- 1Department of Nephrology, Radboud Institute of Molecular Life Sciences, Radboud University Medical Center, Nijmegen, Netherlands
- 2Division of Nephrology, Department of Internal Medicine, The Einthoven Laboratory for Vascular and Regenerative Medicine, Leiden University Medical Center, Leiden, Netherlands
Background: The glomerular endothelial glycocalyx is degraded during inflammation. The glycocalyx plays a pivotal role in endothelial function and is involved in many processes including binding of chemokines and cytokines, leukocyte trafficking, and preventing proteinuria. HS-based therapeutics are a promising novel class of anti-inflammatory drugs to restore a compromised endothelial glycocalyx under inflammatory conditions. Recently, we demonstrated that treatment with HS extracted from unstimulated glomerular endothelial glycocalyx (unstimulated HSglx) reduced albuminuria during anti-GBM induced glomerulonephritis. Since endothelial HS domains are distinct in unstimulated versus inflammatory conditions, we hypothesized that 1) unstimulated HSglx, 2) LPS-stimulated HSglx, 3) the HS-mimetic fucoidan and 4) the glycosaminoglycan preparation sulodexide, which is a mixture of low molecular weight heparin and dermatan sulfate, might have different beneficial effects in experimental glomerulonephritis.
Methods: The effect of unstimulated HSglx, LPS HSglx, Laminaria japonica fucoidan, or sulodexide on experimental glomerulonephritis was tested in LPS-induced glomerulonephritis in mice. Analyses included urinary albumin creatinine measurement, cytokine expression in plasma and renal cortex, and renal influx of immune cells determined by flow cytometry and immunofluorescence staining. Furthermore, the observed in vivo effects were evaluated in cultured glomerular endothelial cells and peripheral blood mononuclear cells by measuring cytokine and ICAM-1 expression levels. The ability of the compounds to inhibit heparanase activity was assessed in a heparanase activity assay.
Results: Treatment of mice with LPS HSglx or sulodexide near-significantly attenuated LPS-induced proteinuria. All treatments reduced plasma MCP-1 levels, whereas only fucoidan reduced IL-6 and IL-10 plasma levels. Moreover, all treatments reversed cortical ICAM-1 mRNA expression and both fucoidan and sulodexide reversed cortical IL-6 and nephrin mRNA expression. Sulodexide decreased renal influx of CD45+ immune cells whereas renal influx of macrophages and granulocytes remained unaltered for all treatments. Although all compounds inhibited HPSE activity, fucoidan and sulodexide were the most potent inhibitors. Notably, fucoidan and sulodexide decreased LPS-induced mRNA expression of ICAM-1 and IL-6 by cultured glomerular endothelial cells.
Conclusion: Our data show a potentially protective effect of glycosaminoglycans and fucoidan in experimental glomerulonephritis. Future research should be aimed at the further identification of defined HS structures that have therapeutic potential in the treatment of glomerular diseases.
Introduction
Glomerulonephritis is characterized by immune-mediated glomerular damage in primary glomerular disease such as anti-glomerular basement membrane disease or secondary glomerular disease like post-infectious glomerulonephritis induced kidney failure (Tecklenborg et al., 2018). Glomerulonephritis is characterized by glomerular influx of leukocytes, albuminuria and loss of kidney function, which may ultimately proceed to end-stage renal disease (Duffield, 2010).
The endothelial glycocalyx is a thick glycan layer that lines blood vessels, contributes to charge and size-selective blood filtration and plays a central role in inflammation. A large proportion of the endothelial glycocalyx is comprised of non-sulfated hyaluronic acid, which provides the gel-like structure of the glycocalyx. Heparan sulfate (HS) is a linear, negatively charged glycosaminoglycan (GAG) that provides specificity to the endothelial glycocalyx function by binding of chemokines and cytokines, adherence of leukocytes, and contributes to the size and charge selective barrier function of the endothelium (Fridén et al., 2011; Garsen et al., 2014; van der Vlag and Buijsers, 2020). The diverse functions of HS are dictated by its enormous structural complexity, which leads to an immense number of structurally different domains that can bind specific ligands and thus modulates multitude physiological and pathological processes (Kreuger and Kjellén, 2012).
The HS-modifying enzymes are differentially expressed in tissues, cell types and under, for example, inflammatory conditions, thereby creating tissue-, cell- and context-specific expression of HS domains (Saphire et al., 2001; Carter et al., 2003; Rops et al., 2004a). Previously, we identified specific HS domains in the glomerular endothelial glycocalyx that are involved in binding of leukocytes, which was associated with an altered expression of HS-modifying enzymes (Rops et al., 2007a; Rops et al., 2008). Under healthy conditions, endothelial HS prevents the adhesion of inflammatory components, such as selectins and integrins expressed by leukocytes, as well as cytokines and chemokines. However, extensive remodelling of HS occurs during inflammation and results in the display of pro-inflammatory HS domains (Rops et al., 2007b; Rops et al., 2008; Farrugia et al., 2018). These pro-inflammatory HS domains can bind chemokines that attract and activate leukocytes, as well as selectins and integrins expressed by leukocytes. This pro-inflammatory attraction and activation contributes to the infiltration of leukocytes that release tissue-damaging effector molecules.
HS in the endothelial glycocalyx can be degraded by heparanase-1 (HPSE) under inflammatory conditions. HPSE is the only known mammalian enzyme that can cleave HS. HPSE can degrade HS domains, thereby allowing the synthesis of new HS domains that, for example, can bind chemokines, selectins and integrins (Garsen et al., 2014; van der Vlag and Buijsers, 2020). The expression of HPSE is increased in the majority of glomerular diseases and linked to a reduced HS expression and the development of proteinuria. Notably, we recently showed that HPSE is essential for the development of proteinuria and renal damage in experimental glomerulonephritis and diabetic nephropathy, since HPSE-deficient mice displayed reduced proteinuria compared to wild-type mice (Gil et al., 2012; Garsen et al., 2016).
The importance of HPSE in the development of proteinuria implies that compounds that inhibit HPSE activity may have therapeutic benefits for patients with inflammatory glomerular diseases (Masola et al., 2018; van der Vlag and Buijsers, 2020). Drugs that inhibit HPSE can be divided into two groups. Firstly, inhibitors that directly block the HS-binding and active site of HPSE. Secondly, HS-mimetics, which are drugs that structurally resemble HS such as heparin-based drugs (Farrugia et al., 2018; van der Vlag and Buijsers, 2020). Currently, a few heparin-based HPSE inhibitors are under development. The heparin-based HS mimetic SST0001 has for instance been shown to reduce albuminuria 2-fold in experimental type 1 and 2 diabetic nephropathy (Ritchie et al., 2011; Gil et al., 2012). Another GAG preparation that has been explored as possible therapeutic in glomerular disease is sulodexide, which is a mixture of low molecular weight heparin (LMWH) and dermatan sulfate. Conflicting results have been obtained with regard to sulodexide. Sulodexide effectively restored the glycocalyx thickness and showed a trend towards normalization of systemic albumin clearance in a study of type 2 diabetes mellitus patients, whereas no such effect was observed in two other studies (Poplawska et al., 1997; Broekhuizen et al., 2010; Lewis et al., 2011; Packham et al., 2012; Rabelink et al., 2017). These contradicting findings can possibly be explained by lack of insight into the specific structures within different sulodexide preparations due to different animal sources used for isolation.
Fucoidans are a class of heterogenous, branched, sulfated polysaccharides that are rich in fucose residues. Fucoidans can be considered as HS-mimetics and might therefore inhibit HPSE activity as well. In particular, fucoidan extracted from the brown seaweed Laminaria japonica resembles HS due to the presence of sulfate groups at similar positions (C-2 and C-3) on the sugar residues and some alpha-1,4 glycosidic linkages in the backbone of the polysaccharide (Wang et al., 2010; Yuan et al., 2022). Due to the low toxicity and wide availability, fucoidans, from a variety of sources, have been investigated as novel carbohydrate-based drugs in cancer, acute pancreatitis, COVID-19, and various kidney diseases (Carvalho et al., 2014; van Weelden et al., 2019; Wang et al., 2022; Yuan et al., 2022; Zahan et al., 2022).
In a recent study, we demonstrated that treatment with exogenous HS extracted from glycocalyx of non-activated glomerular endothelial cells (unstimulated HSglx) attenuates albuminuria in anti-glomerular basement membrane (GBM) induced glomerulonephritis (Maciej-Hulme, van Gemst et al., recently accepted in Frontiers). Since endothelial HS domains are distinct in unstimulated versus inflammatory conditions, we hypothesize that HS extracted under different conditions and compounds similar to HS might have diverse beneficial effects in experimental glomerulonephritis. Therefore, we investigated the effect of 1) unstimulated HSglx, 2) HS-derived from activated glomerular endothelial cells (LPS HSglx), 3) the GAG related HS-mimetic fucoidan and 4) the GAG sulodexide, which is a mixture of low molecular weight heparin (LMWH) and dermatan sulfate, on the clinical outcome of experimental glomerulonephritis in mice. Furthermore, we explored the ability of these four compounds to inhibit HPSE activity.
Methods
Animals
Eight-to-ten-week-old C57Bl/6J female mice (Charles River, Cologne, Germany) were kept under specific pathogen-free conditions and housed in a temperature-controlled room with a 12-h light/dark cycle with ad libitum access to food and water. All experiments were approved by the Animal Ethical Committee of the Radboud University Nijmegen and the Dutch government.
Experimental disease models
Experimental LPS-induced glomerulonephritis was induced in ten-week-old wt C57Bl/6J mice by an i.p. Injection with 110 µg LPS (O111:B4; Sigma-Aldrich, Zwijndrecht, Netherlands) as described previously (Garsen et al., 2016). The mice were treated with 11 µg of HS extracted from unstimulated endothelial glycocalyx (Unstimulated HSglx), HS extracted from LPS stimulated endothelial glycocalyx (LPS HSglx), fucoidan extracted from Laminaria japonica (kind gift of Dr. Bob Long, MicroVascular Health Solutions LLC, Alpine, UT) or sulodexide (kind gift of Dr. Enrique Poradosu, Keryx Biopharmaceuticals, Boston, United States) in PBS via i. v. Injection. I.v. Injection with the treatment compounds was performed prior to i.p LPS injection. Mice were sacrificed 48 h after LPS injection.
Kidneys were collected and snap frozen in liquid nitrogen and urine was collected after 18 h housing in metabolic cages upon termination. Urinary albumin was measured by radial immunodiffusion (Mancini) as described previously (Garsen et al., 2016). Blood urea nitrogen (BUN) and urinary or plasma creatinine concentrations were determined routinely in our clinical diagnostic facility.
Cell culture
Mouse glomerular endothelial cells (mGEnC-1) were cultured as described previously (Rops et al., 2004b). Differentiated mGEnC-1 were stimulated with 100 ng/mL LPS (O111:B4; Sigma-Aldrich) and treated with 10 μg/mL of unstimulated HSglx, LPS HSglx, Fucoidan or sulodexide. Treatment was added 1 h prior to LPS stimulation.
