- 1The First Affiliated Hospital of Henan University, Henan University, Kaifeng, China
- 2Institute of Molecular Medicine, Henan International Joint Laboratory for Nuclear Protein Regulation, School of Basic Medical Sciences, Henan University, Kaifeng, China
Phoenixin is a newly discovered neuropeptide generated from small integral membrane protein 20. Phoenixin is a ligand for the G protein-coupled receptor 173 (GPR173) and has been detected in central and peripheral tissues of human, rats, mice, bovine, and zebrafish. It was initially involved in regulating reproductive function by stimulating the luteinizing hormone release from pituitary cells by increasing the level of gonadotropin-releasing hormone. Recently, many functions of phoenixin have been generalized, including regulation of food intake, memory, Alzheimer’s disease, anxiety, inflammation, neuronal and microglial activity, energy metabolism and body fluid balance, cardiovascular function, and endocrine activity. In addition, the interaction between phoenixin and nesfatin-1 have been revealed. The present article summarized the latest research progress on physiological function of phoenixin, suggesting that it is a potential target for novel drug development and clinical application.
Introduction
Phoenixin (PNX) is a novel neuropeptide that is cleaved from the C-terminal of small integral membrane protein 20 (SMIM20) (Lyu et al., 2013; Billert et al., 2020). The precursor for phoenixin is encoded by the gene C4orf52 and synthesized on cytoplasmic ribosomes, and it is cleaved by ectodomain shedding, a regulated process whereby membrane proteins are cleaved (Hayashida et al., 2010; McIlwraith and Belsham, 2018). Phoenixin includes two active subtypes: phoenixin-14 (14 amino acids) and phoenixin-20 (20 amino acids). Phoenixin is a known potential ligand for GPR173, an isolated G-protein-coupled receptor (GPCR) (Stein et al., 2016; McIlwraith et al., 2019). Recent studies have reported that phoenixin could perform many functions through GPR173 (Billert, et al., 2020). Xuan et al. showed that phoenixin-20 accelerated the proliferation of granulosa cells (GCs), induced estradiol (E2) production, and promoted follicular growth through its receptor GPR173 (Xuan Phuoc et al., 2019). In addition, phoenixin-20 reduced lipopolysaccharide (LPS)-induced cytotoxicity by activating GPR173 (Sun et al., 2020). Recent studies have indicated that phoenixin is involved in various physiological and pathological functions (Figure 1).
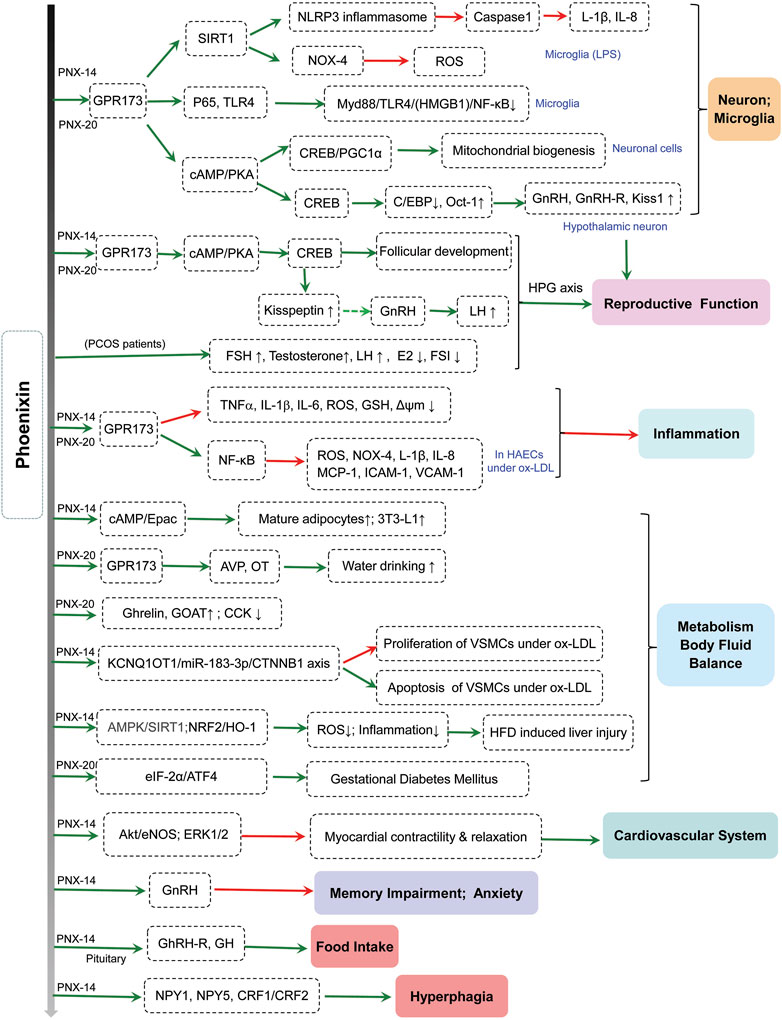
FIGURE 1. The physiological and pathological roles of phoenixin. AHA, Anterior hypothalamic area; Akt, protein kinase B; AMPK, AMP-activated protein kinase; ATF4, activating transcription factor 4; AVP, arginine vasopressin; cAMP, cyclic adenosine monophosphate; C/EBP, CCAAT/enhancer binding protein; CREB, cAMP-response element binding protein; CRF1/CRF2, corticotropin-releasing factor 1/2; CCK, cholecystokinin; E2, estradiol; eIF-2α, eukaryotic translation initiation factor 2α; eNOS, endothelial nitric oxide synthase; ERK, extracellular signal-related kinase; FSH, follicle-stimulating hormone; FSI, fasting serum insulin; GH, growth hormone; GhRH-R, growth hormone-releasing hormone receptor; GnRH, gonadotropin-releasing hormone; GnRH-R, gonadotropin-releasing hormone receptor; GOAT, ghrelin-O-acyl transferase; HAECs, human aortic endothelial cells; HFD, high-fat diet; HMGB1, high mobility group box1; HO-1, heme oxygenase-1; ICAM-1, intercellular adhesion molecule-1; IL-1β, interleukin-1β; IL-8, interleukin-8; LH, luteinizing hormone; LPS, lipopolysaccharide; MCP-1, monocyte chemotactic protein-1; Myd88, myeloid differentiation primary response 88; NF-κB, nuclear factor-κB; NLRP3, NOD-like receptor protein 3; NOX-4, NADPH oxidase 4; NPY1, neuropeptide Y1; NPY5, neuropeptide Y5; NRF2, Erythroid 2-related factor 2; NST, nucleus of the solitary tract; Oct-1, octamer-binding transcription factor-1; OT, oxytocin; ox-LDL, oxidized low-density lipoprotein; PCOS, polycystic ovary syndrome; PKA, protein kinase A; PGC-1α, peroxisome-proliferator-activated receptor coactivator (PGC)-1α; ROS, reactive oxygen species; SIRT1, Sirtuin1; TLR4, toll-like receptor 4; VCAM-1, vascular cell adhesion molecule-1; VSMCs, vascular smooth muscle cells; Δψm; mitochondrial membrane potential.
