- 1Department of General Surgery, Xiangya Hospital Central South University, Changsha, China
- 2Xiangya School of Medicine, Central South University, Changsha, China
- 3Department of Pathology, Xiang-ya Hospital, Central South University, Changsha, China
- 4Key Laboratory of Hunan Province in Neurodegenerative Disorders, Xiangya Hospital, Central South University, Changsha, China
- 5National Clinical Research Center for Geriatric Disorders, Xiangya Hospital, Central South University, Changsha, China
The tumor microenvironment (TME) is an intricate ecosystem that is actively involved in various stages of cancer occurrence and development. Some characteristics of tumor biological behavior, such as proliferation, migration, invasion, inhibition of apoptosis, immune escape, angiogenesis, and metabolic reprogramming, are affected by TME. Studies have shown that non-coding RNAs, especially long-chain non-coding RNAs and microRNAs in cancer-derived exosomes, facilitate intercellular communication as a mechanism for regulating angiogenesis. They stimulate tumor growth, as well as angiogenesis, metastasis, and reprogramming of the TME. Exploring the relationship between exogenous non-coding RNAs and tumor-associated endothelial cells, as well as their role in angiogenesis, clinicians will gain new insights into treatment as a result.
1 Introduction
The tumor microenvironment (TME), an intricate ecosystem, actively participates in every stage of cancer development (Hanahan and Weinberg, 2011; Yang et al., 2020). As a dynamic ecosystem containing a variety of cell types and non-cellular components, TME plays a major role in tumor growth, metastasis, and drug resistance. Cancers exhibit some biological behaviors, such as proliferation, migration, invasion, immune escape, angiogenesis, and metabolic reprogramming, all of which are affected by TME. Biological functions, including autocrine and paracrine functions, are regulated by the complex communication network within TMEs. Exocrine-mediated communication is an important emerging pathway of paracrine signal transduction (Giraldo et al., 2019). Exosomes can carry molecules such as DNA, RNA, and proteins to adjacent cells, where they act as effective signaling molecules between cancer cells and surrounding cells constituting TME. Nontumor cells in TME, such as fibroblasts, endothelial cells (ECs), and immune cells, are affected by tumor-associated active substances and their original cell functions undergo tumor-like changes, constantly adapting to the new environment and promoting tumor growth. The TME is composed of different cell types with various functions, which regulates excessive cell-cell interactions. These interactions orchestrate reprogramming to the environment allowed by each cancer and may have a significant impact on cancer development, progression, and treatment resistance.
ECs are involved in tumor growth, tumor-induced angiogenesis, and vascular secretory functions for self-renewal and differentiation after trauma and thrombosis (Barachini et al., 2023). Angiogenesis plays an important role in all stages of cancer development (Aguilar-Cazares et al., 2019). Angiogenesis is a complex process of growing new capillaries from preexisting blood vessels, typically involving the following steps: stimulation of ECs with vascular endothelial growth factor (VEGF), proliferation, migration, and differentiation of vascular ECs, vessel branches and vessel formation (Ahir et al., 2020; Yang et al., 2022). Tumor vascular growth is a key factor in cancer progression, which is closely related to metastasis and a poor prognosis. Tumor angiogenesis is a recognized target for anticancer therapy by targeting growth factors, their cell surface receptors, and associated signaling pathways. Tissue hypoxia induces an overproduction of VEGF, leading to an imbalance between pro-angiogenic factors and anti-angiogenic factors, causing excessive abnormal angiogenesis that plays a central role in tumor progression (Jászai and Schmidt, 2019). The supply of energy and the removal of waste products are key factors in the development of cancer cells (Anderson and Simon, 2020). Tumor cells can communicate with adjacent tissues through the release of exosomes (Stec et al., 2015; Dominiak et al., 2020). Exosomes contain a variety of substances that promote angiogenesis and thus accelerate cancer invasion and metastasis (Głuszko et al., 2019), and the release of some exosomes also affects immune function (Aslan et al., 2019). Evidence suggests that non-coding RNAs (ncRNAs), especially long-chain non-coding RNAs (lncRNAs) and microRNAs (miRNAs) in cancer-derived exosomes, play an important role in regulating angiogenesis by facilitating intercellular communication, which in turn stimulates tumor growth, as well as angiogenesis, metastasis, and reprogramming of TME (shown in Figure 1) (Zhao et al., 2020).
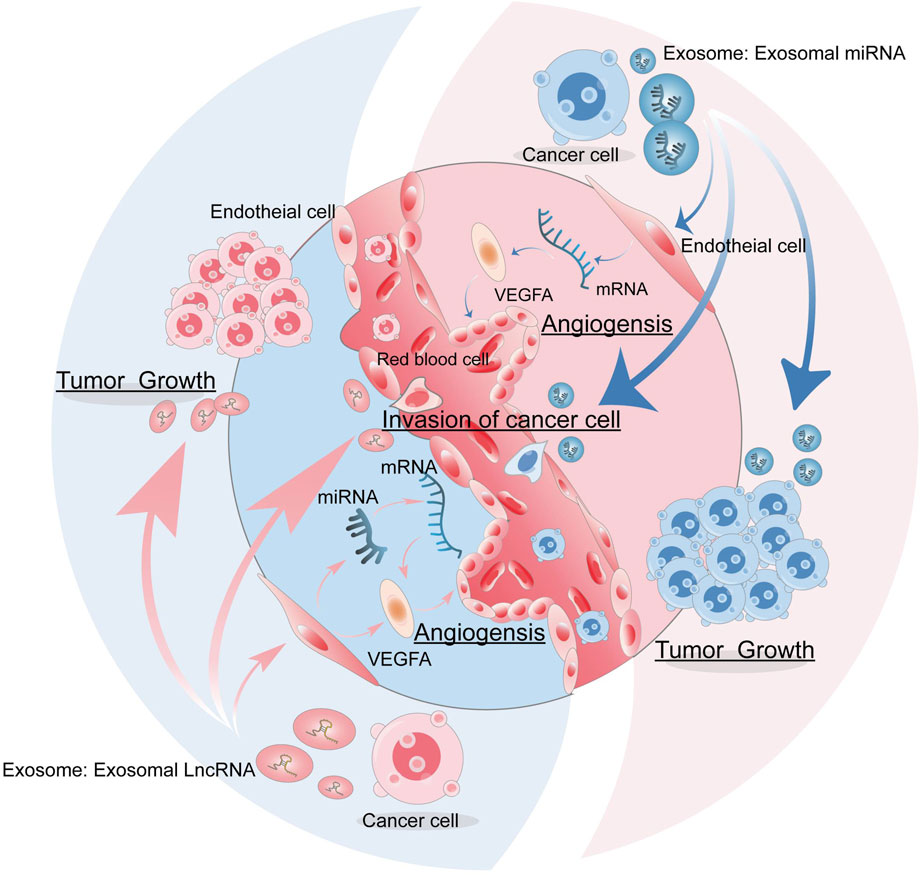
FIGURE 1. The role of exosomes in tumor angiogenesis. Tumor cells can release exosomes, which carry miRNA and lncRNAs that can act on endothelial cells to promote tumor angiogenesis, as well as stimulate tumor growth, invasion, and metastasis.
In hypoxic environments, hypoxia can induce overexpression of ncRNA, which is released by exosomes and participates in tumor angiogenesis by reacting with ECs and other angiogenic cells, thus affecting tumor progression (He et al., 2022; Jia et al., 2022; Yang et al., 2022). In addition to ECs, there are many other remaining cell-derived exosomes in TME that can also promote angiogenesis and thus help tumor cell metastasis. For example, exosomal ncRNAs released by tumor cells regulate ECs and promote or inhibit angiogenesis (Ahmadi and Rezaie, 2020). Tumor-associated macrophage (TAM)-derived exosomal ncRNAs regulate tumor cells and promote angiogenesis (Xu et al., 2022). Stem cells-derived exosomal ncRNAs regulate tumor cells and inhibit tumor angiogenesis (Yang and Teng, 2023). Tumor-derived exosomes (TEXs) of lung cancer cells can transfer miR-21 to ECs in vitro and stimulate ECs angiogenesis to increase VEGF expression and secretion, thus helping to invade and metastasize lung cancer cells (Forder et al., 2021). The overexpression of exosome-derived miR-16 and miR-100 from mesenchymal stem cells downregulates VEGF expression in breast cancer cells, thus inhibiting angiogenesis and tumor growth in vivo and in vitro (Soheilifar et al., 2022). In hepatoma cells, cancer stem cells upregulate VEGF by delivering overexpressed lncRNAH19 to ECs to promote angiogenesis and tumor growth (Yao et al., 2023). TAM-derived exosomes are enriched with miR-501-3p, which enhances the metastatic capacity of pancreatic ductal adenocarcinoma (PDAC) cells (Yin et al., 2019; Cocks et al., 2022). Blood vessel formation is inseparable from the role of ECs, and the relationship between exosomal miRNAs and lncRNAs and endothelial cells in TME is the focus of this review. By summarizing their relationship to explore the role of exogenous ncRNAs in tumor-associated endothelial cells and also their specific role in angiogenesis, clinicians will be able to gain new insights in cancer treatment.
2 Important position of exosomes
Previous studies have shown that a series of growth factors, cell surface receptors, and a large number of signaling molecules drive remodeling of the blood and lymphatic system in cancer (Stacker et al., 2014; Fares et al., 2020; Arcucci et al., 2021a). Recent studies have identified important roles for ncRNAs in the regulation of key aspects of cancer biology, including tumor angiogenesis and lymphangiogenesis. NcRNAs are a class of RNA molecules that do not encode proteins (Zampetaki et al., 2018), of which miRNA is the most studied, which along with lncRNA is the main focus of this review. miRNAs are small RNA molecules that mediate post-transcriptional regulation by targeting mRNAs, thereby resulting in the reduction of gene expression through mRNA degradation and/or translational repression. Nuclear miRNAs have been shown to play a role in transcriptional regulation through the recruitment of transcriptional activators and chromatin remodeling proteins of repressors (Bartel, 2009; Liu et al., 2018). It should be noted that different miRNAs can work together to focus on the expression of the same or multiple genes in related molecular pathways (Uhlmann et al., 2012). LncRNA exhibits a series of different regulatory functions in different cell compartments (Zampetaki et al., 2018). LncRNA plays a role in transcriptional regulation by binding chromatin remodeling proteins and recruiting transcription factors, activators, and inhibitors (Man et al., 2018).
