- 1Shahid Beheshti University of Medical Sciences, Tehran, Iran
- 2Department of Medical Genetics, Tehran University of Medical Sciences, Tehran, Iran
- 3Department of Pharmacognosy, College of Pharmacy, Hawler Medical University, Erbil, Iraq
- 4Department of Medical Genetics, Shahid Beheshti University of Medical Sciences, Tehran, Iran
Ferroptosis is a kind of cell death which has distinctive features differentiating it from autophagy, necrosis and apoptosis. This iron-dependent form of cell death is described by an increase in lipid reactive oxygen species, shrinkage of mitochondria and decrease in mitochondrial cristae. Ferroptosis is involved in the initiation and progression of many diseases and is regarded as a hotspot of investigations on treatment of disorders. Recent studies have shown that microRNAs partake in the regulation of ferroptosis. The impact of microRNAs on this process has been verified in different cancers as well as intervertebral disc degeneration, acute myocardial infarction, vascular disease, intracerebral hemorrhage, preeclampsia, hemorrhagic stroke, atrial fibrillation, pulmonary fibrosis and atherosclerosis. miR-675, miR-93, miR-27a, miR-34a and miR-141 have been shown to affect iron metabolism, antioxidant metabolism and lipid metabolism, thus influencing all pivotal mechanisms in the ferroptosis process. In the current review, we summarize the role of microRNAs in ferroptosis and their involvement in the pathetiology of malignant and non-malignant disorders.
Introduction
As a newly recognized kind of cell type, ferroptosis is associated with accumulation of large amounts of iron accumulation and lipid peroxidation during the process of cell death (Li J. et al., 2020). This concept has been firstly proposed by Dixon et al. as an iron-dependent way of cell death described by an increase in lipid reactive oxygen species (ROS) (Dixon et al., 2012). It has several distinctive features that distinguishes this mode of cell death from autophagy, necrosis and apoptosis (Dixon et al., 2012; Xie et al., 2016). Lack of swelling of the cytoplasm and cell organelles and absence of cell membrane splitting differentiate ferroptosis from necrosis. Moreover, absence of cell shrinkage and chromatin condensation, lack of establishment of apoptotic bodies and absence of cytoskeleton breakdown differentiate ferrptosis from apoptosis. Finally, the typical closed bilayer membrane organizations which are produced during autophagy are never seen in ferroptosis (Li J. et al., 2020). From a morphological point of view, ferroptosis is characterized by shrinkage of mitochondria and decrease in mitochondrial cristae, features which are not seen in other types of cell death (Yagoda et al., 2007; Yang and Stockwell 2008; Dixon et al., 2012). Ballooning is a specific phenotype acquired by cells during ferroptosis, defined by the establishment of a clear, rounded cell chiefly consisting of empty cytosol (Battaglia et al., 2020). Notably, during the process of ferroptosis the cell membrane is not affected, the size of nucleus is not changed, and chromatin is not condensed. However, the intracellular content of glutathione (GSH) is depleted and activity of glutathione peroxidase 4 (GPX4) is decreased. Thus, the metabolism of lipid peroxides by GPX4 is impaired. Subsequently, oxidation lipids of by Fe2+ in a Fenton-like mode results in production of massive quantities of ROS, which induces ferroptosis (Yang and Stockwell 2008; Angeli et al., 2014). Ferroptosis is regulated by several genetic factors, most of them being involved in modulation of iron homeostasis and lipid peroxidation (Li J. et al., 2020).
Ferroptosis happens through two main routes, i.e., the extrinsic and the intrinsic pathways. While the former is called the transporter-dependent pathway, the latter is regulated by enzymes. This process is initiated by a redox imbalance between synthesis of oxidants and antioxidants due to the abnormalities in the expressions and activities of several redox-active enzymes that synthetize or detoxicate free radicals and products of oxidation of lipids. Therefore, ferroptosis is finely regulated at several phases. This ROS-associated kind of cell death is related with two major biochemical features, i.e., iron buildup and lipid peroxidation (Tang et al., 2021).
Ferroptosis is involved in the initiation and progression of many diseases and is regarded as a hotspot of investigations on treatment of disorders (Li J. et al., 2020). Recent studies have shown that microRNAs (miRNAs) partake in the regulation of ferroptosis. The impact of miRNAs on this process has been verified in different cancers as well as non-malignant conditions. In the current review, we summarize the role of miRNAs in ferroptosis and their involvement in the pathetiology of malignant and non-malignant disorders.
miRNAs effect on ferroptosis in cancers
miRNAs are a group of small-sized non-coding transcripts that can specifically bind with their target transcripts and induce its degradation or inhibit its translation. Through regulating several biological processes, these transcripts have fundamental roles in the process of development and cellular homeostasis (Liu et al., 2014). They can also affect expression of genes which has role in iron metabolism (Zolea et al., 2017). An experiment in melanoma cell lines has shown the role of miR-9 regulation of ferroptosis through influencing expression of GOT1. This miRNA could suppress expression of GOT1 through binding to 3′-UTR of GOT1 transcript. This binding leads to reduction of erastin- and RSL3-associated ferroptosis. On the other hand, miR-9 silencing could increase the response of neoplastic cells to erastin and RSL3. Moreover, the impact of miR-9 suppression in accumulation of lipid ROS and induction of ferroptosis can be abolished by suppression of glutaminolysis process (Zhang et al., 2018).
The Kaposi’s sarcoma herpes virus (KSHV)-encoded miRNAs have been shown to enhance expression of xCT which encodes a membrane-associated amino acid transporter. This process is mainly accomplished via inhibition of BACH-1, a modulator of transcription which recognizes antioxidant response elements within promoter regions. Enhancement of xCT expression by KSHV miRNAs has an important role in promotion of cell permissiveness for KSHV infection and protection of infected cells from reactive nitrogen species-induced cell death (Qin et al., 2010).
miR-17* is another miRNA that participate in the pathogenesis of cancers through influencing ferroptosis. This miRNA can inhibit activity of a number of enzymes participating in mitochondrial antioxidant pathways, namely manganese superoxide dismutase (MnSOD), GPX2 and thioredoxin reductase-2 (TrxR2). Forced up-regulation of miR-17* in PC-3 cells has decreased expressions of these antioxidant proteins through binding to their 3′-UTR. Cumulatively, miR-17* can inhibit prostate carcinogenesis via suppression of mitochondrial antioxidant enzymes (Xu et al., 2010a). Another experiment in prostate cancer has shown downregulation of mitochondrial antioxidant enzymes by miR-17-3p and subsequent enhancement of sensitivity of these cells to radiation (Xu et al., 2018).
Another study in multiple myeloma has shown that miR-17-5p regulates expression of the iron exporter ferroportin (FPN1), promote cell proliferation, enhance cell cycle progression, and suppress apoptosis. Expression of miR-17-5p is suppressed by the transcription factor Nrf2. Nrf2 also decreases FPN1 expression and enhanced accumulation of iron and production of ROS in the cells (Kong et al., 2019).
miR-18a is another miRNA which is involved in the regulation of ferroptosis. This miRNA has been shown to suppress expression of ALOXE3 in glioblastoma cells. Besides, ALOXE3 knock-down has enhanced secretion of 12-HETE from glioblastoma cells, decreasing migration of these cells through activation of GsPCR/PI3K/Akt axis (Yang X. et al., 2021).
miR-20a has also been shown to regulate expression of FPN through binding to its 3′-UTR. Experiments in lung cancer cells have shown that down-regulation of FPN increases cell proliferation and colony formation, most probably through enhancing iron accessibility for neoplastic cells (Babu and Muckenthaler 2016).
