0.0266) and disease free survival (p = 0.050), high TGFB1 mRNA expression showed a significant association with poor patient outcome presented as shortened progression-free survival (p = 3.267e-4) and disease free survival (p = 0.0433).
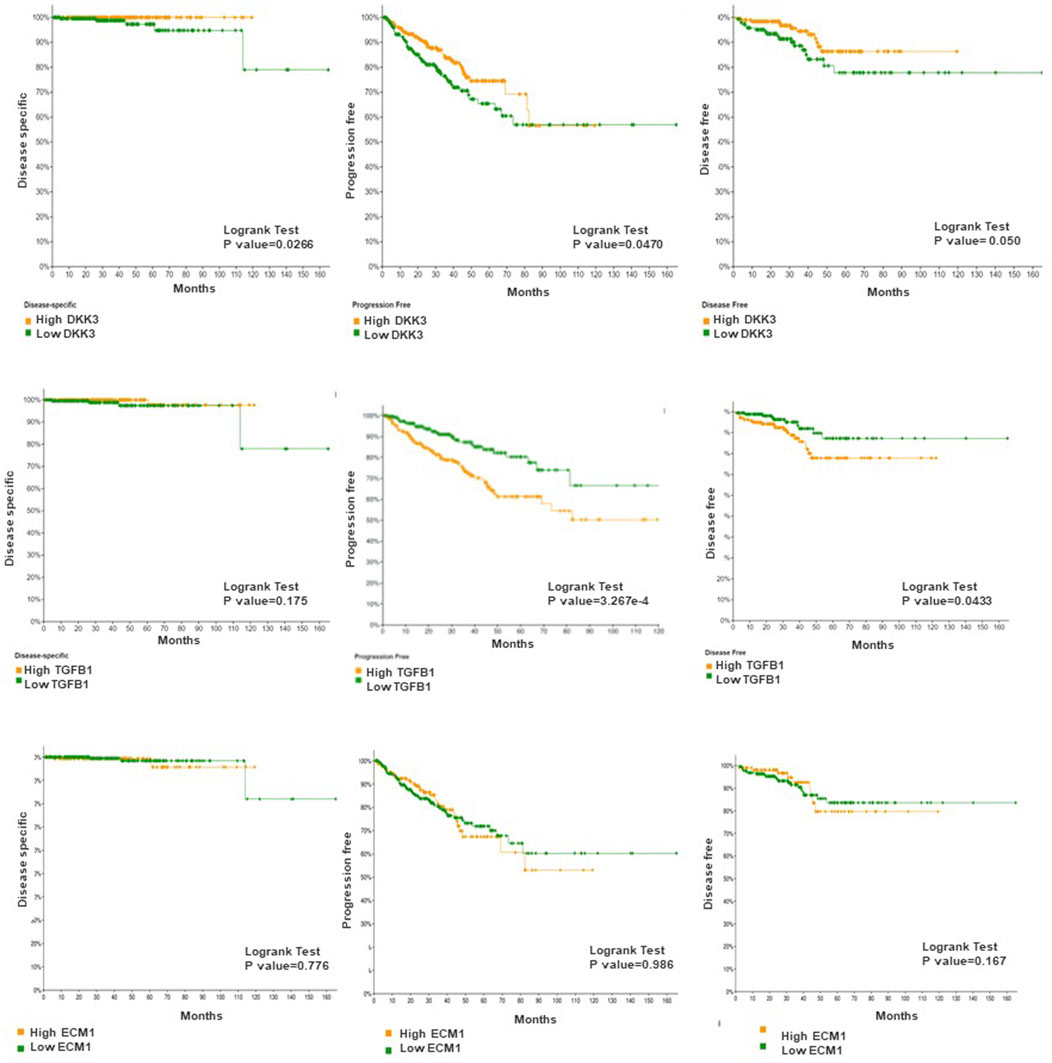
Figure 5. The association between DKK3, ECM1 and TGFB1 mRNA expression and patient survival. Log-rank test p-values were used to evaluate the statistical significance between the low and the high expression level.
