- 1Laboratory of Cellular and Molecular Pathology of Cardiovascular System, Avtsyn Research Institute of Human Morphology of FSBSI “Petrovsky National Research Centre of Surgery”, Moscow, Russia
- 2Department of Systemic Rheumatic Diseases, VA Nasonova Research Institute of Rheumatology, Moscow, Russia
- 3Faculty of Biology, Department of Biophysics, Lomonosov Moscow State University, Moscow, Russia
- 4Medical Institute, Peoples’ Friendship University of Russia named after Patrice Lumumba (RUDN University), Moscow, Russia
Introduction: Systemic scleroderma (SSc) is a chronic autoimmune disease of inflammatory origin. Mitochondrial dysfunction is considered as an important mechanism in the pathogenesis of SSc. Currently mitochondrial DNA (mtDNA) copy number is used as a surrogate marker of mitochondrial dysfunction. Previous studies demonstrate that innate immune cells are important participants in inflammatory and fibrotic processes in SSc. The aim of the study was to evaluate the number of mtDNA copies in CD14+ monocytes and whole blood of patients with SSc in comparison with healthy individuals.
Methods: Absolute mtDNA copy number was measured using digital PCR. It was found that the number of mtDNA copies in CD14+ monocytes was significantly higher in patients with SSc compared to control, while the number of mtDNA copies in the whole blood did not have significant differences.
Results: The correlation analysis revealed an inverse association of mtDNA copy number with disease duration and the relationship between pro-inflammatory activation of CD14+ monocytes in terms of LPS-stimulated IL-6 secretion and mtDNA copy number. At the same time, basal and LPS-stimulated secretion of IL-6 by cultured CD+ monocytes were significantly higher in SSc group in comparison with control.
Discussion: The study results suggest that increase of mtDNA copy number in CD14+ monocytes is a possible mechanism to maintain the reduced function of defective mitochondria in monocytes from patients with SSc associated with the development and progression of SSc.
1 Introduction
Systemic sclerosis (SSc) is an autoimmune disease characterized by fibrosis of the skin and internal organs, as well as vasculopathy. Despite of the fact that SSc is not a widespread disease, this pathology has the highest mortality rate among all rheumatic diseases (Volkmann et al., 2023). The etiology of SSc remains unknown; probably, the combined effect of genetic predisposition and external factors plays a decisive role in the induction of the disease (Rosendahl et al., 2022).
Mitochondria are considered as an important link in the regulation of both innate and adaptive immunity; mitochondrial dysfunction leads to disruption of the immune system (Xu et al., 2020). As a result of mitochondrial dysfunction, there is a disruption in the electron transport chain leading to an increase in reactive oxygen species, that, in turn, affects the secretion of pro-inflammatory cytokines, which leads to the activation and migration of immune cells to sites of inflammation (Zank et al., 2018). Mitochondrial DNA (mtDNA) copy number is considered as a surrogate marker of mitochondrial dysfunction. Changes in the amount of mtDNA can lead to increased oxidative stress and contribute to the development of inflammation (Malik and Czajka, 2013). A number of studies have shown that the mtDNA copy number in patients with autoimmune rheumatic diseases (ARDs) differs from healthy individuals (Svendsen et al., 2019; Giaglis et al., 2021).
Monocytes play an important role in the pathogenesis of SSc. Most peripheral blood CD14+ monocytes differentiate into tissue macrophages, which secrete proinflammatory cytokines and chemokines, leading to chronic inflammation in patients with SSc (Mathai et al., 2010). It is known that the number of CD14+ monocytes is increased in patients with SSc (Higashi-Kuwata et al., 2010). Increased level of circulating pro-inflammatory cytokines in SSc was demonstrated in several clinical trials (Lescoat et al., 2021). In particular, high serum levels of pro-inflammatory cytokine interleukin-6 (IL-6) and important role of IL-6 in the pathogenesis of SSc were demonstrated, that determined the use of IL-6 as a target for biological therapy of SSc (Sambo et al., 1999). Mitochondrial dysfunction in circulating monocytes may cause dysfunction of monocytes and lead to the progression of chronic inflammation in SSc.