Nuclear miRNAs can affect transcription by active ting or silencing of transcribed genes (Liu et al., 2018), and miRNAs participate in post-transcriptional processes by regulating mRNA. For example, miR-29-b regulates the expression of VEGFA and Akt3 by negatively inhibiting angiogenesis (Chen et al., 2017; Li et al., 2017). LncRNA Hotair can promote angiogenesis by directly activating the transcription of VEGFA genes (Fu et al., 2016). LncRNA can influence the cell cycle by regulating mRNAs. For example, lncRNA MALAT1 can regulate the variable splicing of the carcinogenic transcription factor B-MYB in endothelial cells (Tripathi et al., 2013), WTAPP1 lncRNA promotes migration by increasing the expression of matrix metalloproteinase MMP1 (Li et al., 2018), and tie-1As lncRNA selectively binds and degrades tie-1 mRNA, leading to specific defects in cell connection and tube formation (Li et al., 2010). Furthermore, lncRNA H19 regulates the biological behaviors of endothelial cells by suppressing miR-29a, thus inhibiting angiogenesis (Jia et al., 2016). LncRNAs facilitate epigenetic control of gene expression by recruiting transcription activators or inhibitors (Lam et al., 2013; Melo et al., 2013) or chromatin remodeling proteins as transcription regulators (Creamer and Lawrence, 2017). After gene transcription, LncRNAs can also be regulated, mainly by regulating mRNA splicing (Gong and Maquat, 2011), or by eliciting proteins that degrade mRNAs (Hutchinson et al., 2007) or acting as bait for proteins involved in mRNA degradation (Lee et al., 2016). LncRNAs can regulate various cancer-associated mRNAs by competitively sponging various miRNAs, and thus participate in relevant signaling pathways (Zhong et al., 2019). It is worth emphasizing that both miRNAs and lncRNAs can regulate the gene expression in complex biological responses: miRNAs regulate gene expression of proteins associated with their related molecular pathways by targeting mRNAs, and in addition, miRNAs can collaborate with other molecules to precisely mediate gene silencing. LncRNAs regulate gene expression by controlling chromatin remodeling, or by targeting miRNAs regulate gene expression by controlling chromatin remodeling or by targeting miRNAs (Guo et al., 2020; Mao et al., 2020).
2.1 The relationship between exosomal miRNAs and endothelial cells
Endothelial cells can form vascular systems to transport nutrients and metabolites, which can help tumor proliferation, invasion, and metastasis. Crosstalk stimulation between tumor cells and endothelial cells can promote the growth of both, improve tumor malignancy, and even develop resistance to treatment (Shweiki et al., 1992; Carmeliet and Jain, 2011). Tumor cells and certain immune cell subsets can promote angiogenesis by expressing and secreting growth factors or inducing hypoxia (Ding et al., 2014; Zhou et al., 2014), resulting in leakage of vascular structures that promote angiogenesis and metastatic spread of tumor cells. MiRNAs are endogenous ncRNAs consisting of 21–25 nucleotides that promote post-transcriptional regulation of target genes mainly by binding to the 3′untranslated region (UTR) of mRNAs. Meanwhile, miRNAs regulate more than 30% of gene expression in the body, and their functions are closely related to cell proliferation, differentiation, apoptosis, embryonic development, tissue and organ formation, as well as the occurrence and development of various diseases (Bartel, 2004). Recent studies have shown that exosome-mediated miRNAs transfer from cancer cells to endothelial cells, contributing to the breakdown of the endothelial cell barrier and allowing cancer cells to spread and metastasize to distant locations, such as cell-derived exosomal miR-27b-3p in colorectal cancer (Zhou et al., 2014; Dou et al., 2021). Furthermore, miRNA-containing exosomes from leukemia cells, such as miR-17-92, play an important role in communication between tumor and endothelial cells, thus regulating the process of tumor angiogenesis (Umezu et al., 2013).
Exosomal miRNAs can regulate the migration of tumor endothelial cells and the formation of lymphatic and blood vessels (Table 1; Figure 2). Within tumors, most exosomal miRNAs are thought to be produced by tumor cells (Huang et al., 2022). When internalized by endothelial cells, some of these miRNAs can stimulate angiogenesis or lymphangiogenesis by inhibiting the expression of proteins that inhibit the main pathways driving these processes (Duan et al., 2019; Kim et al., 2020; Masoumi-Dehghi et al., 2020). Exosomal miRNAs have been shown to downregulate several anti-angiogenic transcription factors in endothelial cells or inhibit the expression of VEGFA, a key inducer of angiogenesis, thus turning on the angiogenic switch (Li J. et al., 2020). For example, in gastric cancer, exosomal miR-130a and miR-155 secreted by gastric cancer cells can inhibit the expression of the transcription factor c-MYB, indirectly promoting the expression of VEGFA (Arcucci et al., 2021b), which promotes angiogenesis and further assists in invasion and metastasis of gastric cancer cells.
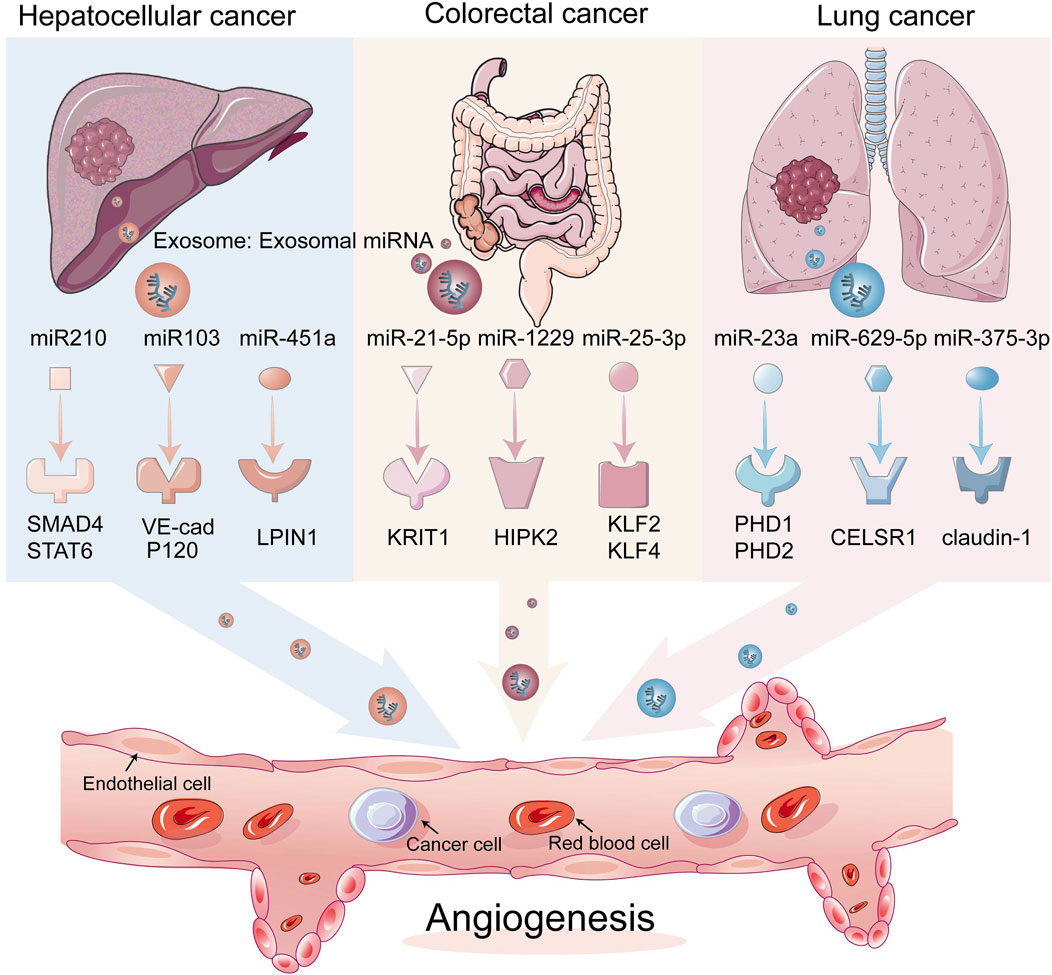
FIGURE 2. Exosomal miRNAs acting on endothelial cells affect tumor angiogenesis. Exosomal miRNAs can translocate from tumor cells to endothelial cells, which in turn acts on angiogenesis-related transcription factors, thereby stimulating angiogenesis.
2.1.1 Hepatocellular carcinoma cells
Current studies have shown that in hepatocellular carcinoma (HCC), exosomal miR-210 secreted by HCC cells can be transferred to endothelial cells, thus promoting tumor angiogenesis by targeting SMAD4 and STAT6 (Lin X. J. et al., 2018). miR-1290 targeting SMEK1 promotes angiogenesis of hepatocellular carcinoma, and miR-451a targeting LPIN1 suppresses hepatocellular tumorigenesis by regulating tumor cell apoptosis and angiogenesis (Zhao et al., 2019; Wang Q. et al., 2021). HANR is responsible for lymphangiogenesis in HCC cells via the exosomal miR-296 and the EAG1/VEGF axis (Shi et al., 2019). miR-103 was delivered to ECs through exosomes and then attenuated the integrity of the endothelial junction by directly inhibiting the expression of VE-Cadherin (VE-Cad) (Fang et al., 2018). miR-638 can promote vascular permeability by downregulating endothelial expression of VE-Cad and ZO-1 (Yokota et al., 2021). Exosomal miR-200b-3p from hepatocytes inhibited endothelial ERG expression, while reduction of miR-200b-3p in cancer cells promoted angiogenesis in HCC tissues by improving endothelial ERG expression (Moh-Moh-Aung et al., 2020).
2.1.2 Colorectal cancer cells
The exosome miR-21-5p can be delivered from colon cancer cells to endothelial cells, targeting KRIT1 and thus inducing angiogenesis and vascular permeability, as can the exosome miR-25-3p, which also transfers to ECs and promotes CRC metastasis by targeting KLF2 and KLF4 to regulate growth factors in endothelial cells. Furthermore, there is miR-1229 that promotes angiogenesis by targeting HIPK2 (Zeng et al., 2018; Hu et al., 2019; He Q. et al., 2021). miR-27b-3p is transferred by EMT-CRC cells into the exosomes of human umbilical vein endothelial cells (HUVEC), weakening the vascular barrier (Dou et al., 2021).