DKK3, TGFB1 and ECM1 act as the immune-associated genes in PCa
We were also interested to analyze the relationship between DKK3, TGFB1 and ECM1 and immune cell infiltration in prostate cancer (Figure 6). The results showed that gene expression level against tumor purity is highly significant for the three biomarkers with negative Spearman’s rho values (−0.5, −0.329 and −0.41), respectively in DKK3, TGFB1 and ECM1 suggesting that these biomarkers are highly expressed in the microenvironment of PCa (Figure 6). Moreover, all biomarkers have a positive significant correlation with B cells, CD4+ T cells, CD8+ T cells, Neutrophils, Macrophages, and Dendritic cells. Importantly, DKK3 has the highest correlation with macrophage at 60%, while ECM1 showed the highest correlation at about 54% with dendritic cells and TGFB1 has the highest correlation with neutrophil and dendritic cells at 60% and 68% respectively. Importantly, all biomarkers had a better correlation with CD4 than CD8.
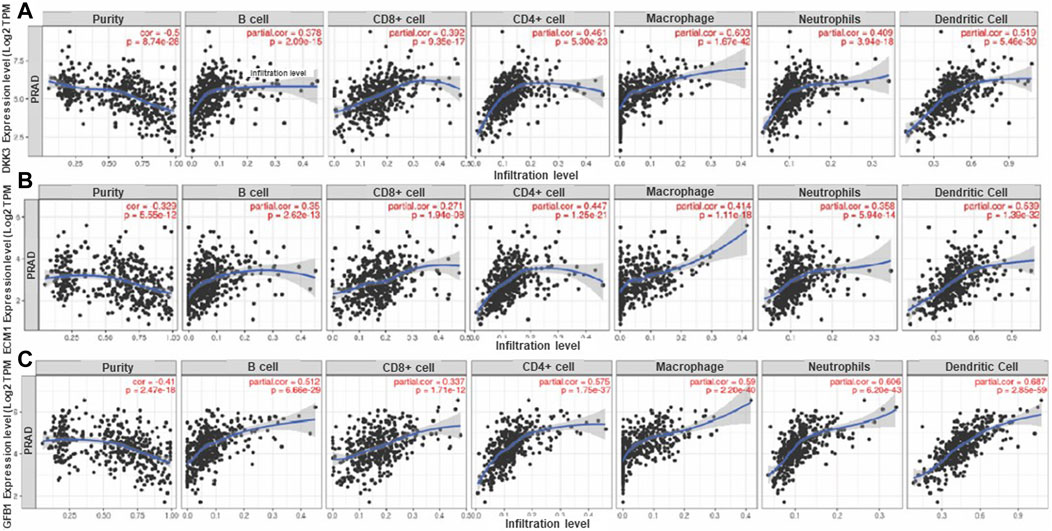
Figure 6. Correlation of (A) DKK3, (B) ECM1 and (C) TGFB1 gene expression level with immune cell infiltration in PCa. The scatterplots displayed Spearman's rho value correlation and statistical significance showing the potential interplay between DKK3, TGFBI and ECMI biomarkers and immune cells (B-Cell, CD8+ T Cell, CD4+ T Cell, Macrophage, Neutrophil and Dendritic Cell) in the prostate cancer tumor microenvironment. The log2 TPM gene expression values are presented on the y-axis, the average immune cell infiltration levels are presented on the x-axis. The blue curve and gray area in the figures show the general trend direction. TPM: transcripts per million. (Data generated from TIMER Webtool).
Biological functions and interconnections of the DKK3, TGFB1 and ECM1 genes
To explore the potential mechanisms that involve DKK3, TGFB1 and ECM1 in the carcinogenesis of PCa, we explored GeneMania to build up a gene–gene interaction network for these biomarkers. As shown in Figure 7, the network revealed that DKK3, TGFB1 and ECM1 share many genes within the same gene family or similar protein domain under a series of cooperators suggesting that there is a possibility of the interplay between the 3 markers through interaction with proteins from a similar family. For instance, MMP9 presents a key marker in the network that showed physical interconnection with TGFB1 and ECM1, and co-expression with DKK3 suggesting the potential direct/indirect connections between these particular biomarkers. Knowing that MMP9 codes for a matrix metalloproteinase protein that is reported to promote metastasis and angiogenesis through decomposition of the extracellular matrix in several tumours which further supports the possible involvement of DKK3, TGFB1 and ECM1 in the PCa progression.
