- 1Department of Thoracic Surgery, Suining Central Hospital, An Affiliated Hospital of Chongqing Medical University, Suining, China
- 2Institute of Surgery, Graduate School, Chengdu University of Traditional Chinese Medicine, Chengdu, China
- 3Department of Physical Examination, Suining Central Hospital, An Affiliated Hospital of Chongqing Medical University, Suining, China
- 4Institute of Surgery, Graduate School, Zunyi Medical University, Zunyi, China
- 5Institute of Surgery, Graduate School, North Sichuan Medical College, Nanchong, China
- 6Department of Respiratory and Critical Care Medicine, Suining Central Hospital, An Affiliated Hospital of Chongqing Medical University, Suining, China
Non-small cell lung cancer (NSCLC) is one of the main causes of cancer-related death worldwide, with a serious impact on human health and life. The identification of NSCLC at an early stage is a formidable task that frequently culminates in a belated diagnosis. LncRNA is a kind of noncoding RNA with limited protein-coding capacity, and its expression is out of balance in many cancers, especially NSCLC. A large number of studies have reported that lncRNA acts a vital role in regulating angiogenesis, invasion, metastasis, and the proliferation and apoptosis of tumor cells, affecting the occurrence and development of NSCLC. Abundant evidence demonstrates that lncRNAs may serve as potential biomarkers for NSCLC diagnosis and prognosis. In this review, we summarize the latest progress in characterizing the functional mechanism of lncRNAs involved in the development of NSCLC and further discuss the role of lncRNAs in NSCLC therapy and chemotherapy resistance. We also discuss the advantages, limitations, and challenges of using lncRNAs as diagnostic or prognostic biomarkers in the management of NSCLC.
1 Introduction
Lung cancer has the second-highest cancer incidence and accounts for the majority of cancer deaths worldwide (1,800,000 deaths per year) based on the Global Cancer Observatory in 2020 (Sung et al., 2021). According to histopathology, lung cancer is composed of two different types: small cell lung cancer (SCLC) and non-small cell lung cancer (NSCLC). Non-small cell lung cancer accounts for about 85% of the total number of lung cancers, mainly including adenocarcinoma (AC), squamous cell carcinoma (SCC) and large cell carcinoma (Wang C. et al., 2020). Most patients with NSCLC are diagnosed at a late stage since there are no specific clinical symptoms in the early stages (Tsiouda et al., 2020). Surgery, chemotherapy and targeted therapy are currently useful treatments, but the 5 years survival rate in NSCLC patients remains below 15% (Huang W. et al., 2020). Thus, patients’ survival and outcome depend on the early detection of NSCLC. Low-dose CT (LDCT) screening is the primary means of early diagnosis. However, LDCT has two disadvantages: 1) the false-positive rate is high, which can easily lead to overdiagnosis (Aberle et al., 2011), and 2) accumulated radiation from screening and follow-up causes an increased risk of cancer (McCunney and Li, 2014). The progression of NSCLC is a biological process regulated by various factors. Therefore, there is a demand to further illustrate the mechanisms of NSCLC occurrence and seek more reliable diagnostic biomarkers.
Long noncoding RNAs (lncRNAs) are a type of RNA with more than 200 nucleotides (Quinn and Chang, 2016). Due to the lack of long protein-coding open reading frames, lncRNAs are widely recognized as not having the ability to encode proteins (Zhang et al., 2023b). Emerging evidence suggests that some lncRNAs do contain small open reading frames that can be translated by ribosomes to encode peptides (Zhang et al., 2023c; Pei et al., 2023). In transcription, lncRNA is a by-product produced by RNA polymerase II, which is initially considered as a junk fragment (Entezari et al., 2022). In 1991, Borsani et al. (1991) confirmed that lncRNA XIST is correlated with the inactivation of the x chromosome. Since then, more and more studies have shown that lncRNA is essential for biological function. The abnormal expression of lncRNA usually causes various diseases, particularly malignancy (Beermann et al., 2016). LncRNA expression is differential between carcinoma and para-carcinoma tissues, suggesting its relevance to cancer occurrence and progression. For example, the expression of a novel lncRNA MCM3AP-AS1 in hepatocellular carcinoma tissues is significantly higher than that in normal liver tissues (Wang Y. et al., 2019). LncRNA-CDC6 expression increases in breast cancer tissue and its expression is closely associated with the progression of breast cancer (Kong et al., 2019).
Research on lncRNAs in recent years has shown that lncRNAs regulate target genes through epigenetics, transcriptional regulation, and post-transcriptional regulation (Zhang et al., 2021b; Du et al., 2021). Diverse biological processes can be regulated by lncRNAs, including cell proliferation, apoptosis, invasion, metastasis and drug resistance (Lin and Yang, 2018). In addition, some lncRNAs, such as HOTAIR, NEAT1, MALAT1 and MEG3, have been shown to play positive or negative regulatory roles during malignant tumor progression (Kim et al., 2018; Esposito et al., 2019). In various human body fluids, such as plasma (Lin et al., 2022), sputum (Gupta et al., 2019), saliva (Shieh et al., 2021) and urine (Huang et al., 2021), lncRNA can be detected easily and stably, and its expression varies with disease progression. Therefore, detecting lncRNA expression can be used as a new strategy for the early diagnosis and prognosis prediction of NSCLC.
In this review, we briefly describe the classification and biological functions of lncRNA and outline the roles of lncRNA in lung cancer, particularly NSCLC. We further summarize the diagnosis and limitations of lncRNAs in NSCLC. Finally, we summarize the role of lncRNAs as therapeutic targets and prognostic predictive markers in NSCLC and detail the possible challenges in this field.
2 Classification and molecular biological functions of lncRNA
Protein-coding genes make up only 2% of the human genome, while the remaining 98% do not encode proteins (Qian et al., 2019). Functional RNA that does not encode proteins is called non-coding RNA (ncRNA) (Zhang et al., 2020). Depending on the length, ncRNA is classified into small noncoding RNA and lncRNA (Li L. et al., 2021). LncRNA is transcribed by RNA polymerase II. After transcription, similar to mRNA, lncRNA is usually capped by 7-methyl guanosine (m7G) at its 5′ ends and polyadenylated at its 3′ ends (Statello et al., 2021). However, compared with mRNA, the length of lncRNA is shorter, the exons are fewer, and the primary sequence is less conservative (Lagarde et al., 2017). There are several methods used for classifying lncRNAs, one of which categorizes lncRNAs based on their location relative to protein-coding genes (Figure 1): 1) sense lncRNA; 2) antisense lncRNA; 3) intronic lncRNA; 4) bidirectional lncRNA; and 5) intergenic lncRNA (Yousefi et al., 2020).
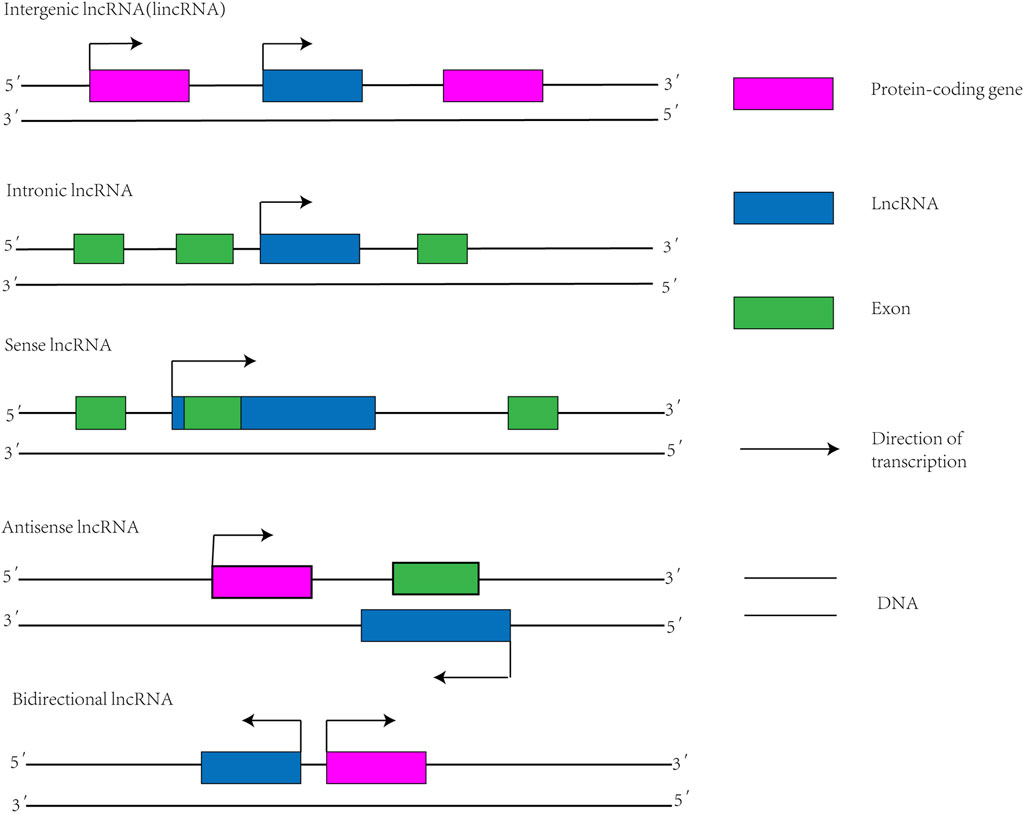
FIGURE 1. Classification diagram of lncRNAs. Intergenic lncRNA: also known as lincRNA, located between two protein-coding genes and capable of independent transcription. Intronic lncRNA: A transcript that is located in the intronic region of a protein-coding gene and has no overlap with its exon. Sense lncRNA: Transcribed from the justice chain of a protein-coding gene, overlapping with at least one exon of the protein-coding gene located on the same chain and transcribed in the same direction. Antisense lncRNA: Transcription by DNA strands complementary to protein-coding genes that are transcribed in opposite directions and overlap at least one exon of the forward gene. Bidirectional lncRNA: Shares promoters with protein-coding genes, but transcribes in the opposite direction to protein-coding genes.
In some studies, the function of lncRNAs is determined by their structure. Among them, secondary structures play a crucial role in lncRNAs (Derrien et al., 2012). Base complementary pairing forms the secondary structure of lncRNAs, which includes bulges, junctions, hairpin loops, stem loops, inner loops, helices, subdomains, and pseudoknots (Cruz and Westhof, 2009). Studies have shown that these secondary structures increase the stability of lncRNAs and affect their functional interactions with proteins, DNA and other RNAs (Pidíková and Herichová, 2021). For instance, MALAT1, which contains uracil-rich regions, forms a triple helix to increase stability (Zhang Y. et al., 2019). The lncRNA GAS5 signals a negative regulatory effector and has an A-type double helix structure. Its double helix structure interacts with the DNA-binding domain of the steroid receptor to repress steroid-mediated transcription (Hudson et al., 2014). These demonstrate that there is an important role for the secondary structure of lncRNAs in biological functions.
There are three main ways in which lncRNAs regulate genes: epigenetic regulation, transcriptional and post-transcriptional regulation, and their biological function depends on their location in the cell (Jia et al., 2022). In the nucleus, some lncRNAs can become histone modifiers through methylation or demethylation, thereby regulating the chromatin state and ultimately promoting or repressing gene transcription (Herman et al., 2022). LncRNA HOTAIR interacts with polycomb repressive complex 2 (PRC2) and promotes trimethylation of histone H3 Lys 27, thereby suppressing gene expression by epigenetic mechanisms (Balas et al., 2021). Moreover, the regulation of protein-coding genes by lncRNAs has also been proven through both cis-acting or trans-acting mechanisms in transcription (Ponting et al., 2009; Le Beguec et al., 2018). LncRNAs can modulate gene expression through three different regulatory mechanisms in cis-regulation: 1) lncRNA transcripts recruit specific transcription factors that regulate gene regulation. 2) LncRNAs directly regulate the expression of adjacent genes. 3) DNA sequences within a lncRNA locus can activate or repress the expression of genes in its vicinity (Merry et al., 2015). For example, XIST can silence genes present on the X chromosome by recruiting specific silencing factors (Markaki et al., 2021). In the cytoplasm, LncRNA can act as a molecular sponge for miRNA to regulate gene expression, thus reducing the targeting effect of miRNA on mRNA. This process is called endogenous competitive RNA (ceRNA) mechanism (Karreth and Pandolfi, 2013). Further, LncRNA forms a specific lncRNA protein complex (lncRNPs) with RNA binding proteins, which leads to changes in mRNA splicing and transcription and regulates signal pathways in some biological environments (Jonas et al., 2020; Cao et al., 2023). The lncRNA PTTG3P forms an RNA-protein complex with ILF3, which increases the stability of MAP2K6 and E2F1 mRNAs, thereby promoting NSCLC progression (Wang et al., 2023).