Human peripheral blood mononuclear cells (PBMCs) were isolated by differential centrifugation over Ficoll-Paque (Lymphoprep, StemCell Technologies, Inc.). PBMCs were washed three times in PBS, resuspended in RPMI culture medium supplemented with 2 mM glutamax, 1 mM pyruvate and penicillin/streptomycin (all from Thermo Fisher Scientific, Breda, Netherlands), and counted on a Casy counter. PBMCs from at least three different donors were seeded into 96-well flat bottom plates in a density of 500,000 cells per well. Cells were allowed to adhere for 1 h at 37°C. Cells were washed three times with PBS prior to stimulations. PBMCs were stimulated with 10 ng/mL LPS (E. coli 055:B5; Cat#trlrl-pb5lps; Invivogen, Huissen, Netherlands) and 10 μg/mL of unstimulated HSglx, LPS HSglx, fucoidan or sulodexide. Treatment was added 1 h prior to LPS stimulation.
Extraction, isolation and fractionation of glycocalyx constituents
Glycocalyx was extracted from mGEnC cell layers by overnight digestion with 125 μg/mL proteinase K (Merck chemicals B.V., Amsterdam, Netherlands) in 50 mM Tris-HCl (pH: 7.9), 10 mM NaCl, 3 mM MgCl2, 1% triton X-100 buffer, followed by overnight DNAse-I (Qiagen, Venlo, Netherlands) and RNAse (GE-healthcare, Eindhoven, Netherlands) treatment at 37°C. NaCl was added to digested extracts (final concentration of 2 M), followed by chloroform (1:1), vortexing and centrifugation for 20 min at 4,636 g to separate the phases. The upper layer (aqueous phase) was dialyzed against 5 × 5 L baths of Milli-Q H2O using SnakeSkin dialysis membranes (MWCO 3500 Da, Thermo Fisher Scientific) and dried using a Savant SC210A Speed-Vac concentrator (Thermo Fisher Scientific). Isolation of HSglx from extracted glycocalyx was performed as described previously (Guimond et al., 2009; Maciej-Hulme et al., 2020). In brief, dialyzed and concentrated glycocalyx extracts were digested with 125 mU of Chondroitinase ABC (Sigma-Aldrich) in 25 mM Tris, 2 mM Mg(Ac)2 pH 8 for 18 h before fractionation by anion exchange chromatography using DEAE-sepharose CL-6B beads (Sigma-Aldrich) equilibrated in PBS. Bound HSglx was washed with 0.25 M NaCl in PBS, pH 7.4 and then eluted with 2 M NaCl in PBS, pH 7.4. Isolated HSglx was desalted via PD10 desalting columns (GE Healthcare, Sephadex G25) using Milli-Q H2O. Size fractionation of purified mGEnC HSglx was performed in 0.25 M ammonium bicarbonate at 0.22 mL/min using a BioGel P10 resin column (75 mm × 16 mm, 90–180 µm beads, Bio-Rad, Lunteren, Netherlands). 1 mL fractions were collected and pooled into corresponding peaks. Pooled fractions were dialyzed against Milli-Q water and dried. Mass spectrometry was performed as previously described (Maciej-Hulme, van Gemst et al., recently accepted in frontiers) and Fraction 2 (F2) was selected based on activity in previous experiments (Maciej-Hulme, van Gemst et al., recently accepted in frontiers) as well as the small size of these oligosaccharide structures (between tetra- and hexasaccharides).
Cytokine measurements
Cytokine levels in plasma from mice were measured using the LEGENDplex mouse inflammation panel (Cat# 740150; Biolegend, Amsterdam, Netherlands). The cytokines included in this panel are IL-1α, IL-1β, IL-6, IL-10, IL-12p70, IL-17A, IL-23, IL-27, MCP-1, IFN-β, IFN-γ, TNF-α, and GM-CSF. The experiment was performed according to manufacturer’s instructions and data was analyzed using the provided software.
Cytokine production of mGEnC-1 and PBMCs was measured in culture supernatants using the commercial ELISA kits mouse DuoSet MCP-1 (Cat#DY479; R&D systems, Bio-techne, Abingdon, United Kingdom), human DuoSet IL-6 (Cat#DY206; R&D systems), human DuoSet TNF-α (Cat#DY210; R&D systems), and human DuoSet IL-10 (Cat#DY217; R&D systems). The entire procedure was performed according to manufacturer’s instructions. Samples were diluted 20 times in PBA (1% BSA in PBS) for PBMC culture supernatants and 5 times in PBA for mGEnC-1 culture supernatants.
RNA isolation and real-time PCR
RNA was isolated from the kidney cortex using RNeasy mini kit (Qiagen) and from mGEnC-1 using TRIzol (Thermo Fisher Scientific) and 1 µg of RNA was reverse transcribed into cDNA using the Transcription First Strand cDNA synthesis kit (Roche, Woerden, Netherlands) according to manufacturer’s instructions. Quantitative PCR was performed with SYBR Green (Roche) on a CFX 96 C1000 Thermal Cycler (Bio-rad). Data was analyzed using glyceraldehyde-3-phosphate dehydrogenase (GAPDH) as housekeeping gene and by making use of the delta-delta CT method. Sequences of gene-specific primers are listed in Table 1.
Immunofluorescence staining
Glomerular presence of granulocytes and monocytes, and expression of ICAM-1, VCAM-1, agrin (marker for glomerular basement membrane (GBM)), PDGFR (marker for mesangial cells), and CD31 (marker for endothelial cells) was determined by immunofluorescence staining on 2 µm sections of fresh-frozen kidneys. Primary antibodies included rat anti-mouse Gr-1 (Ly6G) (cat#14-5931-85, Invitrogen, RRID:AB_467731), rat anti-mouse CD68 (Cat#MCA 1957, RRID:AB_322219 Serotec, Oxford, United Kingdom), rat anti-mouse ICAM-1 (Cat# 14-0541-85, RRID:AB_467302, Thermo Fisher Scientific), rabbit anti-mouse VCAM-1 (Cat# NBP2-67292, Novus Biologicals), Armenian hamster anti-mouse MI-91 (anti-agrin (Raats et al., 1998)), rabbit anti-mouse PDGFR (Cat# ab32570, RRID:AB_777165, Abcam, Cambridge, United Kingdom), and rat anti-mouse CD31 (Cat# 553369, RRID:AB_394815, BD Biosciences, Vianen, Netherlands). Secondary antibodies included goat anti-rat alexa 488 (Cat# A-11006, RRID:AB_2534074, Thermo Fisher Scientific) for Gr-1, CD68 and ICAM-1, chicken anti-rabbit alexa 488 (Cat# A-21441, RRID:AB_2535859, Thermo Fisher Scientific) for VCAM-1 and PDGFR, goat anti-rat alexa 594 (Cat#A-11007, RRID:AB_10561522, Thermo Fisher Scientific) for CD31, and goat anti-armenian hamster 594 (Cat#A78966, Thermo Fisher Scientific) for MI-91. Quantification of granulocytes and monocytes was performed by counting the number of granulocytes and monocytes in 50 glomeruli per section. Staining intensity of ICAM-1 was scored in 30 glomeruli per section on a scale between 0 (no staining) and 10 (maximal staining). Counting and scoring was performed on blinded sections by two independent investigators.
Flow cytometry
Half of a kidney, directly obtained after sacrificing, was used for flow cytometry analysis. Kidneys were cut in pieces and put in an enzymatic digestion solution containing 0.2 mg/mL Collagenase IV (Cat#C5138, Sigma-Aldrich) and 0.05 mg/mL deoxyribonuclease (DNase) I (Cat#1 0104159 001, Sigma-Aldrich), and placed in a 37°C incubator for 60 min. Cells were forced through a 70 μm strainer and resuspended in an Ammonium-Chloride-Potassium (ACK) lysis buffer (155 mM NH4Cl, 10 mM KHCO3, 0.1 mM EDTA) for 5 min at room temperature (RT). For flow cytometry staining, 5 × 106 cells were used per sample. Possible Fc receptors on cells were blocked with mouse BD FC block (Cat#553142, BD Bioscience). The antibodies that were used in this study are described in Table 2. The antibody dilutions ranged from 1:200 to 1:100. Flow cytometry was performed with the NovoSampler Pro (ACEA Biosciences, San Diego, United States), and data were analyzed in the NovoExpress software version 1.5.6 (ACEA Biosciences) as described in Supplementary Figure S4.
HPSE activity assay
The inhibition of HPSE activity by unstimulated HSglx, LPS HSglx, fucoidan or sulodexide was determined by an in-house developed HPSE activity assay, which was validated by the use of recombinant active human HPSE (Cat#7570-GH-005, Bio-techne), and performed as described previously (Buijsers et al., 2020a). Briefly, Nunc maxisorp flat bottom 96 plates (Thermo Scientific, Breda, Netherlands) were coated with 10 μg/mL heparan sulfate from bovine kidney (HSBK) (Sigma-Aldrich) in HS coating buffer, overnight in a humidified chamber at RT. Subsequently, plates were blocked for minimal 2 h with 1% bacto-gelatin (Difco laboratories, Detroit, Michigan, United States) in PBS at RT, whereupon HPSE inhibitors were added to the plate with a standard amount of recombinant HPSE in HPSE buffer. Remaining HSBK was detected with primary mouse anti-rat IgM HS antibody JM403 (Cat#370730-S, RRID: AB_10890960, 1 μg/mL in PBST, Amsbio, Abingdon, United Kingdom) for 1 h at RT. Subsequently, plates were incubated with secondary goat anti-mouse IgM HRP antibody (Cat#1020-05, RRID: AB_2794201, dilution 1:10,000 in PBST, Southern Biotech, Uden, Netherlands) for 1 h at RT. Finally, 3,3′,5,5′-tetramethylbenzidine (TMB) substrate (Invitrogen) was added and the reaction was stopped by addition of 2 M sulfuric acid, and absorbance was measured at 450 nm.
Statistical analyses
Values are expressed as mean ± SEM. Shapiro-Wilk test or Kolmogorov-Smirnov test was performed to test for normality of data. Significance was determined by one-way ANOVA followed by Dunnett’s test or Kruskal–Wallis test followed by Dunn’s test to compare more than two groups. All analyses were performed using GraphPad Prism V.9.1.2 (La Jolla, CA, United States). p values less than 0.05 were considered as statistically significant.
Results
Treatment with LPS HSglx or sulodexide tend to reduce proteinuria in mice with experimental glomerulonephritis
Since LPS HSglx is extracted from endothelial cells under inflammatory conditions, it is likely that LPS HSglx is enriched in pro-inflammatory HS, which fuels the hypothesis that LPS HSglx might be more effective for the treatment of glomerulonephritis compared to unstimulated HSglx. Indeed, LPS HSglx attenuated proteinuria almost significantly (p = 0.07) in mice with LPS-induced glomerulonephritis, whereas unstimulated HSglx was less potent. Sulodexide tended to reduce (p = 0.05) proteinuria in mice with LPS-induced glomerulonephritis as well while no clear effects were observed for fucoidan (Figure 1A). Notably, the LPS-induced glomerulonephritis model did not affect kidney function as measured by blood urea levels (Figure 1B) and plasma creatinine levels (data not shown).