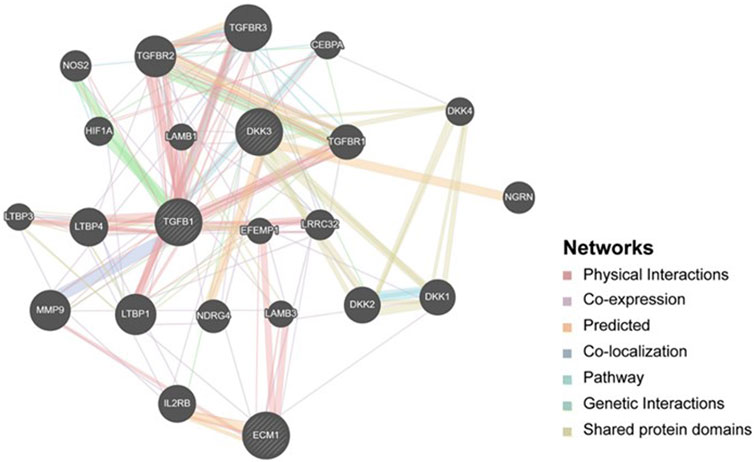
Figure 7. Gene-Gene interaction network including DKK3, TGFB1 and ECM1. It shows interaction strength (edge thickness), interaction type (colour), multiple edges between nodes, and protein score (node size) defined using a stylesheet constructed with GeneMANIA. The interconnections between studied genes were evaluated based on physical interaction, co-expression, predicted, co-localization, common pathway, genetic interaction and shared protein domains.
Furthermore, gene ontologies including biological process and molecular function were performed to reveal the functional enrichment of DKK3, TGFB1 and ECM1 and their co-operators. The significant annotated pathways were represented in Figure 7. Interestingly, it identifies cytokine activity (GO:0005125, p-value = 0.02) and macrophage-related pathways such as negative regulation of macrophage cytokine production (GO:0010936, p-value = 7.5E-04) among the differentially regulated pathways involving mainly TGFB1 as a key player which further supports the findings of immune cell infiltration in PCa (Figure 8). Additionally, the regulation of canonical Wnt signaling pathway (GO:0060828, p-value = 4.74E-04) was identified among the relevant significantly regulated biological processes involving TGFB1 and DKK3, which could be of a pivotal role in PCa pathogenesis, proliferation and resistance to treatment. Taken together, these findings highlight the potential utility of DKK3, TGFB1 and ECM1as prognostic markers for PCa.
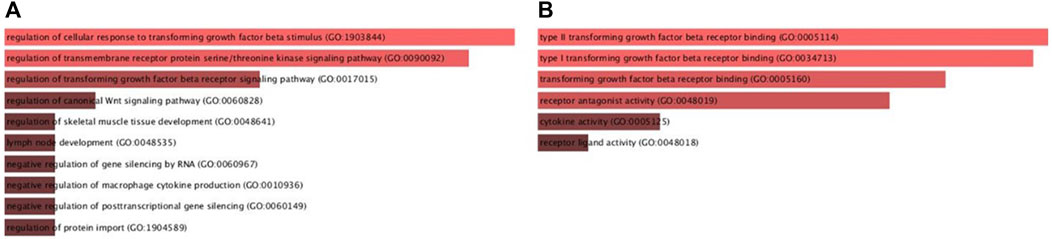
Figure 8. Gene ontologies enrichment analysis (A) Biological Process and (B) Molecular Functions of DKK3, TGFBI and ECMI using Enricher database.