3 LncRNA and the occurrence and progression of NSCLC
The occurrence and progression of NSCLC are caused by dysregulated gene expression, usually involving oncogene activation and tumor suppressor gene inhibition. LncRNA can act as a key regulator to affect tumor cell proliferation and apoptosis, tumor angiogenesis, and tumor invasion and metastasis (Figure 2). Table 1 shows the mechanism of lncRNAs in the progression of NSCLC.
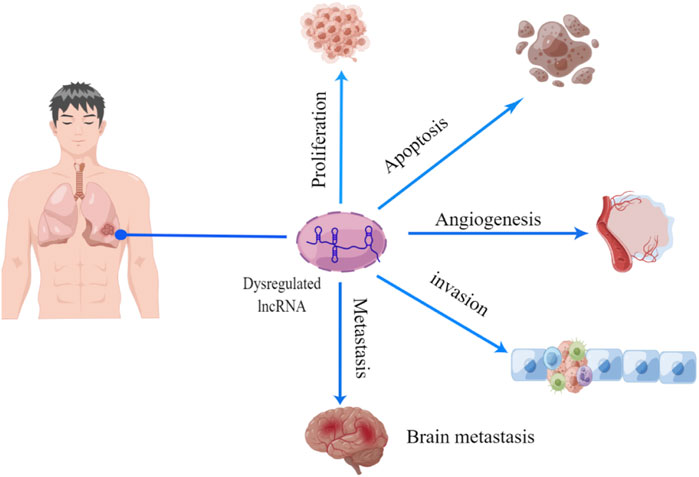
FIGURE 2. Function of lncRNA in NSCLC progression. There is growing evidence that lncRNA act as a series of novel regulators of tumorigenesis, including cell proliferation, apoptosis, angiogenesis, migration, and invasion.
3.1 LncRNAs regulate the proliferation and apoptosis of NSCLC
The infinite proliferation of cells is one of the ten attributes of tumors (Hanahan, 2022). Several researchers verified that lncRNAs can affect NSCLC proliferation by interacting with miRNAs. For instance, an increase in lncRNA small nucleolar RNA host gene 20 (SNHG20) expression in NSCLC is associated with an unfavorable prognosis. The upregulation of SNHG20 can improve proliferation and inhibit apoptosis. Mechanistically, SNHG20 increases the expression of ZEB2 and RUNX2 through sponging miR-154 to ultimately promote NSCLC progression (Lingling et al., 2019). Metadherin (MTDH) enables cancer cells to adhere tightly to blood vessels and consequently reach other distant organs. High expression of lncRNA prostate cancer non-coding RNA 1 (PRNCR1) leads to significant overexpression of MTDH through sponging miR-126-5p to promote the proliferation of cancer cells. Conversely, the knockdown of PNCR1 promotes apoptosis and hinders proliferation in NSCLC cells. The use of miR-126-5p inhibitors eliminates this effect, suggesting that PNCR1 is able to regulate NSCLC proliferation and metastasis by sponging miR-126-5p (Guo et al., 2020). The proliferation rate of tumor cells can be boosted by the KLF12 protein. By inhibiting miR-188-5p, lncRNA DARS-AS1 can upregulate the concentration of KLF12 in cells, thereby promoting the proliferation and invasion of lung adenocarcinoma (LUAD) (Liu et al., 2021).
On the other hand, lncRNA regulates the proliferation and apoptosis of tumor cells by affecting cell cycle. Cyclin D1, as a key regulator of the cell cycle, is primarily responsible for promoting cell proliferation (Liu et al., 2018). According to some studies, the expression of lncRNA MIR503HG is downregulated in NSCLC. Using overexpression experiments, overexpression of MIR503HG inhibits cyclin D1 expression and prevents cell division in the G1 cycle, resulting in a decrease in cell proliferation (Xu S. et al., 2020). LncRNA ARAP1-AS1 is upregulated in NSCLC. A knockout of lncRNA ARAP1-AS1 can inhibit the expression of cyclin D1, thereby arresting the cell cycle at G0/G1, which significantly inhibits cell proliferation (Tao X. et al., 2020). Additionally, according to the reports of Chen et al., there is a positive relevance between the expression of the lncRNA MINCR and the proliferation of lung cancer cells, which indicates that silencing of MINCR reduces cell proliferation in PC9 cells via reducing the expression levels of cell cycle protein A, cell cycle protein D, CD4 and CDK2 (Chen et al., 2019). The overexpression of lncRNA GAN1 can induce cell apoptosis and inhibit tumor growth by arresting cells in the G0/G1 phase. In addition, GAN1 can act as a miR26a-5p sponge and upregulate the PTEN level, thus inhibiting cell proliferation and inducing apoptosis in NSCLC (Wang et al., 2021).
Abnormal proliferation of tumors is connected with abnormal energy metabolism. Under aerobic conditions, cancer cells prefer glycolysis for energy metabolism, which is less efficient in ATP and energy production compared to oxidative phosphorylation. This process of energy metabolism is called the Warburg effect (Warburg, 1956). Glycolysis is essential for cancer cell proliferation (Liberti and Locasale, 2016). The lncRNA can target miRNAs to control the glycolysis of cancer cells. The study showed that LINC00857 activates SPAG5 expression in lung tumor cells by reducing miRNA-149 expression, resulting in glycolysis and cell proliferation (Wang L. et al., 2020). In another experiment, the effect of lncRNA LINC00243 in the regulation of glycolysis is studied. Glycolysis is stimulated by the overexpression of LINC00243, enhancing lung tumor progression. By downregulating miR-507, LINC00243 positively regulates PDK4 to promote glycolysis and proliferation in NSCLC cells (Feng and Yang, 2020). In addition, lncRNA HOXA11-AS promotes PKM3 expression by binding to miR-2b-148p, thereby promoting LUAD proliferation and glycolysis (Chen W. et al., 2023).
Currently, most of the studies of lncRNA on NSCLC proliferation and apoptosis are still in vitro experiments. However, the mechanisms by which lncRNAs affect proliferation and apoptosis are more complex due to the tumor microenvironment, so more in vivo experiments are needed to validate the results of in vitro experiments.
3.2 LncRNAs regulate angiogenesis in NSCLC
When the tumor grows to a certain size, nutrition is also provided to the tumor cells through tumor vascular production to ensure the further growth of the tumor. Tumor neovascularization features high-passage, irregular vascularization, intravascular infiltration, and immature vascularization (Matsunaga and Tomita, 2020). As a result of pathological hyperplasia blood vessel abnormalities, tumor neovascularization is frequently associated with lung cancer development and occurrence. Generally, tumor angiogenesis is an intricate mechanism regulated by several angiogenic factors and signaling pathways (Nakhjavani et al., 2021), such as vascular endothelial growth factor (VEGF) (Wang et al., 2018) and the angiopoietin (Ang)/Tie2 signaling pathways. VEGF is the primary regulator that promotes the proliferation of vascular endothelial cells and can directly promote the proliferation and metastasis of tumors (Goel and Mercurio, 2013; Frezzetti et al., 2017). It has been well documented that the expression of LINC00173.v1 is upregulated in SCC and negatively connected with patient survival. Overexpression of LINC00173.v1 stimulates the proliferation of vascular endothelial cells and promotes vascular neogenesis and metastasis of SCC. Mechanistically, LINC00173.v1 promotes VEGFA expression by sponging miR-511-5p, and VEGFA acts directly on vascular endothelial cells to promote angiogenesis (Chen J. et al., 2020). During vascular remodeling, increased expression of Ang2 activates Tie2 receptors, leading to signal transduction and thereby promoting endothelial cell proliferation (Bupathi et al., 2014). Overexpression of lncRNA EPIC1 increases the density of new blood vessels in a study. Furthermore, in NSCLC, EPIC1 stimulates vascular endothelial cell proliferation via the Ang2/Tie2 axis, resulting in angiogenesis and channel formation (Hou et al., 2021).
Vasculogenic mimicry (VM) was first proposed in aggressive human melanoma by Maniotis et al. In tumor tissue, it boosts the growth, invasion, and metastasis of tumors through the rapid generation of new blood vessels (Maniotis et al., 1999; Jiang X. et al., 2020). VM was questioned by some researchers when it was first proposed in 1999, but after intensive research, the role of VM in tumor angiogenesis has been demonstrated. For example, It was found that LINC00312 is overexpressed in lung adenocarcinoma and positively affects tumor invasion and metastasis. LINC00312 can directly bind to the transcription factor Y-Box binding protein 1 (YBX1) to increase the average density of VM in lung adenocarcinoma tissue, thereby causing tumor neovascularization (Peng et al., 2018). Moreover, there is a gene-binding site for estrogen receptor beta (ERβ) on lncRNA MALAT1, and ERβ positively regulates MALAT1 through complementary pairing with the estrogen response element (ERE) on the MALAT1 promoter. Overexpressed MALAT1 targets miR-145-5p, increased the expression of neural precursor cells expressed developmentally downregulated 9 (NEDD9), and promoted VM formation and cell invasion in NSCLC (Yu et al., 2019).
To summarize, when NSCLC grows to a certain stage, it metastasizes either locally or at a distant location, and tumor angiogenesis provides suitable conditions for invasion and metastasis. Therefore, lncRNAs not only regulate tumor angiogenesis but also affect the metastasis of NSCLC.
3.3 LncRNAs regulate the invasion and metastasis of NSCLC
Tumor angiogenesis provides suitable conditions for tumor metastasis and invasion. Tumor invasion and metastasis caused by epithelial mesenchymal transition (EMT) can increase patient mortality (Li S. et al., 2019). EMT, first proposed by Greenberg in 1982, is a key process in cancer cell metastasis (Ashrafizadeh et al., 2020). In this procedure, epithelial cell markers are absent, such as E-cadherin. Expression of mesenchymal cell markers, such as N-cadherin, vimentin and fibronectin, is upregulated. This causes reorganization of the cytoskeleton and enhances their migratory capacity and adhesion to neighboring cells (Dongre and Weinberg, 2019). It has been demonstrated that lncRNA can influence the process of EMT. For example, according to a previous study, the levels of lncRNA XIST and ZEB2 mRNA increase in NSCLC tissues. LncRNA XIST is involved in the progression of tumors as an oncogene and may affect TGF-β-induced EMT via raising the level of ZEB2, thereby speeding up the invasion and migration of NSCLC. In addition, lncRNA XIST acts as a miRNA sponge to inhibit miR-367 and miR-141 expression. Nevertheless, overexpression of miR-141 and miR-367 block TGF-β-induced EMT, and thus the invasion and metastasis efficiency of NSCLC are reduced (Li et al., 2018). Among NSCLC tissues, Linc00460 is overexpressed, and hindering Linc00460 expression affects the expression of EMT-related proteins, thereby inhibiting cancer cell invasion and metastasis (Yue and Zhang, 2018). LncRNA CRYBG3 can directly bind to eEF1A1, promote its entry into the nucleus, and thus strengthen the transcription of MDM2. Overexpressed MDM2 binds to MDM2-binding protein (MTBP), which reduces the binding between MTBP and ACTN4, thus increasing ACTN4-mediated cell migration (Wu et al., 2021).