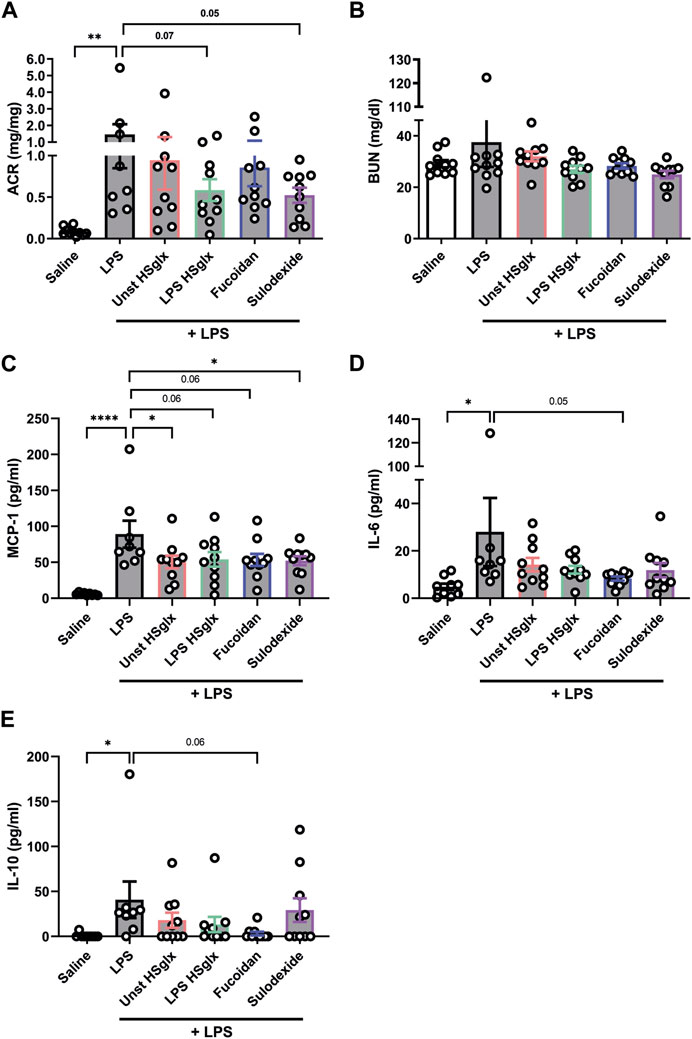
FIGURE 1. Albuminuria and plasma cytokine levels in mice with LPS-induced glomerulonephritis are in part normalized by treatment with unstimulated HSglx, LPS HSglx, fucoidan or sulodexide in mice. (A). Urinary albumin/creatinine ratio and (B). Renal function, as measured by BUN of mice injected with LPS (48 h) and treated with unstimulated HSglx, LPS HSglx, fucoidan or sulodexide. (C). MCP-1, (D). IL-6, and (E). IL-10 plasma protein levels of mice injected with LPS (48 h) and treated with unstimulated HSglx, LPS HSglx, fucoidan or sulodexide. Data are expressed as mean ± SEM. *p < 0.05, **p < 0.01, ****p < 0.0001. n ≥ 8. Unstimulated HSglx, HS extracted from unstimulated endothelial glycocalyx; LPS HSglx, HS extracted from LPS stimulated endothelial glycocalyx; ACR, urinary albumin/creatinine ratio; BUN, blood urea nitrogen plasma level; MCP-1, monocyte chemoattractant protein-1.
Treatment with GAGs or fucoidan affects cytokine expression levels in experimental glomerulonephritis
Since HS plays a predominant role in inflammation and cytokine increase is a main feature in LPS-induced glomerulonephritis, we investigated if cytokine expression was altered in the mice treated with unstimulated HSglx, LPS HSglx, fucoidan or sulodexide compared to LPS-injected mice that did not receive treatment. MCP-1, IL-6 and IL-10 plasma levels showed a significant increase in mice with LPS-induced glomerulonephritis compared to control mice (Figures 1B–D). On the contrary, no significant differences in plasma levels were detected between control mice and mice with LPS-induced glomerulonephritis for TNF-α, IL-23, IL-17A, IL-27, IL-12, IL-1α, IL-1β, IFN-γ, IFN-β, and GM-CSF (Supplementary Figures S1, S2).
Notably, MCP-1 plasma levels tended to be normalized by all 4 treatment conditions compared to mice with LPS-induced glomerulonephritis without further treatment (Figure 1C), which was not reflected in the cortical mRNA expression level of MCP-1 (Figure 2A). IL-6 plasma levels (Figure 1D) in mice with LPS-induced glomerulonephritis treated with fucoidan were near-significantly (p = 0.05) decreased, whereas treatment with unstimulated HSglx, LPS HSglx or sulodexide showed a trend to decrease plasma IL-6 levels, which was in part mirrored in the cortical mRNA expression levels for IL-6 (Figure 2B). IL-10 plasma levels in mice with LPS-induced glomerulonephritis tended to decrease for all 4 treatments (Figure 1E) even though no differences were detected at the cortical mRNA levels for IL-10 (Figure 2C). Notably, the TNF-α mRNA expression level in the renal cortex did not significantly increase in mice with LPS-induced glomerulonephritis, and was not further affected by any of the treatments (Supplementary Figure S3A).
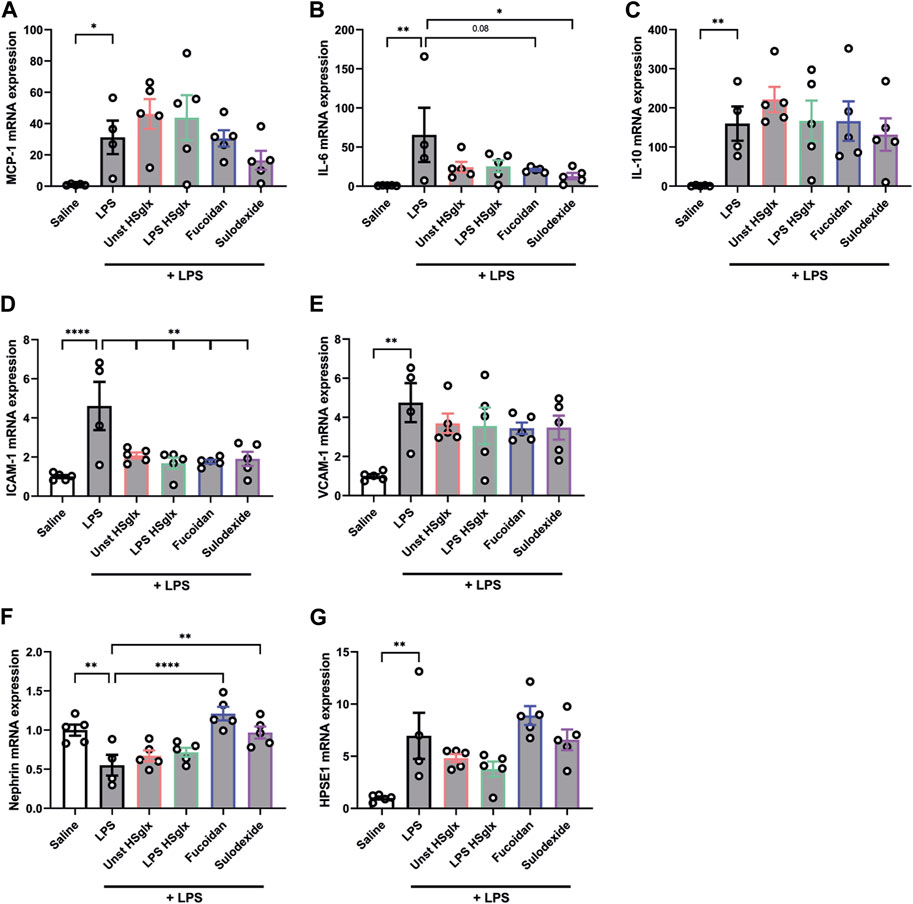
FIGURE 2. Cortical mRNA expression levels of IL-6, ICAM-1 and Nephrin in mice with LPS-induced glomerulonephritis are affected by treatment with unstimulated HSglx, LPS HSglx, fucoidan or sulodexide. (A). MCP-1, (B). IL-6, (C). IL-10, (D). ICAM-1, (E). VCAM-1, (F). Nephrin, and (G). HPSE1 cortical mRNA expression of mice injected with LPS (48 h) and treated with unstimulated HSglx, LPS HSglx, fucoidan or sulodexide. Data are expressed as mean ± SEM. *p < 0.05, **p < 0.01, ****p < 0.0001. n ≥ 4. Unstimulated HSglx, HS extracted from unstimulated endothelial glycocalyx; LPS HSglx, HS extracted from LPS stimulated endothelial glycocalyx; MCP-1, monocyte chemoattractant protein-1; ICAM-1, intercellular adhesion molecule 1; VCAM-1, vascular cell adhesion molecule-1; HPSE1, heparanase.
The endothelial nitric oxide synthase (eNOS) was previously shown to be involved in LPS-induced cytokine increase (Gross et al., 2015). In our study, the cortical eNOS mRNA expression did not significantly differ between mice with LPS-induced glomerulonephritis and control mice and was also not further affected by any of the GAG/fucoidan treatments (Supplementary Figure S3C).
The results of our in vivo experiments collectively indicate that treatment of LPS-induced glomerulonephritis with GAGs or fucoidan partially affect cytokine expression levels in plasma and kidney cortex.
Treatment with GAGs or fucoidan affects endothelial expression of cell adhesion molecules in experimental glomerulonephritis
Intercellular adhesion molecule 1 (ICAM-1) and vascular cell adhesion molecule 1 (VCAM-1) are endothelial adhesion molecules of the immunoglobulin superfamily with a critical role in leukocyte trafficking on endothelial cells in various inflammatory diseases (Brady, 1994; Hill et al., 1994). Since glomerular influx of immune cells plays a critical role in the development of some glomerular diseases (Duffield, 2010), the mRNA expression of endothelial leukocyte adhesion molecules ICAM-1 and VCAM-1 in the renal cortex was measured. Both ICAM-1 (Figure 2D) and VCAM-1 (Figure 2E) cortical mRNA expression showed a significant increase in LPS-induced glomerulonephritis compared to control mice. Unstimulated HSglx, LPS HSglx, fucoidan or sulodexide significantly reduced the LPS-induced cortical ICAM-1 mRNA expression although none of the treatments reduced the LPS-induced cortical VCAM-1 mRNA expression (Figures 2D, E).