Expression of phoenixin
In rat brain, phoenixin and nesfatin-1 were co-localized to a large extent; hence, they are considered to have synergistic effects in some aspects. Presently, phoenixin has been detected in the central nervous system and peripheral tissues of rats, domestic pigs, spotted scat, zebrafish, chicken, and so on. Immunohistochemical results demonstrated that phoenixin immunoreactivity (irPNX) was found in the trigeminal spinal tract, superficial dorsal horn, and nucleus of the solitary tract (NTS), as well as in the cell population of dorsal root, trigeminal nerve, and nodular ganglion in the spinal cord of rats (Lyu, et al., 2013). Immunoreactive neural structures of the phoenixin protein in pig spinal cord showed that irPNX had a similar distribution pattern in grey matter of all spinal cord slices and varicose nerve fibers forming dense plexus, which could be observed mainly in the first and second layers of the dorsal horn (Lepiarczyk et al., 2020). Phoenixin-like and phoenixin receptor GPR173-like immunoreactivity was detected in zebrafish gonads and zebrafish liver (ZFL) cells (Rajeswari et al., 2022). In addition, phoenixin has been detected in the hypothalamus, pituitary gland, and liver. (Wang et al., 2018). Phoenixin is widely distributed in central and peripheral tissues, especially in the spinal cord and the hypothalamic-pituitary-gonadal (HPG) axis, which are closely related to neuroendocrine functions.
The intensity of phoenixin immunoreactivity in adult rat tissues from high to low was hypothalamus, heart, thymus, oesophagus, stomach, spleen, pancreas, lung, pituitary, and kidney (Yosten et al., 2013). In the hypothalamus, phoenixin was found in the arcuate nucleus (Arc), dorsal hypothalamus (DH), paraventricular nucleus (PVN), supraoptic nucleus (SON), ventromedial hypothalamus (VMH), and zona incerta (ZI) (Yosten, et al., 2013). Phoenixin was detected in magnocellular neurosecretory nuclei, and it suggested that phoenixin might be released into the circulatory system via the hypophyseal portal system (Palasz et al., 2015). In addition, phoenixin could be secreted by the peripherial tissues. Billert et al. reported that phoenixin was secreted from 3T3-L1 and rat primary adipocytes (Billert et al., 2018). Phoenixin was secreted from pancreatic islets, and the secretion of phoenixin depended on glucose concentration (Billert et al., 2019). Recent reports demonstrated that phoenixin is a multidimensional peptide (McIlwraith et al., 2021), indicating its roles in regulating many physiological and pathological functions (Table 1).
Function of phoenixin
Reproductive function
The hypothalamus, pituitary gland, and gonads produce neurohormones that are the vital regulatory hormones that promoting sexual maturity and maintain reproductive function. Recent literature suggested that phoenixin exerted roles in HPG axis activity and stress-induced fertility disorders (Yosten, et al., 2013; Golyszny et al., 2022). It was firstly found that phoenixin regulated pituitary gonadotropin secretion by modulating expression of the gonadotropin-releasing hormone (GnRH) receptor (Yosten, et al., 2013). Phoenixin/smim20 gene and protein are expressed in the brain including the hypothalamus regions important in the control of reproduction in adult zebrafish (Ceriani et al., 2021). Phoenixin has been proved to as an essential mediator in regulating ovarian periodicity. Phoenixin enhances the secretion of luteinizing hormone (LH) stimulated by GnRH. Moreover, it shows a direct effect on gonadotropins (Stein, et al., 2016). Phoenixin-20 regulates the expression of GnRH by reducing the expression of the C/EBP gene and increasing the expression of Oct-1. C/EBP and OCT-1 are two essential transcription factors of the GnRH promoter (Schalla and Stengel, 2018; Treen A. K. et al., 2016). Rybska et al. found that the development of canine uterine disorders, including endometrial hyperplasia or pyometra induced a downregulation of phoenixin and its receptor GPR173 expression (Rybska et al., 2022). Phoenixin can activate the cAMP/PKA pathway and lead to CREB phosphorylation through GPR173, and it induced an increase expression of genes related to follicular development (Xuan Phuoc, et al., 2019). Based on these results, phoenixin had quite a little expression in the hypothalamus in central nervous system, and it played a role in regulating HPG activity at multiple levels by activating GPR173 and GnRH receptor.
Recently, it was showed that phoenixin had a role in regulating HPG hormones and reproductive processes in fish (zebrafish and spotted scat). Phoenixin-like and putative phoenixin receptor Gpr173-like immunoreactivity was detected in zebrafish gonads and ZFL cells (Rajeswari and Unniappan, 2020). The genes involved in the steroid production pathway (cyp11a1, cyp17a1, 17βhsd, and cyp19a1a) were upregulated in the gonads of fish administered with phoenixin-20, and the expression of vitellogenin isoforms and estrogen receptor (esr2a and esr2b) mRNAs were upregulated in ZFL cells in vitro. Phoenixin-20 stimulated mRNAs encoding HPG hormones, such as regulation of vitellogenin and upregulation of steroidogenic mRNAs in the gonads (Rajeswari and Unniappan, 2020). In spotted scat, phoenixin can stimulate reproduction not only through the HPG axis but also directly through the pituitary gland (Wang et al., 2019). It is noteworthy that the phoenixin protein is dominant in the female reproductive system, because the stimulating effect of this protein on the HPG axis could be detected only in female cells or animals. However, even in male rats, phoenixin exerts a certain influence on the regulation of male sex hormones, which may not be through the activation of the HPG axis.
Phoenixin and nesfatin-1 have a similar distribution pattern in the brain. Both phoenixin and nesfatin-1 significantly increased the secretion of follicle-stimulating hormone (FSH), LH, and testosterone in rat plasma without inducing any changes in plasma GnRH, thus indicating that both these neuropeptides play a synergistic role in regulating male sex hormones (Guvenc et al., 2019). Nesfatin-1, a polypeptide containing 82-amino acid, was derived from the precursor protein nucleobindin 2, and it was secreted by the hypothalamus (Oh et al., 2006). Nesfatin-1 had a widespread distribution in central and peripheral tissues, and it was implicated in multiple physiological and pathological processes, including glucose and lipid metabolism, gastrointestinal functions, thermogenesis, depression, anxiety, as well as cardiovascular and reproductive functions (Luo et al., 2021).
A clinical study showed that the concentrations of LH, FSH, and nesfatin-1 in patients with PCOS were positively correlated with the level of phoenixin (Ullah et al., 2017). The nesfatin-1 play a dual effect in reproductive physiology. The nesfatin-1 level was lower in polycystic ovary syndrome (PCOS), and the decrease of nesfatin-1 may contribute to the mechanism governing PCOS, according the analysis of patients with PCOS (Demir Çaltekin et al., 2021) and animal model with PCOS (Xu et al., 2017). However, Ademoglu et al. reported that it had high concentrations of serum nesfatin-1 in PCOS patients (Ademoglu et al., 2014). The serum insulin in patients with PCOS was significantly higher than control group (Ullah, et al., 2017). In contrast, the concentrations of E2 and fasting serum insulin (FSI) were significantly negatively correlated with the level of phoenixin (Ullah, et al., 2017; Kalamon et al., 2020). These results indicate that phoenixin may play a role in developing of PCOS. Kulinska et al. demonstrated that the reduced phoenixin serum level and GPR173 expression might contribute to HPG axis dysregulation in women with endometriosis (Kulinska et al., 2021). In patients with endometriosis, the phoenixin and LH/FSH ratio had diagnostic relevance (Kulinska, et al., 2021), suggesting a significance of phoenixin in the diagnosis and treatment of endometriosis. More clinical research is wanted for an application of phoenixin in endometriosis and related diseases.