2.1.3 Lung cancer cells
miR-23a directly inhibits its targets, prolyl hydroxylases 1 and 2 (PHD1 and 2), in exosomes from lung cancer cells, resulting in the accumulation of hypoxia-inducible factor 1 alpha (HIF-1α) in endothelial cells. Finally, hypoxic lung cancer cells enhanced angiogenesis through hypoxic cancer-derived exosomes under normoxic and hypoxic conditions (Hsu et al., 2017). For lung adenocarcinoma (LUAD), miRNAs affect cancer cells and ECs bidirectionally; for example, miR-629-5p in lung adenocarcinoma transfers to endothelial cells, and by inhibiting CELSR1, which is lower in endothelial cells in invasive LUAD (a miR-30a-5p, a non-canonical cadherin, increases endothelial monolayer permeability, while overexpression of miR-30a-5p in endothelial cells inhibited tumor development (Li et al., 2020b; Tao et al., 2021). The exosome miR-141 is transported into HUVEC cells and targets KLF12 to promote angiogenesis in small cell lung cancer (SCLC), and miR-375-3p destroys vascular endothelial cells by directly binding to the 3′UTR of the tight junction protein CLDN1 and negatively regulating its expression tight junctions (Mao et al., 2020; Mao et al., 2021). miR-486-5p in non-small cell lung cancer (NSCLC) targets the CADM1/tight junction axis in vascular endothelial cells to promote metastasis of non-small cell lung cancer cells (Sun et al., 2021).
2.2 The relationship between exosomal lncRNAs and endothelial cells
LncRNAs are a diverse class of transcribed RNA molecules that are more than 200 nucleotides llong and have limited protein coding potential (Nagano and Fraser, 2011; Spizzo et al., 2012). Current estimates from the GENCODE database (www.gencodegenes.org) suggest that the human genome contains approximately 16,000 lncRNA genes encoding over 28,000 distinct lncRNAs. Many lncRNAs have emerged as key players in the regulation of numerous biological processes in cancer, such as differentiation, cell cycle regulation, and immune responses (Guttman et al., 2009; Qiu et al., 2015; Bach and Lee, 2018). They can act directly as tumor suppressors or oncogenes, or be regulated by well-known tumor suppressors or oncogenes at the transcriptional or post-transcriptional level (Barsyte-Lovejoy et al., 2006; Huarte et al., 2010). ECs that line the inner surface of the blood vessels are an important part of the matrix in the TME (Junttila and de Sauvage, 2013; Kohlhapp et al., 2015). They are believed to be critical for angiogenesis and tumor metastasis, and lncRNAs may affect tumor progression by regulating endothelial cell biological behavior (Table 2; Figure 3). For example, lncRNA H19 has been reported to be significantly upregulated in glioma-associated endothelial cells cultured in glioma-conditioned medium. Knockdown of lncRNA H19 inhibited glioma-induced endothelial cell proliferation, migration, and tube formation in vitro. Mechanistic evidence suggests that lncRNA H19 regulates the biological behavior of glioma-associated endothelial cells by inhibiting miR-29a (Jia et al., 2016). Furthermore, lncRNA-APC1 plays an important tumor suppressor role in the pathogenesis of colorectal cancer. The following mechanistic studies show that lncRNA-APC1 reduces exosome production in colorectal cancer cells by reducing Rab5b mRNA stability, and this effect inhibits tumor angiogenesis by inhibiting the over-activation of the MAPK pathway in endothelial cells (Wang F. W. et al., 2021). Dysregulated lncRNAs affect endothelial cell biological behavior through multiple mechanisms, so regulation of specific lncRNA expression in tumor cells or/and endothelial cells may have a significant impact on cancer progression.
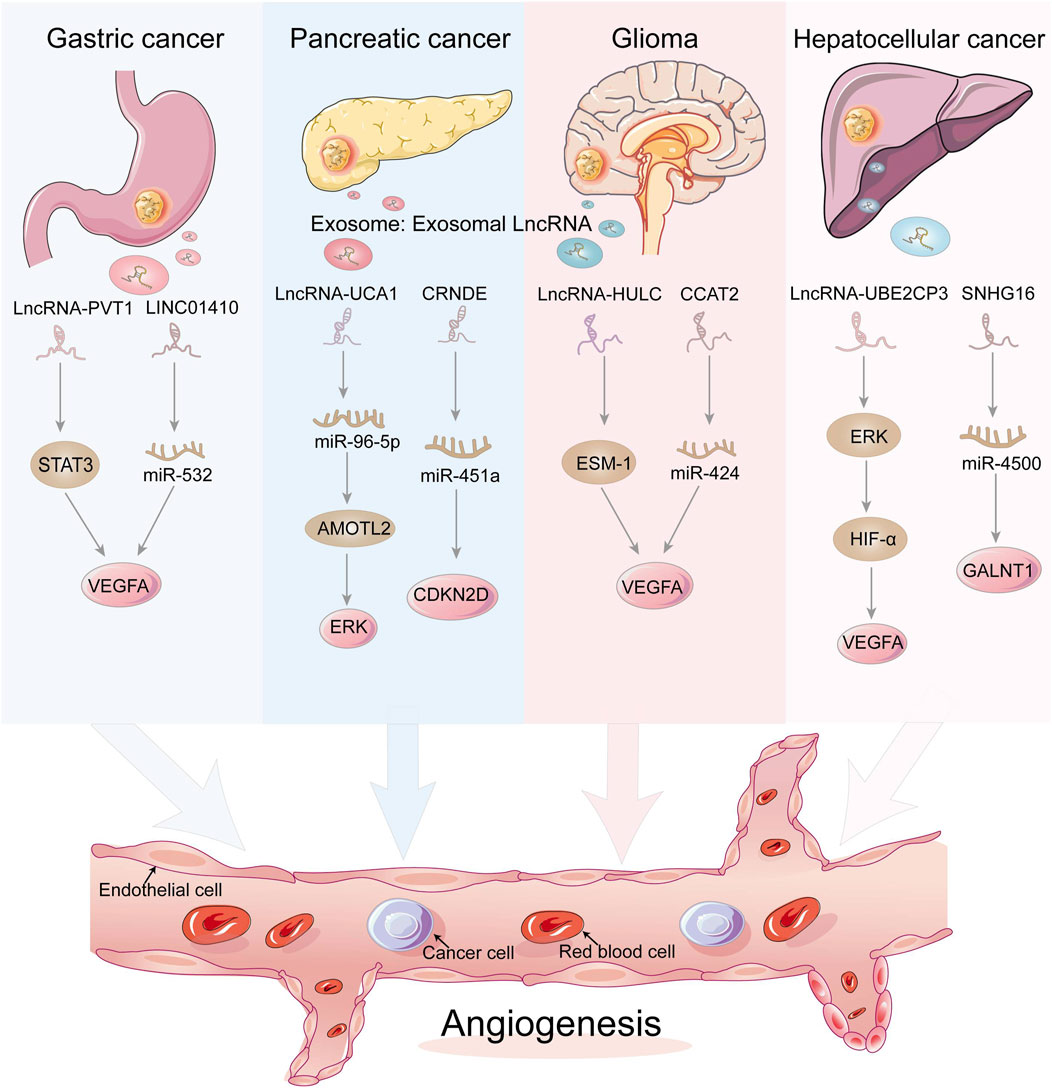
FIGURE 3. Exosomal LncRNAs act on endothelial cells to regulate tumor angiogenesis through a variety of mechanisms. Dysregulated lncRNAs can act directly as tumor factors or act on miRNAs at the transcriptional level, which in turn regulate tumor angiogenesis.
2.2.1 Gastric cancer cells
PVT1 is an oncogenic lncRNA that is significantly expressed in gastric cancer, especially in patients with low differentiation and progressive stages. PVT1 can bind to different proteins to exert oncogenic effects, and in gastric cancer, PVT1 can bind to the signal transduction activator STAT3 to ensure that it is not degraded, thus activating the STAT3 signaling pathway and thus increasing VEGFA in gastric cancer, thus activating the STAT3 signaling pathway and increasing the expression of VEGFA to promote gastric cancer angiogenesis. At the same time, activated STAT3 can also occupy the promoter of PVT1 and promote PVT1 expression, forming a positive feedback regulation (Zhao et al., 2018). Similarly, in NSCLC, the lncRNA TNK2-AS1 can also bind to STAT3 to inhibit its degradation, thus activating the STAT3 signaling pathway and promoting tumor progression and angiogenesis. In addition, STAT3 can also bind to the lncRNA TNK2-AS1 promoter to promote its transcription in positive feedback (Wang et al., 2018a). LINC01410 is also one of the molecules that promote angiogenesis in gastric cancer. LINC01410 can inhibit miR-532-5p expression, while silencing miR-532-5p reduces inhibition of NCF2, thus upregulating NCF2 expression and activating the NF-κB signaling pathway, exacerbating malignant progression and angiogenesis of gastric cancer. Interestingly, NCF2 can bind to the LINC01410 promoter, thereby promoting its transcription, forming a positive feedback loop that exacerbates the development of gastric carcinogenesis (Zhang et al., 2018).
2.2.2 Pancreatic cancer cells
An important feature of the tumor microenvironment is hypoxia caused by inadequate oxygen flow and abnormal tumor vasculature, and exposure of cancer cells to conditions of oxygen deficiency increases the release of exosomes, which in turn promotes angiogenesis and tumor metastasis. In hypoxic PC cells, the expression of lncRNA UCA1 increases and can be transferred to human microvascular endothelial cells HUVECs, promoting angiogenesis and tumor growth via the miR-96-5p/AMOTL2/ERK1/2 axis (Guo et al., 2020). In addition to this, PC cell-derived exosomal CRNDE enhanced angiogenesis by binding to miR-451a to increase CDKN2D expression (Zhu et al., 2021).
2.2.3 Glioma cells
One of the keys to glioma development is abnormal generation of tumor blood vessels, and high-grade gliomas clearly have a higher density of tumor blood vessels that contribute more to tumor development than low-grade gliomas. It has been shown that glioma cells can regulate the tumor microenvironment by secreting exosomes, for example, glioma exosomes can promote angiogenesis by transferring LINC-POU3F3 to human brain microvascular endothelial cells (HBMEC) (Lang et al., 2017a). Additionally, LINC-CCAT2 was found to be highly expressed in glioma cells U87-MG and could be transferred to HUVECs to activate the production of the angiogenic factors VEGFA and TGFβ, while inhibiting the expression of the apoptotic molecules Bax and caspase-3, thus promoting angiogenesis and inhibiting apoptosis in glioma cells (Lang et al., 2017b). LncRNA HULC is one of the most common oncogenes with the potential to promote invasion and angiogenesis. In glioma, Zhu Yu et al. showed that HULC can activate the PI3K/AKT/mTOR signaling pathway, which in turn regulates downstream angiogenic factors VEGF and ESM-1. Furthermore, in a hypoxic environment, HULC can upregulate HIF-1α, which is also one of the key molecules that promote the secretion of angiogenic factors (Zhu et al., 2016).