Subsequently, the ICAM-1 and VCAM-1 protein localization and expression levels were determined by immunofluorescence (IF) staining on kidney sections, in which agrin staining was used to visualize the GBM. Both glomerular ICAM-1 (Figures 3A, B) and VCAM-1 (Figure 3C) protein expression were increased in mice with LPS-induced glomerulonephritis compared to control mice. Increased glomerular ICAM-1 expression colocalized with the endothelial marker CD31 (Supplementary Figure S5A), thereby suggesting activation of the glomerular endothelium. ICAM-1 expression also appeared increased in tubular apical membranes and peritubular capillaries (Figure 3A). Nevertheless, glomerular ICAM-1 protein expression in mice with LPS-induced glomerulonephritis mice was not affected by treatment with GAGs or Fucoidan (Figure 3B). VCAM-1 expression did not colocalize with the endothelial cell marker CD31 upon LPS stimulation (Supplementary Figure S5B). In fact, the increased VCAM-1 expression appears to colocalize with the mesangial marker platelet-derived growth factor receptor (PDGFR) (Figure 3C).
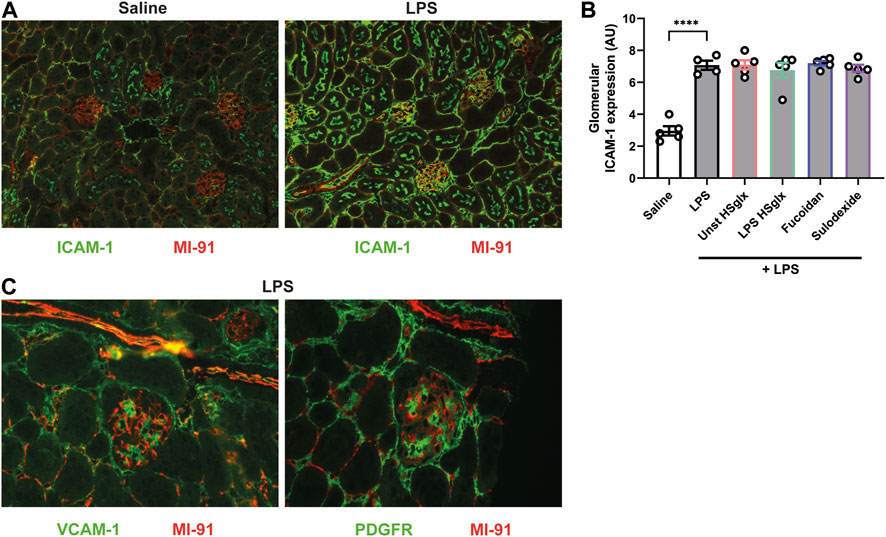
FIGURE 3. Endothelial ICAM-1 expression is increased in LPS-induced glomerulonephritis and is not affected by treatment with unstimulated HSglx, LPS HSglx, fucoidan or sulodexide. (A). Expression of ICAM-1 in control kidneys and kidneys of mice with LPS-induced glomerulonephritis combined with agrin staining to visualize the glomerular basement membrane (B). Semiquantitative analysis of the glomerular expression of ICAM-1 in control mice and mice with LPS induced glomerulonephritis, untreated and treated with unstimulated HSglx, LPS HSglx, fucoidan or sulodexide. (C). Expression of VCAM-1 and the mesangial cell marker PDGFR shows that VCAM-1 is upregulated in mesangial cells in LPS-induced glomerulonephritis. IF staining is presented on subsequent kidney sections, in which the same glomerulus was imaged because the antibodies for VCAM-1 and PDGFR could not be combined on a single kidney section. Data are expressed as mean ± SEM. ****p < 0.0001. n ≥ 4. Unstimulated HSglx, HS extracted from unstimulated endothelial glycocalyx; LPS HSglx, HS extracted from LPS stimulated endothelial glycocalyx; ICAM-1, intercellular adhesion molecule 1; VCAM-1, vascular cell adhesion molecule-1; PDGFR, platelet-derived growth factor receptor; AU, arbitrary units.
Fucoidan and sulodexide prevent podocyte damage in experimental glomerulonephritis
Nephrin is expressed at the podocyte intercellular junction in the glomerulus and is a key component of the podocyte slit diaphragm, which is crucial to glomerular filtration. Previous studies have shown decreased nephrin expression in various human proteinuric kidney diseases as well as animal models of glomerular disease (Luimula et al., 2000; Verma et al., 2018). Therefore, we were interested if the LPS-induced decrease in nephrin expression in the renal cortex could be reversed by treatment with unstimulated HSglx, LPS HSglx, fucoidan, or sulodexide. Unstimulated HSglx and LPS HSglx showed a slight increase in cortical nephrin expression, whereas both fucoidan or sulodexide significantly attenuated the LPS-induced decrease in cortical nephrin expression (Figure 2F). Notably, the cortical mRNA expression of desmin, which is a podocyte activation and damage marker, was not affected by LPS-induced glomerulonephritis (Supplementary Figure S3B). These results suggest that in particular fucoidan and sulodexide seem to protect LPS-induced podocyte damage.
Treatment with unstimulated HSglx, fucoidan or sulodexide reduce renal influx of immune cells in experimental glomerulonephritis
The influx of immune cells in the kidney was determined since immune cells play a prime role in the development of glomerulonephritis. The influx of all CD45+, a versatile marker for all leukocytes, immune cells was increased in kidneys of LPS-induced glomerulonephritis mice compared to control mice and tended to be decreased by treatment with unstimulated HSglx and fucoidan, whereas treatment with sulodexide resulted in a significantly decreased influx (Figure 4A).
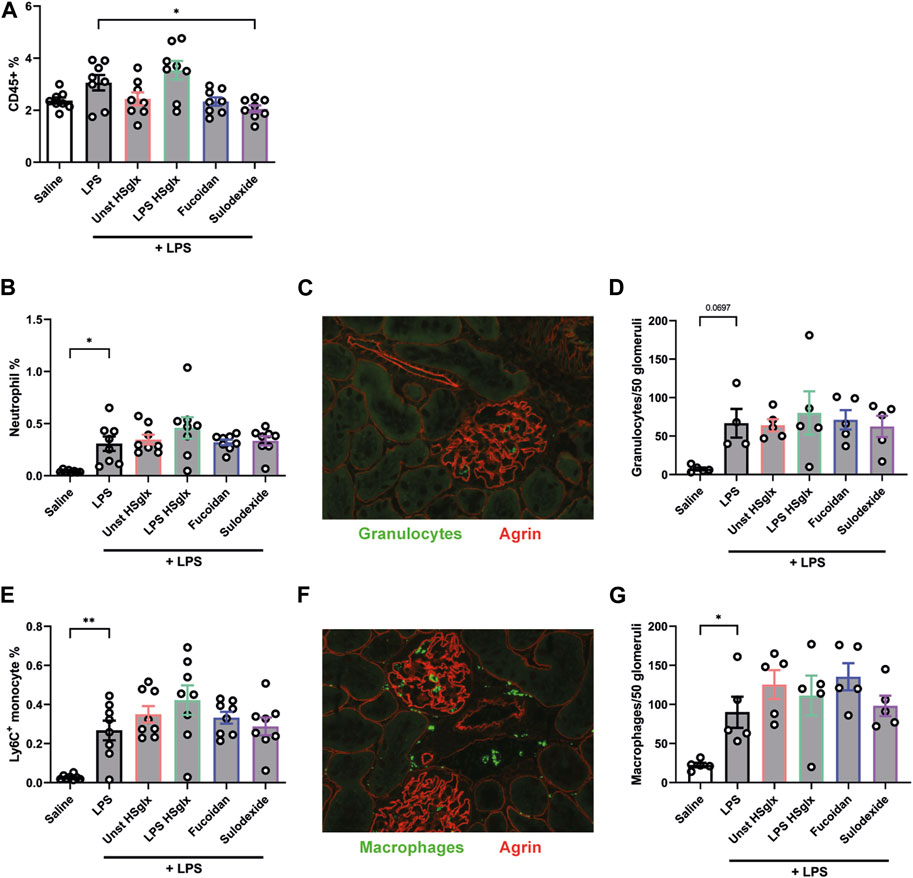
FIGURE 4. Treatment with unstimulated HSglx, fucoidan or sulodexide resulted in a decreased renal influx of immune cells in LPS-induced glomerulonephritis. Quantitative analysis of the influx of immune cells, specifically granulocytes and monocytes using flow cytometry and immunofluorescence. (A). CD45+ leukocyte cell percentage of all gated cells using flow cytometry analysis. (B). Neutrophil as percentage of all gated cells using flow cytometry analysis. (C). Glomerular granulocyte influx as stained for with the marker Gr-1 in immunofluorescence. (D). Quantitative analysis of the glomerular influx of granulocytes in mice injected with LPS and treated with unstimulated HSglx, LPS HSglx, fucoidan or sulodexide. (E). Monocyte Ly6Chigh cell percentage of all gated cells using flow cytometry analysis. (F). Glomerular macrophage influx as stained for with the marker CD68 in immunofluorescence. (G). Quantitative analysis of the glomerular influx of macrophages (CD68) in mice with LPS-induced glomerulonephritis, untreated and treated with unstimulated HSglx, LPS HSglx, fucoidan or sulodexide. Data are expressed as mean ± SEM. *p < 0.05, **p < 0.01. n ≥ 4. Unstimulated HSglx, HS extracted from unstimulated endothelial glycocalyx; LPS HSglx, HS extracted from LPS stimulated endothelial glycocalyx; Gr-1, granulocyte-differentiation antigen; Ly6C, lymphocyte antigen 6C.
The influx of neutrophils (Figure 4B) and Ly6C+ monocytes (Figure 4E) was increased in the kidneys of mice with LPS-induced glomerulonephritis but remained unaffected by treatment with unstimulated HSglx, LPS HSglx, fucoidan or sulodexide. Notably, quantitative analysis of glomerular granulocytes (Figures 4C, D) and glomerular macrophages influx (Figures 4F, G) revealed comparable results with the aforementioned influx of immune cells into the whole kidney as measured by flow cytometry. In summary, the inability of the compounds to attenuate the ICAM-1 protein expression in experimental glomerulonephritis is mirrored in the glomerular influx of granulocytes and monocytes.
Unstimulated HSglx, LPS HSglx, fucoidan and sulodexide all dose-dependently inhibit HPSE activity
Previous studies have shown the importance of HPSE in the development of experimental glomerulonephritis (Garsen et al., 2016). In this study, we confirmed that cortical HPSE mRNA expression was increased in the LPS-induced glomerulonephritis mice compared to controls but remained largely unaltered with the treatments (Figure 2G). Subsequently, we addressed the ability of the different compounds to inhibit HPSE activity. We observed a dose-dependent inhibition of HPSE activity in vitro (Figure 5) for all compounds, which is in line with previous reports that proved the HPSE inhibitory capacity of HS and sulodexide (Masola et al., 2012; Farrugia et al., 2018). Notably, our data proved direct inhibition of heparanase activity by fucoidan, which was not reported previously, and fucoidan even showed highest potency in HPSE activity inhibition followed by sulodexide, unstimulated HSglx and LPS HSglx. These results suggest that inhibition of HPSE activity might contribute to the observed beneficial effects of GAGs and fucoidan in experimental glomerulonephritis.