In liver cancer cells, miR-18a has been shown to reduce expression of GCLC- a gene that regulates biosynthesis of glutathione. miR-18a also reduces GSH levels in tumor tissues (Anderton et al., 2017). Moreover, in this type of cancer, miR-22 targets TfR1 and inhibits cell cycle progression and growth (Greene et al., 2013). Besides, miR-152 is another miRNA that regulates ferroptosis in liver cancer cells (Huang et al., 2010). Another experiment in liver cancer cells shows the role of miR-503 in reduction of intracellular levels of SOD and glutathione (Wang et al., 2014).
The role of miRNAs in the regulation of ferroptosis has also been assessed in colorectal cancer cells. In this type of cancer, miR-24-2 levels has been inversely correlated with the levels of superoxide dismutase (SOD) (He et al., 2018). Moreover, induction of ROS by GT-094 has been found to be correlated with modulation of the miR-27a:ZBTB10-Sp1/Sp3/Sp4 axis (Pathi et al., 2011). miR-145 and miR-149 are two other miRNAs that affect expression of TFR1 and DMT1 in colorectal cancer cells (Hamara et al., 2013).
Lung cancer is another type of cancer in which the role of miRNAs in the regulation of ferroptosis has been vastly investigated. For instance, miR-155 silencing has been shown to inhibit GST-π expression in A549/dox cells. miR-155 induces doxorubicin resistance via modulation of drug transportation and drug metabolism (Lv et al., 2016). miR-196a is another miRNA that has an indirect effect on ferrptosis. Suppression of this miRNA has suppressed stem cell self-renewal capacity, tumor growth and tumorigenicity through enhancement of expression of GPX3 (Liu et al., 2019). Moreover, miR-302a-3p has been found to induce ferroptosis in lung cancer cells via targeting ferroportin (Wei et al., 2021). Besides, miR-324-3p enhances cisplatin-induced ferroptosis in lung cancer cells (Deng et al., 2021).
Therefore, the effects of miRNAs on ferroptosis can be regarded as a mechanism for induction/prevention of different malignancies. Moreover, modulation of expression of ferroptosis-related miRNAs can be regarded as a potential treatment strategy for cancers. Table 1 shows the role of miRNAs in the regulation of ferroptosis in cancers.
Cumulatively, miRNAs participating in the regulation of iron metabolism, antioxidant metabolism and lipid metabolism are associated with ferroptosis process (Luo et al., 2021a). We have constructed the network between these miRNAs using the Cytoscape software. Five miRNAs, namely miR-675, miR-93, miR-27a, miR-34a and miR-141 have been found to be involved in these three metabolic pathways (Figure 1). Pre-miR-675 is produced by lncRNA H19. FTH1 silencing upregulates expressions of H19 and its cognate miR-675. Activation of H19/miR-675 participates in the FTH1 silencing-related alterations in iron metabolism (Di Sanzo et al., 2018). miR-93 regulates expression of NRF2 and has a role in breast carcinogenesis (Singh et al., 2013). miR-27a directly inhibits expression of SCD1 (Drayton et al., 2014). miR-34a directly suppresses expression of ACSL4 (Jiang et al., 2020). Finally, miR-141 inhibits Nrf2 signaling through targeting Keap1 (Wu et al., 2018).
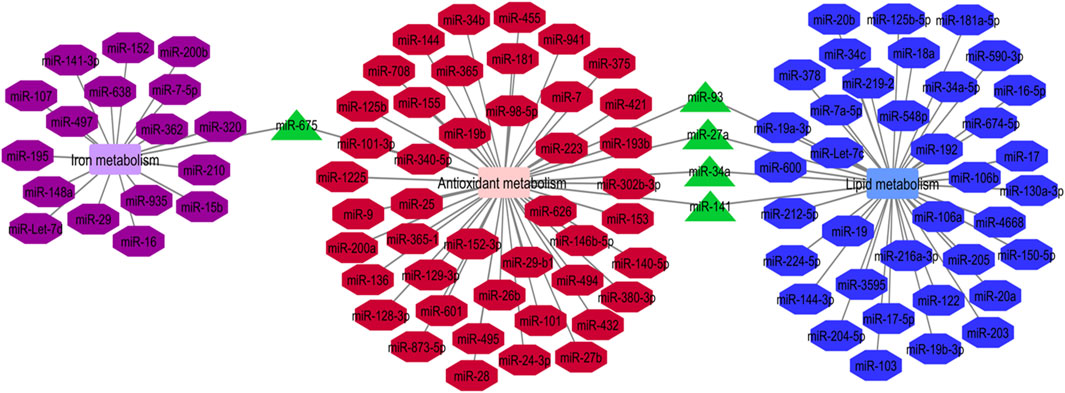
FIGURE 1. Iron metabolism, antioxidant metabolism and lipid metabolism are the pivotal mechanisms in the ferroptosis process. The mentioned metabolisms-related miRNAs network was represented by Cytoscape software. The common miRNAs (miR-675, miR-93, miR-27a, miR-34a and miR-141) in these three networks with the most degree and betweenness centrality as the key miRNAs are shown by green triangles (Luo et al., 2021a).
miRNAs effects on ferroptosis in non-malignant conditions
miRNAs have important roles in the ferroptosis in non-malignant conditions. Parkinson’s disease is an example of disorders in which the role of miRNAs in the regulation of ferroptosis has been assessed. An experiment in this field has shown down-regulation of GPX4 in the animal model of this disorder in association with down-regulation of FTH1 and over-expression of miR-335. miR-335 mimic could decrease expression of FTH1, increase ferroptosis and facilitate progression of Parkinson’s disease. Mechanistically, miR-335 targets 3′-UTR of FTH1. FTH1 silencing in 6-OHDA-induced cells has increased the pro-ferroptosis impact of miR-335 and promoted pathologic events in the course of Parkinson’s disease. In fact, miR-335 enhances ferroptosis via reduction of FTH1 and subsequent enhancement of iron release, lipid peroxidation and ROS buildup, while decreasing mitochondrial membrane potential (Li X. et al., 2021). Figure 2 depicts this process.
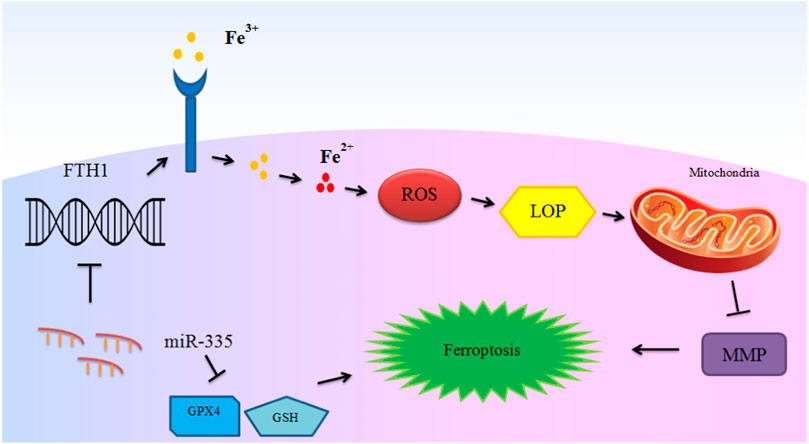
FIGURE 2. The mechanism of ferroptosis induction in Parkinson’s disease by miR-335. miR-335 targets FTH1 and degrades it to promote iron release, lipid peroxidation and reactive oxygen species (ROS) accumulation, and decreases mitochondrial membrane potential (MMP) and intensify ferroptosis and PD pathology. Although glutathione peroxidase 4 (GPX4) is not directly targeted by miR-335, up-regulation of miR-335 also leads to reduction of the levels of this ferroptosis marker protein (Li X. et al., 2021).