Discussion
Previous reports highlighted the role of the Dickkopf (Dkk) gene family in determining cell fate during embryonic development (Hoang et al., 2004; Krupnik et al., 1999; Hoang et al., 2004; Niehrs, 2006). Moreover, the DKK3 gene was found to encode essential intracellular regulators of cellular proliferation (Leonard et al., 2017). A tumour suppressor role of DKK3 was suggested since ectopic expression of Dkk3 was found to reduce cancer growth, in contrast, loss of Dkk3 expression induces hyperproliferation of cells (Veeck and Dahl, 2012; Fujii et al., 2014). In addition, other reports also found lower levels of DKK3 in various tumours including gastric, ovarian, lung, bladder and PCa (Kurose et al., 2004; Chim et al., 2008; Yue et al., 2008; Ueno et al., 2011).
Our earlier report proposed a tumour suppressor role of DKK3 in PCa through opposing TGFBI and ECM-1 function (Al Shareef et al., 2018). However, a comprehensive analysis of the prognostic role of DKK3, as well as its relationship with the expression of TGFB1 and ECM1 in a large sample size of PCa was not investigated. Here we have analysed the prognostic value of DKK3, TGFB1 and ECM1 and their association with well-known prognostic clinicopathological parameters in PCa using multiple cohorts in various publicly available databases. Additionally, we conducted an investigation into the pathways involved in the interaction between DKK3, TGFB1, and ECM1.
This study reveals that ECM1 and TGFB1 are upregulated in cancerous tissues in comparison to normal tissues. Conversely, DKK3 expression was found to be downregulated in malignant tissue in comparison to normal tissue. These results align with prior research that has demonstrated decreased expression of DKK3 in various types of cancer as compared to healthy tissue (Kurose et al., 2004; Chim et al., 2008; Yue et al., 2008; Ueno et al., 2011). This also supports our previous notion that DKK3 might exert a protective role against PCa (Al Shareef et al., 2018) and highlighted a possible role of its loss in PCa tumourigenesis.
Our results also showed a gradual loss of DKK3 mRNA expression between and during cancer progression with its least expression in samples obtained from patients with metastasis. Interestingly, our results showed that DKK3 expression was more able to predict tumour progression than KLK3 (coding for PSA). Indeed, PSA is considered one of the most relevant markers for PCa diagnosis and management (Bonk et al., 2019). Moreover, recent reports highlighted KLK3 as a possible predictive marker for molecular lymph-node staging in PCa patients (Lunger et al., 2021). However, our analysis showed that while KLK3 expression was significantly upregulated in primary cancer samples compared to normal, its expression was not significantly variable between metastatic and primary tumour samples. Indeed, this statement suggests that the clinical usefulness of PSA (KLK3) in assessing tumor progression is greatly restricted, and instead emphasizes the potential benefits of utilizing DKK3 expression as an indicator of cancer progression. In addition, our results also showed a significant association between low DKK3 expression and poor patient outcomes represented as shortened disease-specific, disease free and progression-free survival. While the association between DKK3 expression and patient outcome in PCa was not thoroughly investigated, recent reports showed a significant association between low DKK3 expression and reduced recurrence-free survival in breast cancer, cervical cancer, colorectal cancer, endometrial cancer and gastric cancer (Wang et al., 2012; Xu et al., 2012; Ryu et al., 2013; Lorsy et al., 2016).
Our results also highlighted a possible interplay between DKK3, TGFB1 and ECM1 in PCa through modulation of some biological processes and molecular functions including members of TGF-β signaling and the Wnt signaling pathway. Wnt Signaling was found to play an essential role in the modulation of PCa microenvironment to promote drug resistance as well as cancer stem cell expansion and self-renewal (Kypta and Waxman, 2012). Our analysis also identified pathways involved in the regulation of macrophage cytokine production as one of the enriched pathways in the regulation of DKK3, TGFB1 and ECM1 function in PCa. This highlighted a possible mechanism through which those genes modulate PCa microenviroment through regulation of macrophage function, which is now believed to play a role in PCa progression and metastatic cascades of PCa (Lo and Lynch, 2018). Further studies are still needed for comprehensive analysis for the role of DKK3, TGFB1 and ECM1 in PCa including the use of human single cell/spatial analysis of human Pca. Indeed this might improve our understanding to the prognostic role and their role in pathogenesis of prostate including progression and metastasis.