2.2.4 Hepatocellular carcinoma cells
As tumor growth requires more and more nutrients, this requires the secretion of angiogenic substances to promote tumor angiogenesis. LncRNA has been shown to regulate ECs function and promote the expression of angiogenic factors to regulate angiogenesis. Lin et al. demonstrated that the lncRNA UBE2CP3 can activate the ERK/HIF-1α/p70S6K signaling pathway, increase VEGFA expression and regulate ECs function, thus promoting angiogenesis in hepatocellular carcinoma (Lin J. et al., 2018). Cancer stem-like cells, also known as CD90+ hepatocellular carcinoma cells, are enriched in lncRNA H19, which can be released by encapsulating in exosomes and then transported to endothelial cells, promoting the expression of the angiogenic factor VEGF in endothelial cells and thus regulating hepatocellular carcinoma angiogenesis (Conigliaro et al., 2015). Direct exosomal transfer of MALAT1 to hepatocytes leads to increased invasion and migration of hepatocytes through activation of extracellular signal-regulated kinase 1/2 (ERK1/2) signaling (Li et al., 2020c). Exosomal SNHG16 increases GALNT1 expression by sponging miR-4500 to promote angiogenesis. The SNHG16/miR-4500/GALNT1 axis plays an important role in exosome-mediated angiogenesis and tumor growth in vitro and in vivo (Li et al., 2021). Furthermore, elevated expression of lncRNA-OR3A4 in hepatocellular carcinoma is associated with angiogenesis and promotes the tube formation capacity of HUVEC, mainly through activation of the AGGF1/AKT/mTOR pathway (Li et al., 2019). CRNDE is upregulated in many tumors, promotes cell growth and migration, and is a recognized oncogene, also in hepatoblastoma. CRNDE knockdown inhibits tumor angiogenesis and reduces cell viability in hepatoblastoma, primarily through regulation of mTOR signaling (Dong et al., 2017).
2.2.5 Other cancer cells
Some other cancer exosomal lncRNAs are still associated with endothelial cells (Table 2). Osteosarcoma originates from bone and is the most common of primary malignancies. Zhang et al. showed that lncRNA MALAT1 is associated with osteosarcoma angiogenesis and hypoxic response and that MALAT1 activates the mTOR/HIF-1α pathway, thereby promoting the production of angiogenic factors (Zhang Z. C. et al., 2017). In lung adenocarcinoma, the lncRNA LOC100132354 can affect the downstream target gene VEGFA to promote tumor angiogenesis (Wang et al., 2018b). Some non-angiogenic lncRNAs have the ability to inhibit angiogenesis. For example, GAS5 can inhibit the activation of the Wnt/β-catenin pathway to suppress angiogenesis in CRC (Song et al., 2019). Regarding MEG3, a recognized tumor suppressor, it inhibits tumor progression in breast cancer mainly by suppressing AKT signaling and also inhibits capillary angiogenesis in endothelial cells by reducing the expression of tumor angiogenic factors (Lu et al., 2020). The lncRNA MALAT1 can be transported through exosomes to endothelial cells in epithelial ovarian cancer (EOC) and then regulates the vasculature of endothelial cells by generating related genes that stimulate pro-angiogenic behavior. In addition, serum exosomal MALAT1 levels were strongly associated with advanced and metastatic outcomes, which were independent predictors of overall survival in EOC (Qiu et al., 2018). Interestingly, lncRNAs can affect exosome production in addition to being transported by exosomes. In colorectal cancer, activation of the Adenomatous Polyp in Colon (APC) gene of lncRNA (lncRNA APC1) can directly affect the stability of Rab5b mRNA, thereby inhibiting exosome production by CRC cells and ultimately tumor angiogenesis (Wang F. W. et al., 2021). Moreover, exosomal lncRNA PDAT1 regulates the activity of the miR-329-3p/Netrin-1-CD146 complex to promote tumor metastasis (Fang et al., 2022). In lung cancer, the exosomal lncRNA LINC01356 and the exosomal lnc-MMP2-2 derived from NSCLC cells play a key role in the remodeling of the blood-brain barrier, thereby participating in brain metastasis (Geng et al., 2022). Exosomal lnc-MMP2-2 promotes brain metastasis via the miRNA-1207-5p/EPB41L5 axis (Wu et al., 2021). In thyroid cancer, exosome FGD5-AS1 targets the miR-6838-5p/VAV2 axis to promote angiogenesis and metastasis (Liu et al., 2022).
3 Conclusion and prospect on endothelial cells and exosomes
Exosomes are important carriers of cell-to-cell communication signals and genetic material in the tumor microenvironment. In this review, we divide them into different types of cancer and summarize the relationship between miRNAs and lncRNAs with endothelial cells, promoting tumor angiogenesis and tumor angiogenesis. Mechanisms of lymphangiogenesis, demonstrating the complexity of their mediated angiogenesis in cancer development. Although ncRNAs do not encode proteins, they do play critical roles in regulating the levels of many cellular and extracellular proteins, particularly in the early stages of certain tumors, by mediating gene silencing at the transcriptional level to regulate the expression of cancer-related proteins, which in turn affects aspects of angiogenesis, apoptosis, and tumor metastasis. NcRNAs can be used as a new class of markers for early clinical diagnosis and prognosis, and exosomes can be used as carriers to deliver them to various parts of the body, helping them participate more actively in intercellular communication and function. Cancer-derived exosomal ncRNAs can promote tumor angiogenesis and lymphangiogenesis by altering gene expression in a vatiety of cell types, including endothelial cells. Therefore, the regulatory functions of ncRNAs in tumor angiogenesis and lymphangiogenesis can be considered multidimensional.
The mechanistic summary in this paper can help develop effective and precise cancer therapies and, based on current research related to the regulation of tumor angiogenesis by ncRNAs, can be used to develop new cancer biomarkers and therapies depending on the type of cancer. Identifying the different mechanisms involved in identifying therapeutic approaches has seminal implications for new cancer treatments, and more research is needed to achieve this. In addition, certain specific ncRNAs can be used as a new class of markers for early clinical diagnosis and prognosis, also providing a new idea for tumor treatment. LncRNAs and miRNAs may be a feasible strategy to monitor the efficacy of anti-angiogenic therapy and predict prognosis. In addition, regulation of angiogenesis-related signaling pathways may also serve as a new therapeutic direction, and the molecular mechanisms of miRNAs and lncRNAs in tumor development and development need to be investigated in more depth, thus contributing to the improvement of tumor diagnosis and treatment.
Author contributions
S-LD and W-JF contributed to the direction and guidance of this review; S-LD and Y-KJ collected formal resources, wrote the original draft and prepared the figures; L-SP, DO, Z-JZ and J-PW provided critical revisions and contributed to the editing of the paper. All authors contributed to the article and approved the submitted version.
Funding
This work was partially supported by the National Natural Science Foundation of China (project NO. 81602167), the Hunan Provincial Natural Science Foundation of China (project NO. 2017JJ3494 and 2021JJ31100), and the Science and Technology Program Foundation of Changsha City (project NO. kq2004085).
Acknowledgments
We thank all authors to collect data and make improvement of this manuscript.
Conflict of interest
The authors declare that the research was conducted in the absence of any commercial or financial relationships that could be construed as a potential conflict of interest.
Publisher’s note
All claims expressed in this article are solely those of the authors and do not necessarily represent those of their affiliated organizations, or those of the publisher, the editors and the reviewers. Any product that may be evaluated in this article, or claim that may be made by its manufacturer, is not guaranteed or endorsed by the publisher.
Abbreviations
TME, Tumor microenvironment; ECs, endothelial cells; VEGF, vascular endothelial growth factor; ncRNAs, non-coding RNAs; lncRNAs, long-chain non-coding RNAs; miRNAs, microRNAs; TAM, Tumor-associated macrophage; TEXs, Tumor-derived exosomes; PDAC, pancreatic ductal adenocarcinoma; UTR, untranslated region; VASH2, Vasohibin 2; HCC, hepatocellular carcinoma; VE-Cad, VE-Cadherin; CRC, colorectal cancer; HUVEC, human umbilical vein endothelial cells; PHD1 and 2, prolyl hydroxylases 1 and 2; HIF-1α, hypoxia-inducible factor 1 alpha; LUAD, lung adenocarcinoma; SCLC, small cell lung cancer; NSCLC, non-small cell lung cancer; HBMEC, human brain microvascular endothelial cells; ERK1/2, extracellular signal-regulated kinase 1/2; EOC, epithelial ovarian cancer; APC, Adenomatous Polyp in Colon; EOC, epithelial ovarian cancer.