Another experiment has shown aberrant expression of IL-6 and its receptor in cartilage samples of patients with intervertebral disc degeneration. Notably, IL-6 could down-regulate expression of miR-10a-5p, leading to derepression of IL-6R expression. IL-6 has a role in induction of ferroptosis in cartilage cells through stimulating oxidative stress and upsetting iron homeostasis. Up-regulation of miR-10a-5p could decrease IL-6R levels and attenuate IL-6-associated ferroptosis to some extent (Bin et al., 2021).
Expression of miR-15a-5p has been shown to be increased in acute myocardial infarction. miR-15a-5p silencing has decreased mortality of myocardial cells in hypoxic conditions. Notably, GPX4 has been identified as the direct target of miR-15a-5p. Up-regulation of miR-15a-5p has enhanced ferroptosis and intensified myocardial cell damage during hypoxia. Knock-down of the transcription factor Egr-1 has led to down-regulation of miR-15a-5p, and subsequent up-regulation of GPX4, which results in reduction of ferroptosis and alleviation of myocardial damage (Fan et al., 2021).
miR-17-92 is another miRNA which modulates ferroptosis. This miRNA has been shown to protect endothelial cells from erastin-associated ferroptosis. In fact, over-expression of miR-17-92 can reduce erastin-associated growth suppression and ROS production in endothelial cells. Mechanistically, miR-17-92 exerts its effects through suppression of Zinc lipoprotein A20 expression. miR-17-92 up-regulation or A20 suppression has enhanced expression of ACSL4 endothelial cells (Xiao F. J. et al., 2019).
Ferroptosis in animal model of intracerebral hemorrhage has been associated with reduced levels of miR-19b-3p and enhancement of IRP2 levels. Expression of IRP2 as a direct target of miR-19b-3p has been suppressed by miR-19b-3p mimic-transfected adipose-derived stem cells. These exosomes could also attenuate hemin-associated cell damage and ferroptosis, thus improving neurologic function in the effected animals (Yi and Tang 2021). Taken together, ferroptosis-associated miRNAs are involved in the pathogenesis of a variety of non-malignant conditions, such as intervertebral disc degeneration, acute myocardial infarction, vascular diseases, intracerebral hemorrhage and preeclampsia. Table 2 shows the role of miRNAs in regulation of ferroptosis in non-malignant conditions.
Effects of different treatments on expression of ferroptosis-associated miRNAs
A number of drugs and treatments have been found to influence course of disorders through affecting expression of ferroptosis-associated miRNAs. For instance, experiments in animal models of intracerebral hemorrhage have shown that acupuncture can amend neuron cells death, inflammatory responses, and ferroptosis through downregulation of miR-23a-3p. The effects of acupuncture on alleviation of ferroptosis and reduction of miR-23a-3p expression have been verified by the observed enhancemnet of nuclear translocation of NFE2L2 and expression of heme oxygenase-1 and glutathione peroxidase 4 as well as reduction of iron and malondialdehyde levels and decrease in the accumulation of reactive oxygen species. Furthermore, antagomiR-23a-3p could inhibit the intracerebral hemorrhage-induced enhancemnet of Fluoro-Jade B-positive cells, production of proinflammatory cytokines, ferroptosis, and activity of NFE2L2. Mechanistically, miR-23a-3p has binding site on NFE2L2 (Kong et al., 2021). On the other hand, isorhynchophylline has been shown to ameliorate ferroptosis-associated nerve injury in the context of intracerebral hemorrhage through modulation of miR-122-5p (Zhao et al., 2021). Experiments in mouse hippocampal HT-22 cells exposed to ferric ammonium citrate alone or together with Isorhynchophylline have shown that Isorhynchophylline reduces the ferric ammonium citrate-associated cell injury. Isorhynchophylline also reduces the ferric ammonium citrate-induced reduction of miR-122-5p expression and ameliorates ferroptosis. Besides, miR-122-5p inhibitor could diminish the protective effect of Isorhynchophylline against ammonium citrate-associated ferroptosis in these cells. Mechanistically, miR-122-5p targets TP53, and restoration of TP53 attenuates the effect of miR-122-5p on ferroptotic markers and expression of SLC7A11. Taken together, miR-122-5p/TP53/SLC7A11 axis has been suggested as a potential mechanism in the etiology of intracranial hemorrhage (Zhao et al., 2021).
Moreover, metformin can induce ferroptosis of breast cancer cells through influencing expression of the GPX4 targeting miRNA miR-324-3p. Up-regulation of miR-324-3p has suppressed viability of breast cancer cells. In fact, metformin can be regarded as a potential anti-cancer agent via activation of ferroptosis (Hou et al., 2021). Similarly, lidocaine and levobupivacaine enhance ferroptosis of cancer cells through targeting miR-382-5p (Sun D. et al., 2021) and miR-489-3p (Mao et al., 2021), respectively.
Table 3 shows the effects of different treatments on expression of ferroptosis-associated miRNAs.
Discussion
Several miRNAs have been found to affect ferroptosis through binding with 3′-UTR of genes participating in this process. GOT1, GPX2, GPX3, GPX4, FPN, GSH, GST, FTL, TFR1 and NRF2 are examples of ferroptosis-associated molecules which are regulated by miRNAs. Moreover, a number of miRNAs can affect ferroptosis through indirect routes. For instance, miR-152 can reduce expression of DNA methyltransferase one leading to global DNA hypomethylation and enhancement of expression of GSTP1 (Huang et al., 2010).
Since ferroptosis can eradicate cancer cells in an independent way from apoptosis (Zhang X. et al., 2020), identification of the role of miRNAs in this process can propose new ways for combatting cancer progression. It is worth mentioning that some of above-mentioned miRNAs that regulate ferroptosis, have additional roles in the regulation of other types of cell death. Thus, these miRNAs can induce cancer cell death from different routes.
Bioinformatics tools have facilitated identification of miRNAs with highest involvement in the ferroptosis, thus proposing the most appropriate targets for management of ferroptosis-associated disorders.
A number of long non-coding RNAs and circRNAs have been found to affect expression of ferroptosis-related miRNAs. CircRHOT1, circABCB10, circRNA_000479, circFNDC3B, circIL4R, circ_0067934, circ-TTBK2, circ_0007142, circ_0013731, circKIF4A, circ0097009, lncOIP5-AS1, lncMT1DP and lncMEG8 have been recognized as competing endogenous RNAs for miRNAs that partake in the ferroptosis. Therefore, ferroptosis can be regulated by several members of non-coding RNAs.
Notably, acupuncture and a number of drugs such as physcion 8-O-β-glucopyranoside, isorhynchophylline, metformin, lidocaine and levobupivacaine have been shown to affect ferroptosis through modulation of miRNAs. Thus, identification of the role of miRNAs in the regulation of ferroptosis can facilitate design of novel therapeutic agents for treatment of diverse neoplastic or neurodegenerative disorders.
Based on the vast impact of ferroptosis on development of disorders, therapies targeting this process can be proposed as treatment modalities for several disorders including neoplastic and neurodegenerative disorders. Manipulation of expression of ferroptosis-associated miRNAs through different methods is regarded as a potential strategy to affect ferroptosis and intervene with the pathoetiology of mentioned disorders. Since ferroptosis might have opposite effects on the physiology of organs, context-based strategies are needed in this regard. Other issues that should be addressed before incorporation of miRNA-based therapies in the clinical settings are identification of safe and efficient methods for delivery of these kinds of therapies into the specific cells and monitoring the cellular response to these modalities.