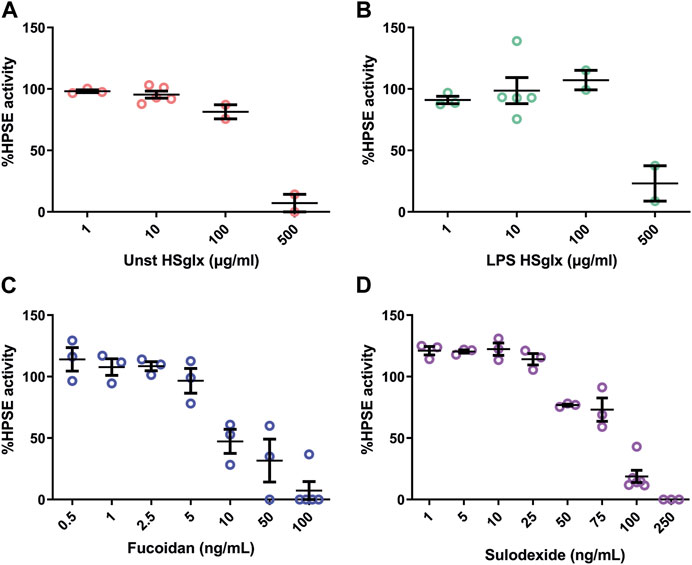
FIGURE 5. HPSE activity is dose-dependently inhibited by unstimulated HSglx, LPS HSglx, fucoidan or sulodexide. Dose response inhibition of recombinant human HPSE with (A) unstimulated HSglx (B). LPS HSglx, (C). fucoidan and (D). sulodexide. Data are expressed as mean ± SEM. Unstimulated HSglx, HS extracted from unstimulated endothelial glycocalyx; LPS HSglx, HS extracted from LPS stimulated endothelial glycocalyx; HPSE, heparanase-1.
Pre-treatment with fucoidan reduces LPS-induced expression of ICAM-1 and IL-6 in mGEnC-1, but not in PBMCs
The observed effects of unstimulated HSglx, LPS HSglx, fucoidan and sulodexide on cytokine mRNA and protein levels and ICAM-1 mRNA levels in LPS-induced glomerulonephritis can be attributed to both glomerular cells and immune cells. It is important to gain a better understanding about which cells are targeted in what manner by the treatments. Therefore, both mouse glomerular endothelial cells (mGEnC-1) and human peripheral blood mononuclear cells (PBMC) were pre-treated with unstimulated HSglx, LPS HSglx, fucoidan or sulodexide and subsequently stimulated with LPS. Both unstimulated HSglx and LPS HSglx did not affect the LPS-induced mRNA expression of ICAM-1 (Figure 6A), IL-6 (Figure 6B), or MCP-1 (Figure 6C) nor the protein secretion of MCP-1 in mGEnC-1 activated with LPS (Figure 6D). Sulodexide showed a decreased trend of ICAM-1 (Figure 6A) and IL-6 (Figure 6B) mRNA expression levels (Figure 6B) while no alterations were observed for MCP-1 expression levels (Figures 6C, D). Fucoidan significantly attenuated LPS-induced mGEnC-1 mRNA expression of ICAM-1 (Figure 6A) and showed a decreased trend of IL-6 mRNA expression (Figure 6B). Although fucoidan increased the mGEnC-1 mRNA expression of MCP-1 (Figure 6C), no effect was observed on MCP-1 protein secretion (Figure 6D). In addition, the PBMC protein secretion of IL-6, IL-10 and TNF-α was not attenuated by the addition of GAGs or fucoidan (Figures 7A–C). Taken together, pre-treatment with fucoidan was able to normalize the ICAM-1 and IL-6 expression of mGEnC-1, but not PBMCs, exposed to LPS.
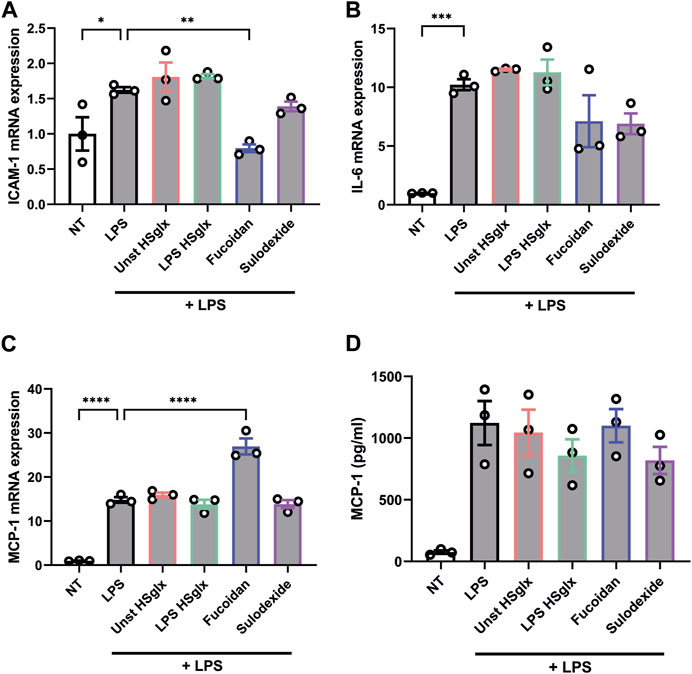
FIGURE 6. LPS-induced ICAM-1 mRNA expression in cultured mouse glomerular endothelial cells is normalized by pre-treatment with fucoidan. qPCR analysis of (A). ICAM-1, (B). IL-6, (C). MCP-1 mRNA expression and protein secretion levels of (D). MCP-1 measured in culture supernatant by ELISA of mouse glomerular endothelial cells pre-treated with 10 μg/mL unstimulated HSglx, LPS HSglx, fucoidan or sulodexide for 1 h and subsequently stimulated with 100 ng/mL LPS for 24 h. Data are expressed as mean ± SEM. *p < 0.05, **p < 0.01, ***p < 0.001, ****p < 0.0001. n ≥ 3. Unstimulated HSglx, HS extracted from unstimulated endothelial glycocalyx; LPS HSglx, HS extracted from LPS stimulated endothelial glycocalyx; ICAM-1, intercellular adhesion molecule 1; MCP-1, monocyte chemoattractant protein-1.
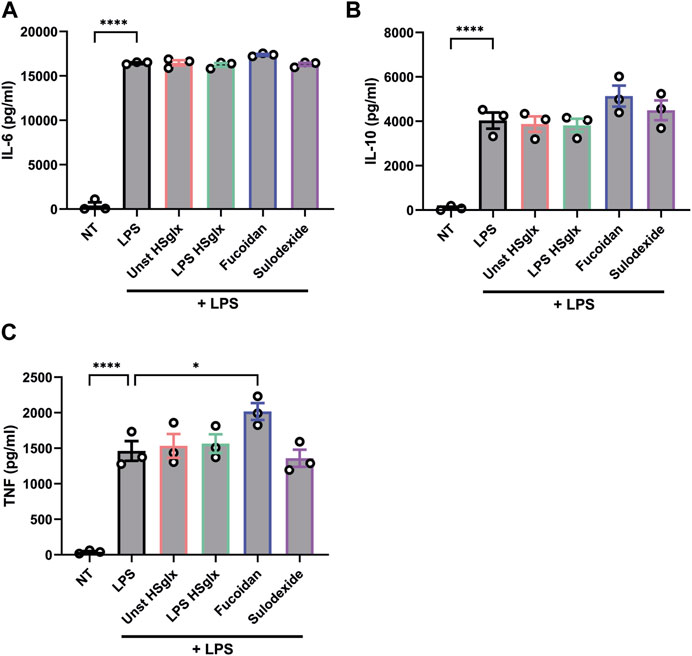
FIGURE 7. LPS-induced IL-6, IL-10 and TNF protein secretion is not affected by pre-treatment with unstimulated HSglx, LPS HSglx, fucoidan or sulodexide in human peripheral blood mononuclear cells. Protein secretion levels of (A). IL-6, (B). IL-10 and (C). TNF measured in culture supernatant of human peripheral blood mononuclear cells pre-treated with 10 μg/mL unstimulated HSglx, LPS HSglx, fucoidan or sulodexide for 1 h and subsequently stimulated with 10 ng/mL LPS for 24 h. Data are expressed as mean ± SEM. *p < 0.05, ****p < 0.0001. n ≥ 3. Unstimulated HSglx, HS extracted from unstimulated endothelial glycocalyx; LPS HSglx, HS extracted from LPS stimulated endothelial glycocalyx.
Discussion
GAG-based therapeutics targeting the glomerular influx of leukocytes are a newly emerging class of drugs for the treatment of a wide range of inflammatory glomerular diseases (Lindahl and Kjellen, 2013; Maciej-Hulme et al., 2018; Muralidar et al., 2021). The current study evaluated the beneficial effects of four HS(-related) compounds in experimental glomerulonephritis. We showed that treatment of mice with LPS HSglx or sulodexide near-significantly attenuated LPS-induced proteinuria. In addition, unstimulated HSglx, LPS HSglx, fucoidan and sulodexide all normalized plasma MCP-1 levels and cortical ICAM-1 mRNA expression whereas no decrease in renal influx of macrophages and granulocytes was observed. Furthermore, fucoidan and sulodexide reversed cortical IL-6 and nephrin mRNA expression. Collectively, we were able to show that unstimulated HSglx, LPS HSglx, fucoidan and sulodexide all have a potentially protective effect in experimental glomerulonephritis.
HS and diversity in HS domains on (glomerular) endothelium are known to play a crucial role in inflammation (Rops et al., 2004a). Although limited information has been gathered on the chemical structure of pro-inflammatory HS domains, N- and 6-O-sulfation are important for leukocyte binding to the glomerular endothelium (Rops et al., 2008; van Gemst et al., 2021). The importance of sulfation of HS in inflammation was demonstrated with the use of N-Deacetylase and N-Sulfotransferase 1 (NDST1) deficient endothelial cells. NDST1 deficient endothelial cells produced HS with low levels of sulfation and behave different under inflammatory conditions compared to wild-type cells, both in vivo and in vitro (Wang et al., 2005). The four different compounds that were tested in this study are all sulfated, but might all comprise slightly different sulfation due to their nature. Therefore, unstimulated HSglx, LPS HSglx, fucoidan and sulodexide were expected to have distinct protective effects in experimental glomerulonephritis.
The influx of immune cells plays a key role in the development of glomerulonephritis. However, we only observed a decrease of kidney influx for all CD45+ cells for unstimulated HSglx, fucoidan and sulodexide, whereas none of the treatments reduced the influx of granulocytes and monocytes in LPS-induced glomerulonephritis. We recently showed decreased influx of granulocytes and macrophages by treatment with unstimulated HSglx in anti-GBM induced glomerulonephritis in mice (Maciej-Hulme, van Gemst et al., recently accepted in Frontiers). Although the unaffected influx of granulocytes and monocytes by any treatment was unexpected, it is in line with the unaltered glomerular ICAM-1 protein expression in the different treatment groups compared to the untreated LPS-induced glomerulonephritis group. The decreased plasma levels of pro-inflammatory cytokines suggest that the immune cells might have been less activated even though the amount of glomerular infiltrating immune cells remained similar or that the majority of produced cytokines are secreted by cell sources other than kidney infiltrating immune cells.