Conclusion
To summarize, our study utilized in silico analyses to investigate the potential utility of DKK3, TGFB1 and ECM1 as prognostic markers for PCa. Our findings suggest that while DKK3, TGFB1 could serve as promising biomarkers for predicting disease progression and patient outcomes, ECM1 expression showed no significant association with various clinicopathological parameters. For that reason, only DKK3 and TGFB1 can be used as a reliable prognostic markers in prostate cancer and the he incorporation of these biomarkers into clinical practice could enhance PCa patient stratification and facilitate the adoption of tailored therapeutic strategies for each individual. Furthermore, our analysis identified a group of key pathways that appear to be central in the interplay between DKK3, TGFB1 and ECM1, resulting in the modulation of the tumour microenvironment.
Data availability statement
The original contributions presented in the study are included in the article/Supplementary Material, further inquiries can be directed to the corresponding author.
Author contributions
ZA: Conceptualization, Data curation, Funding acquisition, Investigation, Methodology, Supervision, Writing–original draft, Writing–review and editing. MH: Data curation, Validation, Writing–original draft, Writing–review and editing, Conceptualization. AB: Data curation, Formal Analysis, Writing–original draft, Writing–review and editing. IT: Validation, Writing–review and editing. NA-R: Writing–review and editing. RH: Data curation, Validation, Writing–review and editing. IH: Investigation, Methodology, Validation, Writing–original draft, Writing–review and editing, Conceptualization.
Funding
The author(s) declare that financial support was received for the research, authorship, and/or publication of this article. ZA is funded by University of Sharjah, seed grant and targeted grant project number (1901090161). RH is funded by ASPIRE, the technology program management pillar of Abu Dhabi’s Advanced Technology Research Council (ATRC), via the ASPIRE Precision Medicine Research Institute Abu Dhabi (VRI-20-10) grant.
Acknowledgments
We would like to thank Dr. Abdulqadir Alzarooni, Chief of ER and Surgical Services, Consultant & Chair of Urology for their support and contribution to this manuscript.
Conflict of interest
The authors declare that the research was conducted in the absence of any commercial or financial relationships that could be construed as a potential conflict of interest.
Publisher’s note
All claims expressed in this article are solely those of the authors and do not necessarily represent those of their affiliated organizations, or those of the publisher, the editors and the reviewers. Any product that may be evaluated in this article, or claim that may be made by its manufacturer, is not guaranteed or endorsed by the publisher.
Supplementary material
The Supplementary Material for this article can be found online at: https://www.frontiersin.org/articles/10.3389/fmolb.2024.1351888/full#supplementary-material
References
Al Shareef, Z., Kardooni, H., Murillo-Garzon, V., Domenici, G., Stylianakis, E., Steel, J. H., et al. (2018). Protective effect of stromal Dickkopf-3 in prostate cancer: opposing roles for TGFBI and ECM-1. Oncogene 37 (39), 5305–5324. doi:10.1038/s41388-018-0294-0