Ferroptosis-related miRNAs can alter response of cancer cells to chemotherapeutic modalities. Therefore, manipulation of expression of these miRNAs not only affects the progression and evolution of cancer, but also influences the response to a variety of treatment options. In spite of extensive research on effectiveness of these modalities in cancer cell lines and animal models, there is no clinical trial for appraisal of these methods in the clinical settings. However, it is expected that combination of miRNA-based therapies with conventional or targeted anti-cancer therapies enhances the effectiveness of these therapies. Since cancer cells are heterogeneous in terms of miRNAs signature, it is necessary to have a miRNA profile for each patient before implementation of these novel methods in the clinical settings.
Author contributions
SG-F wrote the manuscript and revised it. SK, RM-L, and BH collected the data and designed the figures and tables. All authors read and approved the submitted version.
Funding
This study was financially supported by Grant from Medical School of Shahid Beheshti University of Medical Sciences.
Conflict of interest
The authors declare that the research was conducted in the absence of any commercial or financial relationships that could be construed as a potential conflict of interest.
Publisher’s note
All claims expressed in this article are solely those of the authors and do not necessarily represent those of their affiliated organizations, or those of the publisher, the editors and the reviewers. Any product that may be evaluated in this article, or claim that may be made by its manufacturer, is not guaranteed or endorsed by the publisher.
Abbreviations
reactive oxygen species; (ROS), glutathione peroxidase 4; (GPX4), Kaposi’s sarcoma herpes virus; (KSHV), manganese superoxide dismutase; (MnSOD), thioredoxin reductase-2; (TrxR2), ferroportin; (FPN1), glutamic-oxaloacetic transaminase 1; (GOT1), Glutathione; (GSH), glutathione S-transferase; (GST), Fms-like tyrosine kinase 3; (FTL3), transferrin receptor 1; (TFR1), Nuclear factor erythroid 2-related factor 2; (NRF2).
References
Al-Kafaji, G., Al-Mahroos, G., Abdulla Al-Muhtaresh, H., Sabry, M. A., Abdul Razzak, R., and Salem, A. H. (2017). Circulating endothelium-enriched microRNA-126 as a potential biomarker for coronary artery disease in type 2 diabetes mellitus patients. Biomarkers 22 (3-4), 268–278. doi:10.1080/1354750X.2016.1204004
Anderton, B., Camarda, R., Balakrishnan, S., Balakrishnan, A., Kohnz, R. A., Lim, L., et al. (2017). MYC-driven inhibition of the glutamate-cysteine ligase promotes glutathione depletion in liver cancer. EMBO Rep. 18 (4), 569–585. doi:10.15252/embr.201643068
Angeli, J. P. F., Schneider, M., Proneth, B., Tyurina, Y. Y., Tyurin, V. A., Hammond, V. J., et al. (2014). Inactivation of the ferroptosis regulator Gpx4 triggers acute renal failure in mice. Nat. Cell Biol. 16 (12), 1180–1191. doi:10.1038/ncb3064
Babu, K. R., and Muckenthaler, M. U. (2016). miR-20a regulates expression of the iron exporter ferroportin in lung cancer. J. Mol. Med. 94 (3), 347–359. doi:10.1007/s00109-015-1362-3
Bai, T., Liang, R., Zhu, R., Wang, W., Zhou, L., and Sun, Y. (2020). MicroRNA-214-3p enhances erastin-induced ferroptosis by targeting ATF4 in hepatoma cells. J. Cell Physiol. 235 (7-8), 5637–5648. doi:10.1002/jcp.29496
Bao, C., Zhang, J., Xian, S. Y., and Chen, F. (2021). MicroRNA-670-3p suppresses ferroptosis of human glioblastoma cells through targeting ACSL4. Free Radic. Res. 55, 853–864. doi:10.1080/10715762.2021.1962009
Bao, W. D., Zhou, X. T., Zhou, L. T., Wang, F., Yin, X., Lu, Y., et al. (2020). Targeting miR-124/Ferroportin signaling ameliorated neuronal cell death through inhibiting apoptosis and ferroptosis in aged intracerebral hemorrhage murine model. Aging Cell 19 (11), e13235. doi:10.1111/acel.13235
Battaglia, A. M., Chirillo, R., Aversa, I., Sacco, A., Costanzo, F., and Biamonte, F. (2020). Ferroptosis and cancer: Mitochondria meet the “iron maiden” cell death. Cells 9 (6), 1505. doi:10.3390/cells9061505
Benassi, B., Marani, M., Loda, M., and Blandino, G. (2013). USP2a alters chemotherapeutic response by modulating redox. Cell death Dis. 4 (9), e812. doi:10.1038/cddis.2013.289
Bin, S., Xin, L., Lin, Z., Jinhua, Z., Rui, G., and Xiang, Z. (2021). Targeting miR-10a-5p/IL-6R axis for reducing IL-6-induced cartilage cell ferroptosis. Exp. Mol. Pathol. 118, 104570. doi:10.1016/j.yexmp.2020.104570
Chan, J. J., Kwok, Z. H., Chew, X. H., Zhang, B., Liu, C., Soong, T. W., et al. (2018). A FTH1 gene: Pseudogene: microRNA network regulates tumorigenesis in prostate cancer. Nucleic acids Res. 46 (4), 1998–2011. doi:10.1093/nar/gkx1248
Chekhun, V. F., Lukyanova, N. Y., Burlaka, А. P., Bezdenezhnykh, N. A., Shpyleva, S. I., Tryndyak, V. P., et al. (2013). Iron metabolism disturbances in the MCF-7 human breast cancer cells with acquired resistance to doxorubicin and cisplatin. Int. J. Oncol. 43 (5), 1481–1486. doi:10.3892/ijo.2013.2063
Chen, B., Wang, H., Lv, C., Mao, C., and Cui, Y. (2021a). Long non-coding RNA H19 protects against intracerebral hemorrhage injuries via regulating microRNA-106b-5p/acyl-CoA synthetase long chain family member 4 axis. Bioengineered 12 (1), 4004–4015. doi:10.1080/21655979.2021.1951070
Chen, W., Fu, J., Chen, Y., Li, Y., Ning, L., Huang, D., et al. (2021b). Circular RNA circKIF4A facilitates the malignant progression and suppresses ferroptosis by sponging miR-1231 and upregulating GPX4 in papillary thyroid cancer. Aging (Albany NY) 13 (12), 16500–16512. doi:10.18632/aging.203172
Chen, Y., Zhang, Z., Yang, K., Du, J., Xu, Y., and Liu, S. (2015). Myeloid zinc-finger 1 (MZF-1) suppresses prostate tumor growth through enforcing ferroportin-conducted iron egress. Oncogene 34 (29), 3839–3847. doi:10.1038/onc.2014.310
Choi, J.-Y., An, B. C., Jung, I. J., Kim, J. H., and Lee, S.-w. (2019). MiR-921 directly downregulates GPx3 in A549 lung cancer cells. Gene 700, 163–167. doi:10.1016/j.gene.2019.02.086
Deng, S. H., Wu, D. M., Li, L., Liu, T., Zhang, T., Li, J., et al. (2021). miR-324-3p reverses cisplatin resistance by inducing GPX4-mediated ferroptosis in lung adenocarcinoma cell line A549. Biochem. Biophys. Res. Commun. 549, 54–60. doi:10.1016/j.bbrc.2021.02.077
Di Sanzo, M., Chirillo, R., Aversa, I., Biamonte, F., Santamaria, G., Giovannone, E., et al. (2018). shRNA targeting of ferritin heavy chain activates H19/miR-675 axis in K562 cells. Gene 657, 92–99. doi:10.1016/j.gene.2018.03.027
Ding, C., Ding, X., Zheng, J., Wang, B., Li, Y., Xiang, H., et al. (2020). miR-182-5p and miR-378a-3p regulate ferroptosis in I/R-induced renal injury. Cell Death Dis. 11 (10), 929. doi:10.1038/s41419-020-03135-z
Dixon, S. J., Lemberg, K. M., Lamprecht, M. R., Skouta, R., Zaitsev, E. M., Gleason, C. E., et al. (2012). Ferroptosis: An iron-dependent form of nonapoptotic cell death. Cell 149 (5), 1060–1072. doi:10.1016/j.cell.2012.03.042
Dong, Z., Ren, L., Lin, L., Li, J., Huang, Y., and Li, J. (2015). Effect of microRNA-21 on multidrug resistance reversal in A549/DDP human lung cancer cells. Mol. Med. Rep. 11 (1), 682–690. doi:10.3892/mmr.2014.2662
Drayton, R. M., Dudziec, E., Peter, S., Bertz, S., Hartmann, A., Bryant, H. E., et al. (2014). Reduced expression of miRNA-27a modulates cisplatin resistance in bladder cancer by targeting the cystine/glutamate exchanger SLC7A11. Clin. cancer Res. 20 (7), 1990–2000. doi:10.1158/1078-0432.CCR-13-2805
Fan, K., Huang, W., Qi, H., Song, C., He, C., Liu, Y., et al. (2021). The Egr-1/miR-15a-5p/GPX4 axis regulates ferroptosis in acute myocardial infarction. Eur. J. Pharmacol. 909, 174403. doi:10.1016/j.ejphar.2021.174403
Fang, S., Chen, W., Ding, J., Zhang, D., Zheng, L., Song, J., et al. (2021). "Oxidative Medicine and Cellular Longevity Hsa_circ_0013731 mediated by E2F1 inhibits ferroptosis in hepatocellular carcinoma cells by sponging miR-877-3p and targeting SLC7A11."