We showed that unstimulated HSglx, LPS HSglx, fucoidan and sulodexide could all dose-dependently inhibit HPSE activity. Fucoidan and sulodexide were most potent in HPSE inhibition and also demonstrated more pronounced effects on LPS-induced cortical mRNA and protein expression of IL-6 compared to the HSglx fractions. Notably, HPSE is known to induce cytokine release both directly and by HPSE mediated HS release. Increased HPSE activity releases the HS sequestered pro-inflammatory cytokines that can subsequently bind to their respective receptor (Goldberg et al., 2013; Gordts et al., 2014; Farrugia et al., 2018). These pro-inflammatory cytokines contribute to a disturbed glomerular endothelium and thereby albuminuria. HPSE-deficient mice show reduced cortical mRNA expression of pro-inflammatory cytokines (Garsen et al., 2016). Moreover, we recently showed that HPSE inhibition by heparanase-2 treatment resulted in attenuated glomerulonephritis accompanied with decreased cortical mRNA expression of IL-6 (Buijsers et al., 2023). Our observed effects of fucoidan or sulodexide in LPS-induced glomerulonephritis might thus partially be attributed to their HPSE-inhibiting capacity.
Additional beneficial effects of HS-mimetics in the development of inflammatory diseases were reported by others, which is in line with the reduced cytokine expression in glomerulonephritis by unstimulated HSglx, LPS HSglx, fucoidan and sulodexide in our study. For instance, LMWH has numerous anti-inflammatory properties and various mechanisms underlying the anti-inflammatory effect of LMWH have been proposed (Young, 2008; Ludwig, 2009; Mousavi et al., 2015). For example, LMWH can bind the majority of chemokines and cytokines and thereby is able to mediate the trafficking of leukocytes (Ramdin et al., 1998; Frevert et al., 2003; Buijsers et al., 2020b). The potential of LMWH as a therapeutic compound for inflammatory diseases has been supported by experimental models in bronchial asthma, ulcerative colitis, burns, ischemia-reperfusion, arthritis, and peritonitis (Wan et al., 2002; Young, 2008).
Similarly, fucoidan has been shown to have a protective effect on the kidneys by preventing fibrosis, inflammation, oxidative stress, and apoptosis (Carvalho et al., 2014; Zahan et al., 2022). The anti-inflammatory effect of fucoidan was demonstrated in recent studies, in which fucoidan significantly suppressed the secretion of pro-inflammatory mediators such as nitric oxide and the cytokines TNF-α and IL-1β upon LPS stimulation of macrophages (Jeong et al., 2017) and reduced the expression of proinflammatory cytokines including IL-6, TNF-α, and ICAM-1 in murine acute pancreatitis and streptozotocin-induced diabetes mellitus rat model (Carvalho et al., 2014; Aleissa et al., 2020; Zahan et al., 2022).
Fucoidan reduced the LPS-induced ICAM-1 and IL-6 mRNA expression of mGEnC-1, but no decrease of LPS-induced cytokine secretion was observed for PBMCs. This finding does not comply with previous studies, which demonstrated that fucoidan does affect the immune cells response. One study showed that the treatment of fucoidan reduced LPS-induced TNF-α, IL-1β, IL-6, and other pro-inflammatory cytokines in RAW264.7 mouse macrophages (Fernando et al., 2017). However, the fucoidan used in this study is extracted from Chnoospora minima whereas fucoidan from Laminaria japonica was used in our study. In addition, the dose of fucoidan at which an effect was detected, was five-to-ten times higher in the aforementioned study compared to our study. Another important difference is the use of human cells in our study compared to the murine study performed in RAW264.7 macrophage cell-line respectively. However, fucoidan was shown to reduce the responsiveness of human monocytes to LPS as well (Pozharitskaya et al., 2020).
Both cortical ICAM-1 and VCAM-1 mRNA expression were increased in LPS-induced glomerulonephritis in our study while the four treatments could only reduce the ICAM-1 mRNA expression and not the VCAM-1 mRNA expression. IF staining revealed that ICAM-1 was upregulated in the endothelial cells whereas VCAM-1 was upregulated in the mesangial cells, but not in the endothelial cells, upon LPS stimulation, which might explain the discrepancy in reduced VCAM-1 and ICAM-1 mRNA expression by the GAG treatments. Upregulation of VCAM-1 in mesangial cells due to LPS administration has been previously reported in a number of studies (Alpers et al., 1993; Khachigian et al., 1997; Lee et al., 2012). ICAM-1 protein expression was not decreased by any treatment. We hypothesize that this discrepancy between mRNA and protein expression might be due to kinetic differences in expression between the ICAM-1 mRNA and protein levels.
There are some limitations in our study. First, the LPS-induced glomerulonephritis model presented relatively mild, since there was no effect on kidney function. Second, we only used female mice in this study because female and male mice respond to LPS in different magnitude. Nevertheless, we induced experimental glomerulonephritis in other recent studies in male mice as well and no obvious discrepancies can be observed in disease manifestation between those studies and the current study (Garsen et al., 2016). Last, the route of administration of fucoidan and sulodexide can be discussed. Fucoidans and sulodexide are generally administered orally (Lauver and Lucchesi, 2006; Yung et al., 2013; Zahan et al., 2022) while we injected fucoidan or sulodexide intra-venously. However, there are some studies that administered fucoidan intra-peritoneal or sub-cutaneous (Zahan et al., 2022), and sulodexide intra-peritoneal and intra-venously (Borawski et al., 2007; Jo et al., 2014).
The in vivo effects of the treatments on glomerulonephritis were directed to different cell types; endothelial cells, podocytes and immune cells all seem to be involved. The observation that all treatments were able to alter cytokine and ICAM-1 mRNA expression levels in vivo but not in vitro suggest that the interplay between various cell types is crucial for the effect in experimental glomerulonephritis. Therefore, future research should focus on the interplay of these cells as can be performed in glomerulus on a chip models for instance.
In conclusion, we found that unstimulated HSglx, LPS HSglx, fucoidan and sulodexide display differential effects in experimental glomerulonephritis. These results contribute to a better understanding of the HS domains that are required to inhibit inflammatory processes and therefore take us one step closer towards defined HS oligosaccharides for the treatment of glomerular diseases.
Data availability statement
The raw data supporting the conclusion of this article will be made available by the authors, without undue reservation.
Ethics statement
The animal study was reviewed and approved by the Animal Ethical Committee of the Radboud University Nijmegen and the Dutch government.
Author contributions
BB, TN, and JV designed the experiments. BB, MJ, MB-B, MG, RS, and NP performed the experiments. MM-H and MG produced the HSglx fractions. BB, MJ, and MB-B analyzed the data. BB, TN, and JV wrote the manuscript. TN and JV supervised the study. JV secured funding. All authors contributed to the article and approved the submitted version.
Funding
BB was supported by the Radboud Institute of Molecular Life Sciences (RIMLS) PhD-program. The study was further supported by consortium grant LSHM16058-SGF (GLYCOTREAT; a collaboration project financed by the PPP allowance made available by Top Sector Life Sciences & Health to the Dutch Kidney Foundation to stimulate public-private partnerships).
Acknowledgments
The authors would like to thank Bob Long (MicroVascular Health Solutions LLC, Alpine, UT) for the kind gift of L. japonica fucoidan preparations and Enrique Poradosu (Keryx Biopharmaceuticals, Boston, United States) for the kind gift of Sulodexide.
Conflict of interest
The authors declare that the research was conducted in the absence of any commercial or financial relationships that could be construed as a potential conflict of interest.
Publisher’s note
All claims expressed in this article are solely those of the authors and do not necessarily represent those of their affiliated organizations, or those of the publisher, the editors and the reviewers. Any product that may be evaluated in this article, or claim that may be made by its manufacturer, is not guaranteed or endorsed by the publisher.
Supplementary material
The Supplementary Material for this article can be found online at: https://www.frontiersin.org/articles/10.3389/fmolb.2023.1223972/full#supplementary-material
SUPPLEMENTARY FIGURE S1 | No significant effects can be observed on plasma levels of TNF-α, IL-23, IL17A, IL27, and IL12 for LPS-induced glomerulonephritis in mice. (A). TNF-α, (B). IL-23, (C). IL17A, (D). IL27, and (E). IL12 plasma protein levels of mice injected with LPS (48 hours) and treated with unstimulated HSglx, LPS HSglx, fucoidan or sulodexide. Data are expressed as mean ± SEM. n ≥ 8. Unstimulated HSglx, HS extracted from unstimulated endothelial glycocalyx; LPS HSglx, HS extracted from LPS stimulated endothelial glycocalyx; TNF-α, tumor necrosis factor-alfa.
SUPPLEMENTARY FIGURE S2 | No significant effects can be observed on plasma levels of IL-1α, IL-1β, IFN-γ, IFN- β, and GM-CSF for LPS-induced glomerulonephritis in mice. (A). IL-1α, (B). IL-1β, (C). IFN-γ, (D). IFN-β, and (E). GM-CSF plasma protein levels of mice injected with LPS (48 h) and treated with unstimulated HSglx, LPS HSglx, fucoidan or sulodexide. Data are expressed as mean ± SEM. n≥8. Unstimulated HSglx, HS extracted from unstimulated endothelial glycocalyx; LPS HSglx, HS extracted from LPS stimulated endothelial glycocalyx; IFN, interferon; GM-CSF, Granulocyte-macrophage colony-stimulating factor.
SUPPLEMENTARY FIGURE S3 | Cortical mRNA expression of TNF-α is increased during LPS-induced glomerulonephritis in mice but is unaltered by unstimulated HSglx, LPS HSglx, fucoidan or sulodexide treatment whereas no effect on desmin and eNOS mRNA expression is observed in LPS-induced glomerulonephritis. (A). TNF-α, (B). desmin, and (C). eNOS cortical mRNA expression of mice injected with LPS (48 h) and treated with unstimulated HSglx, LPS HSglx, fucoidan or sulodexide. Data are expressed as mean ± SEM. *p<0.05. n ≥ 4. Unstimulated HSglx, HS extracted from unstimulated endothelial glycocalyx; LPS HSglx, HS extracted from LPS stimulated endothelial glycocalyx; TNF-α, tumor necrosis factor-alfa; eNOS, endothelial nitric oxide synthase.
SUPPLEMENTARY FIGURE S4 | Gating strategy for flow cytometry analysis. All cells were first gated to select only single cells (p5). Subsequently, live CD45+ cells were selected and further divided into lineage positive (NK cells, T cells, B cells, and erythrocytes) and lineage negative cells (granulocytes, monocytes, macrophages, and dendritic cells). The lineage negative cells were gated for the presence of Ly6G to isolate the neutrophils from the lineage negative population. Next, Ly6G negative cells were gated for CD11c and Ly6C to detect the monocytes.
