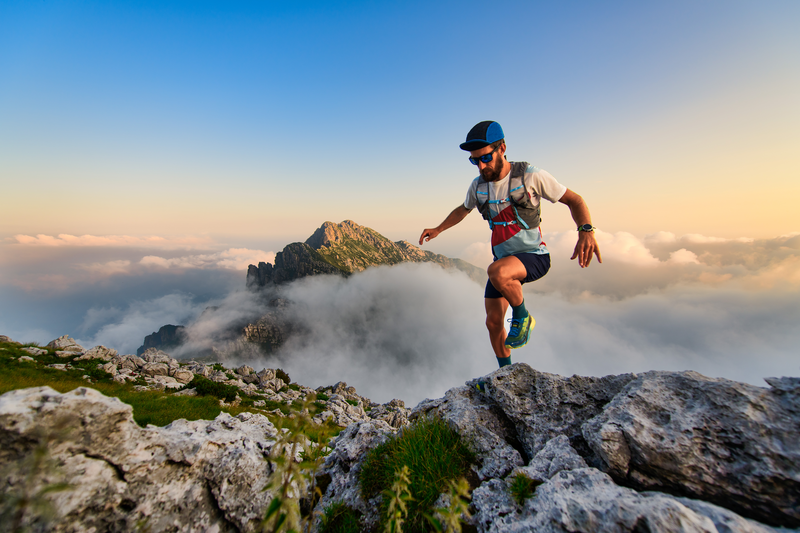
95% of researchers rate our articles as excellent or good
Learn more about the work of our research integrity team to safeguard the quality of each article we publish.
Find out more
BRIEF RESEARCH REPORT article
Front. Mol. Biosci. , 12 August 2022
Sec. Molecular Diagnostics and Therapeutics
Volume 9 - 2022 | https://doi.org/10.3389/fmolb.2022.940449
This article is part of the Research Topic Role of the SUMOylation in Cancer Regulation View all 6 articles
Functional loss of E-cadherin is frequent during tumor progression and occurs through a variety of mechanisms, including proteolytic cleavage. E-cadherin downregulation leads to the conversion of a more malignant phenotype promoting Epithelial to Mesenchymal Transition (EMT). The UBC9/SUMO pathway has been also shown to be involved in the regulation of EMT in different cancers. Here we found an increased expression of UBC9 in the progression of Head and Neck Cancer (HNC) and uncovered a role for UBC9/SUMO in hampering the HPV-mediated E-cadherin cleavage in HNC.
Head and Neck Cancer (HNC) is the sixth most common cancer worldwide (Bray et al., 2018) with a 5-year survival of 50%–60%. It arises in the mucosal epithelium of the oral cavity, oropharynx, larynx, or hypopharynx. Oral cavity and larynx cancers are generally associated with the use of tobacco and excessive alcohol consumption, whereas oropharynx cancers are increasingly attributed to infection with human papillomavirus (HPV), primarily HPV16. Thus, HNC can be classified into HPV-negative and HPV-positive HNC and, whereas the incidence of HPV-negative tumors is slowly declining, those of HPV-positive is rapidly increasing in the western countries. Despite HPV-positive oropharyngeal squamous cell carcinoma (OPC) has been shown to have a more favorable prognosis compared to HPV-negative HNC, HPV-OPC often tends to exhibit large cystic metastases in the cervical lymph nodes, with a clinically and radiologically occult primary tumor(Paver et al., 2020). High-risk HPVs affect numerous cellular pathways, trying to complete its life-cycle and to induce persistent infection. One of the affected pathways is the Epithelial to Mesenchymal transition (EMT). EMT is a physiological process during embryonic development, wound closure, and fibrosis, although in solid tumors, EMT increases their invasiveness and metastatic activity (Yang et al., 2020). Many pathogens affect this pathway targeting in particular E-cadherin. E-cadherin is a cell-cell adhesion transmembrane molecule with a crucial role in cell adhesion and in maintaining an epithelial cell phenotype; thus, loss of E-cadherin promotes EMT. It has been shown that HPV reduces E-cadherin expression by epigenetic and transcriptional regulation (Laurson et al., 2010), whereas other pathogens, such as Helicobacter pylori, promote loss of E-cadherin by inducing its shedding (Weydig et al., 2007). Another pathway affected by HPV is the SUMO pathway, and we recently published that the unique SUMO-conjugating enzyme, UBC9 increases its expression in cervical cancer and in HNC progression (Mattoscio, 2015; Mattoscio et al., 2017). UBC9 has been shown to mainly target nuclear proteins, but also membrane proteins require binding to UBC9 to maintain their localization (Collec et al., 2007; Xiong et al., 2017). Here we show that UBC9 interacts with E-cadherin and the loss of SUMO increases E-cadherin cleavage in HPV-positive HNC cell lines. HPV infection causes increased E-cadherin cleavage and degradation, which affects E-cadherin localization at the plasma membrane.