Abnormally expressed lncRNA takes part in the invasion and metastasis of NSCLC by regulating the expression of signaling pathway genes, such as phosphoinositide 3-kinase (PI3K) (Jiang W. et al., 2020), mitogen-activated protein kinase (MAPK) (Zhang et al., 2018), Wnt/β-catenin signaling (Liu S. et al., 2020), TGF-β/SMAD signaling (Fan et al., 2023b), and Hippo (Zeng et al., 2021). For example, there is a strong correlation between the PI3K/AKT pathway and the proliferation, differentiation, and metastasis of cells (Yu and Cui, 2016). According to some research, Fer-1-like protein 4 (FER1L4) can decrease cell proliferation and metastasis in NSCLC by hindering PI3K/Akt signaling (Gao et al., 2019). The lncRNA NEAT1 is upregulated in NSCLC tissues and cells. NEAT1 overexpression triggers invasion and migration through aiming the has-miR-376b-3p/SULF1 axis. Moreover, by participating in the phosphorylation of MAPK and Akt, NEAT1 also regulates NSCLC progression, introducing a new avenue for cancer pathogenesis (Chen L. M. et al., 2020). As a result of the sponge action of lncRNA JPX, miRNA-33a-5p expression is decreased, bringing about an increase in Twist1 expression and aiding the EMT process by activating the Wnt/β-catenin pathway. This accelerates the malignant process of NSCLC (Pan et al., 2020). Mitochondrial RNA Processing Endoribonuclease (RMRP) can recruit YBX1 to the promoter region of TGFBR1, leading to activation of the TGFBR1/SMAD2/SMAD3 pathway, which increases NSCLC cell invasion and migration (Yin et al., 2023). The lncRNA non-small cell LCAT1 (NSCLCAT1) reportedly increases the invasion and migration of cells in NSCLC by interacting with CDH1 to regulate the Hippo signaling pathway (Zhao et al., 2019).
In conclusion, invasion and metastasis are multi-step malignant processes, of which lncRNA may be one of the regulatory factors. Investigating blockers targeting lncRNA may reduce metastasis in NSCLC, thereby improving clinical treatment and patient prognosis.
4 LncRNAs as diagnostic markers in NSCLC
NSCLC is most commonly diagnosed at a late stage, which results in a very low survival rate for patients. The early detection and intervention of NSCLC can limit tumor advancement and improve the overall survival rate of patients. Imaging examination can be used for the early screening of NSCLC, but due to its high false-positive rate, it cannot differentiate NSCLC from benign lung lesions. Conventional tumor markers have low specificity and cannot accurately diagnose NSCLC. For example, carcinoembryonic antigen (CEA) is elevated not only in NSCLC but also in digestive tract tumors (Gao et al., 2018; Jiao et al., 2021). As a result, there is an urgent need for more specific and reliable biomarkers for the diagnosis of NSCLC. The study found that lncRNA not only exists stably in peripheral blood but also suits quantitative detection, so it may be utilized as a new molecular marker for the diagnosis of NSCLC.
Area Under Curve (AUC) is defined as the area under the ROC curve, with a value between 0 and 1. AUC provides a visual evaluation of the authenticity of the test method, and a higher AUC value indicates a higher accuracy of the test (Mandrekar, 2010). Some benign lung diseases and NSCLC have similar symptoms, such as cough and hemoptysis. Imaging features on CT are not effective in distinguishing between them, which will affect the doctor’s judgment and treatment measures. Fortunately, some lncRNAs have high value in diagnosing lung cancer and distinguishing benign lung lesions. For example, patients with NSCLC have notably upper concentrations of circulating lncRNA XLOC_009167 in their whole blood samples. Compared with healthy controls, lncRNA XLOC_009167 has an AUC value of 0.7398 for the diagnosis of lung cancer, with a sensitivity of 78.7% and a specificity of 61.8%. This suggests that lung cancer can be diagnosed by XLOC_009167. Moreover, the AUC value of XLOC_009167 in distinguishing lung cancer and pneumonia is 0.7005, the sensitivity is 90.1%, and the specificity is 50.0%. The results show that XLOC_009167 may be useful in distinguishing lung cancer from pneumonia (Jiang et al., 2018). In NSCLC patients, lncRNA ADAMTS9-AS2 is remarkably lower than in benign lung lesions or normal controls (p < 0.001). According to the ROC curve analysis of ADAMTS9-AS2, the AUC value of plasma ADAMTS9-AS2 for diagnosing NSCLC is 0.957, and the sensitivity (95%) and specificity (99.1%) of plasma ADAMTS9-AS2 for diagnosing NSCLC are higher than those of CYFRA 21-1 (61.3% sensitivity and 60% specificity). Additionally, ADAMTS9-AS2 expression decreases with tumor stage progression. This suggests that ADAMTS9-AS2 may be a molecular marker for early NSCLC detection (Abdul-Maksoud et al., 2021). Therefore, the discovery of lncRNA in plasma will open a new door for the early diagnosis of NSCLC.
However, some lncRNAs, when used as single diagnostic markers, are not sensitive to diagnosing NSCLC. Combining lncRNAs with conventional tumor markers is a feasible way to improve the efficiency of NSCLC diagnosis. A study found that GAS5 expression is lower in the serum of NSCLC patients (p < 0.001). Through ROC curve analysis, GAS5 has a higher AUC value (0.857) than CEA (0.758) in distinguishing NSCLC patients from healthy controls. Further research found that by combining GAS5 with CEA, the AUC of the combined group is 0.929, indicating that GAS5 combined with CEA can improve the positive rate of diagnosis in NSCLC patients (Li et al., 2019). In another study, the expression levels of lncRNA TBILA (p < 0.001) and AGAP2-AS1 (p < 0.001) in the serum of NSCLC patients are notably more than those of healthy controls. In addition, postoperative serum TBILA and AGAP2-AS1 levels are significantly lower compared with preoperative treatment. Through the ROC curve analysis of TBILA and AGAP2-AS1, the results show that in the diagnosis of NSCLC, TBILA has an AUC value of 0.775, AGAP2-AS1 has an AUC value of 0.734, and the AUC value of the combined serum marker Cyfra21-1 is 0.853, indicating that this combination can increase the diagnostic ability of NSCLC (Tao et al., 2020). The lncRNAs SOX2OT and ANRIL are overexpressed in NSCLC. In addition, two long noncoding RNAs and three tumor markers (CEA, CYFRA21-1, and SCCA) are used to establish an NSCLC diagnostic panel; the AUC of this combination is 0.853, the sensitivity is 77.1%, and the specificity is 79.2%. The results show that the combination has a significantly greater power of diagnosis for NSCLC than lncRNA alone (Xie et al., 2018).
There is evidence that combinations of lncRNAs are more accurate than individual lncRNAs in diagnosing NSCLC. For example, the AUC value of the combined application of GAS5 and SOX2OT is 0.902, and the sensitivity and specificity reach 83.8% and 81.4%, which are more than those of GAS5 and SOX2OT alone (Kamel et al., 2019). NSCLC patients express more SNHG1 and RMRP in plasma than the control group (both p ≤ 0.05), and among the development cohort, compared with either gene alone, these two genes together have a diagnostic sensitivity of 84.13% for NSCLC, thereby improving the rate of diagnosis of NSCLC (Lin et al., 2018). Four lncRNAs (RMRP, NEAT1, TUG1, and MALAT1) are screened using qRT-PCR in 265 plasma samples (including 148 NSCLC and 117 controls) with differential expression levels between NSCLC and controls. A combination of four lncRNAs is established, and an ROC analysis is conducted to evaluate the diagnostic performance of the established four-lncRNA panel. The AUC of the combination for diagnosing NSCLC is 0.86, and this AUC value is significantly higher than the AUC value of an individual lncRNA. Further research found that the four-lncRNA panel also provides the ability to differentiate between certain benign diseases, including COPD, tuberculosis, and inflammation of the lung (Yuan et al., 2020).
As mentioned earlier, lncRNAs (Table 2) have high diagnostic efficiency and are promising markers for the diagnosis of NSCLC. However, these studies also have certain limitations. First, the number of samples used is not sufficient. In future experiments, it will be necessary to expand the sample size, conduct further multi-center cohort studies, and use an independent database for external validation. Second, the mechanism of the dysregulated expression of lncRNAs is still unclear, and exploring this mechanism will help to establish new diagnostic markers. Finally, lncRNA expression levels must be examined in other cancers to screen for the most specific lncRNAs in NSCLC diagnosis. The new model of combining lncRNA with chest CT and traditional tumor markers may be further used in the future for the diagnosis of NSCLC.
5 LncRNAs as prognosis markers in NSCLC
The prognosis of patients is closely related to TNM staging and the treatment of NSCLC. The overall survival rate of NSCLC patients is low because of drug resistance. At present, there is no accurate method to evaluate the prognosis of NSCLC. It has been found that lncRNAs can predict lymph node metastasis and TNM staging. Therefore, lncRNAs can be used as potential prognostic markers for NSCLC. Researchers revealed that lncRNA AC099850.3 is greatly upregulated in LUAD. Through Cox multivariate regression analysis, the results show that lncRNA AC099850.3 is an independent prognostic indicator that is associated with overall survival (OS), disease-free survival (DSS), and progress-free survival (PFS) among patients with LUAD (Chen et al., 2022b). LncRNA DPP10-AS1 expression is higher in 94 lung cancer tissues compared with normal tissues. DPP10-AS1 promotes the proliferation of lung cancer cells, which leads to a poor prognosis in patients. It is possible to use DPP 10-AS1 as an independent prognostic predictor and to determine a patient’s prognosis (Tian et al., 2021). Recently, lncRNA has been increasingly proven to have the potential for tumor prognosis. The level of lncRNA KTN1-AS1 is correlated with TNM stage (p = 0.0029), histological grade (p = 0.012) and lymph node metastasis (p = 0.020), and the high expression of KTN1-AS1 can reduce the OS of NSCLC patients (Liu et al., 2020). There is a shorter overall survival for NSCLC patients with high expression of the lncRNA PTTG3P. Moreover, the total survival of the high-expression group of PTTG3P in females and males with NSCLC is shorter than the low-expression group, and there is also a correlation between PTTG3P and DFS (Huang et al., 2020).
These results reveal that lncRNAs can serve as a prognostic predictor of tumors, and their expression level in tumors can be used to evaluate the clinicopathological features and overall survival of patients. Table 3 summarizes lncRNAs that affect the prognosis of NSCLC.
6 LncRNA and the treatment of NSCLC
Despite the rapid progression of chemotherapy and targeted therapies in the treatment of NSCLC, the appearance of drug resistance is inevitable. Emerging evidence suggests that dysregulation of lncRNAs plays a crucial role in the development of drug resistance in NSCLC cells. Therefore, targeting dysregulated lncRNAs may provide a novel therapeutic strategy for NSCLC.
6.1 LncRNAs regulate drug resistance of NSCLC
Cisplatin (DDP) is one of the basic chemotherapy drugs for the treatment of NSCLC due to its broad and strong anti-cancer effect (Zhou et al., 2018; Arbour and Riely, 2019). However, cisplatin-based chemotherapy still faces significant challenges owing to acquired drug resistance (Huang et al., 2019). Hence, it is vital to learn the mechanisms of cisplatin resistance to improve the efficacy of clinical treatment. Several studies have revealed that lncRNAs are key regulators of resistance to chemotherapy drugs. For example, lncRNA LINC01116 has a high expression in patients with lung adenocarcinoma, and the dysregulated expression of lncRNA LINC01116 leads to the resistance of lung adenocarcinoma to cisplatin through the EMT process. Conversely, LINC01116 knockdown may enhance sensitivity to cisplatin by regulating apoptosis and blocking the cell cycle (Wang et al., 2020). As lncRNA ZXF1 activates the MAPK signaling cascades of ERK, JNK and p38, it promotes cisplatin resistance and cancer progression, leading to treatment failure and tumor recurrence (Yu et al., 2020). According to another study, LINC01224 may compete with miR-2467, promoting tumor progression and increasing DDP resistance in NSCLC (Xiao et al., 2021).