The purpose of this study was to investigate the copy number of mtDNA in CD14+ monocytes and all cell populations circulating in the blood of patients with SSc and apparently healthy individuals and to assess the association of mtDNA copy number with pro-inflammatory status of monocytes assessed by IL-6 secretion by cultured monocytes under basal and pro-inflammatory conditions, mitochondrial activity, and clinical characteristics of patients with SSc.
2 Materials and methods
2.1 Study design
The study included 25 patients with SSc and a control group of 25 apparently healthy individuals without SSc and other autoimmune or chronic inflammatory diseases. SSc patients included in the study did not receive glucocorticoid therapy. Exclusion criteria were age younger than 20 years or older than 70 years; the presence of diabetes mellitus, cancer, decompensated renal or liver failure, chronic cardiovascular failure NYHA class III-IV.
Table 1 demonstrates the general characteristics, clinical and laboratory manifestations of SSc patients. Most patients (80%) were diagnosed with a limited form of SSc. The disease activity score for SSc patients was adapted by the European Scleroderma Study Group and the SSc Clinical Trials Consortium (Valentini et al., 2001). Deterioration of the skin (Δ skin), Raynaud’s syndrome (Δ vascular) and cardiovascular manifestations (Δ heart/lungs) in the month preceding hospitalization were identified when interviewing the patient. The presence of scleredema, i.e., swelling of the skin of the hands, digital necrosis and arthritis, was determined during examination. The study of external respiration function was carried out using a Master Screen PFT device (Viasys Healthcare, Germany). Spirometry and body plethysmography were performed to determine standard static and dynamic ventilation indicators. The diffusion capacity of the lungs was assessed by the single breath method and expressed as a percentage of the expected value; 80%–120% was considered normal values. The level of complement components (C3 and/or C4) was determined in the patient’s blood serum by immunonephelometry (BN ProSpec, Siemens); hypocomplementemia was considered as a decrease in the level below normal for each indicator; normal values were considered for C3 0.9–1.8 g/L, for C4 0.1–0.4 g/L. The clinical and laboratory parameters that made up the SSc activity index were recorded and scored, after which the overall SSc activity index was calculated. The mean value of the activity index was 2.1 (1.8) points. A low activity (<3 points) was detected in 76% of SSc patients, a high activity (≥3 points) was detected in 24% of patients (24%).
2.2 CD14+ monocytes isolation
CD14+ monocytes were isolated from whole blood samples using a standard method for isolating the leukocyte fraction in a Ficoll gradient, followed by the selection of CD14+ cells by magnetic separation on columns (Miltenyi Biotec, United States) using paramagnetic nanoparticles (Miltenyi Biotec, United States).
2.3 DNA isolation
Isolation of DNA from CD14+ monocytes and whole blood was carried out using the ExtractDNA Blood and Cells kit (Evrogen, Russia) according to the manufacturer’s protocol. The concentration and purity of the obtained DNA were determined using a BIOSPEC-NANO spectrophotometer (Shimadzu, Japan).
2.4 Quantitative determination of mtDNA copies
The copy number of mtDNA and nDNA was determined by digital PCR on a QIAcuity Eight instrument (Qiagen, Germany). For amplification, primers (Synthol, Russia) and the reaction mixture QuantiFast SYBR Green Master Mix (Qiagen, Germany) were used. PCR was performed for 42 cycles, with pre-denaturation for 2 min at 95°C for the first cycle, the next 40 cycles included denaturation for 15 s at 95°C, annealing for 30 s at 60°C and elongation for 30 s at 72°C, the final cycle (temperature extension) lasted 5 min at 40°C. For each test sample, the PCR reaction was performed at least three times.
PCR reactions were performed in a total volume of 12 μL using 5 μL of genomic DNA, 0.4 μL of forward and reverse primers, 4 μL of QuantiFast SYBR Green Master Mix and 2.2 μL of deionized water.
To determine the number of mtDNA and nDNA copies, primers F MT-ND4, R MT-ND4, F NCOA3, R NCOA3 were used. Nucleotide sequence F MT-ND4: 5′-ccattctcctcctatccctcaac-3′, R MT-ND4: 5′-cacaatctgatgttttggttaaactatattt-3′, F NCOA3: 5′-gagtttcctggacaaatgag-3′, R NCOA3: 5′-cattgtttcatatctctggcg-3′. Primers were selected based on literature data (Bai and Wong, 2005; Gu et al., 2013). The value of mtDNA copy number per cell that was used for analysis was calculated as a ratio of mtDNA and nDNA copies (Shoop et al., 2022).