Food intake
Phoenixin immunoreactivity was detected in the Arc and NTS (Yosten, et al., 2013; Prinz and Stengel, 2017), and its receptor GPR173 was expressed in the Arc and PVN (Stein, et al., 2016; Treen Alice K. et al., 2016). These brain areas are closely related to the regulation of food intake (Prinz et al., 2017; Schalla and Stengel, 2019), thus suggesting a potential role of phoenixin in food intake. The phoenixin expression in the hypothalamus was modulated by peripheral signals, such as hormones and fatty acids (McLlwraith et al., 2018). It was speculated that diet could stimulate phoenixin secretion, act on GnRH neurons and induce subsequent activation of GnRH (Valsamakis et al., 2021). It was reported that an intracerebroventricular (i.c.v.) injection of phoenixin-14 under illumination induced a dose-dependent increase in food intake in rats. Specifically, the in-depth evaluation of food intake showed that i.c.v. injection of phoenixin-14 induced an increase in meal size, meal duration, meal time, and food intake rate, while the interval between meals and the satiety ratio decreased (Schalla et al., 2017). However, when phoenixin-14 was i.c.v. injected in dark or injected intraperitoneally under light, it did not affect food intake (Schalla, et al., 2017). In addition, phoenixin was involved in regulating the feeding of spotted scat. After fasting for 2 days and 7 days, the expression of the phoenixin protein in the hypothalamus was increased significantly, but decreased significantly after feeding again, and the mRNA level of ghrhr (growth hormone-releasing hormone receptor) and gh (growth hormone) was up-regulated in the pituitary after treatment with phoenixin-14 in vitro (Wang, et al., 2018). Therefore, it was speculated that phoenixin might be involved in regulating the feeding of spotted scat by increasing the expression of ghrhr and gh in the pituitary gland (Wang, et al., 2018). In neonatal chickens, i.c.v. administration of phoenixin-14 induced hyperphagia, which was mediated by neuropeptide Y1 (NPY1), neuropeptide Y5 (NPY5), and corticotropin-releasing factor 1/2 (CRF1/CRF2) receptors (Rajaei et al., 2022). In patients with anorexia nervosa (AN), the serum phoenixin level was decreased in malnourished patients (accAN) (Palasz et al., 2019). Phoenixin may play an important role in the etiology and course of AN. Phoenixin level is closely related to some eating disorder symptoms, thus indicating its potential role in regulating food intake in patients with AN (Palasz, et al., 2019).
Memory and Alzheimer’s diseases
Neuropeptides are commonly involved in regulating learning and memory. Phoenixin-14 (i.c.v.) promotes memory formation and prolongs memory retention time for tasks of novel object recognition and target location recognition in animals. Phoenixin-14 injected into the hippocampus can also enhance memory, while its i.c.v. administration could alleviate memory impairment caused by amyloid-β1-42 peptide aggregation and scopolamine, and the GnRH receptor was involved in this process (Jiang et al., 2015b). Recently, a clinical study compared the levels of phoenixin in individuals with subjective memory impairment, mild cognitive impairment, and mild Alzheimer’s disease, and demonstrated that the average plasma phoenixin level in the mild cognitive impairment group was negatively correlated with logical memory (Yuruyen et al., 2017). The average plasma phoenixin level in the subjective memory impairment group was positively correlated with immediate recall; this finding suggested that the plasma phoenixin level may have a predictive effect on cognitive memory impairment (Yuruyen, et al., 2017). Phoenixin may be a potential target to enhance memory and treat Alzheimer’s disease.
Anxiety
Phoenixin regulates the secretion of pituitary gonadotropins by enhancing the expression of the GnRH receptor gene (Millar and Newton, 2013; Yosten, et al., 2013). GnRH plays a role in regulating brain response to anxiety (Umathe et al., 2008; Telegdy et al., 2009), thus indicating a potential role of phoenixin in regulating anxiety. Phoenixin-14 exerted a dose-dependent anti-anxiety effect on mice in the open field test and elevated plus maze test, and the anti-anxiety effect appeared after the injection of phoenixin-14 into the anterior hypothalamus (AHA), not in the amygdala (Jiang et al., 2015a). It is assumed that phoenixin might be involved in the mechanism of anxiety regulation (Palasz et al., 2018). In clinical trials, after evaluating depression (PHQ-9) and perceived stress (PSQ-20) of 68 inpatients with extensive psychometric anxiety (GAD-7), it was found that the phoenixin level was negatively correlated with anxiety in obese men (Hofmann et al., 2017). A recent study indicated that restraint stress reduced circulating phoenixin levels in male rats (Schalla et al., 2020). Thus, phoenixin plays a crucial role in emtional behavior, such as anxiety. However, whether phoenixin had an effect on neurotransmitter or nervous excitation need to be investigated.
Neuronal and microglial activity
Phoenixin was shown to stimulate the excitability of nerve cells and promote the occurrence of mitochondrial organisms. Phoenixin-20 promoted the mitochondrial biogenesis of neurons mainly by regulating the CREB/PGC-1α pathway. Specifically, the expression levels of mitochondrial regulatory factors, including PGC-1α, NRF-1, and TFAM, were increased in cultured neuronal M17 cells after treatment with phoenixin-20 (Yang, et al., 2020). In mHypoA cell lines, phoenixin-20 can stimulate the expression of the GnRH, GnRH-R, and Kiss1 genes through GPR173 by activating the cAMP/PKA pathway of CREB or through the expression of C/EBP and/or Oct-1 (Treen, et al., 2016). In microglia, phoenixin-14 inhibited the signaling pathway of myeloid differentiation primary response 88 (Myd88)/toll-like receptor 4 (TLR4)/high mobility group box 1 (HMG B1)/nuclear factor-κB (NF-κB) by preventing the nuclear translocation of p65 protein and decreasing the activation of TLR4 (Ma et al., 2020). In BV2 microglia, phoenixin-20 exhibits an inhibitory effect on NOD-like receptor protein 3 (NLRP3) inflammasome induced by LPS, subsequently leading to the suppression of IL-1β and IL-18 secretion; moreover, the neuroprotective effect of phoenixin-20 was dependent on silent information regulator 1 (Zeng et al., 2020). In addition, phoenixin-20 significantly inhibits the NADPH oxidase 4 (NOX-4) and reactive oxygen species (ROS) induced by LPS (Zeng, et al., 2020). Electrophysiological recordings from brain slices demonstrated that phoenixin-14 reduced the interictal-like events in the entorhinal cortex and hippocampus (Kalkan et al., 2021), suggesting a potential role of phoenixin-14 in epileptiform activity. Recent study demonstrated that inflammatory stress induced by LPS up-regulated phoenixin expression and activity in the specific brain nuclei of SD Rats, indicating that several stressors could activate phoenixin signaling (Friedrich et al., 2022).
Phoenixin-20 also increased the ratio of mitochondrial DNA to nuclear DNA (mtDNA/nDNA) and promoted the expression of multiple mitochondrial genes and the mitochondrial respiratory rate and ATP production (Yang, et al., 2020). The role of phoenixin-20 in neuronal cells suggests that it could be considered as a therapeutic target for neurodegenerative diseases (Yang, et al., 2020). Phoenixin-20 can also directly control the excitability of nucleus of NTS neurons. Phoenixin increased the discharge frequency of some NTS neurons, and nearly 50% of NTS neurons were depolarized after treatment with phoenixin-20 (Grover et al., 2020). Wang et al. found that phoenixin-20 could mitigate brain infarction by promoting microglia M2 polarization using an ischemic stroke middle cerebral artery occlusion (MCAO) mouse model, and it was dependent on GPR173 (Wang et al., 2022). In vitro experiments demonstrated that phoenixin-14 alleviated morphine-induced cellular senescence in M17 neuronal cell through regulating yes-associated protein expression (Hu et al., 2022). In brief, phoenixin exerted a role on neuroprotection mainly by suppressing inflammation and ROS, and the experiments using human neuron and glial cells in vitro, and clinical studies are wanted to promote its applied research.