HPV-positive and negative HNC cell lines were obtained from different sources (Brenner et al., 2010), as already described in our previous studies (Citro et al., 2019; Citro et al., 2020; Citro et al., 2022) and in Supplementary Table S1. Cells were cultured in Dulbecco’s Modified Eagle’s Medium (DMEM) with stable glutamine, sodium pyruvate (EuroClone), 10% fetal bovine serum (FBS), antibiotics (250 μM/ml penicillin and 25 μM/ml streptomycin), and non-essential amino acids (Lonza), in a humidified incubator with 5% CO2 atmosphere at 37°C. Adult human epidermal keratinocytes (HKs) were prepared and maintained as previously described (Mattoscio et al., 2017). Fresh tumors and adjacent normal tissue samples from HNC patients were collected and processed as described in (Citro et al., 2022). Samples were collected from eight HPV-negative and four HPV-positive HNC patients. Six samples were collected from the larynx, three from the oral cavity and the tongue, and three from the oropharynx.
Tissue samples were formalin-fixed and paraffin-embedded (FFPE) according to established procedures (Crosta et al., 2021). Primary antibodies, anti-UBC9, and anti-E-cadherin were incubated overnight at 4°C. DAKO Envision Kit was utilized, and after the chromogenic visualization step using the 3,3′-diaminobenzidine (DAB) chromogen, slides were counterstained with hematoxylin and coverslipped. Images were generated using a bright field microscope Leica DM6 and semi-quantified by pathologist visual scoring of staining. Lymphocytes staining was used as internal control.
Cells were lysed in a sodium dodecyl sulfate (SDS) lysis buffer: a 1:3 mixture of buffer I [5% SDS, 0.15M Tris-HCl (pH 6.8), 30% glycerol] and buffer II [25 mM Tris-HCl (pH 8.3), 50 mM NaCl, 0.5% NP-40, 0.1% SDS, 1 mM EDTA], containing 0.5 mM N-ethylmaleimide and protease inhibitors. After lysis, an equal amount of protein for each sample was resuspended in denaturing sample loading buffer, separated on SDS-PAGE gel and immunoblotted with the indicated antibodies (Supplementary Table S2). Membranes were then incubated with the appropriate horseradish peroxidase (HRP) secondary antibodies and the signal was acquired with Chemidoc (Bio-Rad). Densitometric analysis of the intensity of the protein bands was preformed using ImageJ software (National Institutes of Health) and standardized to the housekeeper levels.
RNA was extracted from cells with the Quick-RNA MiniPrep kit (Zymo research). cDNA was generated by reverse transcription-PCR with reverse transcriptase (NEB). Relative levels of specific mRNAs were determined with the fast SYBR Green detection chemistry system (NEB) using a QuantStudio 6 Fast Real-Time PCR system (ThermoFisher).
HNC cell lines were lysed in Tris-HCl lysis buffer (50 mM Tris-HCl pH 7.6, 150 mM NaCl, 0.8% NP-40, 10% glycerol, 1 mM EDTA, proteases inhibitors, 0.5 mM NEM). Immunoprecipitation was performed using protein extracts incubated with the indicated antibodies o/n at 4°C. Samples were loaded on SDS-PAGE gel and immunoblotted with the indicated antibodies. The total extracts (input) were loaded as control.
PLXSN HPV16E6/E7 and inducible shUBC9 pTRIPZ lentiviral vector (OpenBiosystem) were previously described (Mattoscio et al., 2017; Citro et al., 2013). HNC cell lines were transfected with Lipofectamine 2000 (Thermo Fisher Scientific), following manufacturers’ instruction with siRNA oligos: siLuciferase (siLUC), siE-cadherin (5′-CAGACAAAGACCAGGACUATT-3′), or siSUMO1 (Santa Cruz Biotechnology).
Cells were seeded on coverslips and fixed with 4% PFA. Anti-E-cadherin (rabbit) and anti-β-catenin (mouse) were incubated o/n at 4°C. Goat anti-rabbit Alexa 488 and donkey anti-mouse Alexa 647 were used as secondary antibody and nuclei were stained with DAPI. Coverslips were then mounted and pictures were taken using a Leica SP8 AOBS confocal microscope. ImageJ JACoP plugin was used to measure colocalization.