The researchers were blinded to the group status and clinical characteristics of the study participants.
2.5 Analysis of IL-6 secretion by cultured CD14+ monocytes
CD14+ monocytes obtained by immunomagnetic separation as described above, were seeded into two wells of a 48-well plate at a rate of 500,000 cells per well and cultured in 0.5 mL of serum-free X-VIVO medium (Lonza, Germany) containing L-glutamine, gentamicin and phenol red at 37°C. In the first well cells were cultivated under basal conditions. In the second well, the inflammatory response of monocytes was stimulated by the addition of LPS from Escherichia coli, serotype 026:B6 (Sigma-Aldrich, Israel) at a concentration 1 μg/mL. The cells were cultured for 24 h, then the samples of culture fluid were collected and stored at −70°C for analysis of IL-6 secretion. The concentration of IL-6 in culture fluid samples was determined by ELISA using a commercial Human IL-6 DuoSet ELISA kit (R&D Systems, United States).
2.6 Analysis of mitochondrial activity
The MitoTracker Orange staining assay was performed to evaluate the mitochondrial activity. The isolated CD14+ monocytes in the amount of 1 million cells were resuspended in the medium in eppendorf-type test tubes with the addition of MitoTracker orange dye (Thermo Fisher Scientific, United States) at a concentration of 2 μL/mL and were incubated at 37°C with an open lid for 30 min. Then the cells were centrifugated at 8,000–13,000 g for 6 min. The fluorescence intensity was analyzed with flow cytometer MACSQuant VYB (Miltenyi Biotec, Germany). The obtained mean values of fluorescence intensity were used for statistical processing.
2.7 Statistical analysis
The sample size was estimated using Cochran’s equation together with a correction for small populations (2–12 cases per million people) of a known size, to calculate sample size for Precision Level 0.05, Confidence Level 95%, Estimated Proportion 0.5. Statistical analysis of the obtained data was carried out using the R programming language for statistical computing. The Mann-Whitney U test was used to assess a statistically significant difference between the two samples. The data on clinical characteristics of study participants are presented as mean value and standard deviation [Mean (SD)]. The obtained values of mtDNA copy number are presented as median and quartiles [Me (Q1, Q3)]. Pearson correlation analysis was performed to investigate the association of mtDNA copy number with clinical characteristics of SSc study participants.
3 Results
A total of 50 participants were included in the study: 25 patients with SSc and 25 control participants without SSc. The mean age of study participants was 47.9 (12.1) years old in the group of SSc patients and 49.7 (9.7) years old in the control group, p = 0.556. Study groups were also matched by gender, 80% of SSc patients and 72% of control study participants were female, p = 0.518. The study was conducted in accordance with the Declaration of Helsinki of 1975 and its revised version of 2013. The study protocol was approved by the Local Ethics Committee of the Nasonova Research Institute of Rheumatology at 10 February 2022. All participants provided written informed consent to participate in the study.
The number of mtDNA copies was measured in CD14+ monocytes and all cell populations circulating in the blood of patients with SSc and apparently healthy individuals. It was shown that the absolute number of mtDNA copies of CD14+ monocytes was significantly higher in the group of patients with SSc, 108 [60–162] compared to the control group, 72 [59–79], p = 0.011*, while the absolute number of mtDNA copies of all cell populations circulating in the blood was decreased in SSc patients, 109 [72–171] than in control individuals, 128 [85–227], but the difference between groups was not significant, p = 0.171. The obtained data are presented in Figure 1.