Baghban, R., Roshangar, L., Jahanban-Esfahlan, R., Seidi, K., Ebrahimi-Kalan, A., Jaymand, M., et al. (2020). Tumor microenvironment complexity and therapeutic implications at a glance. Cell. Commun. Signal. CCS 18 (1), 59. doi:10.1186/s12964-020-0530-4
Barsouk, A., Padala, S. A., Vakiti, A., Mohammed, A., Saginala, K., Thandra, K. C., et al. (2020). Epidemiology, staging and management of prostate cancer. Med. Sci. (Basel). 8 (3), 28. doi:10.3390/medsci8030028
Bartha, A., and Gyorffy, B. (2021). TNMplot.com: a web tool for the comparison of gene expression in normal, tumor and metastatic tissues. Int. J. Mol. Sci. 22 (5), 2622. doi:10.3390/ijms22052622
Bonk, S., Kluth, M., Hube-Magg, C., Polonski, A., Soekeland, G., Makropidi-Fraune, G., et al. (2019). Prognostic and diagnostic role of PSA immunohistochemistry: a tissue microarray study on 21,000 normal and cancerous tissues. Oncotarget 10 (52), 5439–5453. doi:10.18632/oncotarget.27145
Chen, N., and Zhou, Q. (2016). The evolving Gleason grading system. Chin. J. Cancer Res. 28 (1), 58–64. doi:10.3978/j.issn.1000-9604.2016.02.04
Chim, C. S., Pang, R., and Liang, R. (2008). Epigenetic dysregulation of the Wnt signalling pathway in chronic lymphocytic leukaemia. J. Clin. Pathol. 61 (11), 1214–1219. doi:10.1136/jcp.2008.060152
Frantzi, M., Hupe, M. C., Merseburger, A. S., Schanstra, J. P., Mischak, H., and Latosinska, A. (2020). Omics derived biomarkers and novel drug targets for improved intervention in advanced prostate cancer. Cancer. Diagn. (Basel) 10 (9), 658. doi:10.3390/diagnostics10090658
Fujii, Y., Hoshino, T., and Kumon, H. (2014). Molecular simulation analysis of the structure complex of C2 domains of DKK family members and β-propeller domains of LRP5/6: explaining why DKK3 does not bind to LRP5/6. Acta Med. Okayama 68 (2), 63–78. doi:10.18926/AMO/52403
Greenberg, D. C., Wright, K. A., Lophathanon, A., Muir, K. R., and Gnanapragasam, V. J. (2013). Changing presentation of prostate cancer in a UK population--10 year trends in prostate cancer risk profiles in the East of England. Br. J. Cancer 109 (8), 2115–2120. doi:10.1038/bjc.2013.589
Harris, W. P., Mostaghel, E. A., Nelson, P. S., and Montgomery, B. (2009). Androgen deprivation therapy: progress in understanding mechanisms of resistance and optimizing androgen depletion. Nat. Clin. Pract. Urol. 6 (2), 76–85. doi:10.1038/ncpuro1296
Hoang, B. H., Kubo, T., Healey, J. H., Yang, R., Nathan, S. S., Kolb, E. A., et al. (2004). Dickkopf 3 inhibits invasion and motility of Saos-2 osteosarcoma cells by modulating the Wnt-beta-catenin pathway. Cancer Res. 64 (8), 2734–2739. doi:10.1158/0008-5472.can-03-1952
Hsieh, S. Y., Hsieh, P. S., Chiu, C. T., and Chen, W. Y. (2004). Dickkopf-3/REIC functions as a suppressor gene of tumor growth. Oncogene 23 (57), 9183–9189. doi:10.1038/sj.onc.1208138
Katase, N., Lefeuvre, M., Tsujigiwa, H., Fujii, M., Ito, S., Tamamura, R., et al. (2013). Knockdown of Dkk-3 decreases cancer cell migration and invasion independently of the Wnt pathways in oral squamous cell carcinomaderived cells. Oncol. Rep. 29 (4), 1349–1355. doi:10.3892/or.2013.2251
Kikuchi, A., Matsumoto, S., and Sada, R. (2021). Dickkopf signaling, beyond Wnt-mediated biology. Semin. Cell. Dev. Biol. 125, 55–65. doi:10.1016/j.semcdb.2021.11.003
Krupnik, V. E., Sharp, J. D., Jiang, C., Robison, K., Chickering, T. W., Amaravadi, L., et al. (1999). Functional and structural diversity of the human Dickkopf gene family. Gene 238 (2), 301–313. doi:10.1016/s0378-1119(99)00365-0