In recent years, molecular targeted therapy has emerged as a significant approach to treat NSCIC. However, the emergence of resistance to targeted therapies in NSCLC is inevitable. lncRNAs can affect the formation of drug resistance mechanisms for targeted therapy. Increasing lncRNA UCA1 expression can make NSCLC cells more resistant to gefitinib, and the mechanism of occurrence is that lncRNA UCA1 upregulates the expression of FOSL2 by acting as a molecular sponge of miR-143, thus resulting in enhanced resistance to gefitinib in NSCLC cells (Chen et al., 2020). In addition, the lncRNA CCAT1 can reduce the expression level of miR-218, further upregulating HOXA1 expression and correspondingly promoting gefitinib resistance in NSCLC (Jin et al., 2020). Drug resistance in NSCLC is correlated with EZH2, which is the core subunit of polycomb repressive complex 2 (PRC2) (Zhan et al., 2019). By binding to EZH2, the lncRNA CASC9 inhibits the expression of DUSP1 in gefitinib-resistant PC9/GR cells, thus increasing the drug resistance to gefitinib (Chen et al., 2020). LncRNA LINC00969 inhibits the activation of the NLRP3/caspase-1/GSDMD-associated cellular pyroptosis signaling pathway, thereby promoting gefitinib resistance in NSCLC cells (Dai et al., 2023).
Overall, lncRNAs play a key role in drug resistance in NSCLC, but the mechanism of drug resistance is more complex. Knockdown or enhancement of lncRNA expression may promote drug sensitivity in cancer cells, thus lncRNAs are promising therapeutic targets.
6.2 LncRNAs as therapeutic target in NSCLC
A number of lncRNAs have increased or decreased expression in NSCLC tissues. Therefore, inhibition of oncogenic lncRNAs or promotion of anticancer lncRNAs can be one of the means of treatment for NSCLC. Overexpression of the lncRNA SNHG18 can stimulate NSCLC proliferation and invasion. In a mouse model, when SNHG18-knockdown NSCLC cells are transplanted into nude mice, tumor weight and metastatic potential are lower than in the control group (Fan et al., 2021). LncRNA HOXC-AS3 is connected to the occurrence of various cancers; there is evidence that HOXC-AS3 expression is up in NSCLC tissues and cells. Knockdown of HOXC-AS3 inhibits tumor progression and reduces the proliferation rate of cancer cells, and in vivo in the nude mice xenograft model, HOXC-AS3 knockdown consistently reduces tumor volume and weight compared to controls (Su et al., 2022). In addition, Wang et al. reported that overexpression of lncRNA ZNRD1-AS1 diminishes the proliferation of H1299 cells, and ZNRD1-AS1 overexpressing mice has lower tumor weights compared to the blank group, which is the same as the results of in vitro cellular studies (Wang et al., 2022a).
Tumor drug resistance is one of the difficulties in the therapeutic process. Knockdown or overexpression of lncRNAs can recover the sensitivity of cancer cells to drugs, thus improving drug efficacy. For example, linc00665 expression is upregulated in NSCLC tissues. When linc00665 is knocked down, the number of H1975 and H1299 cells is greatly reduced by the same concentration of DDP. In a mouse model, the knockdown of linc00665 also increases the sensitivity of H1299 cells to DDP (Yang et al., 2021). Furthermore, the lncRNA APCDD1L-AS1 expression in Icotinib-resistant cells is also significantly elevated. Knocking down the expression of APCDD1L-AS1 in Icotinib-resistant cells induces a marked reduction in the protein and phosphorylation levels of EGFR and significantly increases the reaction of lung adenocarcinoma cells to Icotinib (Xie et al., 2021). Thus, lncRNAs can be emerging therapeutic targets for NSCLC.
7 Conclusion
There is a high mortality rate associated with NSCLC, which is a highly malignant tumor. The survival rate of malignant tumor patients can be improved by early screening and diagnosis. However, there are no effective screening methods for NSCLC at an early stage. Researchers detected dysregulation of lncRNA expression in NSCLC tissues. This article reviews the role of lncRNA in regulating the progression of NSCLC and its influence on the diagnosis, treatment, and prognosis of NSCLC. First, lncRNAs regulate cells’ proliferation and apoptosis in three different ways: 1) lncRNAs can act as ceRNAs and then regulate the expression of related proteins; 2) lncRNAs cause cell division arrest in the G1 phase, thereby affecting cell proliferation and apoptosis; and 3) lncRNAs regulate the glycolytic pathway. As the tumor grows, lncRNA expression abnormally contributes to the proliferation of vascular endothelial cells by affecting the secretion of angiogenic factors by tumor cells and regulating the signaling of Ang/Tie2, which affects the growth and progress of NSCLC. In addition, tumor angiogenesis is also influenced by VM. Secondly, the metastasis and invasion of lung tumor cells are regulated by lncRNAs, which can induce EMT and enhance the metastasis and invasion of NSCLC. lncRNAs can also promote or inhibit EMT by regulating the transduction of various signal pathways. Finally, compared with the paracancerous tissue, there is a significant upregulation or downregulation of many lncRNAs found in tumor tissues, and their expression differences make lncRNAs potential diagnostic markers of NSCLC. Although some lncRNAs are effective at diagnosing NSCLC, clinical diagnosis generally relies on a joint approach. Combining lncRNAs with conventional tumor markers can improve diagnostic performance. LncRNAs can make lung tumor cells resistant to cisplatin, gefitinib, etc. Restoration of dysregulated lncRNA expression improves cancer cell responses to chemotherapy. Gene therapy targeting lncRNAs is a new strategy. Some lncRNAs have a correlation with lymph node metastasis and TNM stage, suggesting that lncRNAs can also be used as prognostic indicators. In summary, lncRNA is linked to the onset and development of NSCLC and may be taken as an indicator of diagnosis and prognosis.
8 Challenges and prospects
LncRNAs can exist stably in human body fluids and show specific expression profiles in various types of NSCLC, which provides a potential choice for the diagnosis of NSCLC. Some lncRNAs are expressed at opposite levels in tissue and serum, which may be related to the sample type (tissues and serum), the demographic feature of the study population (race, region, etc.), and the detection methods used. It was found that the same lncRNA is expressed to opposite degrees in different cancers, which indicates that the potential molecular mechanism of lncRNAs in cancer progression is more complicated. There are always differences between the results of some in vitro experiments and clinical phenomena because in vitro experiments cannot completely simulate anti-tumor immunity in the human body. Therefore, higher-quality studies are needed in the future to explore the mechanisms of lncRNAs in NSCLC development, create combinations of lncRNAs with higher specificity and conduct comprehensive validation in large-scale studies. In the future, some lncRNA-related clinical trials will be conducted to validate the results of in vitro experiments and animal models.
Author contributions
JF: Methodology, Writing–original draft, Writing–review and editing. LY: Methodology, Writing–review and editing. HY: Methodology, Writing–review and editing. SeT: Software, Writing–original draft, Writing–review and editing. ZW: Software, Writing–original draft. TD: Software, Writing–original draft. HC: Validation, Writing–original draft. SZ: Formal Analysis, Writing–original draft. HH: Data curation, Writing–original draft. TL: Data curation, Writing–original draft. SoT: Visualization, Writing–original draft. RH: Conceptualization, Resources, Supervision, Writing–review and editing. HZ: Conceptualization, Resources, Supervision, Writing–review and editing.
Funding
The authors declare financial support was received for the research, authorship, and/or publication of this article. Medical Science and Technology Project of Sichuan Provincial Health Commission: 21PJ118 and Research Project of Suining Central Hospital: 2022yz102.
Conflict of interest
The authors declare that the research was conducted in the absence of any commercial or financial relationships that could be construed as a potential conflict of interest.
Publisher’s note
All claims expressed in this article are solely those of the authors and do not necessarily represent those of their affiliated organizations, or those of the publisher, the editors and the reviewers. Any product that may be evaluated in this article, or claim that may be made by its manufacturer, is not guaranteed or endorsed by the publisher.
References
Abdul-Maksoud, R. S., Rashad, N. M., Elsayed, W. S. H., Elsayed, R. S., Sherif, M. M., Abbas, A., et al. (2021). The diagnostic significance of circulating lncRNA ADAMTS9-AS2 tumor biomarker in non-small cell lung cancer among the Egyptian population. J. Gene Med. 23 (12), e3381. doi:10.1002/jgm.3381
Aberle, D. R., Adams, A. M., Berg, C. D., Black, W. C., Clapp, J. D., Fagerstrom, R. M., et al. (2011). Reduced lung-cancer mortality with low-dose computed tomographic screening. N. Engl. J. Med. 365 (5), 395–409. doi:10.1056/NEJMoa1102873
Arbour, K. C., and Riely, G. J. (2019). Systemic therapy for locally advanced and metastatic non-small cell lung cancer: a review. JAMA 322 (8), 764–774. doi:10.1001/jama.2019.11058
Ashrafizadeh, M., Ang, H. L., Moghadam, E. R., Mohammadi, S., Zarrin, V., Hushmandi, K., et al. (2020). MicroRNAs and their influence on the ZEB family: mechanistic aspects and therapeutic applications in cancer therapy. Biomolecules 10 (7), 1040. doi:10.3390/biom10071040