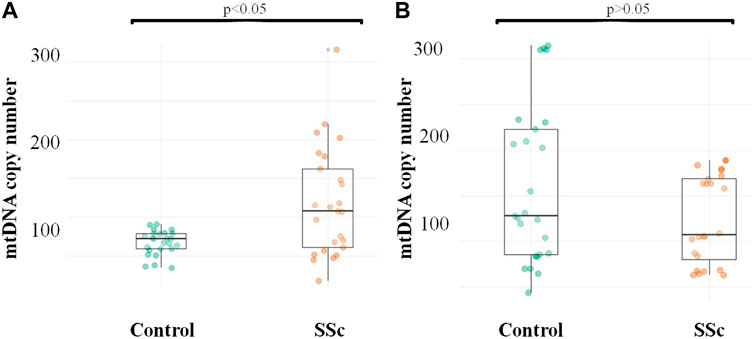
FIGURE 1. mtDNA copy number in CD14+ monocytes and whole blood of patients with SSc and healthy study participants (A) Quantification of CD14+ monocyte mtDNA; (B) Quantification of mtDNA of all cell populations circulating in the blood.
The analysis of association of mtDNA copy number with clinical characteristics of patients with SSc revealed the significant inverse correlation of mtDNA copy number in CD14+ monocytes with disease duration with r = −0.420, p = 0.037* (Figure 2). The inverse relationship was also observed between C14+ mtDNA copy number and disease activity, however, the correlation was not statistically significant, r = −0.315, p = 0.254. No association of mtDNA copy number in CD14+ monocytes and other clinical characteristics of patients with SSc was observed as well as no clinical characteristics correlated with mtDNA copy number in whole blood samples of patients with SSc.
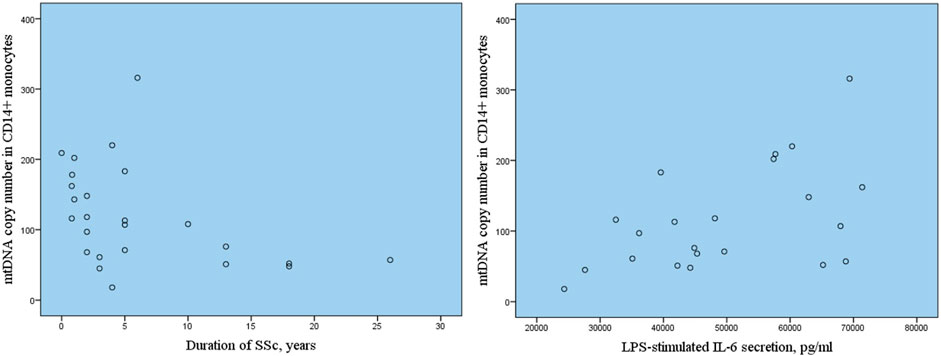
FIGURE 2. Correlation of mtDNA copy number in CD14+ monocytes with SSc duration and LPS-stimulated secretion of pro-inflammatiry cytokine IL-6.
Next the association of mtDNA copy number in CD14+ monocytes with inflammatory activation of monocytes assessed by basal and LPS-stimulated secretion of pro-inflammatory cytokine Il-6 was investigated. Table 2 presents the results of measurements of Il-6 secretion by cultured monocytes of study participants with SSc in comparison with apparently healthy individuals. It was demonstrated that basal and LPS-stimulated secretion of IL-6 was significantly higher in SSc group in comparison with control group. The correlation analysis demonstrated that increased LPS-stimulated secretion of IL-6 by cultured CD14+ monocytes of study participants with SSc was associated with higher mtDNA copy number in CD14+ monocytes, r = 0.569, p = 0.006*.
The mitochondrial activity was analyzed using MitoTracker Orange staining. The mean fluorescence intensity of CD14+ monocytes when stained with MitoTracker Orange was 681 (153) in SSc group vs. 685 (89) in control, p = 0.897.