Gai, C., Liu, C., Wu, X., Yu, M., Zheng, J., Zhang, W., et al. (2020). MT1DP loaded by folate-modified liposomes sensitizes erastin-induced ferroptosis via regulating miR-365a-3p/NRF2 axis in non-small cell lung cancer cells. Cell Death Dis. 11 (9), 751. doi:10.1038/s41419-020-02939-3
Ghanbarian, M., Afgar, A., Yadegarazari, R., Najafi, R., and Teimoori-Toolabi, L. (2018). Through oxaliplatin resistance induction in colorectal cancer cells, increasing ABCB1 level accompanies decreasing level of miR-302c-5p, miR-3664-5p and miR-129-5p. Biomed. Pharmacother. 108, 1070–1080. doi:10.1016/j.biopha.2018.09.112
Gomaa, A., Peng, D., Chen, Z., Soutto, M., Abouelezz, K., Corvalan, A., et al. (2019). Epigenetic regulation of AURKA by miR-4715-3p in upper gastrointestinal cancers. Sci. Rep. 9 (1), 16970. doi:10.1038/s41598-019-53174-6
Greene, C. M., Varley, R. B., and Lawless, M. W. (2013). MicroRNAs and liver cancer associated with iron overload: Therapeutic targets unravelled. World J. gastroenterology WJG 19 (32), 5212–5226. doi:10.3748/wjg.v19.i32.5212
Hamara, K., Bielecka-Kowalska, A., Przybylowska-Sygut, K., Sygut, A., Dziki, A., and Szemraj, J. (2013). Alterations in expression profile of iron-related genes in colorectal cancer. Mol. Biol. Rep. 40 (10), 5573–5585. doi:10.1007/s11033-013-2659-3
He, H. W., Wang, N. N., Yi, X. M., Tang, C. P., and Wang, D. (2018). Low-level serum miR-24-2 is associated with the progression of colorectal cancer. Cancer Biomarkers 21 (2), 261–267. doi:10.3233/CBM-170321
Hou, Y., Cai, S., Yu, S., and Lin, H. (2021). Metformin induces ferroptosis by targeting miR-324-3p/GPX4 axis in breast cancer. Acta Biochim. Biophys. Sin. (Shanghai) 53 (3), 333–341. doi:10.1093/abbs/gmaa180
Huang, J., Wang, Y., Guo, Y., and Sun, S. (2010). Down-regulated microRNA-152 induces aberrant DNA methylation in Hepatitis B virus–related hepatocellular carcinoma by targeting DNA methyltransferase 1. Hepatology 52 (1), 60–70. doi:10.1002/hep.23660
Jiang, X., Guo, S., Zhang, Y., Zhao, Y., Li, X., Jia, Y., et al. (2020). LncRNA NEAT1 promotes docetaxel resistance in prostate cancer by regulating ACSL4 via sponging miR-34a-5p and miR-204-5p. Cell. Signal. 65, 109422. doi:10.1016/j.cellsig.2019.109422
Kanchan, R. K., Perumal, N., Atri, P., Venkata, R. C., Thapa, I., Nasser, M. W., et al. (2021). "MiR-1253 potentiates cisplatin response in pediatric medulloblastoma by regulating ferroptosis."
Kefas, B., Comeau, L., Erdle, N., Montgomery, E., Amos, S., and Purow, B. (2010). Pyruvate kinase M2 is a target of the tumor-suppressive microRNA-326 and regulates the survival of glioma cells. Neuro-oncology 12 (11), 1102–1112. doi:10.1093/neuonc/noq080
Kindrat, I., Tryndyak, V., de Conti, A., Shpyleva, S., Mudalige, T. K., Kobets, T., et al. (2016). MicroRNA-152-mediated dysregulation of hepatic transferrin receptor 1 in liver carcinogenesis. Oncotarget 7 (2), 1276–1287. doi:10.18632/oncotarget.6004
Kong, Y., Hu, L., Lu, K., Wang, Y., Xie, Y., Gao, L., et al. (2019). Ferroportin downregulation promotes cell proliferation by modulating the Nrf2–miR-17-5p axis in multiple myeloma. Cell death Dis. 10 (9), 624. doi:10.1038/s41419-019-1854-0
Kong, Y., Li, S., Zhang, M., Xu, W., Chen, Q., Zheng, L., et al. (2021). Acupuncture ameliorates neuronal cell death, inflammation, and ferroptosis and downregulated miR-23a-3p after intracerebral hemorrhage in rats. J. Mol. Neurosci. 71 (9), 1863–1875. doi:10.1007/s12031-020-01770-x
Li, J., Cao, F., Yin, H.-l., Huang, Z.-j., Lin, Z.-t., Mao, N., et al. (2020a). Ferroptosis: Past, present and future. Cell death Dis. 11 (2), 88–13. doi:10.1038/s41419-020-2298-2
Li, L., Wang, H., Zhang, J., Chen, X., Zhang, Z., and Li, Q. (2021a). Effect of endothelial progenitor cell-derived extracellular vesicles on endothelial cell ferroptosis and atherosclerotic vascular endothelial injury. Cell Death Discov. 7 (1), 235. doi:10.1038/s41420-021-00610-0
Li, X., Si, W., Li, Z., Tian, Y., Liu, X., Ye, S., et al. (2021b). miR-335 promotes ferroptosis by targeting ferritin heavy chain 1 in in vivo and in vitro models of Parkinson's disease. Int. J. Mol. Med. 47 (4), 61–12. doi:10.3892/ijmm.2021.4894