Balas, M. M., Hartwick, E. W., Barrington, C., Roberts, J. T., Wu, S. K., Bettcher, R., et al. (2021). Establishing RNA-RNA interactions remodels lncRNA structure and promotes PRC2 activity. Sci. Adv. 7 (16), eabc9191. doi:10.1126/sciadv.abc9191
Beermann, J., Piccoli, M. T., Viereck, J., and Thum, T. (2016). Non-coding RNAs in development and disease: background, mechanisms, and therapeutic approaches. Physiol. Rev. 96 (4), 1297–1325. doi:10.1152/physrev.00041.2015
Borsani, G., Tonlorenzi, R., Simmler, M. C., Dandolo, L., Arnaud, D., Capra, V., et al. (1991). Characterization of a murine gene expressed from the inactive X chromosome. Nature 351 (6324), 325–329. doi:10.1038/351325a0
Bupathi, M., Kaseb, A., and Janku, F. (2014). Angiopoietin 2 as a therapeutic target in hepatocellular carcinoma treatment: current perspectives. Onco Targets Ther. 7, 1927–1932. doi:10.2147/OTT.S46457
Cao, Y., Di, X., Cong, S., Tian, C., Wang, Y., Jin, X., et al. (2023). RBM10 recruits METTL3 to induce N6-methyladenosine-MALAT1-dependent modification, inhibiting the invasion and migration of NSCLC. Life Sci. 315, 121359. doi:10.1016/j.lfs.2022.121359
Chen, J., Liu, A., Wang, Z., Wang, B., Chai, X., Lu, W., et al. (2020a). LINC00173.v1 promotes angiogenesis and progression of lung squamous cell carcinoma by sponging miR-511-5p to regulate VEGFA expression. Mol. Cancer 19 (1), 98. doi:10.1186/s12943-020-01217-2
Chen, L. M., Niu, Y. D., Xiao, M., Li, X. J., and Lin, H. (2020b). LncRNA NEAT1 regulated cell proliferation, invasion, migration and apoptosis by targeting has-miR-376b-3p/SULF1 axis in non-small cell lung cancer. Eur. Rev. Med. Pharmacol. Sci. 24 (9), 4810–4821. doi:10.26355/eurrev_202005_21170
Chen, Q., Zhu, C., Jin, Y., Si, X., Jiao, W., He, W., et al. (2020c). Plasma long non-coding RNA RP11-438N5.3 as a novel biomarker for non-small cell lung cancer. Cancer Manag. Res. 12, 1513–1521. doi:10.2147/CMAR.S237024
Chen, Q. W., Cai, Q. Q., Yang, Y., Dong, S., Liu, Y. Y., Chen, Z. Y., et al. (2023a). LncRNA BC promotes lung adenocarcinoma progression by modulating IMPAD1 alternative splicing. Clin. Transl. Med. 13 (1), e1129. doi:10.1002/ctm2.1129
Chen, S., Gu, T., Lu, Z., Qiu, L., Xiao, G., Zhu, X., et al. (2019). Roles of MYC-targeting long non-coding RNA MINCR in cell cycle regulation and apoptosis in non-small cell lung Cancer. Respir. Res. 20 (1), 202. doi:10.1186/s12931-019-1174-z
Chen, W., Li, X., Du, B., Cui, Y., Ma, Y., and Li, Y. (2023b). The long noncoding RNA HOXA11-AS promotes lung adenocarcinoma proliferation and glycolysis via the microRNA-148b-3p/PKM2 axis. Cancer Med. 12 (4), 4421–4433. doi:10.1002/cam4.5103
Chen, X., Guo, J., Zhou, F., Ren, W., Huang, X., Pu, J., et al. (2022a). Long non-coding RNA AL139385.1 as a novel prognostic biomarker in lung adenocarcinoma. Front. Oncol. 12, 905871. doi:10.3389/fonc.2022.905871
Chen, X., Guo, J., Zhou, F., Ren, W., Pu, J., Mutti, L., et al. (2022b). Over-expression of long non-coding RNA-ac099850.3 correlates with tumor progression and poor prognosis in lung adenocarcinoma. Front. Oncol. 12, 895708. doi:10.3389/fonc.2022.895708
Chen, X., Wang, Z., Tong, F., Dong, X., Wu, G., and Zhang, R. (2020d). lncRNA UCA1 promotes gefitinib resistance as a ceRNA to target FOSL2 by sponging miR-143 in non-small cell lung cancer. Mol. Ther. Nucleic Acids 19, 643–653. doi:10.1016/j.omtn.2019.10.047
Chen, Y. L., Liu, Y. N., Lin, Y. T., Tsai, M. F., Wu, S. G., Chang, T. H., et al. (2023c). LncRNA SLCO4A1-AS1 suppresses lung cancer progression by sequestering the TOX4-NTSR1 signaling axis. J. Biomed. Sci. 30 (1), 80. doi:10.1186/s12929-023-00973-9
Chen, Z., Chen, Q., Cheng, Z., Gu, J., Feng, W., Lei, T., et al. (2020e). Long non-coding RNA CASC9 promotes gefitinib resistance in NSCLC by epigenetic repression of DUSP1. Cell Death Dis. 11 (10), 858. doi:10.1038/s41419-020-03047-y
Cruz, J. A., and Westhof, E. (2009). The dynamic landscapes of RNA architecture. Cell 136 (4), 604–609. doi:10.1016/j.cell.2009.02.003
Dai, J., Qu, T., Yin, D., Cui, Y., Zhang, C., Zhang, E., et al. (2023). LncRNA LINC00969 promotes acquired gefitinib resistance by epigenetically suppressing of NLRP3 at transcriptional and posttranscriptional levels to inhibit pyroptosis in lung cancer. Cell Death Dis. 14 (5), 312. doi:10.1038/s41419-023-05840-x
Derrien, T., Johnson, R., Bussotti, G., Tanzer, A., Djebali, S., Tilgner, H., et al. (2012). The GENCODE v7 catalog of human long noncoding RNAs: analysis of their gene structure, evolution, and expression. Genome Res. 22 (9), 1775–1789. doi:10.1101/gr.132159.111
Dongre, A., and Weinberg, R. A. (2019). New insights into the mechanisms of epithelial-mesenchymal transition and implications for cancer. Nat. Rev. Mol. Cell Biol. 20 (2), 69–84. doi:10.1038/s41580-018-0080-4
Du, M., Hu, X., Jiang, X., Yin, L., Chen, J., Wen, J., et al. (2021). LncRNA epb41l4a-AS2 represses nasopharyngeal carcinoma metastasis by binding to YBX1 in the nucleus and sponging MiR-107 in the cytoplasm. Int. J. Biol. Sci. 17 (8), 1963–1978. doi:10.7150/ijbs.55557
Entezari, M., Ghanbarirad, M., Taheriazam, A., Sadrkhanloo, M., Zabolian, A., Goharrizi, M., et al. (2022). Long non-coding RNAs and exosomal lncRNAs: potential functions in lung cancer progression, drug resistance and tumor microenvironment remodeling. Biomed. Pharmacother. 150, 112963. doi:10.1016/j.biopha.2022.112963
Esposito, R., Bosch, N., Lanzos, A., Polidori, T., Pulido-Quetglas, C., and Johnson, R. (2019). Hacking the cancer genome: profiling therapeutically actionable long non-coding RNAs using CRISPR-cas9 screening. Cancer Cell 35 (4), 545–557. doi:10.1016/j.ccell.2019.01.019
Fan, C., González-Prieto, R., Kuipers, T. B., Vertegaal, A. C. O., van Veelen, P. A., Mei, H., et al. (2023a). The lncRNA LETS1 promotes TGF-β-induced EMT and cancer cell migration by transcriptionally activating a TβR1-stabilizing mechanism. Sci. Signal 16 (790), eadf1947. doi:10.1126/scisignal.adf1947
Fan, C., Wang, Q., Kuipers, T. B., Cats, D., Iyengar, P. V., Hagenaars, S. C., et al. (2023b). LncRNA LITATS1 suppresses TGF-β-induced EMT and cancer cell plasticity by potentiating TβRI degradation. Embo J. 42 (10), e112806. doi:10.15252/embj.2022112806
Fan, H., Yuan, J., Li, Y., Jia, Y., Li, J., Wang, X., et al. (2021). MKL1-induced lncRNA SNHG18 drives the growth and metastasis of non-small cell lung cancer via the miR-211-5p/BRD4 axis. Cell Death Dis. 12 (1), 128. doi:10.1038/s41419-021-03399-z
Feng, X., and Yang, S. (2020). Long non-coding RNA LINC00243 promotes proliferation and glycolysis in non-small cell lung cancer cells by positively regulating PDK4 through sponging miR-507. Mol. Cell Biochem. 463 (1-2), 127–136. doi:10.1007/s11010-019-03635-3
Frezzetti, D., Gallo, M., Maiello, M. R., D'Alessio, A., Esposito, C., Chicchinelli, N., et al. (2017). VEGF as a potential target in lung cancer. Expert Opin. Ther. Targets 21 (10), 959–966. doi:10.1080/14728222.2017.1371137
Gao, J., Pan, T., Wang, H., Wang, S., Chai, J., and Jin, C. (2023). LncRNA FAM138B inhibits the progression of non-small cell lung cancer through miR-105-5p. Cell Cycle 22 (7), 808–817. doi:10.1080/15384101.2022.2154556
Gao, X., Wang, N., Wu, S., Cui, H., An, X., and Yang, Y. (2019). Long noncoding RNA FER1L4 inhibits cell proliferation and metastasis through regulation of the PI3K/AKT signaling pathway in lung cancer cells. Mol. Med. Rep. 20 (1), 182–190. doi:10.3892/mmr.2019.10219
Gao, Y., Wang, J., Zhou, Y., Sheng, S., Qian, S. Y., and Huo, X. (2018). Evaluation of serum CEA, CA19-9, CA72-4, CA125 and ferritin as diagnostic markers and factors of clinical parameters for colorectal cancer. Sci. Rep. 8 (1), 2732. doi:10.1038/s41598-018-21048-y
Goel, H. L., and Mercurio, A. M. (2013). VEGF targets the tumour cell. Nat. Rev. Cancer 13 (12), 871–882. doi:10.1038/nrc3627
Guo, R., Hu, T., Liu, Y., He, Y., and Cao, Y. (2020). Long non-coding RNA PRNCR1 modulates non-small cell lung cancer cell proliferation, apoptosis, migration, invasion, and EMT through PRNCR1/miR-126-5p/MTDH axis. Biosci. Rep. 40 (7). doi:10.1042/BSR20193153
Gupta, C., Su, J., Zhan, M., Stass, S. A., and Jiang, F. (2019). Sputum long non-coding RNA biomarkers for diagnosis of lung cancer. Cancer Biomark. 26 (2), 219–227. doi:10.3233/CBM-190161
Han, W., Du, X., Liu, M., Wang, J., Sun, L., and Li, Y. (2019). Increased expression of long non-coding RNA SNHG16 correlates with tumor progression and poor prognosis in non-small cell lung cancer. Int. J. Biol. Macromol. 121, 270–278. doi:10.1016/j.ijbiomac.2018.10.004
Hanahan, D. (2022). Hallmarks of cancer: new dimensions. Cancer Discov. 12 (1), 31–46. doi:10.1158/2159-8290.CD-21-1059
He, C., Huang, D., Yang, F., Huang, D., Cao, Y., Peng, J., et al. (2022). High expression of lncRNA HEIH is helpful in the diagnosis of non-small cell lung cancer and predicts poor prognosis. Cancer Manag. Res. 14, 503–514. doi:10.2147/CMAR.S320965
Herman, A. B., Tsitsipatis, D., and Gorospe, M. (2022). Integrated lncRNA function upon genomic and epigenomic regulation. Mol. Cell 82 (12), 2252–2266. doi:10.1016/j.molcel.2022.05.027
Hou, Y., Jia, H., Cao, Y., Zhang, S., Zhang, X., Wei, P., et al. (2021). LncRNA EPIC1 promotes tumor angiogenesis via activating the Ang2/Tie2 axis in non-small cell lung cancer. Life Sci. 267, 118933. doi:10.1016/j.lfs.2020.118933
Hsu, X. R., Wu, J. E., Wu, Y. Y., Hsiao, S. Y., Liang, J. L., Wu, Y. J., et al. (2023). Exosomal long noncoding RNA MLETA1 promotes tumor progression and metastasis by regulating the miR-186-5p/EGFR and miR-497-5p/IGF1R axes in non-small cell lung cancer. J. Exp. Clin. Cancer Res. 42 (1), 283. doi:10.1186/s13046-023-02859-y