4 Discussion
The present study quantified changes in mtDNA copy number in patients with SSc compared with apparently healthy individuals without ARD. The results demonstrate a significant increase in mtDNA copy number in CD14+ monocytes in patients with SSc compared to controls. Based on our search of the PubMed database, we failed to find published data on the measurements of mtDNA copy number in monocytes from patients with SSc as well as other ARDs. At the same time, insignificant decrease of mtDNA copy number was demonstrated in whole blood of SSc patients. It is known that most studies on mtDNA copy number in diseases associated with mitochondrial dysfunction demonstrate a decrease in mtDNA copy number compared to healthy controls (Ding et al., 2023; Malik, 2023). The previous study on the number of mtDNA copies in the peripheral blood of patients with SSc showed that mtDNA copy number was lower in SSc patients in comparison with healthy participants of the study (Movassaghi et al., 2020). It can be assumed that the differences in mtDNA copy number between SSc and control groups in the current study did not reach statistical significance due to insufficient sample size, while the published study included 46 patients with SSc vs. 49 healthy individuals. However, studies of mtDNA copy number in other ARDs have demonstrated controversial results. In particular, the number of plasma mtDNA copies was increased by 8.8 times in systemic lupus erythematosus compared to the control group (Giaglis et al., 2021). Another recent study demonstrated that mtDNA copy number was statistically higher in blood samples of patients with ankylosing spondylitis in comparison with control subjects (Zhang et al., 2023).
Another important finding of the study was the association of SSc duration and mtDNA copy number in CD14+ monocytes. It has been shown that patients with longer duration of SSc have lower mtDNA copy number. The number of mtDNA copies reflects the number of mitochondria in a cell. Presumably, the increase in the number of mitochondria in CD14+ monocytes may be a consequence of a compensatory mechanism that is necessary to maintain the reduced function of defective mitochondria in monocytes of patients with shorter duration of SSc. At the same time, in patients with a longer duration of the disease mtDNA copy number decreases due to elimination of dysfunctional mitochondria. In this study mitochondrial activity was analyzed using MitoTracker Orange staining. MitoTracker Orange is a mitochondrial dye which is sensitive to mitochondrial potential that can be used as an indicator of mitochondrial activity and oxidative stress (Tomkova et al., 2018). The difference of fluorescence intensity after MitoTracker Orange staining of CD14+ monocytes was not observed between SSc and control despite of increase of mtDNA copy number in monocytes of SSc patients that may explain the compensation of mitochondria function by increasing their number especially taking the fact that mtDNA copy number decreased with disease duration. Mitophagy is a key mechanism aimed to control and remove damaged mitochondria from the cell (Fu et al., 2023). It was previously demonstrated in animal model of SSc using Caveolin-1 (−/−) null mice that mitophagy was increased in the stromal cells of the dermis along with other signs characterizing changes in the skin in SSc (Castello-Cros et al., 2011). The study in patients with another autoimmune disease, Sjögren’s syndrome have shown the decrease of mtDNA copy number and the increase of the expression of genes associated with mitochondrial dynamics in the peripheral blood of patients with Sjögren’s syndrome in comparison with healthy subjects (De Benedittis et al., 2022).
Damaged mitochondria can cause cell death, after which the release of mtDNA contributes to increased systemic inflammation due to the activation of immune cells (Faas and de Vos, 2020). The fact that in this study mtDNA copy number disturbance was demonstrated only in CD14+ monocytes, while in whole blood there was no difference in mtDNA copy number between SSc and controls, allows considering mtDNA copy number in CD14+ monocytes as a potential indicator of mitochondrial dysfunction of monocytes in SSc and confirms the important role of CD14+ monocytes in pathogenesis of SSc. Monocytes have been shown to be important participants in inflammatory and fibrotic processes in SSc through the overproduction of inflammatory cytokines (IL-6, IL-8), chemokines (CCL2, CXCL10), and growth factors (Carvalheiro et al., 2020; Rudnik et al., 2021). The results of the current study demonstrated significantly increased secretion of pro-inflammatory cytokine IL-6 by cultured monocytes of SSc patients under basal and pro-inflammatory conditions in comparison with healthy participants of the study. The increased LPS-stimulated secretion of IL-6 correlated with higher number of mtDNA copies in CD14+ monocytes that suggests the important role of mitochondrial dysfunction in pro-inflammatory monocyte activation in SSc pathogenesis. Several recent studies on mtDNA copy number, mitochondrial heteroplasmy and mitophagy in other ARD report that mitochondrial dysfunction is involved in the activation of innate immune system in the pathogenesis of systemic lupus erythematosus and rheumatoid arthritis (Veale et al., 2017; Quintero-González et al., 2022). The recent study on dermal fibroblasts isolated from SSc and healthy donors demonstrated higher levels of mitochondrial reactive oxygen species (ROS) in SSc fibroblasts along with increased secretion of inflammatory cytokines IL-6 and IL-8 induced by oxidative stress (Kizilay Mancini et al., 2022).