Kuphal, S., Lodermeyer, S., Bataille, F., Schuierer, M., Hoang, B. H., and Bosserhoff, A. K. (2006). Expression of Dickkopf genes is strongly reduced in malignant melanoma. Oncogene 25 (36), 5027–5036. doi:10.1038/sj.onc.1209508
Kurose, K., Sakaguchi, M., Nasu, Y., Ebara, S., Kaku, H., Kariyama, R., et al. (2004). Decreased expression of REIC/Dkk-3 in human renal clear cell carcinoma. J. Urol. 171 (3), 1314–1318. doi:10.1097/01.ju.0000101047.64379.d4
Kypta, R. M., and Waxman, J. (2012). Wnt/β-catenin signalling in prostate cancer. Nat. Rev. Urol. 9 (8), 418–428. doi:10.1038/nrurol.2012.116
Lee, E. J., Nguyen, Q. T. T., and Lee, M. (2020). Dickkopf-3 in human malignant tumours: a clinical viewpoint. Anticancer Res. 40 (11), 5969–5979. doi:10.21873/anticanres.14617
Leonard, J. L., Leonard, D. M., Wolfe, S. A., Liu, J., Rivera, J., Yang, M., et al. (2017). The Dkk3 gene encodes a vital intracellular regulator of cell proliferation. PLoS One 12 (7), e0181724. doi:10.1371/journal.pone.0181724
Lo, C. H., and Lynch, C. C. (2018). Multifaceted roles for macrophages in prostate cancer skeletal metastasis. Front. Endocrinol. (Lausanne) 9, 247. doi:10.3389/fendo.2018.00247
Lopes-Coelho, F., Gouveia-Fernandes, S., and Serpa, J. (2018). Metabolic cooperation between cancer and non-cancerous stromal cells is pivotal in cancer progression. Tumour Biol. J. Int. Soc. Oncodevelopmental Biol. Med. 40 (2), 1010428318756203. doi:10.1177/1010428318756203
Lorsy, E., Topuz, A. S., Geisler, C., Stahl, S., Garczyk, S., von Stillfried, S., et al. (2016). Loss of Dickkopf 3 promotes the tumorigenesis of basal breast cancer. PLoS One 11 (7), e0160077. doi:10.1371/journal.pone.0160077
Lunger, L., Retz, M., Bandur, M., Souchay, M., Vitzthum, E., Jager, M., et al. (2021). KLK3 and TMPRSS2 for molecular lymph-node staging in prostate cancer patients undergoing radical prostatectomy. Prostate Cancer Prostatic Dis. 24 (2), 362–369. doi:10.1038/s41391-020-00283-3
Mistry, M., Parkin, D. M., Ahmad, A. S., and Sasieni, P. (2011). Cancer incidence in the United Kingdom: projections to the year 2030. Br. J. Cancer 105 (11), 1795–1803. doi:10.1038/bjc.2011.430
Niehrs, C. (2006). Function and biological roles of the Dickkopf family of Wnt modulators. Oncogene 25 (57), 7469–7481. doi:10.1038/sj.onc.1210054
Rawla, P. (2019). Epidemiology of prostate cancer. World J. Oncol. 10 (2), 63–89. doi:10.14740/wjon1191
Ryu, S. W., Kim, J. H., Kim, M. K., Lee, Y. J., Park, J. S., Park, H. M., et al. (2013). Reduced expression of DKK3 is associated with adverse clinical outcomes of uterine cervical squamous cell carcinoma. Int. J. Gynecol. Cancer 23 (1), 134–140. doi:10.1097/IGC.0b013e3182754feb
Tagai, E. K., Miller, S. M., Kutikov, A., Diefenbach, M. A., Gor, R. A., Al-Saleem, T., et al. (2019). Prostate cancer patients' understanding of the gleason scoring system: implications for shared decision-making. J. Cancer Educ. 34 (3), 441–445. doi:10.1007/s13187-018-1320-1
Ueno, K., Hirata, H., Majid, S., Chen, Y., Zaman, M. S., Tabatabai, Z. L., et al. (2011). Wnt antagonist DICKKOPF-3 (Dkk-3) induces apoptosis in human renal cell carcinoma. Mol. Carcinog. 50 (6), 449–457. doi:10.1002/mc.20729
Valkenburg, K. C., de Groot, A. E., and Pienta, K. J. (2018). Targeting the tumour stroma to improve cancer therapy. Nat. Rev. Clin. Oncol. 15 (6), 366–381. doi:10.1038/s41571-018-0007-1