Hu, Z., Liu, Y., Tang, J., Luo, R., Qin, J., Mo, Z., et al. (2023). LncRNA HHIP-AS1 suppresses lung squamous cell carcinoma by stabilizing HHIP mRNA. Life Sci. 321, 121578. doi:10.1016/j.lfs.2023.121578
Huang, G., Lou, T., Pan, J., Ye, Z., Yin, Z., Li, L., et al. (2019). MiR-204 reduces cisplatin resistance in non-small cell lung cancer through suppression of the caveolin-1/AKT/Bad pathway. Aging (Albany NY) 11 (7), 2138–2150. doi:10.18632/aging.101907
Huang, H., Du, J., Jin, B., Pang, L., Duan, N., Huang, C., et al. (2021). Combination of urine exosomal mRNAs and lncRNAs as novel diagnostic biomarkers for bladder cancer. Front. Oncol. 11, 667212. doi:10.3389/fonc.2021.667212
Huang, H. T., Xu, Y. M., Ding, S. G., Yu, X. Q., Wang, F., Wang, H. F., et al. (2020a). The novel lncRNA PTTG3P is downregulated and predicts poor prognosis in non-small cell lung cancer. Arch. Med. Sci. 16 (4), 931–940. doi:10.5114/aoms.2020.93535
Huang, W., Yan, Y., Liu, Y., Lin, M., Ma, J., Zhang, W., et al. (2020b). Exosomes with low miR-34c-3p expression promote invasion and migration of non-small cell lung cancer by upregulating integrin α2β1. Signal Transduct. Target Ther. 5 (1), 39. doi:10.1038/s41392-020-0133-y
Hudson, W. H., Pickard, M. R., de Vera, I. M., Kuiper, E. G., Mourtada-Maarabouni, M., Conn, G. L., et al. (2014). Conserved sequence-specific lincRNA-steroid receptor interactions drive transcriptional repression and direct cell fate. Nat. Commun. 5, 5395. doi:10.1038/ncomms6395
Iwai, M., Kajino, T., Nakatochi, M., Yanagisawa, K., Hosono, Y., Isomura, H., et al. (2023). Long non-coding RNA TILR constitutively represses TP53 and apoptosis in lung cancer. Oncogene 42 (5), 364–373. doi:10.1038/s41388-022-02546-w
Jia, M., Feng, S., Cao, F., Deng, J., Li, W., Zhou, W., et al. (2022). Identification of EGFR-related linc00460/mir-338-3p/MCM4 regulatory Axis as diagnostic and prognostic biomarker of lung adenocarcinoma based on comprehensive bioinformatics analysis and experimental validation. Cancers (Basel) 14 (20), 5073. doi:10.3390/cancers14205073
Jiang, N., Meng, X., Mi, H., Chi, Y., Li, S., Jin, Z., et al. (2018). Circulating lncRNA XLOC_009167 serves as a diagnostic biomarker to predict lung cancer. Clin. Chim. Acta 486, 26–33. doi:10.1016/j.cca.2018.07.026
Jiang, W., Kai, J., Li, D., Wei, Z., Wang, Y., and Wang, W. (2020a). lncRNA HOXB-AS3 exacerbates proliferation, migration, and invasion of lung cancer via activating the PI3K-AKT pathway. J. Cell Physiol. 235 (10), 7194–7203. doi:10.1002/jcp.29618
Jiang, X., Wang, J., Deng, X., Xiong, F., Zhang, S., Gong, Z., et al. (2020b). The role of microenvironment in tumor angiogenesis. J. Exp. Clin. Cancer Res. 39 (1), 204. doi:10.1186/s13046-020-01709-5
Jiao, Z., Cao, S., Li, J., Hu, N., Gong, Y., Wang, L., et al. (2021). Clinical associations of preoperative and postoperative serum CEA and lung cancer outcome. Front. Mol. Biosci. 8, 686313. doi:10.3389/fmolb.2021.686313
Jin, X., Liu, X., Zhang, Z., and Guan, Y. (2020). lncRNA CCAT1 acts as a MicroRNA-218 sponge to increase gefitinib resistance in NSCLC by targeting HOXA1. Mol. Ther. Nucleic Acids 19, 1266–1275. doi:10.1016/j.omtn.2020.01.006
Jonas, K., Calin, G. A., and Pichler, M. (2020). RNA-binding proteins as important regulators of long non-coding RNAs in cancer. Int. J. Mol. Sci. 21 (8), 2969. doi:10.3390/ijms21082969
Kamel, L. M., Atef, D. M., Mackawy, A. M. H., Shalaby, S. M., and Abdelraheim, N. (2019). Circulating long non-coding RNA GAS5 and SOX2OT as potential biomarkers for diagnosis and prognosis of non-small cell lung cancer. Biotechnol. Appl. Biochem. 66 (4), 634–642. doi:10.1002/bab.1764
Karreth, F. A., and Pandolfi, P. P. (2013). ceRNA cross-talk in cancer: when ce-bling rivalries go awry. Cancer Discov. 3 (10), 1113–1121. doi:10.1158/2159-8290.CD-13-0202
Kim, J., Piao, H. L., Kim, B. J., Yao, F., Han, Z., Wang, Y., et al. (2018). Long noncoding RNA MALAT1 suppresses breast cancer metastasis. Nat. Genet. 50 (12), 1705–1715. doi:10.1038/s41588-018-0252-3
Kong, X., Duan, Y., Sang, Y., Li, Y., Zhang, H., Liang, Y., et al. (2019). LncRNA-CDC6 promotes breast cancer progression and function as ceRNA to target CDC6 by sponging microRNA-215. J. Cell Physiol. 234 (6), 9105–9117. doi:10.1002/jcp.27587
Lagarde, J., Uszczynska-Ratajczak, B., Carbonell, S., Perez-Lluch, S., Abad, A., Davis, C., et al. (2017). High-throughput annotation of full-length long noncoding RNAs with capture long-read sequencing. Nat. Genet. 49 (12), 1731–1740. doi:10.1038/ng.3988
Le Beguec, C., Wucher, V., Lagoutte, L., Cadieu, E., Botherel, N., Hedan, B., et al. (2018). Characterisation and functional predictions of canine long non-coding RNAs. Sci. Rep. 8 (1), 13444. doi:10.1038/s41598-018-31770-2
Li, C., Lv, Y., Shao, C., Chen, C., Zhang, T., Wei, Y., et al. (2019a). Tumor-derived exosomal lncRNA GAS5 as a biomarker for early-stage non-small-cell lung cancer diagnosis. J. Cell Physiol. 234 (11), 20721–20727. doi:10.1002/jcp.28678
Li, C., Wan, L., Liu, Z., Xu, G., Wang, S., Su, Z., et al. (2018). Long non-coding RNA XIST promotes TGF-beta-induced epithelial-mesenchymal transition by regulating miR-367/141-ZEB2 axis in non-small-cell lung cancer. Cancer Lett. 418, 185–195. doi:10.1016/j.canlet.2018.01.036
Li, H., Chen, Y. K., Wan, Q., Shi, A. Q., Wang, M., He, P., et al. (2021a). Long non-coding RNA LINC00847 induced by E2F1 accelerates non-small cell lung cancer progression through targeting miR-147a/IFITM1 Axis. Front. Med. (Lausanne) 8, 663558. doi:10.3389/fmed.2021.663558
Li, H., Cui, Z., Lv, X., Li, J., Gao, M., Yang, Z., et al. (2020). Long non-coding RNA HOTAIR function as a competing endogenous RNA for miR-149-5p to promote the cell growth, migration, and invasion in non-small cell lung cancer. Front. Oncol. 10, 528520. doi:10.3389/fonc.2020.528520
Li, L., Wei, H., Zhang, Y. W., Zhao, S., Che, G., Wang, Y., et al. (2021b). Differential expression of long non-coding RNAs as diagnostic markers for lung cancer and other malignant tumors. Aging (Albany NY) 13 (20), 23842–23867. doi:10.18632/aging.203523
Li, M., Chen, Y., Zhu, J., Gao, Z., Wang, T., and Zhou, P. (2019b). Long noncoding RNA CASC15 predicts unfavorable prognosis and exerts oncogenic functions in non-small cell lung cancer. Am. J. Transl. Res. 11 (7), 4303–4314.
Li, S., Pradhan, L., Ashur, S., Joshi, A., and Nam, H. J. (2019c). Crystal structure of FOXC2 in complex with DNA target. ACS Omega 4 (6), 10906–10914. doi:10.1021/acsomega.9b00756
Liao, S., Yu, C., Liu, H., Zhang, C., Li, Y., and Zhong, X. (2019). Long non-coding RNA H19 promotes the proliferation and invasion of lung cancer cells and regulates the expression of E-cadherin, N-cadherin, and vimentin. Onco Targets Ther. 12, 4099–4107. doi:10.2147/OTT.S185156
Liberti, M. V., and Locasale, J. W. (2016). The Warburg effect: how does it benefit cancer cells? Trends Biochem. Sci. 41 (3), 211–218. doi:10.1016/j.tibs.2015.12.001
Lin, C., and Yang, L. (2018). Long noncoding RNA in cancer: wiring signaling circuitry. Trends Cell Biol. 28 (4), 287–301. doi:10.1016/j.tcb.2017.11.008
Lin, X., Zhuang, S., Chen, X., Du, J., Zhong, L., Ding, J., et al. (2022). lncRNA ITGB8-AS1 functions as a ceRNA to promote colorectal cancer growth and migration through integrin-mediated focal adhesion signaling. Mol. Ther. 30 (2), 688–702. doi:10.1016/j.ymthe.2021.08.011
Lin, Y., Leng, Q., Zhan, M., and Jiang, F. (2018). A plasma long noncoding RNA signature for early detection of lung cancer. Transl. Oncol. 11 (5), 1225–1231. doi:10.1016/j.tranon.2018.07.016
Lingling, J., Xiangao, J., Guiqing, H., Jichan, S., Feifei, S., and Haiyan, Z. (2019). SNHG20 knockdown suppresses proliferation, migration and invasion, and promotes apoptosis in non-small cell lung cancer through acting as a miR-154 sponge. Biomed. Pharmacother. 112, 108648. doi:10.1016/j.biopha.2019.108648
Liu, C., Li, X., Hao, Y., Wang, F., Cheng, Z., Geng, H., et al. (2020a). STAT1-induced upregulation of lncRNA KTN1-AS1 predicts poor prognosis and facilitates non-small cell lung cancer progression via miR-23b/DEPDC1 axis. Aging (Albany NY) 12 (9), 8680–8701. doi:10.18632/aging.103191
Liu, G., Guo, X., Zhang, Y., Liu, Y., Li, D., Tang, G., et al. (2019). Expression and significance of LncRNA MNX1-AS1 in non-small cell lung cancer. Onco Targets Ther. 12, 3129–3138. doi:10.2147/OTT.S198014
Liu, H. Y., Lu, S. R., Guo, Z. H., Zhang, Z. S., Ye, X., Du, Q., et al. (2020b). lncRNA SLC16A1-AS1 as a novel prognostic biomarker in non-small cell lung cancer. J. Investig. Med. 68 (1), 52–59. doi:10.1136/jim-2019-001080
Liu, S., Yang, N., Wang, L., Wei, B., Chen, J., and Gao, Y. (2020c). lncRNA SNHG11 promotes lung cancer cell proliferation and migration via activation of Wnt/β-catenin signaling pathway. J. Cell Physiol. 235 (10), 7541–7553. doi:10.1002/jcp.29656
Liu, Y., Liang, L., Ji, L., Zhang, F., Chen, D., Duan, S., et al. (2021). Potentiated lung adenocarcinoma (LUAD) cell growth, migration and invasion by lncRNA DARS-AS1 via miR-188-5p/KLF12 axis. Aging (Albany NY) 13 (19), 23376–23392. doi:10.18632/aging.203632
Liu, Y., Liu, T., Jin, H., Yin, L., Yu, H., and Bi, J. (2018). MiR-411 suppresses the development of bladder cancer by regulating ZnT1. Onco Targets Ther. 11, 8695–8704. doi:10.2147/OTT.S173750
Liu, Z., Ning, Z., Lu, H., Cao, T., Zhou, F., Ye, X., et al. (2020d). Long non-coding RNA RFPL3S is a novel prognostic biomarker in lung cancer. Oncol. Lett. 20 (2), 1270–1280. doi:10.3892/ol.2020.11642
Luo, C. L., Xu, Z. G., Chen, H., Ji, J., Wang, Y. H., Hu, W., et al. (2018). LncRNAs and EGFRvIII sequestered in TEPs enable blood-based NSCLC diagnosis. Cancer Manag. Res. 10, 1449–1459. doi:10.2147/CMAR.S164227