The current study had some limitations. The sample size was limited due to low prevalence of SSc, taking that study participants with SSc must not receive glucocorticoid therapy according to the inclusion criteria. This study was a pilot project aimed to assess the phenomenon of mtDNA copy number variation in SSc both in whole blood and CD14+ monocytes, so another important limitation of the study was the fact that the investigation of mitochondrial dysfunction parameters such as ROS production, and mitophagy, as well as mitochondrial heteroplasmy were not performed.
The cause of mitochondrial dysfunction may be mitochondrial heteroplasmy. It has been previously shown that mtDNA copy number decreases with age, while the level of mitochondrial heteroplasmy increases (Stifano and Christmann, 2016). The study on the association of mitochondrial heteroplasmy with the number of mtDNA copies, which reflects mitochondrial dysfunction, is a promising direction for further research of the pathogenesis of SSc as well as other ARDs.
Data availability statement
The original contributions presented in the study are included in the article/supplementary material, further inquiries can be directed to the corresponding author.
Ethics statement
The studies involving humans were approved by the Local Ethics Committee of the Nasonova Research Institute of Rheumatology. The studies were conducted in accordance with the local legislation and institutional requirements. The participants provided their written informed consent to participate in this study. Written informed consent was obtained from the individual(s) for the publication of any potentially identifiable images or data included in this article.
Author contributions
AB: Writing–original draft, Writing–review and editing. EG: Resources, Writing–review and editing. TK: Writing–original draft, Visualization. YM: Validation, Writing–review and editing. TT: Investigation, Writing–original draft. DK: Software, Writing–review and editing. TP: Resources, Writing–review and editing. AM: Conceptualization, Writing–review and editing.
Funding
The author(s) declare financial support was received for the research, authorship, and/or publication of this article. This work was supported by the Russian Science Foundation (Grant # 22-25-00358).
Conflict of interest
The authors declare that the research was conducted in the absence of any commercial or financial relationships that could be construed as a potential conflict of interest.
Publisher’s note
All claims expressed in this article are solely those of the authors and do not necessarily represent those of their affiliated organizations, or those of the publisher, the editors and the reviewers. Any product that may be evaluated in this article, or claim that may be made by its manufacturer, is not guaranteed or endorsed by the publisher.
References
Bai, R. K., and Wong, L. J. C. (2005). Simultaneous detection and quantification of mitochondrial DNA deletion(s), depletion, and over-replication in patients with mitochondrial disease. J. Mol. Diagn. 7, 613–622. doi:10.1016/S1525-1578(10)60595-8
Carvalheiro, T., Lopes, A. P., van der Kroef, M., Malvar-Fernandez, B., Rafael-Vidal, C., Hinrichs, A. C., et al. (2020). Angiopoietin-2 promotes inflammatory activation in monocytes of systemic sclerosis patients. Int. J. Mol. Sci. 21, 9544–9610. doi:10.3390/IJMS21249544
Castello-Cros, R., Whitaker-Menezes, D., Molchansky, A., Purkins, G., Soslowsky, L. J., Beason, D. P., et al. (2011). Scleroderma-like properties of skin from caveolin-1-deficient mice: implications for new treatment strategies in patients with fibrosis and systemic sclerosis. Cell Cycle 10, 2140–2150. doi:10.4161/CC.10.13.16227