SUPPLEMENTARY FIGURE S5 | ICAM-1, but not VCAM-1, colocalizes with the endothelial cell marker CD31. (A). Expression of ICAM-1 and the endothelial cell marker CD31 in control kidneys and kidneys of mice with LPS-induced glomerulonephritis. IF staining is presented on subsequent kidney sections, in which the same glomerulus was imaged because the ICAM-1 and CD31 antibodies could not be combined on a single kidney section. (B). Expression of VCAM-1 and CD31 in control kidneys and kidneys of mice with LPS-induced glomerulonephritis show that VCAM-1 is not upregulated in endothelial cells in LPS-induced glomerulonephritis. ICAM-1, intercellular adhesion molecule 1; VCAM-1, vascular cell adhesion molecule-1.
References
Aleissa, M. S., Alkahtani, S., Abd Eldaim, M. A., Ahmed, A. M., Bungău, S. G., Almutairi, B., et al. (2020). Fucoidan ameliorates oxidative stress, inflammation, DNA damage, and hepatorenal injuries in diabetic rats intoxicated with aflatoxin B(1). Oxid. Med. Cell Longev. 2020, 9316751. doi:10.1155/2020/9316751
Alpers, C. E., Hudkins, K. L., Davis, C. L., Marsh, C. L., Riches, W., McCarty, J. M., et al. (1993). Expression of vascular cell adhesion molecule-1 in kidney allograft rejection. Kidney Int. 44 (4), 805–816. doi:10.1038/ki.1993.315
Borawski, J., Dubowski, M., Pawlak, K., and Mysliwiec, M. (2007). Sulodexide induces hepatocyte growth factor release in humans. Eur. J. Pharmacol. 558 (1-3), 167–171. doi:10.1016/j.ejphar.2006.12.009
Brady, H. R. (1994). Leukocyte adhesion molecules and kidney diseases. Kidney Int. 45 (5), 1285–1300. doi:10.1038/ki.1994.169
Broekhuizen, L. N., Lemkes, B. A., Mooij, H. L., Meuwese, M. C., Verberne, H., Holleman, F., et al. (2010). Effect of sulodexide on endothelial glycocalyx and vascular permeability in patients with type 2 diabetes mellitus. Diabetologia 53 (12), 2646–2655. doi:10.1007/s00125-010-1910-x
Buijsers, B., Garsen, M., de Graaf, M., Bakker-van Bebber, M., Guo, C., Li, X., et al. (2023). Heparanase-2 protein and peptides have a protective effect on experimental glomerulonephritis and diabetic nephropathy. Front. Pharmacol. 14, 1098184. doi:10.3389/fphar.2023.1098184
Buijsers, B., Yanginlar, C., de Nooijer, A., Grondman, I., Maciej-Hulme, M. L., Jonkman, I., et al. (2020a). Increased plasma heparanase activity in COVID-19 patients. Front. Immunol. 11, 575047. doi:10.3389/fimmu.2020.575047
Buijsers, B., Yanginlar, C., Maciej-Hulme, M. L., de Mast, Q., and van der Vlag, J. (2020b). Beneficial non-anticoagulant mechanisms underlying heparin treatment of COVID-19 patients. EBioMedicine 59, 102969. doi:10.1016/j.ebiom.2020.102969
Carter, N. M., Ali, S., and Kirby, J. A. (2003). Endothelial inflammation: The role of differential expression of N-deacetylase/N-sulphotransferase enzymes in alteration of the immunological properties of heparan sulphate. J. Cell Sci. 116, 3591–3600. doi:10.1242/jcs.00662
Carvalho, A. C., Sousa, R. B., Franco Á, X., Costa, J. V., Neves, L. M., Ribeiro, R. A., et al. (2014). Protective effects of fucoidan, a P- and L-selectin inhibitor, in murine acute pancreatitis. Pancreas 43 (1), 82–87. doi:10.1097/MPA.0b013e3182a63b9d
Duffield, J. S. (2010). Macrophages and immunologic inflammation of the kidney. Semin. Nephrol. 30 (3), 234–254. doi:10.1016/j.semnephrol.2010.03.003
Farrugia, B. L., Lord, M. S., Melrose, J., and Whitelock, J. M. (2018). The role of heparan sulfate in inflammation, and the development of biomimetics as anti-inflammatory strategies. J. Histochem Cytochem 66 (4), 321–336. doi:10.1369/0022155417740881
Fernando, I. P. S., Sanjeewa, K. K. A., Samarakoon, K. W., Lee, W. W., Kim, H. S., Kang, N., et al. (2017). A fucoidan fraction purified from Chnoospora minima; a potential inhibitor of LPS-induced inflammatory responses. Int. J. Biol. Macromol. 104, 1185–1193. doi:10.1016/j.ijbiomac.2017.07.031
Frevert, C. W., Kinsella, M. G., Vathanaprida, C., Goodman, R. B., Baskin, D. G., Proudfoot, A., et al. (2003). Binding of interleukin-8 to heparan sulfate and chondroitin sulfate in lung tissue. Am. J. Respir. Cell Mol. Biol. 28 (4), 464–472. doi:10.1165/rcmb.2002-0084OC
Fridén, V., Oveland, E., Tenstad, O., Ebefors, K., Nyström, J., Nilsson, U. A., et al. (2011). The glomerular endothelial cell coat is essential for glomerular filtration. Kidney Int. 79 (12), 1322–1330. doi:10.1038/ki.2011.58
Garsen, M., Benner, M., Dijkman, H. B., van Kuppevelt, T. H., Li, J. P., Rabelink, T. J., et al. (2016). Heparanase is essential for the development of acute experimental glomerulonephritis. Am. J. Pathol. 186 (4), 805–815. doi:10.1016/j.ajpath.2015.12.008
Garsen, M., Rops, A. L., Rabelink, T. J., Berden, J. H., and van der Vlag, J. (2014). The role of heparanase and the endothelial glycocalyx in the development of proteinuria. Nephrol. Dial. Transpl. 29 (1), 49–55. doi:10.1093/ndt/gft410
Gil, N., Goldberg, R., Neuman, T., Garsen, M., Zcharia, E., Rubinstein, A. M., et al. (2012). Heparanase is essential for the development of diabetic nephropathy in mice. Diabetes 61 (1), 208–216. doi:10.2337/db11-1024
Goldberg, R., Meirovitz, A., Hirshoren, N., Bulvik, R., Binder, A., Rubinstein, A. M., et al. (2013). Versatile role of heparanase in inflammation. Matrix Biol. 32 (5), 234–240. doi:10.1016/j.matbio.2013.02.008
Gordts, P., Foley, E. M., Lawrence, R., Sinha, R., Lameda-Diaz, C., Deng, L., et al. (2014). Reducing macrophage proteoglycan sulfation increases atherosclerosis and obesity through enhanced type I interferon signaling. Cell Metab. 20 (5), 813–826. doi:10.1016/j.cmet.2014.09.016
Gross, C. M., Rafikov, R., Kumar, S., Aggarwal, S., Ham, P. B., Meadows, M. L., et al. (2015). Endothelial nitric oxide synthase deficient mice are protected from lipopolysaccharide induced acute lung injury. PLoS One 10 (3), e0119918. doi:10.1371/journal.pone.0119918
Guimond, S. E., Puvirajesinghe, T. M., Skidmore, M. A., Kalus, I., Dierks, T., Yates, E. A., et al. (2009). Rapid purification and high sensitivity analysis of heparan sulfate from cells and tissues TOWARD GLYCOMICS PROFILING. J. Biol. Chem. 284 (38), 25714–25722. doi:10.1074/jbc.M109.032755
Hill, P. A., Lan, H. Y., Nikolic-Paterson, D. J., and Atkins, R. C. (1994). ICAM-1 directs migration and localization of interstitial leukocytes in experimental glomerulonephritis. Kidney Int. 45 (1), 32–42. doi:10.1038/ki.1994.4
Jeong, J-W., Hwang, S. J., Han, M. H., Lee, D-S., Yoo, J. S., Choi, I-W., et al. (2017). Fucoidan inhibits lipopolysaccharide-induced inflammatory responses in RAW 264.7 macrophages and zebrafish larvae. Mol. Cell. Toxicol. 13 (4), 405–417. doi:10.1007/s13273-017-0045-2
Jo, H., Jung, S. H., Kang, J., Yim, H. B., and Kang, K. D. (2014). Sulodexide inhibits retinal neovascularization in a mouse model of oxygen-induced retinopathy. BMB Rep. 47 (11), 637–642. doi:10.5483/bmbrep.2014.47.11.009
Khachigian, L. M., Collins, T., and Fries, J. W. (1997). N-acetyl cysteine blocks mesangial VCAM-1 and NF-kappa B expression in vivo. Am. J. Pathol. 151 (5), 1225–1229.
Kreuger, J., and Kjellén, L. (2012). Heparan sulfate biosynthesis: Regulation and variability. J. Histochem Cytochem 60 (12), 898–907. doi:10.1369/0022155412464972
Lauver, D. A., and Lucchesi, B. R. (2006). Sulodexide: A renewed interest in this glycosaminoglycan. Cardiovasc Drug Rev. 24 (3-4), 214–226. doi:10.1111/j.1527-3466.2006.00214.x
Lee, I. T., Shih, R. H., Lin, C. C., Chen, J. T., and Yang, C. M. (2012). Role of TLR4/NADPH oxidase/ROS-activated p38 MAPK in VCAM-1 expression induced by lipopolysaccharide in human renal mesangial cells. Cell Commun. Signal 10 (1), 33. doi:10.1186/1478-811X-10-33
Lewis, E. J., Lewis, J. B., Greene, T., Hunsicker, L. G., Berl, T., Pohl, M. A., et al. (2011). Sulodexide for kidney protection in type 2 diabetes patients with microalbuminuria: A randomized controlled trial. Am. J. Kidney Dis. 58 (5), 729–736. doi:10.1053/j.ajkd.2011.06.020
Lindahl, U., and Kjellen, L. (2013). Pathophysiology of heparan sulphate: Many diseases, few drugs. J. Intern Med. 273 (6), 555–571. doi:10.1111/joim.12061
Ludwig, R. J. (2009). Therapeutic use of heparin beyond anticoagulation. Curr. Drug Discov. Technol. 6 (4), 281–289. doi:10.2174/157016309789869001
Luimula, P., Ahola, H., Wang, S. X., Solin, M. L., Aaltonen, P., Tikkanen, I., et al. (2000). Nephrin in experimental glomerular disease. Kidney Int. 58 (4), 1461–1468. doi:10.1046/j.1523-1755.2000.00308.x
Maciej-Hulme, M. L., Leprince, A., Lavin, A., Guimond, S., Turnbull, J., Pelletier, J., et al. (2020). High sensitivity (zeptomole) detection of BODIPY heparan sulfate (HS) disaccharides by ion-paired RP-HPLC and LIF detection enables analysis of HS from mosquito midguts. BioRxiv.