Regulation of inflammation
Neuroinflammation commonly occurs in the aging brain and several diseases, such as Alzheimer’s disease, Parkinson’s disease, Acquired Immune Deficiency Syndrome dementia complex, and stroke (Vesce et al., 2007). LPS induces inflammation of astrocytes and activates inflammatory bodies, and it inhibits the gene and protein expression of GPR173 (Wang et al., 2020). Phoenixin-14 can attenuate the production of ROS and decrease the level of superoxide dismutase (SOD) induced by LPS (Wang, et al., 2020). Phoenixin-14 can regulate the expression of proinflammatory cytokines, such as tumor necrosis factor-α (TNFα), interleukin-1β (IL-1β), and interleukin-6 (IL-6), and it can reduce the generation of ROS and increase the level of glutathione induced by oxygen-glucose deprivation/reperfusion injury in BV2 microglia (Ma, et al., 2020). Phoenixin-14 was found to increase the expression of protective nitric oxide synthase and nitric oxide, and decrease the permeability of the endothelial monolayer in human brain endothelial bEnd.3 cells (Zhang and Li, 2020).
Phoenixin-20 inhibited LPS-induced inflammation of dental pulp cells, and phoenixin-20 inhibited the release of pro-inflammatory cytokines and inflammatory mediators induced by LPS, including monocyte chemotactic protein-1 (MCP-1), IL-6, intercellular adhesion molecule-1 (ICAM-1), vascular cell adhesion molecule-1 (VCAM-1), MMP-2 and MMP-9 (Sun, et al., 2020). Phoenixin-20, activating GPR173, significantly ameliorated oxidized low-density lipoprotein (ox-LDL) induced harmful effects by suppressing the NF-κB pathway in human aortic endothelial cells, and the harmful effects were presented as an increase in ROS, NOX-4, IL-1β, interleukin-8 (IL-8), and MCP-1 expression, as well as ICAM-1 and VCAM-1 release (Wei et al., 2021). Recently, Zandeh-Rahimi et al. found that phoenixin-14 could protect indomethacin-induced duodenal ulcer in rats, and phoenixin-14 exhibited a decrease in serum levels of inflammatory cytokines (IL-1β, TNF-α, IL-6, and IL-12), malondialdehyde and myeloperoxidase activity, and an increase on SOD and catalase activity in duodenal ulcer (Zandeh-Rahimi et al., 2022). This result indicated that phoenixin-14 alleviated duodenal ulcer against inflammation via reducing inflammatory cytokines and suppressing oxidative content. On the basis of these findings, phoenixin might be used as a new potential therapeutic agent for ischemic stroke, gastrointestinal inflammatory disorders, and other central nervous system disorders.
Energy metabolism and body fluid balance
Phoenixin is positively correlated with human body mass index, indicating it may play a potential role in regulating body weight (Billert, et al., 2018). The Smim20 and Gpr173 genes were detected in 3T3-L1 and rat primary preadipocytes, and phoenixin-14 stimulated the transformation of 3T3-L1 and rat primary preadipocytes into mature adipocytes through the cAMP/Epac-dependent pathway (Billert, et al., 2018). Phoenixin could promote the production of white adipocytes, and it may be involved in body weight control (Billert, et al., 2018). Phoenixin-14 alleviated high-fat diet-induced liver injury through activation of the AMP-activated protein kinase/Sirtuin1 and Erythroid 2-related factor 2/heme oxygenase-1 (NRF2/HO-1) pathways in experimental nonalcoholic fatty liver disease mice (Yang, et al., 2020). Recently, Chi et al. found that phoenixin-20 ameliorated gestational diabetes mellitus (GDM) symptoms in the GDM mouse model, and phoenixin-20 could significantly inhibit the activation of eukaryotic translation initiation factor 2α/activating transcription factor 4 endoplasmic reticulum (ER) stress signaling pathway in GDM mice (Chi et al., 2021). In streptozotocin-induced diabetes mice, phoenixin-14 protected against cardiac damages through the sirtuin-3 pathway (Yao et al., 2021). In diabetes mouse model, phoenixin could alleviate symptom or protect against cardiac damage, and the related factors, gene, and ER were involved in these processes.
Intraventricular administration of phoenixin stimulated drinking in rats under random conditions and increased the amount of drinking water under thirst at night. In addition, pretreatment with losartan blocked the effect of phoenixin (Haddock et al., 2020). Both phoenixin and its receptor GPR173 are expressed in the SON and PVN of the hypothalamus, and phoenixin may affect the secretion of arginine vasopressin or oxytocin (Gasparini et al., 2018). In zebrafish, phoenixin-20 exerted as a tissue-specific regulator of the liver (suppressor) and muscle (stimulant) insulin-like growth factor signaling (Rajeswari et al., 2022). Phoenixin may be as a regulator for the homeostasis of body fluids and electrolytes, which due to its expressions areas in SON and PVN in hypothalamus.
Exogenous phoenixin induced a reduction of contractility and relaxation of isolated Langendorff-perfused rat hearts, without affecting coronary artery pressure and heart rate, and these effects were accompanied by the increase in phosphorylation of Erk1/2, Akt, and eNOS; these changes may be the reason for alteration in cardiac contractility and diastole function (Rocca et al., 2018). Phoenixin-14 inhibited cell proliferation and facilitated apoptosis of vascular smooth muscle cells under ox-LDL treatment by regulating the KCNQ1OT1/miR-183-3p/CTNNB1 axis (Ling et al., 2021). In addition, phoenixin treatment of isolated hearts with ischemia/reperfusion (I/R) injury showed a smaller infarct area and better contraction recovery than the control group (Rocca, et al., 2018). This demonstrated that phoenixin plays a protective role in the presence of I/R.
Endocrine regulation
Phoenixin expression is regulated by bisphenol A (BPA), fatty acid palmitate, docosahexaenoic acid (DHA), and oleate (McLlwraith, et al., 2018). The BPA, as an endocrine disrupting chemical, has estrogenic activity (Acconcia et al., 2015). The oleate and palmitoleate are monounsaturated fatty acids, and DHA is polyunsaturated fatty acid. These results demonstrated that phoenixin was responsive to estrogenic activity as well as multiple fatty acids. After treatment of immortalized hypothalamic neurons with palmitic acid or BPA for 2-24 h, the expression level of the Gpr173 gene was decreased (McLlwraith, et al., 2018). Furthermore, pretreatment with palmitic acid blocked phoenixin-induced phosphorylated cAMP response element-binding protein (CREB) level (McIlwraith, et al., 2019). GnRH-R analogues (bacarelin and cetrorelix) play a role in phoenixin/smim20 signal transduction of the HPG axis (Suszka-Switek et al., 2019). The expression level of the mitomycin-20 gene was increased in the pituitary gland and especially in the ovary after the administration of bacarelin and cetrorelix in female rats (Suszka-Switek, et al., 2019). GnRH analogues may act as a factor in regulating reproductive function by affecting the expression of phoenixin. In addition, physical effects and restraint stress may affect the expression of phoenixin. Restraint stress significantly increased the expression of phoenixin in many brain nuclei (Friedrich et al., 2020). A recent study demonstrated that phoenixin-20 could act directly on gut cells to regulate metabolic hormones (Mukherjee and Unniappan, 2021). Phoenixin-20 significantly upregulated mRNA levels of ghrelin and ghrelin-O-acyl transferase (GOAT) in MGN3-1 (mouse stomach endocrine) cells, and markedly suppressed mRNA level of cholecystokinin (CCK) in STC-1 (mouse enteroendocrine) cells (Mukherjee and Unniappan, 2021). Celik et al. had demonstrated that phoenixin as well as endocan were higher in both aqueous humor and blood samples compared with the group of patients without diabetic retinopathy with cataract (Celik and Aydin, 2022), indicating a possible close relationship between phoenixin and diabetes mellitus.