De Benedittis, G., Latini, A., Colafrancesco, S., Priori, R., Perricone, C., Novelli, L., et al. (2022). Alteration of mitochondrial DNA copy number and increased expression levels of mitochondrial dynamics-related genes in sjögren’s syndrome. Biomedicines 10, 2699. doi:10.3390/BIOMEDICINES10112699
Ding, X., Fang, T., Pang, X., Pan, X., Tong, A., Lin, Z., et al. (2023). Mitochondrial DNA abnormalities and metabolic syndrome. Front. Cell Dev. Biol. 11, 1153174. doi:10.3389/FCELL.2023.1153174
Faas, M. M., and de Vos, P. (2020). Mitochondrial function in immune cells in health and disease. Biochim. Biophys. acta. Mol. basis Dis. 1866, 165845. doi:10.1016/J.BBADIS.2020.165845
Fu, T., Ma, Y., Li, Y., Wang, Y., Wang, Q., and Tong, Y. (2023). Mitophagy as a mitochondrial quality control mechanism in myocardial ischemic stress: from bench to bedside. Cell Stress Chaperones 28, 239–251. doi:10.1007/S12192-023-01346-9
Giaglis, S., Daoudlarian, D., Voll, R. E., Kyburz, D., Venhoff, N., and Walker, U. A. (2021). Circulating mitochondrial DNA copy numbers represent a sensitive marker for diagnosis and monitoring of disease activity in systemic lupus erythematosus. RMD open 7, e002010. doi:10.1136/RMDOPEN-2021-002010
Gu, F., Chauhan, V., Kaur, K., Brown, W. T., LaFauci, G., Wegiel, J., et al. (2013). Alterations in mitochondrial DNA copy number and the activities of electron transport chain complexes and pyruvate dehydrogenase in the frontal cortex from subjects with autism. Transl. Psychiatry 39 (3), e299. doi:10.1038/tp.2013.68
Higashi-Kuwata, N., Jinnin, M., Makino, T., Fukushima, S., Inoue, Y., Muchemwa, F. C., et al. (2010). Characterization of monocyte/macrophage subsets in the skin and peripheral blood derived from patients with systemic sclerosis. Arthritis Res. Ther. 12, R128. doi:10.1186/AR3066
Kizilay Mancini, O., Acevedo, M., Fazez, N., Cuillerier, A., Fernandez Ruiz, A., Huynh, D. N., et al. (2022). Oxidative stress-induced senescence mediates inflammatory and fibrotic phenotypes in fibroblasts from systemic sclerosis patients. Rheumatol. Oxf. 61, 1265–1275. doi:10.1093/RHEUMATOLOGY/KEAB477
Lescoat, A., Lecureur, V., and Varga, J. (2021). Contribution of monocytes and macrophages to the pathogenesis of systemic sclerosis: recent insights and therapeutic implications. Curr. Opin. Rheumatol. 33, 463–470. doi:10.1097/BOR.0000000000000835
Malik, A. N. (2023). Mitochondrial DNA - novel mechanisms of kidney damage and potential biomarker. Curr. Opin. Nephrol. Hypertens. 32, 528–536. doi:10.1097/MNH.0000000000000922
Malik, A. N., and Czajka, A. (2013). Is mitochondrial DNA content a potential biomarker of mitochondrial dysfunction? Mitochondrion 13, 481–492. doi:10.1016/J.MITO.2012.10.011
Mathai, S. K., Gulati, M., Peng, X., Russell, T. R., Shaw, A. C., Rubinowitz, A. N., et al. (2010). Circulating monocytes from systemic sclerosis patients with interstitial lung disease show an enhanced profibrotic phenotype. Lab. Invest. 90, 812–823. doi:10.1038/LABINVEST.2010.73
Movassaghi, S., Jafari, S., Falahati, K., Ataei, M., Sanati, M. H., and Jadali, Z. (2020). Quantification of mitochondrial DNA damage and copy number in circulating blood of patients with systemic sclerosis by a qPCR-based assay. An. Bras. Dermatol. 95, 314–319. doi:10.1016/J.ABD.2019.11.003
Quintero-González, D. C., Muñoz-Urbano, M., and Vásquez, G. (2022). Mitochondria as a key player in systemic lupus erythematosus. Autoimmunity 55, 497–505. doi:10.1080/08916934.2022.2112181
Rosendahl, A. H., Schönborn, K., and Krieg, T. (2022). Pathophysiology of systemic sclerosis (scleroderma). Kaohsiung J. Med. Sci. 38, 187–195. doi:10.1002/KJM2.12505