Maciej-Hulme, M. L., Skidmore, M. A., and Price, H. P. (2018). The role of heparan sulfate in host macrophage infection by Leishmania species. Biochem. Soc. Trans. 46 (4), 789–796. doi:10.1042/BST20170398
Masola, V., Bellin, G., Vischini, G., Dall'Olmo, L., Granata, S., Gambaro, G., et al. (2018). Inhibition of heparanase protects against chronic kidney dysfunction following ischemia/reperfusion injury. Oncotarget 9 (90), 36185–36201. doi:10.18632/oncotarget.26324
Masola, V., Onisto, M., Zaza, G., Lupo, A., and Gambaro, G. (2012). A new mechanism of action of sulodexide in diabetic nephropathy: Inhibits heparanase-1 and prevents FGF-2-induced renal epithelial-mesenchymal transition. J. Transl. Med. 10, 213. doi:10.1186/1479-5876-10-213
Mousavi, S., Moradi, M., Khorshidahmad, T., and Motamedi, M. (2015). Anti-inflammatory effects of heparin and its derivatives: A systematic review. Adv. Pharmacol. Sci. 2015, 507151. doi:10.1155/2015/507151
Muralidar, S., Gopal, G., and Visaga Ambi, S. (2021). Targeting the viral-entry facilitators of SARS-CoV-2 as a therapeutic strategy in COVID-19. J. Med. Virol. 93, 5260–5276. doi:10.1002/jmv.27019
Packham, D. K., Wolfe, R., Reutens, A. T., Berl, T., Heerspink, H. L., Rohde, R., et al. (2012). Sulodexide fails to demonstrate renoprotection in overt type 2 diabetic nephropathy. J. Am. Soc. Nephrol. 23 (1), 123–130. doi:10.1681/ASN.2011040378
Poplawska, A., Szelachowska, M., Topolska, J., Wysocka-Solowie, B., and Kinalska, I. (1997). Effect of glycosaminoglycans on urinary albumin excretion in insulin-dependent diabetic patients with micro- or macroalbuminuria. Diabetes Res. Clin. Pract. 38 (2), 109–114. doi:10.1016/s0168-8227(97)00096-x
Pozharitskaya, O. N., Obluchinskaya, E. D., and Shikov, A. N. (2020). Mechanisms of bioactivities of fucoidan from the Brown seaweed fucus vesiculosus L. Of the barents sea. Mar. Drugs 18 (5), 275. doi:10.3390/md18050275
Raats, C. J. I., Bakker, M. A. H., Hoch, W., Tamboer, W. P. M., Groffen, A. J. A., van den Heuvel, L. P. W. J., et al. (1998). Differential expression of agrin in renal basement membranes as revealed by domain-specific antibodies. J. Biol. Chem. 273 (28), 17832–17838. doi:10.1074/jbc.273.28.17832
Rabelink, T. J., van den Berg, B. M., Garsen, M., Wang, G., Elkin, M., and van der Vlag, J. (2017). Heparanase: Roles in cell survival, extracellular matrix remodelling and the development of kidney disease. Nat. Rev. Nephrol. 13 (4), 201–212. doi:10.1038/nrneph.2017.6
Ramdin, L., Perks, B., Sheron, N., and Shute, J. K. (1998). Regulation of interleukin-8 binding and function by heparin and alpha2-macroglobulin. Clin. Exp. Allergy 28 (5), 616–624. doi:10.1046/j.1365-2222.1998.00283.x
Ritchie, J. P., Ramani, V. C., Ren, Y., Naggi, A., Torri, G., Casu, B., et al. (2011). SST0001, a chemically modified heparin, inhibits myeloma growth and angiogenesis via disruption of the heparanase/syndecan-1 axis. Clin. Cancer Res. 17 (6), 1382–1393. doi:10.1158/1078-0432.CCR-10-2476
Rops, A. L., Jacobs, C. W., Linssen, P. C., Boezeman, J. B., Lensen, J. F., Wijnhoven, T. J., et al. (2007a). Heparan sulfate on activated glomerular endothelial cells and exogenous heparinoids influence the rolling and adhesion of leucocytes. Nephrol. Dial. Transpl. 22 (4), 1070–1077. doi:10.1093/ndt/gfl801
Rops, A. L., van den Hoven, M. J., Bakker, M. A., Lensen, J. F., Wijnhoven, T. J., van den Heuvel, L. P., et al. (2007b). Expression of glomerular heparan sulphate domains in murine and human lupus nephritis. Nephrol. Dial. Transpl. 22 (7), 1891–1902. doi:10.1093/ndt/gfm194
Rops, A. L., van den Hoven, M. J., Baselmans, M. M., Lensen, J. F., Wijnhoven, T. J., van den Heuvel, L. P., et al. (2008). Heparan sulfate domains on cultured activated glomerular endothelial cells mediate leukocyte trafficking. Kidney Int. 73 (1), 52–62. doi:10.1038/sj.ki.5002573
Rops, A. L., van der Vlag, J., Jacobs, C. W., Dijkman, H. B., Lensen, J. F., Wijnhoven, T. J., et al. (2004b). Isolation and characterization of conditionally immortalized mouse glomerular endothelial cell lines. Kidney Int. 66 (6), 2193–2201. doi:10.1111/j.1523-1755.2004.66009.x
Rops, A. L., van der Vlag, J., Lensen, J. F., Wijnhoven, T. J., van den Heuvel, L. P., van Kuppevelt, T. H., et al. (2004a). Heparan sulfate proteoglycans in glomerular inflammation. Kidney Int. 65 (3), 768–785. doi:10.1111/j.1523-1755.2004.00451.x
Saphire, A. C., Bobardt, M. D., Zhang, Z., David, G., and Gallay, P. A. (2001). Syndecans serve as attachment receptors for human immunodeficiency virus type 1 on macrophages. J. Virol. 75 (19), 9187–9200. doi:10.1128/JVI.75.19.9187-9200.2001
Tecklenborg, J., Clayton, D., Siebert, S., and Coley, S. M. (2018). The role of the immune system in kidney disease. Clin. Exp. Immunol. 192 (2), 142–150. doi:10.1111/cei.13119
van der Vlag, J., and Buijsers, B. (2020). Heparanase in kidney disease. Adv. Exp. Med. Biol. 1221, 647–667. doi:10.1007/978-3-030-34521-1_26
van Gemst, J. J., Passmann, N., Rops, A., van Kuppevelt, T. H., Berden, J. H., Loeven, M. A., et al. (2021). Blocking of inflammatory heparan sulfate domains by specific antibodies is not protective in experimental glomerulonephritis. PLoS One 16 (12), e0261722. doi:10.1371/journal.pone.0261722
van Weelden, G., Bobiński, M., Okła, K., van Weelden, W. J., Romano, A., and Pijnenborg, J. M. A. (2019). Fucoidan structure and activity in relation to anti-cancer mechanisms. Mar. Drugs 17 (1), 32. doi:10.3390/md17010032
Verma, R., Venkatareddy, M., Kalinowski, A., Li, T., Kukla, J., Mollin, A., et al. (2018). Nephrin is necessary for podocyte recovery following injury in an adult mature glomerulus. PLoS One 13 (6), e0198013. doi:10.1371/journal.pone.0198013
Wan, M. X., Liu, Q., Wang, Y., and Thorlacius, H. (2002). Protective effect of low molecular weight heparin on experimental colitis: Role of neutrophil recruitment and TNF-alpha production. Inflamm. Res. 51 (4), 182–187. doi:10.1007/pl00000290
Wang, J., Zhang, Q., Zhang, Z., Zhang, H., and Niu, X. (2010). Structural studies on a novel fucogalactan sulfate extracted from the Brown seaweed Laminaria japonica. Int. J. Biol. Macromol. 47 (2), 126–131. doi:10.1016/j.ijbiomac.2010.05.010
Wang, L., Fuster, M., Sriramarao, P., and Esko, J. D. (2005). Endothelial heparan sulfate deficiency impairs L-selectin- and chemokine-mediated neutrophil trafficking during inflammatory responses. Nat. Immunol. 6 (9), 902–910. doi:10.1038/ni1233
Wang, M. Z., Wang, J., Cao, D. W., Tu, Y., Liu, B. H., Yuan, C. C., et al. (2022). Fucoidan alleviates renal fibrosis in diabetic kidney disease via inhibition of NLRP3 inflammasome-mediated podocyte pyroptosis. Front. Pharmacol. 13, 790937. doi:10.3389/fphar.2022.790937
Young, E. (2008). The anti-inflammatory effects of heparin and related compounds. Thromb. Res. 122 (6), 743–752. doi:10.1016/j.thromres.2006.10.026
Yuan, L., Cheng, S., Sol, W., van der Velden, A. I. M., Vink, H., Rabelink, T. J., et al. (2022). Heparan sulfate mimetic fucoidan restores the endothelial glycocalyx and protects against dysfunction induced by serum of COVID-19 patients in the intensive care unit. ERJ Open Res. 8 (2), 00652-02021. doi:10.1183/23120541.00652-2021
Yung, S., Chau, M. K., Zhang, Q., Zhang, C. Z., and Chan, T. M. (2013). Sulodexide decreases albuminuria and regulates matrix protein accumulation in C57BL/6 mice with streptozotocin-induced type I diabetic nephropathy. PLoS One 8 (1), e54501. doi:10.1371/journal.pone.0054501
Keywords: glycosaminoglycans, heparan sulfate, fucoidan, sulodexide, endothelial glycocalyx, heparanase-1, proteinuria, glomerulonephritis
Citation: Buijsers B, Maciej-Hulme M, Jacobs M, Bebber MB-v, de Graaf M, Salmenov R, Parr N, Rabelink TJ, Nijenhuis T and van der Vlag J (2023) Glycosaminoglycans and fucoidan have a protective effect on experimental glomerulonephritis. Front. Mol. Biosci. 10:1223972. doi: 10.3389/fmolb.2023.1223972
Received: 16 May 2023; Accepted: 26 June 2023;
Published: 05 July 2023.
Edited by:
Chunyu Wang, Rensselaer Polytechnic Institute, United StatesReviewed by:
Kazuma Sakamoto, Nagoya University, JapanXinyue Liu, Harvard Medical School, United States
Copyright © 2023 Buijsers, Maciej-Hulme, Jacobs, Bebber, de Graaf, Salmenov, Parr, Rabelink, Nijenhuis and van der Vlag. This is an open-access article distributed under the terms of the Creative Commons Attribution License (CC BY). The use, distribution or reproduction in other forums is permitted, provided the original author(s) and the copyright owner(s) are credited and that the original publication in this journal is cited, in accordance with accepted academic practice. No use, distribution or reproduction is permitted which does not comply with these terms.
*Correspondence: Johan van der Vlag, Sm9oYW4udmFuZGVyVmxhZ0ByYWRib3VkdW1jLm5s