Interaction with nesfatin-1
In rat brain, nesfatin-1 showed a high degree of co-localization with phoenixin. Nearly one-fifth of cells in the PVN, Arc, ventromedial nucleus and lateral hypothalamic nucleus showed irPNX (Lyu, et al., 2013; Lyu et al., 2018). Bioinformatic survey demonstrated that both phoenixin and nesfatin were as ancient neuropeptides with premetazoan origin (Yanez-Guerra et al., 2022). The expression of nesfatin-1 in 50% of all neurons occurred at the same site, especially in the hypothalamic nucleus in rats, and most of the irPNX neurons showed co-expression with nesfatin-1 (Palasz, et al., 2015). Consequently, both phoenixin and nesfatin-1 were involved in regulating the neuroendocrine activity.
Both phoenixin and nesfatin-1 could significantly enhance the levels of FSH, LH, and testosterone in rat plasma without causing any changes in plasma GnRH level (Guvenc, et al., 2019). Phoenixin and nesfatin-1 can synergistically affect the plasma androgen level (Palasz, et al., 2015; Guvenc, et al., 2019). In addition, phoenixin can directly activate nesfatin-1 immunoreactive neurons in rat brain. After i.c.v. administration of phoenixin, the number of nesfatin-1 immunoreactive neurons was increased in the lateral septal nucleus, PVN, NTS, and SON (Friedrich et al., 2019). Recently, Friedrich et al. proposed that phoenixin had possible physiological interactions with nesfatin-1, especially in stress response (Friedrich and Stengel, 2021). Under restraint stress, the nesfatin-1 level in rat serum was increased, whereas the phoenixin level was decreased (Schalla, et al., 2020). In rats under restraint stress, both nesfatin-1 and phoenixin immunoreactivity was upregulated in the nucleus of the solitary tract (NTS) and raphe pallidus, however they exhibited different expression in other nuclei (Goebel et al., 2009; Friedrich, et al., 2020). The co-localization of phoenixin and nesftin-1 in several nucleus in hypothalamus suggested that the two homologous protein may play similar roles in neuroendocrine regulation. Recent reports demonstrated that phoenixin and nesftin-1 exhibited a same trend on HPG axis activity. In the circumstance of restraint stress, the phoenixin and nesftin-1 immunoreactivity areas in brain are different. The definite interaction and mechanism of phoenixin and nesftin-1 need to be furtherly investigated.
Conclusion
Phoenixin, a recently discovered neuropeptide, plays a role in many physiological processes. In the past few years, our understanding of the role of phoenixin has dramatically improved, which is far beyond its originally described role in reproductive function. Phoenixin also regulates animal food intake, learning and memory, anxiety, inflammatory response, cardiac protection, body weight, adipocyte growth, and body fluid and electrolyte balance. Phoenixin plays a variety of roles by activating GPR173. Phoenixin mainly produces beneficial effects by inhibiting inflammation and oxidative stress in vitro or in vivo experimental disease models. Hence, it might be a promising target for the development of anti-inflammatory and antitumor drugs. Considering the physiological roles of phoenixin, its effects on endocrine disorders and neurodegenerative diseases remains to be further explored. In the future, gene knock-in/knock-out animal models and clinical studies are needed to clarify the physiological function of phoenixin and evaluate its therapeutic potential.
Author contributions
HL and QZ wrote the draft of the manuscript. SL and XJ contributed to the revision and writing of the manuscript.
Funding
This work was supported by the Natural Science Foundation for Excellent Young Scholars of Henan Province (Grant No. 212300410026), the National Natural Science Foundation of China (Grant No. 81971280), and the Program for Young Key Teacher of Henan Province (Grant No. 2020GGJS037).
Conflict of interest
The authors declare that the research was conducted in the absence of any commercial or financial relationships that could be construed as a potential conflict of interest.
Publisher’s note
All claims expressed in this article are solely those of the authors and do not necessarily represent those of their affiliated organizations, or those of the publisher, the editors and the reviewers. Any product that may be evaluated in this article, or claim that may be made by its manufacturer, is not guaranteed or endorsed by the publisher.
Abbreviations
↑, increase; ↓, decrease; AHA, anterior hypothalamic area; fsh, follicular stimulating hormone; GCs, Granulosa cells; GDM, gestational diabetes mellitus; gh, growth hormone; GnRHR, gonadotropin releasing hormone receptor; I/R, ischemia/reperfusion; ICV, intracerebroventricular; IL-1β, interleukin-1β; IL-6, interleukin-6; IP, intraperitoneal; lh, luteinizing hormone; LPS, lipopolysaccharide; MCAO, middle cerebral artery occlusion; NLRP3, NOD-like receptor protein 3; OGD/R, oxygen-glucose deprivation and reoxygenation; ROS, reactive oxygen species; SC, subcutaneous; SD, sprague-dawley; TNFα, tumor necrosis factor α.
References
Acconcia, F., Pallottini, V., and Marino, M. (2015). Molecular mechanisms of action of BPA. Dose. Response. 13, 1559325815610582. doi:10.1177/1559325815610582
Ademoglu, E. N., Gorar, S., Carlıoglu, A., Yazıcı, H., Dellal, F. D., Berberoglu, Z., et al. (2014). Plasma nesfatin-1 levels are increased in patients with polycystic ovary syndrome. J. Endocrinol. Invest. 37, 715–719. doi:10.1007/s40618-014-0089-2
Billert, M., Kołodziejski, P. A., Strowski, M. Z., Nowak, K. W., and Skrzypski, M. (2019). Phoenixin-14 stimulates proliferation and insulin secretion in insulin producing INS-1E cells. Biochim. Biophys. Acta. Mol. Cell. Res. 1866, 118533. doi:10.1016/j.bbamcr.2019.118533
Billert, M., Rak, A., Nowak, K. W., and Skrzypski, M. (2020). Phoenixin: More than reproductive peptide. Int. J. Mol. Sci. 21, 8378. doi:10.3390/ijms21218378
Billert, M., Wojciechowicz, T., Jasaszwili, M., Szczepankiewicz, D., Wasko, J., Kazmierczak, S., et al. (2018). Phoenixin-14 stimulates differentiation of 3T3-L1 preadipocytes via cAMP/Epac-dependent mechanism. Biochim. Biophys. Acta. Mol. Cell. Biol. Lipids 1863, 1449–1457. doi:10.1016/j.bbalip.2018.09.006