References
Aguilar-Cazares, D., Chavez-Dominguez, R., Carlos-Reyes, A., Lopez-Camarillo, C., Hernadez de la Cruz, O. N., and Lopez-Gonzalez, J. S. (2019). Contribution of angiogenesis to inflammation and cancer. Front. Oncol. 9, 1399. doi:10.3389/fonc.2019.01399
Ahir, B. K., Engelhard, H. H., and Lakka, S. S. (2020). Tumor development and angiogenesis in adult brain tumor: Glioblastoma. Mol. Neurobiol. 57, 2461–2478. doi:10.1007/s12035-020-01892-8
Ahmadi, M., and Rezaie, J. (2020). Tumor cells derived-exosomes as angiogenenic agents: Possible therapeutic implications. J. Transl. Med. 18, 249. doi:10.1186/s12967-020-02426-5
Anderson, N. M., and Simon, M. C. J. C. B. (2020). The tumor microenvironment. tumor Microenviron. 30, R921–R925. doi:10.1016/j.cub.2020.06.081
Arcucci, V., Stacker, S. A., and Achen, M. G. (2021b). Control of gene expression by exosome-derived non-coding RNAs in cancer angiogenesis and lymphangiogenesis. Biomolecules 11, 249. doi:10.3390/biom11020249
Arcucci, V., Stacker, S. A., and Achen, M. G. J. B. (2021a). Control of gene expression by exosome-derived non-coding RNAs in cancer angiogenesis and lymphangiogenesis. Biomolecules 11, 249. doi:10.3390/biom11020249
Aslan, C., Maralbashi, S., Salari, F., Kahroba, H., Sigaroodi, F., Kazemi, T., et al. (2019). Tumor-derived exosomes: Implication in angiogenesis and antiangiogenesis cancer therapy. J. Cell. physiology 234, 16885–16903. doi:10.1002/jcp.28374
Bach, D. H., and Lee, S. K. (2018). Long noncoding RNAs in cancer cells. Cancer Lett. 419, 152–166. doi:10.1016/j.canlet.2018.01.053
Bao, L., You, B., Shi, S., Shan, Y., Zhang, Q., Yue, H., et al. (2018). Metastasis-associated miR-23a from nasopharyngeal carcinoma-derived exosomes mediates angiogenesis by repressing a novel target gene TSGA10. Oncogene 37, 2873–2889. doi:10.1038/s41388-018-0183-6
Barachini, S., Ghelardoni, S., and Madonna, R. J. J. o. C. M. (2023). Vascular progenitor cells: From cancer to tissue repair. J. Clin. Med. 12, 2399. doi:10.3390/jcm12062399
Barsyte-Lovejoy, D., Lau, S. K., Boutros, P. C., Khosravi, F., Jurisica, I., Andrulis, I. L., et al. (2006). The c-Myc oncogene directly induces the H19 noncoding RNA by allele-specific binding to potentiate tumorigenesis. Cancer Res. 66, 5330–5337. doi:10.1158/0008-5472.CAN-06-0037
Bartel, D. P. (2004). MicroRNAs: Genomics, biogenesis, mechanism, and function. Cell 116, 281–297. doi:10.1016/s0092-8674(04)00045-5
Bartel, D. P. (2009). MicroRNAs: Target recognition and regulatory functions. Cell 136, 215–233. doi:10.1016/j.cell.2009.01.002
Braga, E. A., Fridman, M. V., Loginov, V. I., Dmitriev, A. A., and Morozov, S. G. (2019). Molecular mechanisms in clear cell renal cell carcinoma: Role of miRNAs and hypermethylated miRNA genes in crucial oncogenic pathways and processes. Front. Genet. 10, 320. doi:10.3389/fgene.2019.00320
Carmeliet, P., and Jain, R. K. (2011). Molecular mechanisms and clinical applications of angiogenesis. Nature 473, 298–307. doi:10.1038/nature10144
Castellano, J. J., Marrades, R. M., Molins, L., Viñolas, N., Moises, J., Canals, J., et al. (2020). Extracellular vesicle lincRNA-p21 expression in tumor-draining pulmonary vein defines prognosis in NSCLC and modulates endothelial cell behavior. Cancers (Basel) 12, 734. doi:10.3390/cancers12030734
Chen, C. W., Fu, M., Du, Z. H., Zhao, F., Yang, W. W., Xu, L. H., et al. (2020). Long noncoding RNA MRPL23-AS1 promoteoid cystic carcinoma lung metastasis. Cancer Res. 80, 2273–2285. doi:10.1158/0008-5472.CAN-19-0819
Chen, H. X., Xu, X. X., Tan, B. Z., Zhang, Z., and Zhou, X. D. (2017). MicroRNA-29b inhibits angiogenesis by targeting VEGFA through the MAPK/ERK and PI3K/akt signaling pathways in endometrial carcinoma. Cell. physiology Biochem. Int. J. Exp. Cell. physiology, Biochem. Pharmacol. 41, 933–946. doi:10.1159/000460510
Chen, X., Zhang, S., Du, K., Zheng, N., Liu, Y., Chen, H., et al. (2021). Gastric cancer-secreted exosomal X26nt increases angiogenesis and vascular permeability by targeting VE-cadherin. Cancer Sci. 112, 1839–1852. doi:10.1111/cas.14740
Cheng, C., Zhang, Z., Cheng, F., and Shao, Z. (2020). Exosomal lncRNA RAMP2-AS1 derived from chondrosarcoma cells promotes angiogenesis through miR-2355-5p/VEGFR2 Axis. Onco Targets Ther. 13, 3291–3301. doi:10.2147/OTT.S244652
Cocks, A., Del Vecchio, F., Martinez-Rodriguez, V., Schukking, M., and Fabbri, M. (2022). Pro-tumoral functions of tumor-associated macrophage EV-miRNA. Seminars cancer Biol. 86, 58–63. doi:10.1016/j.semcancer.2021.08.001
Conigliaro, A., Costa, V., Lo Dico, A., Saieva, L., Buccheri, S., Dieli, F., et al. (2015). CD90+ liver cancer cells modulate endothelial cell phenotype through the release of exosomes containing H19 lncRNA. Mol. Cancer 14, 155. doi:10.1186/s12943-015-0426-x
Creamer, K. M., and Lawrence, J. B. (2017). Xist RNA: A window into the broader role of RNA in nuclear chromosome architecture. Philosophical Trans. R. Soc. Lond. Ser. B, Biol. Sci. 372, 20160360. doi:10.1098/rstb.2016.0360
Ding, B. S., Cao, Z., Lis, R., Nolan, D. J., Guo, P., Simons, M., et al. (2014). Divergent angiocrine signals from vascular niche balance liver regeneration and fibrosis. Nature 505, 97–102. doi:10.1038/nature12681
Dominiak, A., Chełstowska, B., Olejarz, W., and Nowicka, G. (2020). Communication in the cancer microenvironment as a target for therapeutic interventions. Cancers 12, 1232. doi:10.3390/cancers12051232
Dong, R., Liu, X. Q., Zhang, B. B., Liu, B. H., Zheng, S., and Dong, K. R. (2017). Long non-coding RNA-CRNDE: A novel regulator of tumor growth and angiogenesis in hepatoblastoma. Oncotarget 8, 42087–42097. doi:10.18632/oncotarget.14992
Dou, R., Liu, K., Yang, C., Zheng, J., Shi, D., Lin, X., et al. (2021). EMT-cancer cells-derived exosomal miR-27b-3p promotes circulating tumour cells-mediated metastasis by modulating vascular permeability in colorectal cancer. Clin. Transl. Med. 11, e595. doi:10.1002/ctm2.595
Duan, B., Shi, S., Yue, H., You, B., Shan, Y., Zhu, Z., et al. (2019). Exosomal miR-17-5p promotes angiogenesis in nasopharyngeal carcinoma via targeting BAMBI. J. Cancer 10, 6681–6692. doi:10.7150/jca.30757
Fang, J. H., Zhang, Z. J., Shang, L. R., Luo, Y. W., Lin, Y. F., Yuan, Y., et al. (2018). Hepatoma cell-secreted exosomal microRNA-103 increases vascular permeability and promotes metastasis by targeting junction proteins. Hepatology 68, 1459–1475. doi:10.1002/hep.29920
Fang, X., Xu, Y., Li, K., Liu, P., Zhang, H., Jiang, Y., et al. (2022). Exosomal lncRNA PCAT1 promotes tumor circulating cell-mediated colorectal cancer liver metastasis by regulating the activity of the miR-329-3p/netrin-1-cd146 complex. J. Immunol. Res. 2022, 9916228. doi:10.1155/2022/9916228
Fares, J., Fares, M. Y., Khachfe, H. H., Salhab, H. A., Fares, Y. J. S. t., and therapy, t. (2020). Molecular principles of metastasis: A hallmark of cancer revisited. Signal Transduct. Target. Ther. 5, 28. doi:10.1038/s41392-020-0134-x
Forder, A., Hsing, C. Y., Trejo Vazquez, J., and Garnis, C. (2021). Emerging role of extracellular vesicles and cellular communication in metastasis. Cells 10, 3429. doi:10.3390/cells10123429
Fu, W. M., Lu, Y. F., Hu, B. G., Liang, W. C., Zhu, X., Yang, H. D., et al. (2016). Long noncoding RNA Hotair mediated angiogenesis in nasopharyngeal carcinoma by direct and indirect signaling pathways. Oncotarget 7, 4712–4723. doi:10.18632/oncotarget.6731
Geng, S., Tu, S., Bai, Z., and Geng, Y. (2022). Exosomal lncRNA LINC01356 derived from brain metastatic nonsmall-cell lung cancer cells remodels the blood-brain barrier. Front. Oncol. 12, 825899. doi:10.3389/fonc.2022.825899
Giraldo, N. A., Sanchez-Salas, R., Peske, J. D., Vano, Y., Becht, E., Petitprez, F., et al. (2019). The clinical role of the TME in solid cancer. Br. J. cancer 120, 45–53. doi:10.1038/s41416-018-0327-z
Głuszko, A., Szczepański, M. J., Ludwig, N., Mirza, S. M., and Olejarz, W. (2019). Exosomes in cancer: Circulating immune-related biomarkers. BioMed Res. Int. 2019, 1628029. doi:10.1155/2019/1628029
Gong, C., and Maquat, L. E. (2011). lncRNAs transactivate STAU1-mediated mRNA decay by duplexing with 3' UTRs via Alu elements. Nature 470, 284–288. doi:10.1038/nature09701
Guo, Z., Wang, X., Yang, Y., Chen, W., Zhang, K., Teng, B., et al. (2020). Hypoxic tumor-derived exosomal long noncoding RNA UCA1 promotes angiogenesis via miR-96-5p/AMOTL2 in pancreatic cancer. Nucleic acids. 22, 179–195. doi:10.1016/j.omtn.2020.08.021