van Leenders, G., Verhoef, E. I., and Hollemans, E. (2020). Prostate cancer growth patterns beyond the Gleason score: entering a new era of comprehensive tumour grading. Histopathology 77 (6), 850–861. doi:10.1111/his.14214
Veeck, J., and Dahl, E. (2012). Targeting the Wnt pathway in cancer: the emerging role of Dickkopf-3. Biochim. Biophys. Acta 1825 (1), 18–28. doi:10.1016/j.bbcan.2011.09.003
Vellky, J. E., and Ricke, W. A. (2020). Development and prevalence of castration-resistant prostate cancer subtypes. Neoplasia (New York, NY) 22 (11), 566–575. doi:10.1016/j.neo.2020.09.002
Wang, W., Zhu, W., Xu, X. Y., Nie, X. C., Yang, X., Xing, Y. N., et al. (2012). The clinicopathological significance of REIC expression in colorectal carcinomas. Histol. Histopathol. 27 (6), 735–743. doi:10.14670/HH-27.735
Wang, Z., Lin, L., Thomas, D. G., Nadal, E., Chang, A. C., Beer, D. G., et al. (2015). The role of Dickkopf-3 overexpression in esophageal adenocarcinoma. J. Thorac. Cardiovasc Surg. 150 (2), 377–385. doi:10.1016/j.jtcvs.2015.05.006
Warde-Farley, D., Donaldson, S. L., Comes, O., Zuberi, K., Badrawi, R., Chao, P., et al. (2010). The GeneMANIA prediction server: biological network integration for gene prioritization and predicting gene function. Nucleic Acids Res. 38 (Web Server issue), W214–W220. doi:10.1093/nar/gkq537
Xie, Z., Bailey, A., Kuleshov, M. V., Clarke, D. J. B., Evangelista, J. E., Jenkins, S. L., et al. (2021). Gene set knowledge discovery with Enrichr. Curr. Protoc. 1 (3), e90. doi:10.1002/cpz1.90
Xu, X. Y., Xia, P., Yu, M., Nie, X. C., Yang, X., Xing, Y. N., et al. (2012). The roles of REIC gene and its encoding product in gastric carcinoma. Cell. Cycle 11 (7), 1414–1431. doi:10.4161/cc.19823
Yamazaki, H., Suzuki, G., Masui, K., Aibe, N., Shimizu, D., Kimoto, T., et al. (2021). Novel prognostic index of high-risk prostate cancer using simple summation of very high-risk factors. Cancers (Basel) 13 (14), 3486. doi:10.3390/cancers13143486
Keywords: prostate cancer, Dickkopf-3, TGF-β signalling pathway, ECM-1, prognosis
Citation: Al Shareef Z, Hachim MY, Bouzid A, Talaat IM, Al-Rawi N, Hamoudi R and Hachim IY (2024) The prognostic value of Dickkopf-3 (Dkk3), TGFB1 and ECM-1 in prostate cancer. Front. Mol. Biosci. 11:1351888. doi: 10.3389/fmolb.2024.1351888
Received: 07 December 2023; Accepted: 06 May 2024;
Published: 24 May 2024.
Edited by:
Georgina Gonzalez-Avila, National Institute of Respiratory Diseases-Mexico (INER), MexicoReviewed by:
Yun-Sheng Chen, University of Illinois at Urbana-Champaign, United StatesSowmya Ramesh, Johns Hopkins University, United States
Copyright © 2024 Al Shareef, Hachim, Bouzid, Talaat, Al-Rawi, Hamoudi and Hachim. This is an open-access article distributed under the terms of the Creative Commons Attribution License (CC BY). The use, distribution or reproduction in other forums is permitted, provided the original author(s) and the copyright owner(s) are credited and that the original publication in this journal is cited, in accordance with accepted academic practice. No use, distribution or reproduction is permitted which does not comply with these terms.
*Correspondence: Ibrahim Y. Hachim, ihachim@sharjah.ac.ae
†These authors have contributed equally to this work
‡These authors have contributed equally to this work and share senior authorship