Celik, F., and Aydin, S. (2022). Blood and aqueous humor phoenixin, endocan and spexin in patients with diabetes mellitus and cataract with and without diabetic retinopathy. Peptides 150, 170728. doi:10.1016/j.peptides.2021.170728
Ceriani, R., Calfun, C., and Whitlock, K. E. (2021). Phoenixin (smim20), a gene coding for a novel reproductive ligand, is expressed in the brain of adult zebrafish. Gene Expr. Patterns. 39, 119164. doi:10.1016/j.gep.2020.119164
Chi, X., Li, Z., Zhang, L., Xie, X., and Huang, M. (2021). Phoenixin-20 ameliorates gestational diabetes mellitus (GDM) symptoms and placental insults in an experimental mouse model. Int. Immunopharmacol. 101, 108171. doi:10.1016/j.intimp.2021.108171
Demir Çaltekin, M., Caniklioğlu, A., Eris Yalçın, S., Aydoğan Kırmızı, D., Baser, E., and Yalvaç, E. S. (2021). DLK1 and Nesfatin-1 levels and the relationship with metabolic parameters in polycystic ovary syndrome: prospective, controlled study. Turk. J. Obstet. Gynecol. 18, 124–130. doi:10.4274/tjod.galenos.2021.39024
Friedrich, T., Schalla, M. A., Goebel-Stengel, M., Kobelt, P., Rose, M., and Stengel, A. (2022). Inflammatory stress induced by intraperitoneal injection of LPS increases phoenixin expression and activity in distinct rat brain nuclei. Brain Sci. 12, 135. doi:10.3390/brainsci12020135
Friedrich, T., Schalla, M. A., Lommel, R., Goebel-Stengel, M., Kobelt, P., Rose, M., et al. (2020). Restraint stress increases the expression of phoenixin immunoreactivity in rat brain nuclei. Brain Res. 1743, 146904. doi:10.1016/j.brainres.2020.146904
Friedrich, T., Schalla, M. A., Scharner, S., Kuehne, S. G., Goebel-Stengel, M., Kobelt, P., et al. (2019). Intracerebroventricular injection of phoenixin alters feeding behavior and activates nesfatin-1 immunoreactive neurons in rats. Brain Res. 1715, 188–195. doi:10.1016/j.brainres.2019.03.034
Friedrich, T., and Stengel, A. (2021). Role of the novel peptide phoenixin in stress response and possible interactions with nesfatin-1. Int. J. Mol. Sci. 22, 9156. doi:10.3390/ijms22179156
Gasparini, S., Stein, L. M., Loewen, S. P., Haddock, C. J., Soo, J., Ferguson, A. V., et al. (2018). Novel regulator of vasopressin secretion: Phoenixin. Am. J. Physiol. Regul. Integr. Comp. Physiol. 314, R623–R628. doi:10.1152/ajpregu.00426.2017
Goebel, M., Stengel, A., Wang, L., and Tache, Y. (2009). Restraint stress activates nesfatin-1-immunoreactive brain nuclei in rats. Brain Res. 1300, 114–124. doi:10.1016/j.brainres.2009.08.082
Golyszny, M., Obuchowicz, E., and Zielinski, M. (2022). Neuropeptides as regulators of the hypothalamus-pituitary-gonadal (HPG) axis activity and their putative roles in stress-induced fertility disorders. Neuropeptides 91, 102216. doi:10.1016/j.npep.2021.102216
Grover, H. M., Smith, P. M., and Ferguson, A. V. (2020). Phoenixin influences the excitability of nucleus of the solitary tract neurones, effects which are modified by environmental and glucocorticoid stress. J. Neuroendocrinol. 32, e12855. doi:10.1111/jne.12855
Guvenc, G., Altinbas, B., Kasikci, E., Ozyurt, E., Bas, A., Udum, D., et al. (2019). Contingent role of phoenixin and nesfatin-1 on secretions of the male reproductive hormones. Andrologia 51, e13410. doi:10.1111/and.13410
Haddock, C. J., Almeida-Pereira, G., Stein, L. M., Yosten, G. L. C., and Samson, W. K. (2020). A novel regulator of thirst behavior: phoenixin. Am. J. Physiol. Regul. Integr. Comp. Physiol. 318, R1027–R1035. doi:10.1152/ajpregu.00023.2020
Hayashida, K., Bartlett, A. H., Chen, Y., and Park, P. W. (2010). Molecular and cellular mechanisms of ectodomain shedding. Anat. Rec. Hob. 293, 925–937. doi:10.1002/ar.20757
Hofmann, T., Weibert, E., Ahnis, A., Elbelt, U., Rose, M., Klapp, B. F., et al. (2017). Phoenixin is negatively associated with anxiety in obese men. Peptides 88, 32–36. doi:10.1016/j.peptides.2016.12.011
Hu, Y., Shen, X., Liu, F., and Zhu, W. (2022). Phoenixin-14 ameliorates cellular senescence against morphine in M17 neuronal cells. Neurotox. Res. 40, 498–507. doi:10.1007/s12640-022-00489-4
Jiang, J. H., He, Z., Peng, Y. L., Jin, W. D., Mu, J., Xue, H. X., et al. (2015a). Effects of Phoenixin-14 on anxiolytic-like behavior in mice. Behav. Brain Res. 286, 39–48. doi:10.1016/j.bbr.2015.02.011
Jiang, J. H., He, Z., Peng, Y. L., Jin, W. D., Wang, Z., Mu, L. Y., et al. (2015b). Phoenixin-14 enhances memory and mitigates memory impairment induced by Aβ1-42 and scopolamine in mice. Brain Res. 1629, 298–308. doi:10.1016/j.brainres.2015.10.030
Kalamon, N., Blaszczyk, K., Szlaga, A., Billert, M., Skrzypski, M., Pawlicki, P., et al. (2020). Levels of the neuropeptide phoenixin-14 and its receptor GRP173 in the hypothalamus, ovary and periovarian adipose tissue in rat model of polycystic ovary syndrome. Biochem. Biophys. Res. Commun. 528, 628–635. doi:10.1016/j.bbrc.2020.05.101
Kalkan, O. F., Sahin, Z., Ozturk, H., Keser, H., Aydin-Abidin, S., and Abidin, I. (2021). Phoenixin-14 reduces the frequency of interictal-like events in mice brain slices. Exp. Brain Res. 239, 2841–2849. doi:10.1007/s00221-021-06179-5
Kulinska, K. I., Andrusiewicz, M., Dera-Szymanowska, A., Billert, M., Skrzypski, M., Szymanowski, K., et al. (2021). Phoenixin as a new target in the development of strategies for endometriosis diagnosis and treatment. Biomedicines 9, 1427. doi:10.3390/biomedicines9101427
Lepiarczyk, E., Bossowska, A., Majewska, M., Skowronska, A., Kaleczyc, J., and Majewski, M. (2020). Distribution and chemical coding of phoenixin-immunoreactive nerve structures in the spinal cord of the pig. Ann. Anat. 232, 151559. doi:10.1016/j.aanat.2020.151559
Ling, C., Hu, X., Luo, L., Liang, C., Wang, H., and Chen, C. (2021). Phoenixin-14 regulates proliferation and apoptosis of vascular smooth muscle cells by modulation of KCNQ1OT1/miR-183-3p/CTNNB1 axis. Environ. Toxicol. Pharmacol. 86, 103655. doi:10.1016/j.etap.2021.103655
Luo, J. J., Wen, F. J., Qiu, D., and Wang, S. Z. (2021). Nesfatin-1 in lipid metabolism and lipid-related diseases. Clin. Chim. Acta. 522, 23–30. doi:10.1016/j.cca.2021.08.005
Lyu, R.-M., Cowan, A., Zhang, Y., Chen, Y.-H., Dun, S. L., Chang, J.-K., et al. (2018). Phoenixin: a novel brain-gut-skin peptide with multiple bioactivity. Acta Pharmacol. Sin. 39, 770–773. doi:10.1038/aps.2017.195