Guttman, M., Amit, I., Garber, M., French, C., Lin, M. F., Feldser, D., et al. (2009). Chromatin signature reveals over a thousand highly conserved large non-coding RNAs in mammals. Nature 458, 223–227. doi:10.1038/nature07672
Han, W., Sulidankazha, Q., Nie, X., Yilidan, R., and Len, K. (2021). Pancreatic cancer cells-derived exosomal long non-coding RNA CCAT1/microRNA-138-5p/HMGA1 axis promotes tumor angiogenesis. Life Sci. 278, 119495. doi:10.1016/j.lfs.2021.119495
Hanahan, D., and Weinberg, R. A. (2011). Hallmarks of cancer: The next generation. Cell 144, 646–674. doi:10.1016/j.cell.2011.02.013
He, G., Peng, X., Wei, S., Yang, S., Li, X., Huang, M., et al. (2022). Exosomes in the hypoxic TME: From release, uptake and biofunctions to clinical applications. Mol. Cancer 21, 19. doi:10.1186/s12943-021-01440-5
He, L., Zhu, W., Chen, Q., Yuan, Y., Wang, Y., Wang, J., et al. (2019). Ovarian cancer cell-secreted exosomal miR-205 promotes metastasis by inducing angiogenesis. Theranostics 9, 8206–8220. doi:10.7150/thno.37455
He, Q., Ye, A., Ye, W., Liao, X., Qin, G., Xu, Y., et al. (2021a). Cancer-secreted exosomal miR-21-5p induces angiogenesis and vascular permeability by targeting KRIT1. Cell Death Dis. 12, 576. doi:10.1038/s41419-021-03803-8
He, S., Zhang, W., Li, X., Wang, J., Chen, X., Chen, Y., et al. (2021b). Oral squamous cell carcinoma (OSCC)-derived exosomal MiR-221 targets and regulates phosphoinositide-3-kinase regulatory subunit 1 (PIK3R1) to promote human umbilical vein endothelial cells migration and tube formation. Bioengineered 12, 2164–2174. doi:10.1080/21655979.2021.1932222
Hsu, Y. L., Hung, J. Y., Chang, W. A., Lin, Y. S., Pan, Y. C., Tsai, P. H., et al. (2017). Hypoxic lung cancer-secreted exosomal miR-23a increased angiogenesis and vascular permeability by targeting prolyl hydroxylase and tight junction protein ZO-1. Oncogene 36, 4929–4942. doi:10.1038/onc.2017.105
Hu, H. Y., Yu, C. H., Zhang, H. H., Zhang, S. Z., Yu, W. Y., Yang, Y., et al. (2019). Exosomal miR-1229 derived from colorectal cancer cells promotes angiogenesis by targeting HIPK2. Int. J. Biol. Macromol. 132, 470–477. doi:10.1016/j.ijbiomac.2019.03.221
Huang, Y., Kanada, M., Ye, J., Deng, Y., He, Q., Lei, Z., et al. (2022). Exosome-mediated remodeling of the tumor microenvironment: From local to distant intercellular communication. Cancer Lett. 543, 215796. doi:10.1016/j.canlet.2022.215796
Huarte, M., Guttman, M., Feldser, D., Garber, M., Koziol, M. J., Kenzelmann-Broz, D., et al. (2010). A large intergenic noncoding RNA induced by p53 mediates global gene repression in the p53 response. Cell 142, 409–419. doi:10.1016/j.cell.2010.06.040
Hutchinson, J. N., Ensminger, A. W., Clemson, C. M., Lynch, C. R., Lawrence, J. B., and Chess, A. (2007). A screen for nuclear transcripts identifies two linked noncoding RNAs associated with SC35 splicing domains. BMC genomics 8, 39. doi:10.1186/1471-2164-8-39
Jászai, J., and Schmidt, M. H. H. (2019). Trends and challenges in tumor anti-angiogenic therapies. Cells 8, 1102. doi:10.3390/cells8091102
Jia, P., Cai, H., Liu, X., Chen, J., Ma, J., Wang, P., et al. (2016). Long non-coding RNA H19 regulates glioma angiogenesis and the biological behavior of glioma-associated endothelial cells by inhibiting microRNA-29a. Cancer Lett. 381, 359–369. doi:10.1016/j.canlet.2016.08.009
Jia, Z., Jia, J., Yao, L., and Li, Z. (2022). Crosstalk of exosomal non-coding RNAs in the tumor microenvironment: Novel Frontiers. Front. Immunol. 13, 900155. doi:10.3389/fimmu.2022.900155
Jiang, J., Lu, J., Wang, X., Sun, B., Liu, X., Ding, Y., et al. (2021). Glioma stem cell-derived exosomal miR-944 reduces glioma growth and angiogenesis by inhibiting AKT/ERK signaling. Aging (Albany NY) 13, 19243–19259. doi:10.18632/aging.203243
Junttila, M. R., and de Sauvage, F. J. (2013). Influence of tumour micro-environment heterogeneity on therapeutic response. Nature 501, 346–354. doi:10.1038/nature12626
Kim, D. H., Park, S., Kim, H., Choi, Y. J., Kim, S. Y., Sung, K. J., et al. (2020). Tumor-derived exosomal miR-619-5p promotes tumor angiogenesis and metastasis through the inhibition of RCAN1.4. Cancer Lett. 475, 2–13. doi:10.1016/j.canlet.2020.01.023
Kohlhapp, F. J., Mitra, A. K., Lengyel, E., and Peter, M. E. (2015). MicroRNAs as mediators and communicators between cancer cells and the tumor microenvironment. Oncogene 34, 5857–5868. doi:10.1038/onc.2015.89
Kong, X., Li, J., Li, Y., Duan, W., Qi, Q., Wang, T., et al. (2021). A novel long non-coding RNA AC073352.1 promotes metastasis and angiogenesis via interacting with YBX1 in breast cancer. Cell Death Dis. 12, 670. doi:10.1038/s41419-021-03943-x
Lam, M. T., Cho, H., Lesch, H. P., Gosselin, D., Heinz, S., Tanaka-Oishi, Y., et al. (2013). Rev-Erbs repress macrophage gene expression by inhibiting enhancer-directed transcription. Nature 498, 511–515. doi:10.1038/nature12209
Lang, H. L., Hu, G. W., Chen, Y., Liu, Y., Tu, W., Lu, Y. M., et al. (2017a). Glioma cells promote angiogenesis through the release of exosomes containing long non-coding RNA POU3F3. Eur. Rev. Med. Pharmacol. Sci. 21, 959–972.
Lang, H. L., Hu, G. W., Zhang, B., Kuang, W., Chen, Y., Wu, L., et al. (2017b). Glioma cells enhance angiogenesis and inhibit endothelial cell apoptosis through the release of exosomes that contain long non-coding RNA CCAT2. Oncol. Rep. 38, 785–798. doi:10.3892/or.2017.5742
Lee, S., Kopp, F., Chang, T. C., Sataluri, A., Chen, B., Sivakumar, S., et al. (2016). Noncoding RNA NORAD regulates genomic stability by sequestering PUMILIO proteins. Cell 164, 69–80. doi:10.1016/j.cell.2015.12.017
Lei, L., and Mou, Q. (2020). Exosomal taurine up-regulated 1 promotes angiogenesis and endothelial cell proliferation in cervical cancer. Cancer Biol. Ther. 21, 717–725. doi:10.1080/15384047.2020.1764318
Li, J., Yuan, H., Xu, H., Zhao, H., and Xiong, N. (2020a). Hypoxic cancer-secreted exosomal miR-182-5p promotes glioblastoma angiogenesis by targeting kruppel-like factor 2 and 4. Mol. Cancer Res. 18, 1218–1231. doi:10.1158/1541-7786.MCR-19-0725
Li, K., Blum, Y., Verma, A., Liu, Z., Pramanik, K., Leigh, N. R., et al. (2010). A noncoding antisense RNA in tie-1 locus regulates tie-1 function in vivo. Blood 115, 133–139. doi:10.1182/blood-2009-09-242180
Li, S., Qi, Y., Huang, Y., Guo, Y., Huang, T., and Jia, L. (2021). Exosome-derived SNHG16 sponging miR-4500 activates HUVEC angiogenesis by targeting GALNT1 via PI3K/Akt/mTOR pathway in hepatocellular carcinoma. J. Physiol. Biochem. 77, 667–682. doi:10.1007/s13105-021-00833-w
Li, W. D., Zhou, D. M., Sun, L. L., Xiao, L., Liu, Z., Zhou, M., et al. (2018). LncRNA WTAPP1 promotes migration and angiogenesis of endothelial progenitor cells via MMP1 through MicroRNA 3120 and akt/PI3K/autophagy pathways. Stem cells Dayt. Ohio) 36, 1863–1874. doi:10.1002/stem.2904
Li, W., Fu, Q., Man, W., Guo, H., and Yang, P. (2019). LncRNA OR3A4 participates in the angiogenesis of hepatocellular carcinoma through modulating AGGF1/akt/mTOR pathway. Eur. J. Pharmacol. 849, 106–114. doi:10.1016/j.ejphar.2019.01.049
Li, Y., Cai, B., Shen, L., Dong, Y., Lu, Q., Sun, S., et al. (2017). MiRNA-29b suppresses tumor growth through simultaneously inhibiting angiogenesis and tumorigenesis by targeting Akt3. Cancer Lett. 397, 111–119. doi:10.1016/j.canlet.2017.03.032
Li, Y., Zhang, H., Fan, L., Mou, J., Yin, Y., Peng, C., et al. (2020b). MiR-629-5p promotes the invasion of lung adenocarcinoma via increasing both tumor cell invasion and endothelial cell permeability. Oncogene 39, 3473–3488. doi:10.1038/s41388-020-1228-1
Li, Y., Zhang, X., Zheng, Q., Zhang, Y., Ma, Y., Zhu, C., et al. (2020c). YAP1 inhibition in HUVECs is associated with released exosomes and increased hepatocarcinoma invasion and metastasis. Mol. Ther. Nucleic Acids 21, 86–97. doi:10.1016/j.omtn.2020.05.021
Lin, J., Cao, S., Wang, Y., Hu, Y., Liu, H., Li, J., et al. (2018b). Long non-coding RNA UBE2CP3 enhances HCC cell secretion of VEGFA and promotes angiogenesis by activating ERK1/2/HIF-1α/VEGFA signalling in hepatocellular carcinoma. J. Exp. Clin. Cancer Res. 37, 113. doi:10.1186/s13046-018-0727-1
Lin, X. J., Fang, J. H., Yang, X. J., Zhang, C., Yuan, Y., Zheng, L., et al. (2018a). Hepatocellular carcinoma cell-secreted exosomal MicroRNA-210 promotes angiogenesis in vitro and in vivo. Mol. Ther. Nucleic Acids 11, 243–252. doi:10.1016/j.omtn.2018.02.014