Rudnik, M., Hukara, A., Kocherova, I., Jordan, S., Schniering, J., Milleret, V., et al. (2021). Elevated fibronectin levels in profibrotic CD14+ monocytes and CD14+ macrophages in systemic sclerosis. Front. Immunol. 12, 642891. doi:10.3389/fimmu.2021.642891
Sambo, P., Jannino, L., Candela, M., Salvi, A., Donini, M., Dusi, S., et al. (1999). Monocytes of patients wiht systemic sclerosis (scleroderma spontaneously release in vitro increased amounts of superoxide anion. J. Invest. Dermatol. 112, 78–84. doi:10.1046/J.1523-1747.1999.00476.X
Shoop, W. K., Gorsuch, C. L., Bacman, S. R., and Moraes, C. T. (2022). Precise and simultaneous quantification of mitochondrial DNA heteroplasmy and copy number by digital PCR. J. Biol. Chem. 298, 102574. doi:10.1016/J.JBC.2022.102574
Stifano, G., and Christmann, R. B. (2016). Macrophage involvement in systemic sclerosis: do we need more evidence? Curr. Rheumatol. Rep. 18, 2–6. doi:10.1007/S11926-015-0554-8
Svendsen, A. J., Tan, Q., Jakobsen, M. A., Thyagarajan, B., Nygaard, M., Christiansen, L., et al. (2019). White blood cell mitochondrial DNA copy number is decreased in rheumatoid arthritis and linked with risk factors. A twin study. J. Autoimmun. 96, 142–146. doi:10.1016/J.JAUT.2018.09.008
Tomkova, S., Misuth, M., Lenkavska, L., Miskovsky, P., and Huntosova, V. (2018). In vitro identification of mitochondrial oxidative stress production by time-resolved fluorescence imaging of glioma cells. Biochim. Biophys. Acta Mol. Cell Res. 1865, 616–628. doi:10.1016/j.bbamcr.2018.01.012
Valentini, G., Della Rossa, A., Bombardieri, S., Bencivelli, W., Silman, A. J., D’Angelo, S., et al. (2001). European multicentre study to define disease activity criteria for systemic sclerosis. II. Identification of disease activity variables and development of preliminary activity indexes. Ann. Rheum. Dis. 60, 592–598. doi:10.1136/ARD.60.6.592
Veale, D. J., Orr, C., and Fearon, U. (2017). Cellular and molecular perspectives in rheumatoid arthritis. Semin. Immunopathol. 39, 343–354. doi:10.1007/S00281-017-0633-1
Volkmann, E. R., Andréasson, K., and Smith, V. (2023). Systemic sclerosis. Lancet (London, Engl. 401, 304–318. doi:10.1016/S0140-6736(22)01692-0
Xu, Y., Shen, J., and Ran, Z. (2020). Emerging views of mitophagy in immunity and autoimmune diseases. Autophagy 16, 3–17. doi:10.1080/15548627.2019.1603547
Zank, D. C., Bueno, M., Mora, A. L., and Rojas, M. (2018). Idiopathic pulmonary fibrosis: aging, mitochondrial dysfunction, and cellular bioenergetics. Front. Med. 5, 10. doi:10.3389/FMED.2018.00010
Keywords: DNA, mitochondrial, monocytes, systemic sclerosis, autoimmunity, inflammation
Citation: Bogatyreva AI, Gerasimova EV, Kirichenko TV, Markina YV, Tolstik TV, Kiseleva DG, Popkova TV and Markin AM (2023) Mitochondrial DNA copy number in patients with systemic sclerosis. Front. Mol. Biosci. 10:1313426. doi: 10.3389/fmolb.2023.1313426
Received: 10 October 2023; Accepted: 04 December 2023;
Published: 14 December 2023.
Edited by:
Robert Gilkerson, The University of Texas Rio Grande Valley, United StatesReviewed by:
Amit Kishore, Accuscript Consultancy, IndiaRuheena Javed, University of New Mexico, United States
Copyright © 2023 Bogatyreva, Gerasimova, Kirichenko, Markina, Tolstik, Kiseleva, Popkova and Markin. This is an open-access article distributed under the terms of the Creative Commons Attribution License (CC BY). The use, distribution or reproduction in other forums is permitted, provided the original author(s) and the copyright owner(s) are credited and that the original publication in this journal is cited, in accordance with accepted academic practice. No use, distribution or reproduction is permitted which does not comply with these terms.
*Correspondence: Anastasia I. Bogatyreva, bmFzdHlhLmJvZ2F0eXJldmEuOTZAbWFpbC5ydQ==