Lyu, R. M., Huang, X. F., Zhang, Y., Dun, S. L., Luo, J. J., Chang, J. K., et al. (2013). Phoenixin: a novel peptide in rodent sensory ganglia. Neuroscience 250, 622–631. doi:10.1016/j.neuroscience.2013.07.057
Ma, H., Su, D., Wang, Q., Chong, Z., Zhu, Q., He, W., et al. (2020). Phoenixin 14 inhibits ischemia/reperfusion-induced cytotoxicity in microglia. Arch. Biochem. Biophys. 689, 108411. doi:10.1016/j.abb.2020.108411
McIlwraith, E. K., and Belsham, D. D. (2018). Phoenixin: uncovering its receptor, signaling and functions. Acta Pharmacol. Sin. 39, 774–778. doi:10.1038/aps.2018.13
McIlwraith, E. K., Loganathan, N., and Belsham, D. D. (2019). Regulation of Gpr173 expression, a putative phoenixin receptor, by saturated fatty acid palmitate and endocrine-disrupting chemical bisphenol a through a p38-mediated mechanism in immortalized hypothalamic neurons. Mol. Cell. Endocrinol. 485, 54–60. doi:10.1016/j.mce.2019.01.026
McIlwraith, E. K., Zhang, N., and Belsham, D. D. (2021). The regulation of phoenixin: a fascinating multidimensional peptide. J. Endocr. Soc. 6, bvab192. doi:10.1210/jendso/bvab192
McLlwraith, E. K., Loganathan, N., and Belsham, D. D. (2018). Phoenixin expression is regulated by the fatty acids palmitate, docosahexaenoic acid and oleate, and the endocrine disrupting chemical bisphenol a in immortalized hypothalamic neurons. Front. Neurosci. 12, 838. doi:10.3389/fnins.2018.00838
Millar, R. P., and Newton, C. L. (2013). Current and future applications of GnRH, kisspeptin and neurokinin B analogues. Nat. Rev. Endocrinol. 9, 451–466. doi:10.1038/nrendo.2013.120
Mukherjee, K., and Unniappan, S. (2021). Mouse gastric mucosal endocrine cells are sources and sites of action of phoenixin-20. Peptides 141 (3), 170551. doi:10.1016/j.peptides.2021.170551
Oh, I. S., Shimizu, H., Satoh, T., Okada, S., Adachi, S., Inoue, K., et al. (2006). Identification of nesfatin-1 as a satiety molecule in the hypothalamus. Nature 443, 709–712. doi:10.1038/nature05162
Palasz, A., Janas-Kozik, M., Borrow, A., Arias-Carrion, O., and Worthington, J. J. (2018). The potential role of the novel hypothalamic neuropeptides nesfatin-1, phoenixin, spexin and kisspeptin in the pathogenesis of anxiety and anorexia nervosa. Neurochem. Int. 113, 120–136. doi:10.1016/j.neuint.2017.12.006
Palasz, A., Rojczyk, E., Bogus, K., Worthington, J. J., and Wiaderkiewicz, R. (2015). The novel neuropeptide phoenixin is highly co-expressed with nesfatin-1 in the rat hypothalamus, an immunohistochemical study. Neurosci. Lett. 592, 17–21. doi:10.1016/j.neulet.2015.02.060
Palasz, A., Tyszkiewicz-Nwafor, M., Suszka-Switek, A., Bacopoulou, F., Dmitrzak-Weglarz, M., Dutkiewicz, A., et al. (2019). Longitudinal study on novel neuropeptides phoenixin, spexin and kisspeptin in adolescent inpatients with anorexia nervosa - association with psychiatric symptoms. Nutr. Neurosci. 24, 896–906. doi:10.1080/1028415x.2019.1692494
Prinz, P., Scharner, S., Friedrich, T., Schalla, M., Goebel-Stengel, M., Rose, M., et al. (2017). Central and peripheral expression sites of phoenixin-14 immunoreactivity in rats. Biochem. Biophys. Res. Commun. 493, 195–201. doi:10.1016/j.bbrc.2017.09.048
Prinz, P., and Stengel, A. (2017). Control of food intake by gastrointestinal peptides: mechanisms of action and possible modulation in the treatment of obesity. J. Neurogastroenterol. Motil. 23, 180–196. doi:10.5056/jnm16194
Rajaei, S., Zendehdel, M., Rahnema, M., Hassanpour, S., and Asle-Rousta, M. (2022). Mediatory role of the central NPY, melanocortine and corticotrophin systems on phoenixin-14 induced hyperphagia in neonatal chicken. Gen. Comp. Endocrinol. 315, 113930. doi:10.1016/j.ygcen.2021.113930
Rajeswari, J. J., and Unniappan, S. (2020). Phoenixin-20 stimulates mRNAs encoding hypothalamo-pituitary-gonadal hormones, is pro-vitellogenic, and promotes oocyte maturation in zebrafish. Sci. Rep. 10, 6264. doi:10.1038/s41598-020-63226-x
Rajeswari, J. J., Vélez, E. J., and Unniappan, S. (2022). Liver and muscle-specific effects of phoenixin-20 on the insulin-like growth factor system mRNAs in zebrafish. Growth Horm. IGF Res. 63, 101456. doi:10.1016/j.ghir.2022.101456
Rocca, C., Scavello, F., Granieri, M. C., Pasqua, T., Amodio, N., Imbrogno, S., et al. (2018). Phoenixin-14: detection and novel physiological implications in cardiac modulation and cardioprotection. Cell. Mol. Life Sci. 75, 743–756. doi:10.1007/s00018-017-2661-3
Rybska, M., Billert, M., Skrzypski, M., Kubiak, M., Woźna-Wysocka, M., Łukomska, A., et al. (2022). Canine cystic endometrial hyperplasia and pyometra may downregulate neuropeptide phoenixin and GPR173 receptor expression. Anim. Reprod. Sci. 238, 106931. doi:10.1016/j.anireprosci.2022.106931
Schalla, M. A., Goebel-Stengel, M., Friedrich, T., Kühne, S. G., Kobelt, P., Rose, M., et al. (2020). Restraint stress affects circulating NUCB2/nesfatin-1 and phoenixin levels in male rats. Psychoneuroendocrinology 122, 104906. doi:10.1016/j.psyneuen.2020.104906
Schalla, M. A., and Stengel, A. (2018). Phoenixin-a pleiotropic gut-brain peptide. Int. J. Mol. Sci. 19, 1726. doi:10.3390/ijms19061726
Schalla, M. A., and Stengel, A. (2019). The role of phoenixin in behavior and food intake. Peptides 114, 38–43. doi:10.1016/j.peptides.2019.04.002
Schalla, M., Prinz, P., Friedrich, T., Scharner, S., Kobelt, P., Goebel-Stengel, M., et al. (2017). Phoenixin-14 injected intracerebroventricularly but not intraperitoneally stimulates food intake in rats. Peptides 96, 53–60. doi:10.1016/j.peptides.2017.08.004
Stein, L. M., Tullock, C. W., Mathews, S. K., Garcia-Galiano, D., Elias, C. F., Samson, W. K., et al. (2016). Hypothalamic action of phoenixin to control reproductive hormone secretion in females: importance of the orphan G protein-coupled receptor Gpr173. Am. J. Physiol. Regul. Integr. Comp. Physiol. 311, R489–R496. doi:10.1152/ajpregu.00191.2016
Sun, G., Ren, Q., Bai, L., and Zhang, L. (2020). Phoenixin-20 suppresses lipopolysaccharide-induced inflammation in dental pulp cells. Chem. Biol. Interact. 318, 108971. doi:10.1016/j.cbi.2020.108971
Suszka-Switek, A., Palasz, A., Filipczyk, L., Menezes, I. C., Mordecka-Chamera, K., Angelone, T., et al. (2019). The GnRH analogues affect novel neuropeptide SMIM20/phoenixin and GPR173 receptor expressions in the female rat hypothalamic-pituitary-gonadal (HPG) axis. Clin. Exp. Pharmacol. Physiol. 46, 350–359. doi:10.1111/1440-1681.13061