RSEM normalized expression data were extracted from the TCGA PanCancer Atlas on the cBioPortal (Cerami et al., 2012; Gao et al., 2013). Patient samples were grouped as HPV+ and HPV−. This resulted in 72 HPV+ and 410 HPV−samples with data available for the HNC gene expression analysis. Boxplot comparisons of gene expression and statistical analysis were performed using unpaired t test or ANOVA multiple comparison with GraphPad Software (San Diego, California, United States).
The expression of UBC9 is linked to tumorigenesis and increases in different tumors (Lee et al., 2017; Mattoscio, 2015; Mattoscio et al., 2017). We also observed an increased expression of both mRNA (Figure 1A) and protein (Figures 1B,C) UBC9 levels in fresh HNC tissue samples, compared to normal adjacent tissues. This was confirmed by IHC in HNC tissue samples. As Figure 1D shows, UBC9 levels increased from normal tissue to dysplasia, and even more in neoplastic OPC tissue samples. Interestingly, as shown in Figure 1E, high-grade dysplasia and neoplastic tissues presented an intense and diffuse UBC9 staining in both nucleus and cytoplasm, whereas normal adjacent epithelium showed a really faint UBC9 staining.
FIGURE 1. Analysis of UBC9 expression in normal and tumoral HNC tissues. (A) Total RNAs from frozen tissue samples of HNC patients (eight HPV-negative and four HPV-positive) were isolated for RT-qPCR and UBC9 expression which were reported as means ± SEM of fold changes. ***, p < 0.001 (unpaired t test). (B) Protein fraction was extracted from frozen tissue samples of HPV-negative HNC patients and analyzed by immunoblotting with the indicated antibodies. (C) Graph showing means ± SEM of optical density analysis from immunoblotting of UBC9 expression from eight frozen HNC tissue samples. (D) Percentage of UBC9 positivity in nucleus and cytoplasm in dysplastic, tumoral, and matched adjacent mucosa OPC specimens; *, p < 0.05, **, p < 0.01 (two-way ANOVA multiple comparison test). n = 105 (tumor, 56 HPV-negative and 49 HPV-positive), n = 36 (dysplasia, 21 HPV-negative, and 15 HPV-positive), n = 124 (adjacent mucosa, 84 HPV-negative, and 40 HPV-positive). (E) Representative IHC images of FFPE stained with anti-UBC9 antibody from normal (*) and neoplastic OPC tissues (right side of the image) and from high-grade dysplasia, as indicated. Scale bars = 250 μm.
Binding to UBC9 is required for the correct localization at the plasma membrane of different proteins (Collec et al., 2007; Xiong et al., 2017). As shown in Figure 2A and Supplementary Figure S1A, UBC9 was able to specifically interact with E-cadherin preferentially in HPV-positive HNC cell lines, and only in a small portion in HPV-negative cell lines, in accordance with our previous findings showing that HPV upregulates UBC9 expression (Mattoscio et al., 2017). E-cadherin has been previously found to be SUMOylated preferentially by SUMO1 (Ha et al., 2016) and we also confirmed this by co-immunoprecipitation (Supplementary Figure S1B). Thus, we evaluated the effect of SUMO1 knock-down (Supplementary Figure S1C) on migration in both HPV-positive and HPV-negative HNC cell lines. As Figures 2B,C show, we noticed that SUMO1 silencing was decreasing cell motility only in HPV-negative HNC cell lines, probably through an indirect regulation of E-cadherin transcription (Figure 2D). Interestingly, SUMO1 knock-down did not affect E-cadherin transcription in HPV-positive HNC cell lines (Figure 2D), with no significant change in cell migration rate (Figures 2B,C). All these data suggest that E-cadherin is differentially regulated by SUMO in HPV-positive compared to HPV-negative cell lines.
FIGURE 2. UBC9 interaction with E-cadherin and its transcriptional regulation. (A) HPV-positive 93VU147T and HPV-negative UMSCC10A cell lines were transduced with an inducible shUBC9 lentiviral vector, and treated or not with doxycycline for 72 h. Cells were then harvested and whole cell extracts were subjected to anti-UBC9 immunoprecipitation (IP). Immunoblotting with the indicated antibodies was then performed and whole cell extracts were used as input controls. (B) Phase-contrast pictures of scratch wound healing assay performed on HPV-positive 93VU147T and HPV-negative UMSCC19, transfected for 72 h with SUMO1 or LUC siRNA at time 0 and 18 h after the scratch. (C) Graph represented the percentage of wound closure (means ± SEM) of SUMO1 siRNA cells as compared with LUC siRNA transfected; ****, p < 0.0001, ns, not significant (unpaired t test). (D) HPV-positive HNC cell lines (UMSCC47, UPCISCC154, 93VU147T) and HPV-negative (UMSCC10A, UMSCC19, UMSCC23) cell lines were transfected for 72 h with specific siRNA against SUMO1 or Luciferase as control. After transfection, total RNAs were isolated for RT-qPCR and reported as means ± SEM of fold changes of at least three independent experiments; *, p < 0.05, ns, not significant (unpaired t test).