Ma, H. P., Wang, L. X., Li, W., Guo, H. H., Wu, Y., and Li, X. Y. (2020). Upregulation of LINC00504 is associated with aggressive progression and poor prognosis in non-small cell lung cancer. Eur. Rev. Med. Pharmacol. Sci. 24 (2), 699–703. doi:10.26355/eurrev_202001_20047
Mandrekar, J. N. (2010). Receiver operating characteristic curve in diagnostic test assessment. J. Thorac. Oncol. 5 (9), 1315–1316. doi:10.1097/JTO.0b013e3181ec173d
Maniotis, A. J., Folberg, R., Hess, A., Seftor, E. A., Gardner, L. M., Pe'er, J., et al. (1999). Vascular channel formation by human melanoma cells in vivo and in vitro: vasculogenic mimicry. Am. J. Pathol. 155 (3), 739–752. doi:10.1016/S0002-9440(10)65173-5
Markaki, Y., Gan Chong, J., Wang, Y., Jacobson, E. C., Luong, C., Tan, S. Y. X., et al. (2021). Xist nucleates local protein gradients to propagate silencing across the X chromosome. Cell 184 (25), 6174–6192.e32. doi:10.1016/j.cell.2021.10.022
Matsunaga, S., and Tomita, S. (2020). The effect of PHD inhibitor on tumor microenvironment and tumor immune response. Nihon Yakurigaku Zasshi 155 (1), 35–39. doi:10.1254/fpj.19119
McCunney, R. J., and Li, J. (2014). Radiation risks in lung cancer screening programs: a comparison with nuclear industry workers and atomic bomb survivors. Chest 145 (3), 618–624. doi:10.1378/chest.13-1420
Merry, C. R., Forrest, M. E., Sabers, J. N., Beard, L., Gao, X. H., Hatzoglou, M., et al. (2015). DNMT1-associated long non-coding RNAs regulate global gene expression and DNA methylation in colon cancer. Hum. Mol. Genet. 24 (21), 6240–6253. doi:10.1093/hmg/ddv343
Min, L., Zhu, T., Lv, B., An, T., Zhang, Q., Shang, Y., et al. (2022). Exosomal LncRNA RP5-977B1 as a novel minimally invasive biomarker for diagnosis and prognosis in non-small cell lung cancer. Int. J. Clin. Oncol. 27 (6), 1013–1024. doi:10.1007/s10147-022-02129-5
Nakhjavani, M., Smith, E., Yeo, K., Palethorpe, H. M., Tomita, Y., Price, T. J., et al. (2021). Anti-angiogenic properties of ginsenoside Rg3 epimers: in vitro assessment of single and combination treatments. Cancers (Basel) 13 (9), 2223. doi:10.3390/cancers13092223
Pan, J., Fang, S., Tian, H., Zhou, C., Zhao, X., Tian, H., et al. (2020). lncRNA JPX/miR-33a-5p/Twist1 axis regulates tumorigenesis and metastasis of lung cancer by activating Wnt/β-catenin signaling. Mol. Cancer 19 (1), 9. doi:10.1186/s12943-020-1133-9
Pei, H., Dai, Y., Yu, Y., Tang, J., Cao, Z., Zhang, Y., et al. (2023). The tumorigenic effect of lncRNA AFAP1-AS1 is mediated by translated peptide atmlp under the control of m(6) A methylation. Adv. Sci. (Weinh) 10 (13), e2300314. doi:10.1002/advs.202300314
Peng, Z., Wang, J., Shan, B., Li, B., Peng, W., Dong, Y., et al. (2018). The long noncoding RNA LINC00312 induces lung adenocarcinoma migration and vasculogenic mimicry through directly binding YBX1. Mol. Cancer 17 (1), 167. doi:10.1186/s12943-018-0920-z
Pidíková, P., and Herichová, I. (2021). miRNA clusters with up-regulated expression in colorectal cancer. Cancers (Basel) 13 (12), 2979. doi:10.3390/cancers13122979
Ponting, C. P., Oliver, P. L., and Reik, W. (2009). Evolution and functions of long noncoding RNAs. Cell 136 (4), 629–641. doi:10.1016/j.cell.2009.02.006
Qian, X., Zhao, J., Yeung, P. Y., Zhang, Q. C., and Kwok, C. K. (2019). Revealing lncRNA structures and interactions by sequencing-based approaches. Trends Biochem. Sci. 44 (1), 33–52. doi:10.1016/j.tibs.2018.09.012
Quinn, J. J., and Chang, H. Y. (2016). Unique features of long non-coding RNA biogenesis and function. Nat. Rev. Genet. 17 (1), 47–62. doi:10.1038/nrg.2015.10
Shi, L., Li, B., Zhang, Y., Chen, Y., Tan, J., Chen, Y., et al. (2023). Exosomal lncRNA Mir100hg derived from cancer stem cells enhance glycolysis and promote metastasis of lung adenocarcinoma through mircroRNA-15a-5p/31-5p. Cell Commun. Signal 21 (1), 248. doi:10.1186/s12964-023-01281-3
Shieh, T. M., Liu, C. J., Hsia, S. M., Ningrum, V., Liao, C. C., Lan, W. C., et al. (2021). Lack of salivary long non-coding RNA XIST expression is associated with increased risk of oral squamous cell carcinoma: a cross-sectional study. J. Clin. Med. 10 (19), 4622. doi:10.3390/jcm10194622
Statello, L., Guo, C. J., Chen, L. L., and Huarte, M. (2021). Gene regulation by long non-coding RNAs and its biological functions. Nat. Rev. Mol. Cell Biol. 22 (2), 96–118. doi:10.1038/s41580-020-00315-9
Su, H., Fan, G., Huang, J., and Qiu, X. (2022). LncRNA HOXC-AS3 promotes non-small-cell lung cancer growth and metastasis through upregulation of YBX1. Cell Death Dis. 13 (4), 307. doi:10.1038/s41419-022-04723-x
Sun, K., Lu, T., Hu, C., Li, Z., Zhu, J., Zhang, L., et al. (2023). LINC00115 regulates lung adenocarcinoma progression via sponging miR-154-3p to modulate Sp3 expression. Mol. Cell Probes 68, 101909. doi:10.1016/j.mcp.2023.101909
Sun, X., Xin, Y., Wang, M., Li, S., Miao, S., Xuan, Y., et al. (2018). Overexpression of long non-coding RNA KCNQ1OT1 is related to good prognosis via inhibiting cell proliferation in non-small cell lung cancer. Thorac. Cancer 9 (5), 523–531. doi:10.1111/1759-7714.12599
Sung, H., Ferlay, J., Siegel, R. L., Laversanne, M., Soerjomataram, I., Jemal, A., et al. (2021). Global cancer statistics 2020: GLOBOCAN estimates of incidence and mortality worldwide for 36 cancers in 185 countries. CA Cancer J. Clin. 71 (3), 209–249. doi:10.3322/caac.21660
Tang, H., Zhao, L., Li, M., Li, T., and Hao, Y. (2019). Investigation of LINC00342 as a poor prognostic biomarker for human patients with non-small cell lung cancer. J. Cell Biochem. 120 (4), 5055–5061. doi:10.1002/jcb.27782
Tao, X., Zhang, Y., Li, J., Ni, Z., Tao, Z., You, Q., et al. (2020a). Low expression of long non-coding RNA ARAP1-AS1 can inhibit lung cancer proliferation by inducing G0/G1 cell cycle organization. J. Thorac. Dis. 12 (12), 7326–7336. doi:10.21037/jtd-20-3378
Tao, Y., Tang, Y., Yang, Z., Wu, F., Wang, L., Yang, L., et al. (2020b). Exploration of serum exosomal LncRNA TBILA and AGAP2-AS1 as promising biomarkers for diagnosis of non-small cell lung cancer. Int. J. Biol. Sci. 16 (3), 471–482. doi:10.7150/ijbs.39123
Tian, H., Pan, J., Fang, S., Zhou, C., Tian, H., He, J., et al. (2021). LncRNA DPP10-AS1 promotes malignant processes through epigenetically activating its cognate gene DPP10 and predicts poor prognosis in lung cancer patients. Cancer Biol. Med. 18, 675–692. doi:10.20892/j.issn.2095-3941.2020.0136
Tsiouda, T., Sardeli, C., Porpodis, K., Pilikidou, M., Apostolidis, G., Kyrka, K., et al. (2020). Sex differences and adverse effects between chemotherapy and immunotherapy for non-small cell lung cancer. J. Cancer 11 (11), 3407–3415. doi:10.7150/jca.40196
Wang, C., Tan, S., Li, J., Liu, W. R., Peng, Y., and Li, W. (2020a). CircRNAs in lung cancer - biogenesis, function and clinical implication. Cancer Lett. 492, 106–115. doi:10.1016/j.canlet.2020.08.013
Wang, D., Wu, W., Huang, W., Wang, J., Luo, L., and Tang, D. (2019a). LncRNA LUADT1 sponges miR-15a-3p to upregulate Twist1 in small cell lung cancer. BMC Pulm. Med. 19 (1), 246. doi:10.1186/s12890-019-0991-7
Wang, J., Gao, J., Chen, Q., Zou, W., Yang, F., Wei, C., et al. (2020b). LncRNA LINC01116 contributes to cisplatin resistance in lung adenocarcinoma. Onco Targets Ther. 13, 9333–9348. doi:10.2147/OTT.S244879
Wang, J., He, X., Yao, Q., Wang, C., Lu, X., Wang, R., et al. (2023). LncRNA PTTG3P promotes tumorigenesis and metastasis of NSCLC by binding with ILF3 to maintain mRNA stability and form a positive feedback loop with E2F1. Int. J. Biol. Sci. 19 (13), 4291–4310. doi:10.7150/ijbs.81738
Wang, J., Tan, L., Yu, X., Cao, X., Jia, B., Chen, R., et al. (2022a). lncRNA ZNRD1-AS1 promotes malignant lung cell proliferation, migration, and angiogenesis via the miR-942/TNS1 axis and is positively regulated by the m(6)A reader YTHDC2. Mol. Cancer 21 (1), 229. doi:10.1186/s12943-022-01705-7
Wang, J., Zhang, L., Wang, C., Chen, Y., and Sui, X. (2022b). LINC00313/miR-4429 axis provides novel biomarkers for the diagnosis and prognosis of non-small cell lung cancer. Acta Biochim. Pol. 69 (2), 343–348. doi:10.18388/abp.2020_5794
Wang, L., Cao, L., Wen, C., Li, J., Yu, G., and Liu, C. (2020c). LncRNA LINC00857 regulates lung adenocarcinoma progression, apoptosis and glycolysis by targeting miR-1179/SPAG5 axis. Hum. Cell 33 (1), 195–204. doi:10.1007/s13577-019-00296-8
Wang, R. Q., Long, X. R., Zhou, N. N., Chen, D. N., Zhang, M. Y., Wen, Z. S., et al. (2021). Lnc-GAN1 expression is associated with good survival and suppresses tumor progression by sponging mir-26a-5p to activate PTEN signaling in non-small cell lung cancer. J. Exp. Clin. Cancer Res. 40 (1), 9. doi:10.1186/s13046-020-01819-0
Wang, Y., Yang, L., Chen, T., Liu, X., Guo, Y., Zhu, Q., et al. (2019b). A novel lncRNA MCM3AP-AS1 promotes the growth of hepatocellular carcinoma by targeting miR-194-5p/FOXA1 axis. Mol. Cancer 18 (1), 28. doi:10.1186/s12943-019-0957-7
Wang, Y., Zhang, F., Wang, J., Hu, L., Jiang, F., Chen, J., et al. (2018). lncRNA LOC100132354 promotes angiogenesis through VEGFA/VEGFR2 signaling pathway in lung adenocarcinoma. Cancer Manag. Res. 10, 4257–4266. doi:10.2147/CMAR.S177327
Warburg, O. (1956). On the origin of cancer cells. Science 123 (3191), 309–314. doi:10.1126/science.123.3191.309
Wu, A., Tang, J., Guo, Z., Dai, Y., Nie, J., Hu, W., et al. (2021). Long non-coding RNA CRYBG3 promotes lung cancer metastasis via activating the eEF1A1/MDM2/MTBP Axis. Int. J. Mol. Sci. 22 (6), 3211. doi:10.3390/ijms22063211
Xiao, S., Sun, L., Ruan, B., Li, J., Chen, J., Xiong, J., et al. (2021). Long non-coding RNA LINC01224 promotes progression and cisplatin resistance in non-small lung cancer by sponging miR-2467. Pulm. Pharmacol. Ther. 70, 102070. doi:10.1016/j.pupt.2021.102070