Li, Y., Wang, J., Chen, S., Wu, P., Xu, S., Wang, C., et al. (2020b). miR-137 boosts the neuroprotective effect of endothelial progenitor cell-derived exosomes in oxyhemoglobin-treated SH-SY5Y cells partially via COX2/PGE2 pathway. Stem Cell Res. Ther. 11 (1), 330. doi:10.1186/s13287-020-01836-y
Liao, Y., Jia, X., Ren, Y., Deji, Z., Gesang, Y., Ning, N., et al. (2021). Suppressive role of microRNA-130b-3p in ferroptosis in melanoma cells correlates with DKK1 inhibition and Nrf2-HO-1 pathway activation. Hum. Cell 34 (5), 1532–1544. doi:10.1007/s13577-021-00557-5
Liu, B., Li, J., and Cairns, M. J. (2014). Identifying miRNAs, targets and functions. Briefings Bioinforma. 15 (1), 1–19. doi:10.1093/bib/bbs075
Liu, Q., Bai, W., Huang, F., Tang, J., and Lin, X. (2019). Downregulation of microRNA-196a inhibits stem cell self-renewal ability and stemness in non-small-cell lung cancer through upregulating GPX3 expression. Int. J. Biochem. Cell Biol. 115, 105571. doi:10.1016/j.biocel.2019.105571
Lu, J., Xu, F., and Lu, H. (2020). LncRNA PVT1 regulates ferroptosis through miR-214-mediated TFR1 and p53. Life Sci. 260, 118305. doi:10.1016/j.lfs.2020.118305
Luo, M., Wu, L., Zhang, K., Wang, H., Zhang, T., Gutierrez, L., et al. (2018). miR-137 regulates ferroptosis by targeting glutamine transporter SLC1A5 in melanoma. Cell Death Differ. 25 (8), 1457–1472. doi:10.1038/s41418-017-0053-8
Luo, Y., Huang, Q., He, B., Liu, Y., Huang, S., and Xiao, J. (2021a). Regulation of ferroptosis by non‑coding RNAs in the development and treatment of cancer (Review). Oncol. Rep. 45 (1), 29–48. doi:10.3892/or.2020.7836
Luo, Y., Niu, G., Yi, H., Li, Q., Wu, Z., Wang, J., et al. (2021b). Nanomedicine promotes ferroptosis to inhibit tumour proliferation in vivo. Redox Biol. 42, 101908. doi:10.1016/j.redox.2021.101908
Lv, L., An, X., Li, H., and Ma, L. (2016). Effect of miR-155 knockdown on the reversal of doxorubicin resistance in human lung cancer A549/dox cells. Oncol. Lett. 11 (2), 1161–1166. doi:10.3892/ol.2015.3995
Lyu, N., Zeng, Y., Kong, Y., Chen, Q., Deng, H., Ou, S., et al. (2021). Ferroptosis is involved in the progression of hepatocellular carcinoma through the circ0097009/miR-1261/SLC7A11 axis. Ann. Transl. Med. 9 (8), 675. doi:10.21037/atm-21-997
Ma, H., Wang, L. Y., Yang, R. H., Zhou, Y., Zhou, P., and Kong, L. (2019). Identification of reciprocal microRNA-mRNA pairs associated with metastatic potential disparities in human prostate cancer cells and signaling pathway analysis. J. Cell. Biochem. 120 (10), 17779–17790. doi:10.1002/jcb.29045
Ma, L. L., Liang, L., Zhou, D., and Wang, S. W. (2020). Neoplasma.Tumor suppressor miR-424-5p abrogates ferroptosis in ovarian cancer through targeting ACSL4
Ma, Q., Dai, X., Lu, W., Qu, X., Liu, N., and Zhu, C. (2021). Silencing long non-coding RNA MEG8 inhibits the proliferation and induces the ferroptosis of hemangioma endothelial cells by regulating miR-497-5p/NOTCH2 axis. Biochem. Biophys. Res. Commun. 556, 72–78. doi:10.1016/j.bbrc.2021.03.132
Mao, S. H., Zhu, C. H., Nie, Y., Yu, J., and Wang, L. (2021). Levobupivacaine induces ferroptosis by miR-489-3p/slc7a11 signaling in gastric cancer. Front. Pharmacol. 12, 681338. doi:10.3389/fphar.2021.681338
McCormick, R. I., Blick, C., Ragoussis, J., Schoedel, J., Mole, D. R., Young, A. C., et al. (2013). miR-210 is a target of hypoxia-inducible factors 1 and 2 in renal cancer, regulates ISCU and correlates with good prognosis. Br. J. cancer 108 (5), 1133–1142. doi:10.1038/bjc.2013.56
Moradi, M., Goodarzi, N., Faramarzi, A., Cheraghi, H., Hashemian, A. H., and Jalili, C. (2021). Melatonin protects rats testes against bleomycin, etoposide, and cisplatin-induced toxicity via mitigating nitro-oxidative stress and apoptosis. Biomed. Pharmacother. 138, 111481. doi:10.1016/j.biopha.2021.111481
Ni, H., Qin, H., Sun, C., Liu, Y., Ruan, G., Guo, Q., et al. (2021a). MiR-375 reduces the stemness of gastric cancer cells through triggering ferroptosis. Stem Cell Res. Ther. 12 (1), 325. doi:10.1186/s13287-021-02394-7
Ni, T., Chen, X., Huang, X., Pan, S., and Lu, Z. (2021b). "Inhibition of the Long non-coding RNA ZFAS1 attenuates pathogenic ferroptosis by sponging miR-150-5p to activate CCND2 against diabetic cardiomyopathy."