Since UBC9 shows more affinity in binding E-cadherin in HPV-positive cell lines and since lack of SUMOylation did not affect E-cadherin transcription, we asked whether SUMO was influencing the expression of E-cadherin at post-transcriptional level in HPV-positive cell lines. We first assessed the expression of E-cadherin in our panel of cell lines. As shown in Figures 3A,B, we could not detect any significant difference in the expression of E-cadherin at both mRNA and protein level between HPV-positive and negative HNC cell lines, and only a slight decrease in HPV-positive compared to HPV-negative HNC samples from TCGA dataset (Figure 3C). E-cadherin is known to undergo protein cleavage at the intracellular domain upon the activation of different proteolytic events (Steinhusen et al., 2001; Yoo et al., 2012). We transduced E6/E7 in the HPV-negative cell line UMSCC10A (Supplementary Figure S1D) and performed Western blot analysis using an antibody that recognizes the cytoplasmic domain of E-cadherin. As Figure 4A shows, HPV oncoproteins induced the formation of two different E-cadherin peptides at 50 and 30 kDa. We confirmed by E-cadherin silencing (Supplementary Figure S1E) that the 50 and 30 kDa products were specific products of E-cadherin cleavage (Figure 4B compared lane 1 to lane 3 and lane 5 to lane 7). We then treated UMSCC10A cells with staurosporine, which is known to induce caspase-dependent E-cadherin cleavage (Yoo et al., 2012). As shown in Figure 4B, staurosporine was able to cleave E-cadherin into two main products at 30 and 27 kDa, reducing both full length and the 50 kDa bands of E-cadherin (Figure 4B compared lane 1 to lane 2 and lane 5 to lane 6), suggesting that the 27 kDa product derived from the subsequent cleavage of the 50 kDa peptide. Interestingly, SUMO1 knock-down increased E-cadherin cleavage preferentially in HPV-positive cell lines (Figure 4C), hinting that in HPV-positive cell lines UBC9 and SUMO1 are needed to maintain E-cadherin localization at the plasma membrane.
FIGURE 3. Analysis of E-cadherin expression in HPV-positive and HPV-negative HNC. (A) HNC cell lines were lysed and analyzed by immunoblotting with the indicated antibodies (FL, full-length). (B) Total RNAs from HNC cell lines were isolated for RT-qPCR. E-cadherin expression was normalized to RpP0 and shown as means ± SEM of at least three independent experiments. (C) Normalized RNA-seq data were extracted from TCGA database and divided in HPV-positive and HPV-negative HNC cohorts. *, p < 0.05 (unpaired t test).
FIGURE 4. HPV16 and SUMO1 regulation of E-cadherin cleavage and localization. (A) UMSCC10A cells were transduced with empty or HPV16E6/E7 recombinant retroviral vectors. Lysates were analyzed by immunoblotting with the indicated antibodies. Graph represented densitometric analysis and reported as means ± SEM of fold changes, *, p < 0.05 (two-way ANOVA multiple comparison test). n = 7.(B) UMSCC10A cells transduced with empty or HPV16E6/E7 recombinant retroviral vectors, were transfected with specific siRNA against E-cadherin or Luciferase as control. 72 h after transfection, cells were treated or not for 6 h with 1 μM staurosporine, lysed and analyzed by immunoblotting with the indicated antibodies (FL, full-length). Densitometric analysis was performed and optical density values normalized to loading control were reported below each band. (C) HPV-positive HNC cell lines (UMSCC47) and HPV-negative (UMSCC10A) cell lines were transfected for 72 h with specific siRNA against SUMO1 or Luciferase as control. Cells were then lysed and analyzed by immunoblotting with the indicated antibodies. (D) UMSCC10A cells were transduced with empty or HPV16E6/E7 recombinant retroviral vectors. Cells were then seeded and treated with 10 μM MG132 for 16 h, lysed and analyzed by immunoblotting with the indicated antibodies. (E) Representative IHC images of FFPE from tumoral HN oropharyngeal HPV-positive or negative tissues, as indicated, stained with anti-E-cadherin antibody, presented at two different magnifications (40x and 20x).
It has been previously shown that E-cadherin products derived from cleavage are degraded by an intracellular proteolytic pathway (Ito et al., 1999). We then treated UMSCC10A cells, transduced or not with E6/E7 oncoproteins, with the proteasome inhibitor MG132. As shown in Figure 4D, MG132 increased the 50 kDa and the 30 kDa products in UMSCC10A (compare lane 1 to lane 2) and even more in cells transduced with E6/E7 (compare lane 3 to 4), without changing the full-length form, confirming that E-cadherin cleaved products undergo protein degradation.