Xie, W., Wang, Y., Zhang, Y., Xiang, Y., Wu, N., Wu, L., et al. (2021). Single-nucleotide polymorphism rs4142441 and MYC co-modulated long non-coding RNA OSER1-AS1 suppresses non-small cell lung cancer by sequestering ELAVL1. Cancer Sci. 112 (6), 2272–2286. doi:10.1111/cas.14713
Xie, Y., Zhang, Y., Du, L., Jiang, X., Yan, S., Duan, W., et al. (2018). Circulating long noncoding RNA act as potential novel biomarkers for diagnosis and prognosis of non-small cell lung cancer. Mol. Oncol. 12 (5), 648–658. doi:10.1002/1878-0261.12188
Xu, F., Hua, Q., Zhang, A., Di, Z., Wang, Y., Zhao, L., et al. (2021). LncRNA AC020978 facilitates non-small cell lung cancer progression by interacting with malate dehydrogenase 2 and activating the AKT pathway. Cancer Sci. 112 (11), 4501–4514. doi:10.1111/cas.15116
Xu, H., Yu, B., Shen, W., Jin, C., Wang, L., and Xi, Y. (2020a). Over-expression of long non-coding RNA ZEB2-AS1 may predict poor prognosis and promote the migration, invasion, and epithelial-mesenchymal transition of tumor cells in non-small cell lung cancer. Int. J. Biol. Markers 35 (3), 29–35. doi:10.1177/1724600820938385
Xu, S., Cheng, Z., Du, B., Diao, Y., Li, Y., and Li, X. (2023). LncRNA AP000695.2 promotes glycolysis of lung adenocarcinoma via the miR-335-3p/TEAD1 axis. Acta Biochim. Biophys. Sin. (Shanghai) 55 (10), 1592–1605. doi:10.3724/abbs.2023227
Xu, S., Zhai, S., Du, T., and Li, Z. (2020b). LncRNA MIR503HG inhibits non-small cell lung cancer cell proliferation by inducing cell cycle arrest through the downregulation of cyclin D1. Cancer Manag. Res. 12, 1641–1647. doi:10.2147/CMAR.S227348
Xu, Y. H., Tu, J. R., Zhao, T. T., Xie, S. G., and Tang, S. B. (2019). Overexpression of lncRNA EGFRAS1 is associated with a poor prognosis and promotes chemotherapy resistance in nonsmall cell lung cancer. Int. J. Oncol. 54 (1), 295–305. doi:10.3892/ijo.2018.4629
Yang, D., Feng, W., Zhuang, Y., Liu, J., Feng, Z., Xu, T., et al. (2021). Long non-coding RNA linc00665 inhibits CDKN1C expression by binding to EZH2 and affects cisplatin sensitivity of NSCLC cells. Mol. Ther. Nucleic Acids 23, 1053–1065. doi:10.1016/j.omtn.2021.01.013
Yang, J., Qiu, Q., Qian, X., Yi, J., Jiao, Y., Yu, M., et al. (2019). Long noncoding RNA LCAT1 functions as a ceRNA to regulate RAC1 function by sponging miR-4715-5p in lung cancer. Mol. Cancer 18 (1), 171. doi:10.1186/s12943-019-1107-y
Yang, K., Zhang, W., Zhong, L., Xiao, Y., Sahoo, S., Fassan, M., et al. (2023a). Long non-coding RNA HIF1A-As2 and MYC form a double-positive feedback loop to promote cell proliferation and metastasis in KRAS-driven non-small cell lung cancer. Cell Death Differ. 30 (6), 1533–1549. doi:10.1038/s41418-023-01160-x
Yang, Q., Kong, S., Zheng, M., Hong, Y., Sun, J., Ming, X., et al. (2020). Long intergenic noncoding RNA LINC00173 as a potential serum biomarker for diagnosis of non-small-cell lung cancer. Cancer Biomark. 29 (4), 441–451. doi:10.3233/CBM-201616
Yang, Q., Wang, M., Xu, J., Yu, D., Li, Y., Chen, Y., et al. (2023b). LINC02159 promotes non-small cell lung cancer progression via ALYREF/YAP1 signaling. Mol. Cancer 22 (1), 122. doi:10.1186/s12943-023-01814-x
Yin, H., Chen, L., Piao, S., Wang, Y., Li, Z., Lin, Y., et al. (2023). M6A RNA methylation-mediated RMRP stability renders proliferation and progression of non-small cell lung cancer through regulating TGFBR1/SMAD2/SMAD3 pathway. Cell Death Differ. 30 (3), 605–617. doi:10.1038/s41418-021-00888-8
Yousefi, H., Maheronnaghsh, M., Molaei, F., Mashouri, L., Reza Aref, A., Momeny, M., et al. (2020). Long noncoding RNAs and exosomal lncRNAs: classification, and mechanisms in breast cancer metastasis and drug resistance. Oncogene 39 (5), 953–974. doi:10.1038/s41388-019-1040-y
Yu, J. S., and Cui, W. (2016). Proliferation, survival and metabolism: the role of PI3K/AKT/mTOR signalling in pluripotency and cell fate determination. Development 143 (17), 3050–3060. doi:10.1242/dev.137075
Yu, T., Bai, W., Su, Y., Wang, Y., Wang, M., and Ling, C. (2020). Enhanced expression of lncRNA ZXF1 promotes cisplatin resistance in lung cancer cell via MAPK axis. Exp. Mol. Pathol. 116, 104484. doi:10.1016/j.yexmp.2020.104484
Yu, W., Ding, J., He, M., Chen, Y., Wang, R., Han, Z., et al. (2019). Estrogen receptor beta promotes the vasculogenic mimicry (VM) and cell invasion via altering the lncRNA-MALAT1/miR-145-5p/NEDD9 signals in lung cancer. Oncogene 38 (8), 1225–1238. doi:10.1038/s41388-018-0463-1
Yuan, S., Xiang, Y., Guo, X., Zhang, Y., Li, C., Xie, W., et al. (2020). Circulating long noncoding RNAs act as diagnostic biomarkers in non-small cell lung cancer. Front. Oncol. 10, 537120. doi:10.3389/fonc.2020.537120
Yue, Q. Y., and Zhang, Y. (2018). Effects of Linc00460 on cell migration and invasion through regulating epithelial-mesenchymal transition (EMT) in non-small cell lung cancer. Eur. Rev. Med. Pharmacol. Sci. 22 (4), 1003–1010. doi:10.26355/eurrev_201802_14382
Zeng, S. H. G., Xie, J. H., Zeng, Q. Y., Dai, S. H. H., Wang, Y., Wan, X. M., et al. (2021). lncRNA PVT1 promotes metastasis of non-small cell lung cancer through EZH2-mediated activation of hippo/NOTCH1 signaling pathways. Cell J. 23 (1), 21–31. doi:10.22074/cellj.2021.7010
Zhan, J., Wang, P., Li, S., Song, J., He, H., Wang, Y., et al. (2019). HOXB13 networking with ABCG1/EZH2/Slug mediates metastasis and confers resistance to cisplatin in lung adenocarcinoma patients. Theranostics 9 (7), 2084–2099. doi:10.7150/thno.29463
Zhang, C., Gong, C., Li, J., and Tang, J. (2021a). Downregulation of long non-coding RNA LINC-PINT serves as a diagnostic and prognostic biomarker in patients with non-small cell lung cancer. Oncol. Lett. 21 (3), 210. doi:10.3892/ol.2021.12471
Zhang, C., Wang, L., Jin, C., Zhou, J., Peng, C., Wang, Y., et al. (2021b). Long non-coding RNA Lnc-LALC facilitates colorectal cancer liver metastasis via epigenetically silencing LZTS1. Cell Death Dis. 12 (2), 224. doi:10.1038/s41419-021-03461-w
Zhang, H., Wang, S. Q., Zhu, J. B., Wang, L. N., Lin, H., Li, L. F., et al. (2023a). LncRNA CALML3-AS1 modulated by m(6)A modification induces BTNL9 methylation to drive non-small-cell lung cancer progression. Cancer Gene Ther. doi:10.1038/s41417-023-00670-7
Zhang, L., Chi, B., Chai, J., Qin, L., Zhang, G., Hua, P., et al. (2021c). LncRNA ccdc144nl-AS1 serves as a prognosis biomarker for non-small cell lung cancer and promotes cellular function by targeting miR-490-3p. Mol. Biotechnol. 63 (10), 933–940. doi:10.1007/s12033-021-00351-6
Zhang, X., Guo, H., Bao, Y., Yu, H., Xie, D., and Wang, X. (2019a). Exosomal long non-coding RNA DLX6-AS1 as a potential diagnostic biomarker for non-small cell lung cancer. Oncol. Lett. 18 (5), 5197–5204. doi:10.3892/ol.2019.10892
Zhang, X., Lu, N., Wang, L., Wang, Y., Li, M., Zhou, Y., et al. (2020). Circular RNAs and esophageal cancer. Cancer Cell Int. 20, 362. doi:10.1186/s12935-020-01451-0
Zhang, Y., Dong, X., Guo, X., Li, C., Fan, Y., Liu, P., et al. (2023b). LncRNA-BC069792 suppresses tumor progression by targeting KCNQ4 in breast cancer. Mol. Cancer 22 (1), 41. doi:10.1186/s12943-023-01747-5
Zhang, Y., Wang, F., Chen, G., He, R., and Yang, L. (2019b). LncRNA MALAT1 promotes osteoarthritis by modulating miR-150-5p/AKT3 axis. Cell Biosci. 9, 54. doi:10.1186/s13578-019-0302-2
Zhang, Y., Wang, X., Hu, C., and Yi, H. (2023c). Shiny transcriptional junk: lncRNA-derived peptides in cancers and immune responses. Life Sci. 316, 121434. doi:10.1016/j.lfs.2023.121434
Zhang, Y. X., Yuan, J., Gao, Z. M., and Zhang, Z. G. (2018). LncRNA TUC338 promotes invasion of lung cancer by activating MAPK pathway. Eur. Rev. Med. Pharmacol. Sci. 22 (2), 443–449. doi:10.26355/eurrev_201801_14193
Zhao, M., Xin, X. F., Zhang, J. Y., Dai, W., Lv, T. F., and Song, Y. (2020). LncRNA GMDS-AS1 inhibits lung adenocarcinoma development by regulating miR-96-5p/CYLD signaling. Cancer Med. 9 (3), 1196–1208. doi:10.1002/cam4.2776
Zhao, W., Zhang, L. N., Wang, X. L., Zhang, J., and Yu, H. X. (2019). Long noncoding RNA NSCLCAT1 increases non-small cell lung cancer cell invasion and migration through the Hippo signaling pathway by interacting with CDH1. FASEB J. 33 (1), 1151–1166. doi:10.1096/fj.201800408R
Zheng, S., Lu, Z., Liu, C., Wang, X., Jin, R., Mao, S., et al. (2019). The tgfβ-induced long non-coding RNA TBULC promotes the invasion and migration of non-small cell lung cancer cells and indicates poor prognosis. Front. Oncol. 9, 1340. doi:10.3389/fonc.2019.01340
Keywords: lung cancer, lncRNA, biomarker, diagnosis, prognosis, therapy
Citation: Fu J, Yu L, Yan H, Tang S, Wang Z, Dai T, Chen H, Zhang S, Hu H, Liu T, Tang S, He R and Zhou H (2023) LncRNAs in non-small cell lung cancer: novel diagnostic and prognostic biomarkers. Front. Mol. Biosci. 10:1297198. doi: 10.3389/fmolb.2023.1297198
Received: 19 September 2023; Accepted: 21 November 2023;
Published: 13 December 2023.
Edited by:
Jiao Li, Sichuan University, ChinaReviewed by:
Zhang Li, West China Xiamen Hospital of Sichuan University, ChinaYuanli Zuo, Sichuan University, China
Copyright © 2023 Fu, Yu, Yan, Tang, Wang, Dai, Chen, Zhang, Hu, Liu, Tang, He and Zhou. This is an open-access article distributed under the terms of the Creative Commons Attribution License (CC BY). The use, distribution or reproduction in other forums is permitted, provided the original author(s) and the copyright owner(s) are credited and that the original publication in this journal is cited, in accordance with accepted academic practice. No use, distribution or reproduction is permitted which does not comply with these terms.
*Correspondence: Rong He, cm9uZ2gyMDIzQDEyNi5jb20=; Haining Zhou, aGFpbmluZ196aG91QHptdS5lZHUuY24=
†These authors have contributed equally to this work and share first authorship