Liu, B., Chen, J., Shang, F., Lian, M., Shen, X., and Fang, J. (2022). Tumor-derived exosome FGD5-AS1 promotes angiogenesis, vascular permeability, and metastasis in thyroid cancer by targeting the miR-6838-5p/VAV2 Axis. J. Oncol. 2022, 4702855. doi:10.1155/2022/4702855
Liu, H., Lei, C., He, Q., Pan, Z., Xiao, D., and Tao, Y. (2018). Nuclear functions of mammalian MicroRNAs in gene regulation, immunity and cancer. Mol. Cancer 17, 64. doi:10.1186/s12943-018-0765-5
Lu, J., Liu, Q. H., Wang, F., Tan, J. J., Deng, Y. Q., Peng, X. H., et al. (2018). Exosomal miR-9 inhibits angiogenesis by targeting MDK and regulating PDK/AKT pathway in nasopharyngeal carcinoma. J. Exp. Clin. Cancer Res. 37, 147. doi:10.1186/s13046-018-0814-3
Lu, Y., Chen, L., Li, L., and Cao, Y. (2020). Exosomes derived from brain metastatic breast cancer cells destroy the blood-brain barrier by carrying lncRNA GS1-600g8.5. Biomed. Res. Int. 2020, 7461727. doi:10.1155/2020/7461727
Malakoti, F., Targhazeh, N., Karimzadeh, H., Mohammadi, E., Asadi, M., Asemi, Z., et al. (2021). Multiple function of lncRNA MALAT1 in cancer occurrence and progression. Chem. Biol. Drug Des. 101, 1113–1137. doi:10.1111/cbdd.14006
Man, H. S. J., Sukumar, A. N., Lam, G. C., Turgeon, P. J., Yan, M. S., Ku, K. H., et al. (2018). Angiogenic patterning by STEEL, an endothelial-enriched long noncoding RNA. Proc. Natl. Acad. Sci. U. S. A. 115, 2401–2406. doi:10.1073/pnas.1715182115
Mao, S., Lu, Z., Zheng, S., Zhang, H., Zhang, G., Wang, F., et al. (2020). Exosomal miR-141 promotes tumor angiogenesis via KLF12 in small cell lung cancer. J. Exp. Clin. cancer Res. CR 39, 193. doi:10.1186/s13046-020-01680-1
Mao, S., Zheng, S., Lu, Z., Wang, X., Wang, Y., Zhang, G., et al. (2021). Exosomal miR-375-3p breaks vascular barrier and promotes small cell lung cancer metastasis by targeting claudin-1. Transl. Lung Cancer Res. 10, 3155–3172. doi:10.21037/tlcr-21-356
Masoumi-Dehghi, S., Babashah, S., and Sadeghizadeh, M. (2020). microRNA-141-3p-containing small extracellular vesicles derived from epithelial ovarian cancer cells promote endothelial cell angiogenesis through activating the JAK/STAT3 and NF-κB signaling pathways. J. Cell Commun. Signal 14, 233–244. doi:10.1007/s12079-020-00548-5
Melo, C. A., Drost, J., Wijchers, P. J., van de Werken, H., de Wit, E., Oude Vrielink, J. A., et al. (2013). eRNAs are required for p53-dependent enhancer activity and gene transcription. Mol. Cell 49, 524–535. doi:10.1016/j.molcel.2012.11.021
Mezzadra, R., Sun, C., Jae, L. T., Gomez-Eerland, R., de Vries, E., Wu, W., et al. (2017). Identification of CMTM6 and CMTM4 as PD-L1 protein regulators. Nature 549, 106–110. doi:10.1038/nature23669
Moh-Moh-Aung, A., Fujisawa, M., Ito, S., Katayama, H., Ohara, T., Ota, Y., et al. (2020). Decreased miR-200b-3p in cancer cells leads to angiogenesis in HCC by enhancing endothelial ERG expression. Sci. Rep. 10, 10418. doi:10.1038/s41598-020-67425-4
Nagano, T., and Fraser, P. (2011). No-nonsense functions for long noncoding RNAs. Cell 145, 178–181. doi:10.1016/j.cell.2011.03.014
Qiu, J. J., Lin, X. J., Tang, X. Y., Zheng, T. T., Lin, Y. Y., and Hua, K. Q. (2018). Exosomal metastasis-associated lung adenocarcinoma transcript 1 promotes angiogenesis and predicts poor prognosis in epithelial ovarian cancer. Int. J. Biol. Sci. 14, 1960–1973. doi:10.7150/ijbs.28048
Qiu, M., Xu, Y., Wang, J., Zhang, E., Sun, M., Zheng, Y., et al. (2015). A novel lncRNA, LUADT1, promotes lung adenocarcinoma proliferation via the epigenetic suppression of p27. Cell Death Dis. 6, e1858. doi:10.1038/cddis.2015.203
Shang, D., Xie, C., Hu, J., Tan, J., Yuan, Y., Liu, Z., et al. (2020). Pancreatic cancer cell-derived exosomal microRNA-27a promotes angiogenesis of human microvascular endothelial cells in pancreatic cancer via BTG2. J. Cell Mol. Med. 24, 588–604. doi:10.1111/jcmm.14766
Shi, W., Zhang, C., Ning, Z., Hua, Y., Li, Y., Chen, L., et al. (2021). CMTM8 as an LPA1-associated partner mediates lysophosphatidic acid-induced pancreatic cancer metastasis. Ann. Transl. Med. 9, 42. doi:10.21037/atm-20-1013
Shi, Y., Yang, X., Xue, X., Sun, D., Cai, P., Song, Q., et al. (2019). HANR promotes lymphangiogenesis of hepatocellular carcinoma via secreting miR-296 exosome and regulating EAG1/VEGFA signaling in HDLEC cells. J. Cell Biochem. 120, 17699–17708. doi:10.1002/jcb.29036
Shweiki, D., Itin, A., Soffer, D., and Keshet, E. (1992). Vascular endothelial growth factor induced by hypoxia may mediate hypoxia-initiated angiogenesis. Nature 359, 843–845. doi:10.1038/359843a0
Soheilifar, M. H., Masoudi-Khoram, N., Madadi, S., Nobari, S., Maadi, H., Keshmiri Neghab, H., et al. (2022). Angioregulatory microRNAs in breast cancer: Molecular mechanistic basis and implications for therapeutic strategies. J. Adv. Res. 37, 235–253. doi:10.1016/j.jare.2021.06.019
Song, J., Shu, H., Zhang, L., and Xiong, J. (2019). Long noncoding RNA GAS5 inhibits angiogenesis and metastasis of colorectal cancer through the Wnt/β-catenin signaling pathway. J. Cell Biochem. 120, 6937–6951. doi:10.1002/jcb.27743
Spizzo, R., Almeida, M. I., Colombatti, A., and Calin, G. A. (2012). Long non-coding RNAs and cancer: A new frontier of translational research? Oncogene 31, 4577–4587. doi:10.1038/onc.2011.621
Stacker, S. A., Williams, S. P., Karnezis, T., Shayan, R., Fox, S. B., and Achen, M. G. (2014). Lymphangiogenesis and lymphatic vessel remodelling in cancer. Nat. Rev. Cancer 14, 159–172. doi:10.1038/nrc3677
Stec, M., Baj-Krzyworzeka, M., Baran, J., Węglarczyk, K., Zembala, M., Barbasz, J., et al. (2015). Isolation and characterization of circulating micro(nano)vesicles in the plasma of colorectal cancer patients and their interactions with tumor cells. Oncol. Rep. 34, 2768–2775. doi:10.3892/or.2015.4228
Sun, B., Han, Y., and Shi, M. (2021). Stromal-derived miR-486-5p promotes metastasis of non-small-cell lung cancer cells by targeting the CADM1/tight junctions axis in vascular endothelial cells. Cell Biol. Int. 45, 849–857. doi:10.1002/cbin.11531
Tao, K., Liu, J., Liang, J., Xu, X., Xu, L., and Mao, W. (2021). Vascular endothelial cell-derived exosomal miR-30a-5p inhibits lung adenocarcinoma malignant progression by targeting CCNE2. Carcinogenesis 42, 1056–1067. doi:10.1093/carcin/bgab051
Tao, S. C., Huang, J. Y., Wei, Z. Y., Li, Z. X., and Guo, S. C. (2020). EWSAT1 acts in concert with exosomes in osteosarcoma progression and tumor-induced angiogenesis: The "double stacking effect. Adv. Biosyst. 4, e2000152. doi:10.1002/adbi.202000152
Tripathi, V., Shen, Z., Chakraborty, A., Giri, S., Freier, S. M., Wu, X., et al. (2013). Long noncoding RNA MALAT1 controls cell cycle progression by regulating the expression of oncogenic transcription factor B-MYB. PLoS Genet. 9, e1003368. doi:10.1371/journal.pgen.1003368
Uhlmann, S., Mannsperger, H., Zhang, J. D., Horvat, E., Schmidt, C., Küblbeck, M., et al. (2012). Global microRNA level regulation of EGFR-driven cell-cycle protein network in breast cancer. Mol. Syst. Biol. 8, 570. doi:10.1038/msb.2011.100
Umezu, T., Ohyashiki, K., Kuroda, M., and Ohyashiki, J. H. (2013). Leukemia cell to endothelial cell communication via exosomal miRNAs. Oncogene 32, 2747–2755. doi:10.1038/onc.2012.295
Wang, F. W., Cao, C. H., Han, K., Zhao, Y. X., Cai, M. Y., Xiang, Z. C., et al. (2021b). APC-activated long noncoding RNA inhibits colorectal carcinoma pathogenesis through reduction of exosome production. J. Clin. Invest. 131, e149666. doi:10.1172/JCI149666
Wang, H., Wang, L., Zhou, X., Luo, X., Liu, K., Jiang, E., et al. (2020b). OSCC exosomes regulate miR-210-3p targeting EFNA3 to promote oral cancer angiogenesis through the PI3K/AKT pathway. Biomed. Res. Int. 2020, 2125656. doi:10.1155/2020/2125656
Wang, M., Zhao, Y., Yu, Z. Y., Zhang, R. D., Li, S. A., Zhang, P., et al. (2020a). Glioma exosomal microRNA-148a-3p promotes tumor angiogenesis through activating the EGFR/MAPK signaling pathway via inhibiting ERRFI1. Cancer Cell Int. 20, 518. doi:10.1186/s12935-020-01566-4
Wang, Q., Wang, G., Niu, L., Zhao, S., Li, J., Zhang, Z., et al. (2021a). Exosomal MiR-1290 promotes angiogenesis of hepatocellular carcinoma via targeting SMEK1. J. Oncol. 2021, 6617700. doi:10.1155/2021/6617700