We finally investigated whether the increased E-cadherin proteolysis detected in HPV-positive compared to HPV-negative HNC cell lines, was detectable also in tumor samples. We thus screened for IHC different HNC specimens derived from HPV-positive and HPV-negative HNC and, as shown in Figure 4E, we found that in HPV-negative HNC, E-cadherin was preferentially present at the cell surface; HPV-positive HNC showed an intracellular staining of E-cadherin. Moreover, as shown in Supplementary Figure S1F, we confirmed that also in human keratinocytes transduced with HPV16 E6/E7, the presence of the two oncoproteins decreased the integrity of adherent junctions, leading to the dissociation of E-cadherin from β-catenin, which is a consequence of E-cadherin cleavage (Ito et al., 1999). These data show that cell surface E-cadherin is reduced in HPV-positive HNC, and this can be explained by its increased proteolysis.
Confirming our previous data (Mattoscio et al., 2017), here we show that in HNC, UBC9 expression increases with the progression of the tumor. Interestingly, we also noticed that together with the increase of nuclear UBC9 staining, in dysplastic and even more in tumoral tissue, UBC9 appeared in the cytoplasm. Previous data are consistent with these results, as UBC9 protein has been found in the cytosol, nucleus, membranes, and endoplasmic reticulum in a variety of cell types (Gupta et al., 2014), and UBC9 binding has been shown to be crucial for the correct localization at the plasma membrane of different proteins (Collec et al., 2007; Xiong et al., 2017). It has been previously shown that, both UBC9 and SUMO1, promote cell migration, increasing invasion and metastasis in different tumors (Castillo-Lluva et al., 2010; Zhu et al., 2010; LI et al., 2013). Interestingly, here we show that loss of SUMO1 confers a decreased mobility only in HPV-negative HNC cell lines with change in E-cadherin transcription, consistent with the role of the SUMO pathway in affecting E-cadherin transcription through secondary effects (Bogachek et al., 2015). The loss of intercellular adhesion mediated by E-cadherin is a critical change that occurs during the evolution of cancer into invasive disease. HPV16 E6/E7 oncoproteins have been shown to negatively regulate E-cadherin by different mechanisms (Laurson et al., 2010; Caberg et al., 2008). Lower E-cadherin expression has been associated with a higher risk of distant metastasis (Lefevre et al., 2017), but different studies could not find any association between E-cadherin expression and HPV status in OPC (Lefevre et al., 2017; Mohamed et al., 2020). We also could not detect any differences in the expression of full-length E-cadherin between HPV-positive and HPV-negative HNC cell lines and only a small difference in the mRNA expression of E-cadherin between HPV-positive and HPV-negative HNC tissue samples. Interestingly, HNC cell lines transduced with HPV16 E6/E7 showed an increased expression of cleaved E-cadherin peptides, which are targets for proteolytic degradation, in accordance with the evidences showing that HPV increases the activity and the expression of both caspases and metalloproteinases (Herbster et al., 2018; Manzo-Merino et al., 2014). On the other side, HPV is known to increase UBC9 expression (Mattoscio et al., 2017) and this seems to promote the selective binding of E-cadherin to UBC9 in HPV-positive HNC cell lines and protects E-cadherin from its proteolysis. All these data show a different behavior of the SUMO/UBC9 pathway between HPV-positive and negative HNC cell lines. In HPV-negative cells, lack of the SUMO/UBC9 pathway decreases cell motility, as seen in other cancers, and this seems in part due to an increased transcription of E-cadherin. On the contrary, in HPV-positive HNC cell lines, UBC9 is able to interact with E-cadherin and this interaction somehow protects E-cadherin from its HPV-mediated proteolysis. Many evidences indicate that peptides derived from the proteolysis of E-cadherin might promote tumor growth, survival, and motility. In this study, we found a role for the SUMO/UBC9 pathway in interfering with HPV-mediated E-cadherin cleavage, which might transform this tumor suppressor into an oncogenic factor. Moreover, we revealed for the first time, in accordance with previous findings (Hubert et al., 2005; Rodríguez-Sastre et al., 2005) showing an increased cytoplasmatic E-cadherin staining in the progression of cervical cancer, that in HPV-positive HNC specimens E-cadherin staining is prevalently cytoplasmatic compared to HPV-negative HNC. These data uncover an important molecular feature of HPV-positive HNC that might improve the diagnosis and therapy of these tumors.