Pathi, S. S., Jutooru, I., Chadalapaka, G., Sreevalsan, S., Anand, S., Thatcher, G. R. J., et al. (2011). GT-094, a NO-NSAID, inhibits colon cancer cell growth by activation of a reactive oxygen species-microRNA-27a: ZBTB10-specificity protein pathway. Mol. Cancer Res. 9 (2), 195–202. doi:10.1158/1541-7786.MCR-10-0363
Qin, Z., Freitas, E., Sullivan, R., Mohan, S., Bacelieri, R., Branch, D., et al. (2010). Upregulation of xCT by KSHV-encoded microRNAs facilitates KSHV dissemination and persistence in an environment of oxidative stress. PLoS Pathog. 6 (1), e1000742. doi:10.1371/journal.ppat.1000742
Sáenz-de-Santa-María, I., Bernardo-Castiñeira, C., Secades, P., Bernaldo-de-Quirós, S., Rodrigo, J. P., Astudillo, A., et al. (2017). Clinically relevant HIF-1α-dependent metabolic reprogramming in oropharyngeal squamous cell carcinomas includes coordinated activation of CAIX and the miR-210/ISCU signaling axis, but not MCT1 and MCT4 upregulation. Oncotarget 8 (8), 13730–13746. doi:10.18632/oncotarget.14629
Singh, B., Ronghe, A. M., Chatterjee, A., Bhat, N. K., and Bhat, H. K. (2013). MicroRNA-93 regulates NRF2 expression and is associated with breast carcinogenesis. Carcinogenesis 34 (5), 1165–1172. doi:10.1093/carcin/bgt026
Singh, S., Shukla, G. C., and Gupta, S. (2015). MicroRNA regulating glutathione S-transferase P1 in prostate cancer. Curr. Pharmacol. Rep. 1 (2), 79–88. doi:10.1007/s40495-014-0009-3
Song, Y., Cai, W., and Wang, J. (2021a). Upregulation of miR-143-3p attenuates oxidative stress-mediated cell ferroptosis in cardiomyocytes with atrial fibrillation by degrading glutamic-oxaloacetic transaminase 1. Biocell 45 (3), 733–744. doi:10.32604/biocell.2021.013236
Song, Y., Wang, B., Zhu, X., Hu, J., Sun, J., Xuan, J., et al. (2021b). Human umbilical cord blood-derived MSCs exosome attenuate myocardial injury by inhibiting ferroptosis in acute myocardial infarction mice. Cell Biol. Toxicol. 37 (1), 51–64. doi:10.1007/s10565-020-09530-8
Song, Z., Jia, G., Ma, P., and Cang, S. (2021c). Exosomal miR-4443 promotes cisplatin resistance in non-small cell lung carcinoma by regulating FSP1 m6A modification-mediated ferroptosis. Life Sci. 276, 119399. doi:10.1016/j.lfs.2021.119399
Sun, A. G., Meng, F. G., and Wang, M. G. (2017). CISD2 promotes the proliferation of glioma cells via suppressing beclin-1-mediated autophagy and is targeted by microRNA-449a. Mol. Med. Rep. 16 (6), 7939–7948. doi:10.3892/mmr.2017.7642
Sun, D., Li, Y. C., and Zhang, X. Y. (2021a). Lidocaine promoted ferroptosis by targeting miR-382-5p/SLC7A11 Axis in ovarian and breast cancer. Front. Pharmacol. 12, 681223. doi:10.3389/fphar.2021.681223
Sun, K.-X., Jiao, J.-W., Chen, S., Liu, B.-L., and Zhao, Y. (2015). MicroRNA-186 induces sensitivity of ovarian cancer cells to paclitaxel and cisplatin by targeting ABCB1. J. ovarian Res. 8 (1), 80–87. doi:10.1186/s13048-015-0207-6
Sun, W., Shi, R., Guo, J., Wang, H., Shen, L., Shi, H., et al. (2021b). miR-135b-3p promotes cardiomyocyte ferroptosis by targeting GPX4 and aggravates myocardial ischemia/reperfusion injury. Front. Cardiovasc Med. 8, 663832. doi:10.3389/fcvm.2021.663832
Tang, D., Chen, X., Kang, R., and Kroemer, G. (2021). Ferroptosis: Molecular mechanisms and health implications. Cell Res. 31 (2), 107–125. doi:10.1038/s41422-020-00441-1
Tang, S., Wang, Y., Ma, T., Lu, S., Huang, K., Li, Q., et al. (2020). MiR-30d inhibits cardiomyocytes autophagy promoting ferroptosis after myocardial infarction. Panminerva Med. doi:10.23736/S0031-0808.20.03979-8
Tili, E., Michaille, J.-J., Luo, Z., Volinia, S., Rassenti, L. Z., Kipps, T. J., et al. (2012). The down-regulation of miR-125b in chronic lymphocytic leukemias leads to metabolic adaptation of cells to a transformed state. Blood, J. Am. Soc. Hematol. 120 (13), 2631–2638. doi:10.1182/blood-2012-03-415737
Tomita, K., Nagasawa, T., Kuwahara, Y., Torii, S., Igarashi, K., Roudkenar, M. H., et al. (2021). MiR-7-5p is involved in ferroptosis signaling and radioresistance thru the generation of ROS in radioresistant HeLa and SAS cell lines. Int. J. Mol. Sci. 22 (15), 8300. doi:10.3390/ijms22158300
Wang, D., Zhang, N., Ye, Y., Qian, J., Zhu, Y. U., and Wang, C. (2014). Role and mechanisms of microRNA-503 in drug resistance reversal in HepG2/ADM human hepatocellular carcinoma cells. Mol. Med. Rep. 10 (6), 3268–3274. doi:10.3892/mmr.2014.2591
Wang, H. H., Ma, J. N., and Zhan, X. R. (2021a). Circular RNA Circ_0067934 attenuates ferroptosis of thyroid cancer cells by miR-545-3p/slc7a11 signaling. Front. Endocrinol. (Lausanne) 12, 670031. doi:10.3389/fendo.2021.670031
Wang, J., Deng, B., Liu, Q., Huang, Y., Chen, W., Li, J., et al. (2020). Pyroptosis and ferroptosis induced by mixed lineage kinase 3 (MLK3) signaling in cardiomyocytes are essential for myocardial fibrosis in response to pressure overload. Cell Death Dis. 11 (7), 574. doi:10.1038/s41419-020-02777-3
Wang, Y., Chen, H., and Wei, X. (2021b). Circ_0007142 downregulates miR-874-3p-mediated GDPD5 on colorectal cancer cells. Eur. J. Clin. Invest. 51 (7), e13541. doi:10.1111/eci.13541
Wei, D., Ke, Y. Q., Duan, P., Zhou, L., Wang, C. Y., and Cao, P. (2021). MicroRNA-302a-3p induces ferroptosis of non-small cell lung cancer cells via targeting ferroportin. Free Radic. Res. 55, 821–830. doi:10.1080/10715762.2021.1947503
Wu, L., Pan, C., Wei, X., Shi, Y., Zheng, J., Lin, X., et al. (2018). lncRNA KRAL reverses 5-fluorouracil resistance in hepatocellular carcinoma cells by acting as a ceRNA against miR-141. Cell Commun. Signal. 16 (1), 47–15. doi:10.1186/s12964-018-0260-z
Wu, P., Li, C., Ye, D. M., Yu, K., Li, Y., Tang, H., et al. (2021). Circular RNA circEPSTI1 accelerates cervical cancer progression via miR-375/409-3P/515-5p-SLC7A11 axis. Aging (Albany NY) 13 (3), 4663–4673. doi:10.18632/aging.202518
Xian, Z. Y., Hu, B., Wang, T., Cai, J. L., Zeng, J. Y., Zou, Q., et al. (2020). CircABCB10 silencing inhibits the cell ferroptosis and apoptosis by regulating the miR-326/CCL5 axis in rectal cancer. Neoplasma 67 (5), 1063–1073. doi:10.4149/neo_2020_191024N1084
Xiao, F. J., Zhang, D., Wu, Y., Jia, Q. H., Zhang, L., Li, Y. X., et al. (2019a). miRNA-17-92 protects endothelial cells from erastin-induced ferroptosis through targeting the A20-ACSL4 axis. Biochem. Biophys. Res. Commun. 515 (3), 448–454. doi:10.1016/j.bbrc.2019.05.147
Xiao, X., Jiang, Y., Liang, W., Wang, Y., Cao, S., Yan, H., et al. (2019b). miR-212-5p attenuates ferroptotic neuronal death after traumatic brain injury by targeting Ptgs2. Mol. Brain 12 (1), 78. doi:10.1186/s13041-019-0501-0
Xie, Y., Hou, W., Song, X., Yu, Y., Huang, J., Sun, X., et al. (2016). Ferroptosis: Process and function. Cell Death Differ. 23 (3), 369–379. doi:10.1038/cdd.2015.158
Xu, Q., Zhou, L., Yang, G., Meng, F., Wan, Y., Wang, L., et al. (2020). CircIL4R facilitates the tumorigenesis and inhibits ferroptosis in hepatocellular carcinoma by regulating the miR-541-3p/GPX4 axis. Cell Biol. Int. 44 (11), 2344–2356. doi:10.1002/cbin.11444
Xu, Y., Fang, F., Zhang, J., Josson, S., Clair, W. H. St., and Clair, D. K. St. (2010). miR-17* suppresses tumorigenicity of prostate cancer by inhibiting mitochondrial antioxidant enzymes. PloS one 5 (12), e14356. doi:10.1371/journal.pone.0014356
Xu, Z., Zhang, Y., Ding, J., Hu, W., Tan, C., Wang, M., et al. (2018). miR-17-3p downregulates mitochondrial antioxidant enzymes and enhances the radiosensitivity of prostate cancer cells. Mol. Therapy-Nucleic Acids 13, 64–77. doi:10.1016/j.omtn.2018.08.009
Yagoda, N., von Rechenberg, M., Zaganjor, E., Bauer, A. J., Yang, W. S., Fridman, D. J., et al. (2007). RAS-RAF-MEK-dependent oxidative cell death involving voltage-dependent anion channels. Nature 447 (7146), 864–868. doi:10.1038/nature05859
Yang, H., Wu, X.-L., Wu, K.-H., Zhang, R., Ju, L.-L., Ji, Y., et al. (2016). MicroRNA-497 regulates cisplatin chemosensitivity of cervical cancer by targeting transketolase. Am. J. cancer Res. 6 (11), 2690–2699.