Telegdy, G., Tanaka, M., and Schally, A. V. (2009). Effects of the LHRH antagonist Cetrorelix on the brain function in mice. Neuropeptides 43, 229–234. doi:10.1016/j.npep.2009.03.001
Treen, A. K., Luo, V., and Belsham, D. D. (2016). Phoenixin activates immortalized GnRH and kisspeptin neurons through the novel receptor GPR173. Mol. Endocrinol. 30, 872–888. doi:10.1210/me.2016-1039
Ullah, K., Rahman, T. U., Wu, D.-D., Lin, X.-H., Liu, Y., Guo, X.-Y., et al. (2017). Phoenixin-14 concentrations are increased in association with luteinizing hormone and nesfatin-1 concentrations in women with polycystic ovary syndrome. Clin. Chim. Acta. 471, 243–247. doi:10.1016/j.cca.2017.06.013
Umathe, S. N., Bhutada, P. S., Jain, N. S., Shukla, N. R., Mundhada, Y. R., and Dixit, P. V. (2008). Gonadotropin-releasing hormone agonist blocks anxiogenic-like and depressant-like effect of corticotrophin-releasing hormone in mice. Neuropeptides 42, 399–410. doi:10.1016/j.npep.2008.04.005
Valsamakis, G., Arapaki, A., Balafoutas, D., Charmandari, E., and Vlahos, N. F. (2021). Diet-induced hypothalamic inflammation, phoenixin, and subsequent precocious puberty. Nutrients 13, 3460. doi:10.3390/nu13103460
Vesce, S., Rossi, D., Brambilla, L., and Volterra, A. (2007). Glutamate release from astrocytes in physiological conditions and in neurodegenerative disorders characterized by neuroinflammation. Int. Rev. Neurobiol. 82, 57–71. doi:10.1016/s0074-7742(07)82003-4
Wang, J., Zheng, B., Yang, S., Tang, X., Wang, J., and Wei, D. (2020). The protective effects of phoenixin-14 against lipopolysaccharide-induced inflammation and inflammasome activation in astrocytes. Inflamm. Res. 69, 779–787. doi:10.1007/s00011-020-01355-9
Wang, M., Chen, H.-P., Zhai, Y., Jiang, D.-n., Liu, J.-Y., Tian, C.-X., et al. (2019). Phoenixin: expression at different ovarian development stages and effects on genes ralated to reproduction in spotted scat, Scatophagus argus. Comp. Biochem. Physiol. B Biochem. Mol. Biol. 228, 17–25. doi:10.1016/j.cbpb.2018.10.005
Wang, M., Deng, S.-P., Chen, H.-P., Jiang, D.-n., Tian, C.-X., Yang, W., et al. (2018). Phoenixin participated in regulation of food intake and growth in spotted scat, Scatophagus argus. Comp. Biochem. Physiol. B Biochem. Mol. Biol. 226, 36–44. doi:10.1016/j.cbpb.2018.07.007
Wang, S., Liang, R., and Liu, H. (2022). Phoenixin-20 ameliorates brain infarction by promoting microglia M2 polarization in an ischemic stroke model. Metab. Brain Dis. 37, 1517–1526. doi:10.1007/s11011-022-00950-5
Wei, X., Lin, H., Zhang, B., Li, M., Chen, Y., Huang, Y., et al. (2021). Phoenixin-20 prevents ox-LDL-induced attachment of monocytes to human aortic endothelial cells (HAECs): a protective implication in atherosclerosis. Acs Chem. Neurosci. 12, 990–997. doi:10.1021/acschemneuro.0c00787
Xu, Y., Zhang, H., Li, Q., Lao, K., and Wang, Y. (2017). The role of nesfatin-1 expression in letrozole-induced polycystic ovaries in the rat. Gynecol. Endocrinol. 33, 438–441. doi:10.1080/09513590.2017.1290068
Xuan Phuoc, N., Nakamura, T., Osuka, S., Bayasula, B., Nakanishi, N., Kasahara, Y., et al. (2019). Effect of the neuropeptide phoenixin and its receptor GPR173 during folliculogenesis. Reproduction 158, 25–34. doi:10.1530/rep-19-0025
Yañez-Guerra, L. A., Thiel, D., and Jékely, G. (2022). Premetazoan origin of neuropeptide signaling. Mol. Biol. Evol. 39, msac051. doi:10.1093/molbev/msac051
Yang, F., Huang, P., Shi, L., Liu, F., Tang, A., and Xu, S. (2020). Phoenixin 14 inhibits high-fat diet-induced non-alcoholic fatty liver disease in experimental mice. Drug Des. devel. Ther. 14, 3865–3874. doi:10.2147/dddt.s258857
Yang, Y., Lv, Y., Liu, J., Zhang, S., Li, Y., and Shi, Y. (2020). Phoenixin 20 promotes neuronal mitochondrial biogenesis via CREB-PGC-1 alpha pathway. J. Mol. Histol. 51, 173–181. doi:10.1007/s10735-020-09867-8
Yao, B., Lv, J., Du, L., Zhang, H., and Xu, Z. (2021). Phoenixin-14 protects cardiac damages in a streptozotocin-induced diabetes mice model through SIRT3. Arch. Physiol. Biochem. 2021, 1–9. doi:10.1080/13813455.2021.1981946
Yosten, G. L. C., Lyu, R. M., Hsueh, A. J. W., Avsian-Kretchmer, O., Chang, J. K., Tullock, C. W., et al. (2013). A novel reproductive peptide, phoenixin. J. Neuroendocrinol. 25, 206–215. doi:10.1111/j.1365-2826.2012.02381.x
Yuruyen, M., Gultekin, G., Batun, G. C., Yavuzer, H., Akcan, F. E., Doventas, A., et al. (2017). Does plasma phoenixin level associate with cognition? Comparison between subjective memory complaint, mild cognitive impairment, and mild Alzheimer's disease. Int. Psychogeriatr. 29, 1543–1550. doi:10.1017/s1041610217000825
Zandeh-Rahimi, Y., Panahi, N., Hesaraki, S., and Shirazi-Beheshtiha, S. H. (2022). Protective effects of phoenixin-14 peptide in the indomethacin-induced duodenal ulcer: An experimental study. Int. J. Pept. Res. Ther. 28, 43. doi:10.1007/s10989-021-10314-9
Zeng, X., Li, Y., Ma, S., Tang, Y., and Li, H. (2020). Phoenixin-20 ameliorates lipopolysaccharide-induced activation of microglial NLRP3 inflammasome. Neurotox. Res. 38, 785–792. doi:10.1007/s12640-020-00225-w
Keywords: phoenixin, reproduction, inflammation, nesfatin-1, GPR173
Citation: Liang H, Zhao Q, Lv S and Ji X (2022) Regulation and physiological functions of phoenixin. Front. Mol. Biosci. 9:956500. doi: 10.3389/fmolb.2022.956500
Received: 30 May 2022; Accepted: 26 July 2022;
Published: 25 August 2022.
Edited by:
Mohd Sajid Ali, King Saud University, Saudi ArabiaReviewed by:
David Garcia-Galiano, Maimonides Biomedical Research Institute of Cordoba (IMIBIC), SpainTommaso Angelone, University of Calabria, Italy
Copyright © 2022 Liang, Zhao, Lv and Ji. This is an open-access article distributed under the terms of the Creative Commons Attribution License (CC BY). The use, distribution or reproduction in other forums is permitted, provided the original author(s) and the copyright owner(s) are credited and that the original publication in this journal is cited, in accordance with accepted academic practice. No use, distribution or reproduction is permitted which does not comply with these terms.
*Correspondence: Shuangyu Lv, shuangyulv@163.com; Xinying Ji, 10190096@vip.henu.edu.cn