Wang, Y., Han, D., Pan, L., and Sun, J. (2018a). The positive feedback between lncRNA TNK2-AS1 and STAT3 enhances angiogenesis in non-small cell lung cancer. Biochem. Biophys. Res. Commun. 507, 185–192. doi:10.1016/j.bbrc.2018.11.004
Wang, Y., Zhang, F., Wang, J., Hu, L., Jiang, F., Chen, J., et al. (2018b). lncRNA LOC100132354 promotes angiogenesis through VEGFA/VEGFR2 signaling pathway in lung adenocarcinoma. Cancer Manag. Res. 10, 4257–4266. doi:10.2147/CMAR.S177327
Wang, Z. F., Liao, F., Wu, H., and Dai, J. (2019). Glioma stem cells-derived exosomal miR-26a promotes angiogenesis of microvessel endothelial cells in glioma. J. Exp. Clin. Cancer Res. 38, 201. doi:10.1186/s13046-019-1181-4
Wu, D., Deng, S., Li, L., Liu, T., Zhang, T., Li, J., et al. (2021). TGF-β1-mediated exosomal lnc-MMP2-2 increases blood-brain barrier permeability via the miRNA-1207-5p/EPB41L5 axis to promote non-small cell lung cancer brain metastasis. Cell death Dis. 12, 721. doi:10.1038/s41419-021-04004-z
Wu, X. G., Zhou, C. F., Zhang, Y. M., Yan, R. M., Wei, W. F., Chen, X. J., et al. (2019). Cancer-derived exosomal miR-221-3p promotes angiogenesis by targeting THBS2 in cervical squamous cell carcinoma. Angiogenesis 22, 397–410. doi:10.1007/s10456-019-09665-1
Xu, Z., Chen, Y., Ma, L., Chen, Y., Liu, J., Guo, Y., et al. (2022). Role of exosomal non-coding RNAs from tumor cells and tumor-associated macrophages in the tumor microenvironment. Mol. Ther. J. Am. Soc. Gene Ther. 30, 3133–3154. doi:10.1016/j.ymthe.2022.01.046
Yan, W., Wang, Y., Chen, Y., Guo, Y., Li, Q., and Wei, X. (2021). Exosomal miR-130b-3p promotes progression and tubular formation through targeting PTEN in oral squamous cell carcinoma. Front. Cell Dev. Biol. 9, 616306. doi:10.3389/fcell.2021.616306
Yang, E., Wang, X., Gong, Z., Yu, M., Wu, H., Zhang, D. J. S. t., et al. (2020). Exosome-mediated metabolic reprogramming: The emerging role in tumor microenvironment remodeling and its influence on cancer progression. Signal Transduct. Target. Ther. 5, 242. doi:10.1038/s41392-020-00359-5
Yang, J., and Teng, Y. (2023). Harnessing cancer stem cell-derived exosomes to improve cancer therapy. J. Exp. Clin. cancer Res. CR 42, 131. doi:10.1186/s13046-023-02717-x
Yang, K., Zhou, Q., Qiao, B., Shao, B., Hu, S., Wang, G., et al. (2022). Exosome-derived noncoding RNAs: Function, mechanism, and application in tumor angiogenesis. Nucleic acids. 27, 983–997. doi:10.1016/j.omtn.2022.01.009
Yao, C., Wu, S., Kong, J., Sun, Y., Bai, Y., Zhu, R., et al. (2023). Angiogenesis in hepatocellular carcinoma: Mechanisms and anti-angiogenic therapies. Cancer Biol. Med. 20, 25–43. doi:10.20892/j.issn.2095-3941.2022.0449
Yin, Z., Ma, T., Huang, B., Lin, L., Zhou, Y., Yan, J., et al. (2019). Macrophage-derived exosomal microRNA-501-3p promotes progression of pancreatic ductal adenocarcinoma through the TGFBR3-mediated TGF-β signaling pathway. J. Exp. Clin. Cancer Res. 38, 310–320. doi:10.1186/s13046-019-1313-x
Yokota, Y., Noda, T., Okumura, Y., Kobayashi, S., Iwagami, Y., Yamada, D., et al. (2021). Serum exosomal miR-638 is a prognostic marker of HCC via downregulation of VE-cadherin and ZO-1 of endothelial cells. Cancer Sci. 112, 1275–1288. doi:10.1111/cas.14807
You, X., Sun, W., Wang, Y., Liu, X., Wang, A., Liu, L., et al. (2021). Cervical cancer-derived exosomal miR-663b promotes angiogenesis by inhibiting vinculin expression in vascular endothelial cells. Cancer Cell Int. 21, 684. doi:10.1186/s12935-021-02379-9
Zampetaki, A., Albrecht, A., and Steinhofel, K. (2018). Long non-coding RNA structure and function: Is there a link? Front. physiology 9, 1201. doi:10.3389/fphys.2018.01201
Zeng, Z., Li, Y., Pan, Y., Lan, X., Song, F., Sun, J., et al. (2018). Cancer-derived exosomal miR-25-3p promotes pre-metastatic niche formation by inducing vascular permeability and angiogenesis. Nat. Commun. 9, 5395. doi:10.1038/s41467-018-07810-w
Zhang, C. Y., Yu, M. S., Li, X., Zhang, Z., Han, C. R., and Yan, B. (2017c). Overexpression of long non-coding RNA MEG3 suppresses breast cancer cell proliferation, invasion, and angiogenesis through AKT pathway. Tumour Biol. 39, 1010428317701311. doi:10.1177/1010428317701311
Zhang, J. W., Liu, T. F., Chen, X. H., Liang, W. Y., Feng, X. R., Wang, L., et al. (2017b). Validation of aspirin response-related transcripts in patients with coronary artery disease and preliminary investigation on CMTM5 function. Gene 624, 56–65. doi:10.1016/j.gene.2017.04.041
Zhang, J. X., Chen, Z. H., Chen, D. L., Tian, X. P., Wang, C. Y., Zhou, Z. W., et al. (2018). LINC01410-miR-532-NCF2-NF-kB feedback loop promotes gastric cancer angiogenesis and metastasis. Oncogene 37, 2660–2675. doi:10.1038/s41388-018-0162-y
Zhang, Z. C., Tang, C., Dong, Y., Zhang, J., Yuan, T., Tao, S. C., et al. (2017a). Targeting the long noncoding RNA MALAT1 blocks the pro-angiogenic effects of osteosarcoma and suppresses tumour growth. Int. J. Biol. Sci. 13, 1398–1408. doi:10.7150/ijbs.22249
Zhao, J., Du, P., Cui, P., Qin, Y., Hu, C., Wu, J., et al. (2018). LncRNA PVT1 promotes angiogenesis via activating the STAT3/VEGFA axis in gastric cancer. Oncogene 37, 4094–4109. doi:10.1038/s41388-018-0250-z
Zhao, S., Li, J., Zhang, G., Wang, Q., Wu, C., Zhang, Q., et al. (2019). Exosomal miR-451a functions as a tumor suppressor in hepatocellular carcinoma by targeting LPIN1. Cell Physiol. Biochem. 53, 19–35. doi:10.33594/000000118
Zhao, Z., Sun, W., Guo, Z., Zhang, J., Yu, H., and Liu, B. (2020). Mechanisms of lncRNA/microRNA interactions in angiogenesis. Life Sci. 254, 116900. doi:10.1016/j.lfs.2019.116900
Zhong, M. E., Chen, Y., Zhang, G., Xu, L., Ge, W., and Wu, B. (2019). LncRNA H19 regulates PI3K-akt signal pathway by functioning as a ceRNA and predicts poor prognosis in colorectal cancer: Integrative analysis of dysregulated ncRNA-associated ceRNA network. Cancer Cell Int. 19, 148. doi:10.1186/s12935-019-0866-2
Zhou, C. F., Ma, J., Huang, L., Yi, H. Y., Zhang, Y. M., Wu, X. G., et al. (2019). Cervical squamous cell carcinoma-secreted exosomal miR-221-3p promotes lymphangiogenesis and lymphatic metastasis by targeting VASH1. Oncogene 38, 1256–1268. doi:10.1038/s41388-018-0511-x
Zhou, C., Zhang, Y., Yan, R., Huang, L., Mellor, A. L., Yang, Y., et al. (2021). Exosome-derived miR-142-5p remodels lymphatic vessels and induces Ido to promote immune privilege in the tumour microenvironment. Cell Death Differ. 28, 715–729. doi:10.1038/s41418-020-00618-6
Zhou, S. K., Gao, F., Zhong, Z. S., and Yao, H. (2020). Long non-coding RNA colon cancer associated transcript-2 from nasopharyngeal carcinoma-derived exosomes promotes angiogenesis. Zhonghua Er Bi Yan Hou Tou Jing Wai Ke Za Zhi 55, 944–951. doi:10.3760/cma.j.cn115330-20200423-00322
Zhou, W., Fong, M. Y., Min, Y., Somlo, G., Liu, L., Palomares, M. R., et al. (2014). Cancer-secreted miR-105 destroys vascular endothelial barriers to promote metastasis. Cancer Cell 25, 501–515. doi:10.1016/j.ccr.2014.03.007
Zhu, H. Y., Gao, Y. J., Wang, Y., Liang, C., Zhang, Z. X., and Chen, Y. (2021). LncRNA CRNDE promotes the progression and angiogenesis of pancreatic cancer via miR-451a/CDKN2D axis. Transl. Oncol. 14, 101088. doi:10.1016/j.tranon.2021.101088
Keywords: exosomes, endothelial cells, exosomes-derived non-coding RNAs, tumorassociated angiogenesis, tumor microenvironment, lncRNA, miRNA, cancer
Citation: Duan S-L, Fu W-J, Jiang Y-K, Peng L-S, Ousmane D, Zhang Z-J and Wang J-P (2023) Emerging role of exosome-derived non-coding RNAs in tumor-associated angiogenesis of tumor microenvironment. Front. Mol. Biosci. 10:1220193. doi: 10.3389/fmolb.2023.1220193
Received: 10 May 2023; Accepted: 27 July 2023;
Published: 04 August 2023.
Edited by:
Arun Malhotra, University of Miami, United StatesReviewed by:
Nahid Arghiani, Stockholm University, SwedenYu-Ping Yang, University of Miami, United States
Copyright © 2023 Duan, Fu, Jiang, Peng, Ousmane, Zhang and Wang. This is an open-access article distributed under the terms of the Creative Commons Attribution License (CC BY). The use, distribution or reproduction in other forums is permitted, provided the original author(s) and the copyright owner(s) are credited and that the original publication in this journal is cited, in accordance with accepted academic practice. No use, distribution or reproduction is permitted which does not comply with these terms.
*Correspondence: Zhe-Jia Zhang, emhhbmd6aGVqaWFAY3N1LmVkdS5jbg==; Jun-Pu Wang, d2FuZy1qcDIwMTNAY3N1LmVkdS5jbg==