Yang, J., Cao, X. H., Luan, K. F., and Huang, Y. D. (2021a). Circular RNA FNDC3B protects oral squamous cell carcinoma cells from ferroptosis and contributes to the malignant progression by regulating miR-520d-5p/slc7a11 Axis. Front. Oncol. 11, 672724. doi:10.3389/fonc.2021.672724
Yang, W. S., and Stockwell, B. R. (2008). Synthetic lethal screening identifies compounds activating iron-dependent, nonapoptotic cell death in oncogenic-RAS-harboring cancer cells. Chem. Biol. 15 (3), 234–245. doi:10.1016/j.chembiol.2008.02.010
Yang, X., Liu, J., Wang, C., Cheng, K. K., Xu, H., Li, Q., et al. (2021b). miR-18a promotes glioblastoma development by down-regulating ALOXE3-mediated ferroptotic and anti-migration activities. Oncogenesis 10 (2), 15. doi:10.1038/s41389-021-00304-3
Yi, X., and Tang, X. (2021). Exosomes from miR-19b-3p-modified ADSCs inhibit ferroptosis in intracerebral hemorrhage mice. Front. Cell Dev. Biol. 9, 661317. doi:10.3389/fcell.2021.661317
Zhang, H., Deng, T., Liu, R., Ning, T., Yang, H., Liu, D., et al. (2020a). CAF secreted miR-522 suppresses ferroptosis and promotes acquired chemo-resistance in gastric cancer. Mol. Cancer 19 (1), 43. doi:10.1186/s12943-020-01168-8
Zhang, H., Ge, Z., Wang, Z., Gao, Y., Wang, Y., and Qu, X. (2021a). Circular RNA RHOT1 promotes progression and inhibits ferroptosis via mir-106a-5p/STAT3 axis in breast cancer. Aging (Albany NY) 13 (6), 8115–8126. doi:10.18632/aging.202608
Zhang, H., He, Y., Wang, J. X., Chen, M. H., Xu, J. J., Jiang, M. H., et al. (2020b). miR-30-5p-mediated ferroptosis of trophoblasts is implicated in the pathogenesis of preeclampsia. Redox Biol. 29, 101402. doi:10.1016/j.redox.2019.101402
Zhang, H. Y., Zhang, B. W., Zhang, Z. B., and Deng, Q. J. (2020c). Circular RNA TTBK2 regulates cell proliferation, invasion and ferroptosis via miR-761/ITGB8 axis in glioma. Eur. Rev. Med. Pharmacol. Sci. 24 (5), 2585–2600. doi:10.26355/eurrev_202003_20528
Zhang, K., Wu, L., Zhang, P., Luo, M., Du, J., Gao, T., et al. (2018). miR-9 regulates ferroptosis by targeting glutamic-oxaloacetic transaminase GOT1 in melanoma. Mol. Carcinog. 57 (11), 1566–1576. doi:10.1002/mc.22878
Zhang, L., Ye, Y., Tu, H., Hildebrandt, M. A., Zhao, L., Heymach, J. V., et al. (2017). MicroRNA-related genetic variants in iron regulatory genes, dietary iron intake, microRNAs and lung cancer risk. Ann. Oncol. 28 (5), 1124–1129. doi:10.1093/annonc/mdx046
Zhang, X., Wang, L., Li, H., Zhang, L., Zheng, X., and Cheng, W. (2020d). Crosstalk between noncoding RNAs and ferroptosis: New dawn for overcoming cancer progression. Cell death Dis. 11 (7), 580. doi:10.1038/s41419-020-02772-8
Zhang, X., Zhu, J., Xing, R., Tie, Y., Fu, H., Zheng, X., et al. (2012). miR-513a-3p sensitizes human lung adenocarcinoma cells to chemotherapy by targeting GSTP1. Lung cancer 77 (3), 488–494. doi:10.1016/j.lungcan.2012.05.107
Zhang, Y., Guo, S., Wang, S., Li, X., Hou, D., Li, H., et al. (2021b). LncRNA OIP5-AS1 inhibits ferroptosis in prostate cancer with long-term cadmium exposure through miR-128-3p/SLC7A11 signaling. Ecotoxicol. Environ. Saf. 220, 112376. doi:10.1016/j.ecoenv.2021.112376
Zhao, H., Li, X., Yang, L., Zhang, L., Jiang, X., Gao, W., et al. (2021). Isorhynchophylline relieves ferroptosis-induced nerve damage after intracerebral hemorrhage via miR-122-5p/TP53/slc7a11 pathway. Neurochem. Res. 46 (8), 1981–1994. doi:10.1007/s11064-021-03320-2
Zheng, H., Shi, L., Tong, C., Liu, Y., and Hou, M. (2021). circSnx12 is involved in ferroptosis during heart failure by targeting miR-224-5p. Front. Cardiovasc Med. 8, 656093. doi:10.3389/fcvm.2021.656093
Zhou, X., Zhuo, M., Zhang, Y., Shi, E., Ma, X., and Li, H. (2021). miR-190a-5p regulates cardiomyocytes response to ferroptosis via directly targeting GLS2. Biochem. Biophys. Res. Commun. 566, 9–15. doi:10.1016/j.bbrc.2021.05.100
Zolea, F., Battaglia, A. M., Chiarella, E., Malanga, D., Marco, C. D., Bond, H. M., et al. (2017). Ferritin heavy subunit silencing blocks the erythroid commitment of K562 cells via miR-150 up-regulation and GATA-1 repression. Int. J. Mol. Sci. 18 (10), 2167. doi:10.3390/ijms18102167
Keywords: miRNA, ferroptosis, expression, pathwa, cancer
Citation: Mahmoudi-Lamouki R, Kadkhoda S, Hussen BM and Ghafouri-Fard S (2023) Emerging role of miRNAs in the regulation of ferroptosis. Front. Mol. Biosci. 10:1115996. doi: 10.3389/fmolb.2023.1115996
Received: 04 December 2022; Accepted: 06 February 2023;
Published: 15 February 2023.
Edited by:
Wei Ye, Guangdong Academy of Science, ChinaReviewed by:
Yiqun Jiang, Hunan Normal University, ChinaRaffaella Faraonio, University of Naples Federico II, Italy
Copyright © 2023 Mahmoudi-Lamouki, Kadkhoda, Hussen and Ghafouri-Fard. This is an open-access article distributed under the terms of the Creative Commons Attribution License (CC BY). The use, distribution or reproduction in other forums is permitted, provided the original author(s) and the copyright owner(s) are credited and that the original publication in this journal is cited, in accordance with accepted academic practice. No use, distribution or reproduction is permitted which does not comply with these terms.
*Correspondence: Soudeh Ghafouri-Fard, s.ghafourifard@sbmu.ac.ir