The original contributions presented in the study are included in the article/Supplementary Material, further inquiries can be directed to the corresponding authors.
The studies involving human participants were reviewed and approved by the study was carried out in accordance with the recommendations of IEO Biobank for translational medicine (B4MED). The protocol was approved by the IEO committee. All subjects gave written informed consent in accordance with the Declaration of Helsinki. The patients/participants provided their written informed consent to participate in this study.
Conceptualization: MS, SiC, and SuC; Data and materials acquisition: MS, SiC, MC, FM, CM, FP, ML, VB, DL, MT, and MA; Data analysis: MS, SiC, MC, and SuC; Writing and editing and review: MS, SiC, MC, and SuC.
This study was funded by HeadLace within the ERANET cofund joint call 2016–2017 “EU-LAC Health.” SiC is currently a recipient of a FUV (Fondazione Umberto Veronesi) fellowship. This work was partially supported by the Italian Ministry of Health with Ricerca Corrente and 5 × 1000 funds.
We thank IEO Biobank and Alessandra Cavallon (IEO) for FFPE specimen preparation.
The authors declare that the research was conducted in the absence of any commercial or financial relationships that could be construed as a potential conflict of interest.
All claims expressed in this article are solely those of the authors and do not necessarily represent those of their affiliated organizations, or those of the publisher, the editors, and the reviewers. Any product that may be evaluated in this article, or claim that may be made by its manufacturer, is not guaranteed or endorsed by the publisher.
The Supplementary Material for this article can be found online at: https://www.frontiersin.org/articles/10.3389/fmolb.2022.940449/full#supplementary-material
Bogachek, M. V., De Andrade, J. P., and Weigel, R. J. (2015). Regulation of epithelial-mesenchymal transition through sumoylation of transcription factors. Cancer Res. 75, 11–15. doi:10.1158/0008-5472.CAN-14-2824
Bray, F., Ferlay, J., Soerjomataram, I., Siegel, R. L., Torre, L. A., and Jemal, A. (2018). Global cancer statistics 2018: GLOBOCAN estimates of incidence and mortality worldwide for 36 cancers in 185 countries. Ca. Cancer J. Clin. 68, 394–424. doi:10.3322/caac.21492
Brenner, J. C., Graham, M. P., Kumar, B., Saunders, L. M., Kupfer, R., Lyons, R. H., et al. (2010). Genotyping of 73 UM-SCC head and neck squamous cell carcinoma cell lines. Head. Neck 32 (4), 417–426. doi:10.1002/hed.21198
Caberg, J.-H. D., Hubert, P. M., Begon, D. Y., Herfs, M. F., Roncarati, P. J., Boniver, J. J., et al. (2008). Silencing of E7 oncogene restores functional E-cadherin expression in human papillomavirus 16-transformed keratinocytes. Carcinogenesis 29 (7), 1441–1447. doi:10.1093/carcin/bgn145
Castillo-Lluva, S., Tatham, M. H., Jones, R. C., Jaffray, E. G., Edmondson, R. D., Hay, R. T., et al. (2010). SUMOylation of the GTPase Rac1 is required for optimal cell migration. Nat. Cell Biol. 12 (11), 1078–1085. doi:10.1038/ncb2112
Cerami, E., Gao, J., Dogrusoz, U., Gross, B. E., Sumer, S. O., Aksoy, B. A., et al. (2012). The cBio Cancer Genomics Portal: An open platform for exploring multidimensional cancer genomics data. Cancer Discov. 2 (5), 401–404. doi:10.1158/2159-8290.CD-12-0095
Citro, S., Bellini, A., Medda, A., Sabatini, M. E., Tagliabue, M., Chu, F., et al. (2020). Human papilloma virus increases ΔNp63α expression in head and neck squamous cell carcinoma. Front. Cell. Infect. Microbiol. 10, 143. doi:10.3389/fcimb.2020.00143
Citro, S., Bellini, A., Miccolo, C., Ghiani, L., Carey, T. E., and Chiocca, S. (2019). Synergistic antitumour activity of HDAC inhibitor SAHA and EGFR inhibitor gefitinib in head and neck cancer: a key role for ΔNp63α. Br. J. Cancer 120 (6), 658–667. doi:10.1038/s41416-019-0394-9
Citro, S., Jaffray, E., Hay, R. T., Seiser, C., and Chiocca, S. (2013). A role for paralog-specific sumoylation in histone deacetylase 1 stability. J. Mol. Cell Biol. 5 (6), 416–427. doi:10.1093/jmcb/mjt032
Citro, S., Miccolo, C., Medda, A., Ghiani, L., Tagliabue, M., Ansarin, M., et al. (2022). HPV-mediated regulation of SMAD4 modulates the DNA damage response in head and neck cancer. J. Exp. Clin. Cancer Res. 41 (1), 59. doi:10.1186/s13046-022-02258-9
Collec, E., El Nemer, W., Gauthier, E., Gane, P., Lecomte, M. C., Dhermy, D., et al. (2007). Ubc9 interacts with Lu/BCAM adhesion glycoproteins and regulates their stability at the membrane of polarized MDCK cells. Biochem. J. 402 (2), 311–319. doi:10.1042/BJ20060861
Crosta, S., Boldorini, R., Bono, F., Brambilla, V., Dainese, E., Fusco, N., et al. (2021). PD-L1 testing and squamous cell carcinoma of the head and neck: A multicenter study on the diagnostic reproducibility of different protocols. Cancers 13 (2), 292. doi:10.3390/cancers13020292
Gao, J., Aksoy, B. A., Dogrusoz, U., Dresdner, G., Gross, B., Sumer, S. O., et al. (2013). Integrative analysis of complex cancer genomics and clinical profiles using the cBioPortal. Sci. Signal. 6 (269), pl1. doi:10.1126/scisignal.2004088
Gupta, M. K., Gulick, J., Liu, R., Wang, X., Molkentin, J. D., and Robbins, J. (2014). Sumo E2 enzyme UBC9 is required for efficient protein quality control in cardiomyocytes. Circ. Res. 115, 721–729. doi:10.1161/CIRCRESAHA.115.304760
Ha, H. L., Kwon, T., Bak, I. S., Erikson, R. L., Kim, B. Y., and Yu, D. Y. (2016). IGF-II induced by hepatitis B virus X protein regulates EMT via SUMO mediated loss of E- cadherin in mice. Oncotarget 7, 56944–56957. doi:10.18632/oncotarget.10922
Herbster, S., Paladino, A., de Freitas, S., and Boccardo, E. (2018). Alterations in the expression and activity of extracellular matrix components in HPV-associated infections and diseases. Clinics 73 (Suppl. 1), e551s. doi:10.6061/clinics/2018/e551s
Hubert, P., Caberg, J. H., Gilles, C., Bousarghin, L., Franzen-Detrooz, E., Boniver, J., et al. (2005). E-cadherin-dependent adhesion of dendritic and Langerhans cells to keratinocytes is defective in cervical human papillomavirus-associated (pre)neoplastic lesions. J. Pathol. 206 (3), 346–355. doi:10.1002/path.1771
Ito, K., Okamoto, I., ArakiN., , Kawano, Y., NakaoM., , Fujiyama, S., et al. (1999). Calcium influx triggers the sequential proteolysis of extracellular and cytoplasmic domains of E-cadherin, leading to loss of β-catenin from cell – cell contacts. Oncogene 18 (50), 7080–7090. doi:10.1038/sj.onc.1203191
Laurson, J., Khan, S., Chung, R., Cross, K., and Raj, K. (2010). Epigenetic repression of E-cadherin by human papillomavirus 16 E7 protein. Carcinogenesis 31 (5), 918–926. doi:10.1093/carcin/bgq027
Lee, J. S., Choi, H. J., and Baek, S. H. (2017). “Sumoylation and its contribution to cancer, Adv. Exp. Med. Biol., 283–298. doi:10.1007/978-3-319-50044-7_17
Lefevre, M., Rousseau, A., Rayon, T., Dalstein, V., Clavel, C., Beby-Defaux, A., et al. (2017). Epithelial to mesenchymal transition and HPV infection in squamous cell oropharyngeal carcinomas: The papillophar study. Br. J. Cancer 116 (3), 362–369. doi:10.1038/bjc.2016.434
Li, H., Niu, H., Peng, Y., Wang, J., and He, P. (2013). Ubc9 promotes invasion and metastasis of lung cancer cells. Oncol. Rep. 29 (4), 1588–1594. doi:10.3892/or.2013.2268
Manzo-Merino, J., Massimi, P., Lizano, M., and Banks, L. (2014). The human papillomavirus (HPV) E6 oncoproteins promotes nuclear localization of active caspase 8. Virology 450 (451), 146–152. doi:10.1016/j.virol.2013.12.013
Mattoscio, D., Casadio, C., Fumagalli, M., Sideri, M., and Chiocca, S. (2015). The SUMO conjugating enzyme UBC9 as a biomarker for cervical HPV infections. ecancermedicalscience 9, 534. doi:10.3332/ecancer.2015.534
Mattoscio, D., Casadio, C., Miccolo, C., Maffini, F., Raimondi, A., Tacchetti, C., et al. (2017). Autophagy regulates UBC9 levels during viral-mediated tumorigenesis. PLoS Pathog. 13 (3), e1006262. doi:10.1371/journal.ppat.1006262
Mohamed, H., Haglund, C., Jouhi, L., Atula, T., Hagstrom, J., and Makitie, A. (2020). Expression and role of E-cadherin, β-catenin, and vimentin in human papillomavirus–positive and human papillomavirus–negative oropharyngeal squamous cell carcinoma. J. Histochem. Cytochem. 68 (9), 595–606. doi:10.1369/0022155420950841
Paver, E. C., Currie, A. M., Gupta, R., and Dahlstrom, J. E. (2020). Human papilloma virus related squamous cell carcinomas of the head and neck: diagnosis, clinical implications and detection of HPV. Pathology 52 (2), 179–191. doi:10.1016/j.pathol.2019.10.008
Rodríguez-Sastre, M. A., Gonzalez-Maya, L., Delgado, R., Lizano, M., Tsubaki, G., Mohar, A., et al. (2005). Abnormal distribution of E-cadherin and β-catenin in different histologic types of cancer of the uterine cervix. Gynecol. Oncol. 97 (2), 330–336. doi:10.1016/j.ygyno.2004.12.062
Steinhusen, U., Badock, V., TaubeR, R., Bommert, K., and Huber, O. (2001). Cleavage and shedding of E-cadherin after induction of apoptosis. J. Biol. Chem. 276 (7), 4972–4980. doi:10.1074/jbc.M006102200
Weydig, C., Starzinski-Powitz, A., Carra, G., Lower, J., and Wessler, S. (2007). CagA-independent disruption of adherence junction complexes involves E-cadherin shedding and implies multiple steps in Helicobacter pylori pathogenicity. Exp. Cell Res. 313 (16), 459–3471. doi:10.1016/j.yexcr.2007.07.015
Xiong, Y., Ye, C., Yang, N., Li, M., and Liu, H. (2017). Ubc9 binds to ADAP and is required for Rap1 membrane recruitment, Rac1 activation, and integrin-mediated T cell adhesion. J. Immunol. 199 (12), 4142–4154. doi:10.4049/jimmunol.1700572
Yang, J., Antin, P., Berx, G., Blanpain, C., Brabletz, T., Bronner, M., et al. (2020). Guidelines and definitions for research on epithelial–mesenchymal transition. Nat. Rev. Mol. Cell Biol. 21 (6), 341–352. doi:10.1038/s41580-020-0237-9
Yoo, C. B., Yun, S. M., Jo, C., and Koh, Y. H. (2012). γ-Secretase-Dependent cleavage of E-cadherin by staurosporine in breast cancer cells. Cell Commun. Adhes. 19 (1), 11–16. doi:10.3109/15419061.2012.665969
Keywords: head and neck cancer, head and neck squamous cell carcinoma, HPV, SUMO, Ubc9, E-cadherin
Citation: Sabatini ME, Compagnoni M, Maffini F, Miccolo C, Pagni F, Lombardi M, Brambilla V, Lepanto D, Tagliabue M, Ansarin M, Citro S and Chiocca S (2022) The UBC9/SUMO pathway affects E-cadherin cleavage in HPV-positive head and neck cancer. Front. Mol. Biosci. 9:940449. doi: 10.3389/fmolb.2022.940449
Received: 10 May 2022; Accepted: 30 June 2022;
Published: 12 August 2022.
Edited by:
Giovanni Smaldone, IRCCS SDN, ItalyReviewed by:
Narendra Thapa, University of Wisconsin-Madison, United StatesCopyright © 2022 Sabatini, Compagnoni, Maffini, Miccolo, Pagni, Lombardi, Brambilla, Lepanto, Tagliabue, Ansarin, Citro and Chiocca. This is an open-access article distributed under the terms of the Creative Commons Attribution License (CC BY). The use, distribution or reproduction in other forums is permitted, provided the original author(s) and the copyright owner(s) are credited and that the original publication in this journal is cited, in accordance with accepted academic practice. No use, distribution or reproduction is permitted which does not comply with these terms.
*Correspondence: Simona Citro, c2ltb25hLmNpdHJvQGllby5pdA==; Susanna Chiocca, c3VzYW5uYS5jaGlvY2NhQGllby5pdA==
†These authors share first authorship
Disclaimer: All claims expressed in this article are solely those of the authors and do not necessarily represent those of their affiliated organizations, or those of the publisher, the editors and the reviewers. Any product that may be evaluated in this article or claim that may be made by its manufacturer is not guaranteed or endorsed by the publisher.
Research integrity at Frontiers
Learn more about the work of our research integrity team to safeguard the quality of each article we publish.