- 1Laboratório de Planejamento e Desenvolvimento de Fármacos, Instituto de Ciências Exatas e Naturais, Universidade Federal do Pará, Belém, Brazil
- 2Institute of Biodiversity, Federal University of Western Pará, Santarém, Brazil
- 3Structural Bioinformatics Laboratory, Biochemistry, Faculty of Science and Engineering, Åbo Akademi University, Turku, Finland
Peptidoglycan is a cross-linked polymer responsible for maintaining the bacterial cell wall integrity and morphology in Gram-negative and Gram-positive bacteria. The peptidoglycan pathway consists of the enzymatic reactions held in three steps: cytoplasmic, membrane-associated, and periplasmic. The Mur enzymes (MurA-MurF) are involved in a cytoplasmic stage. The UDP-N-acetylglucosamine enolpyruvyl transferase (MurA) enzyme is responsible for transferring the enolpyruvate group from phosphoenolpyruvate (PEP) to UDP-N-acetylglucosamine (UNAG) to form UDP-N-acetylglucosamine enolpyruvate (EP-UNAG). Fosfomycin is a natural product analogous to PEP that acts on the MurA target enzyme via binding covalently to the key cysteine residue in the active site. Similar to fosfomycin, other MurA covalent inhibitors have been described with a warhead in their structure that forms a covalent bond with the molecular target. In MurA, the nucleophilic thiolate of Cys115 is pointed as the main group involved in the warhead binding. Thus, in this minireview, we briefly describe the main recent advances in the design of MurA covalent inhibitors.
Introduction: MurA Molecular Function
The bacterial peptidoglycan is an extensive mesh-like macromolecule, a cross-linked polymer or a net-like layer, essential for maintaining the bacterial cell wall integrity and morphology (Hsu et al., 2019; Pazos and Peters, 2019; Egan et al., 2020; Pham et al., 2021). Peptidoglycan is present in both Gram-negative and Gram-positive bacteria, being mainly single-layered in diderm bacteria (e.g., Escherichia coli) and multilayered in monoderm bacteria (e.g., Bacillus subtilis), respectively (Gupta, 2011; Egan et al., 2020; Megrian et al., 2020).
UDP-N-acetylglucosamine enolpyruvyl transferase (MurA) is a cytoplasmatic enzyme from the peptidoglycan pathway responsible for catalyzing the transfer of enolpyruvate from phosphoenolpyruvate to UDP-N-acetylglucosamine to form UDP-N-acetylglucosamine enolpyruvate and release inorganic phosphate (Evangelina et al., 2021). Since the discovery of fosfomycin antibiotic, multiple studies have been carried out on covalent inhibitors (Mihalovits et al., 2019; Hamilton et al., 2020). Recently, the design of new covalent inhibitors has been receiving considerable attention (Mihalovits et al., 2021).
The peptidoglycan pathway can be divided into three different stages: cytoplasmic, membrane-associated, and periplasmic (Egan et al., 2020). In cytoplasmic stage, the Mur enzyme family (MurA-MurF) is responsible for the conversion of UDP-N-acetylMurAmyl-pentapeptide from UDP-N-acetyl-glucosamine as shown in Figure 1 (Laddomada et al., 2016; Egan et al., 2020).
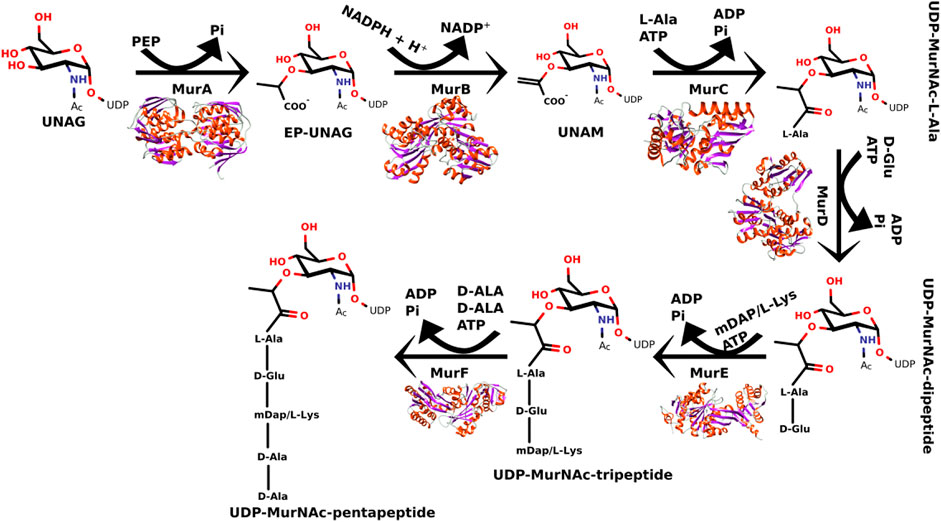
FIGURE 1. Steps of catalytic reactions performed by the MurA-MurF enzymes in the peptidoglycan biosynthesis.
The MurA enzyme is responsible for transferring enolpyruvate group from phosphoenolpyruvate to UDP-N-acetylglucosamine which leads to the formation of UDP-N-acetylglucosamine enolpyruvate (Kumar et al., 2020). Since the phosphoenolpyruvate substrate acts similarly in other enzymes (e.g., 3-deoxy-D-manno-2-octulosonate-8-phosphate synthase (KDO8PS) (Vainer et al., 2005), 3-deoxy-D-arabino-heptulosonate-7-phosphate synthase (DAHPS) (Burschowsky et al., 2018), 5-enolpyruvylshikimate-3-phosphate synthase (EPSPS) (De Oliveira et al., 2020), and N-acetylneuraminic acid (NeuB) synthase (Popović et al., 2019), it has been shown that analogs of the natural substrate may potentially inhibit MurA (Araújo et al., 2019; De Oliveira et al., 2020). Fosfomycin, cis-1,2-epoxypropyl phosphonic acid, is a MurA inhibitor, which is an antibiotic analogous to PEP used for the treatment of cystitis (Zhanel et al., 2018; Aghamali et al., 2019; Simon et al., 2021).
MurA and its Covalent Inhibition
Covalent inhibitors can chemically modify the active site of a target protein through covalent binding (Keeley et al., 2018; Martin et al., 2019; Ray and Murkin, 2019). It was believed that covalent inhibitors could pose a high risk to human health due to their toxicity. However, since the last century, a high number of commercially available covalent drugs used to treat various human diseases have been released (Ray and Murkin, 2019; Mihalovits et al., 2021).
The interaction between a drug and its target through covalent binding can be achieved in two steps: first, a reversible interaction when the inhibitor binds to the protein active site forming the equilibrium bond. In the second step, the warhead covalent inhibitor forms a covalent bond with its target (Ábrányi-Balogh et al., 2018; Sutanto et al., 2020). Among other variables, the strength of this bond governs the reversibility of the complexes formed. In the case of the MurA enzyme, the highly nucleophilic thiolate of Cys115 is utilized to bind the warheads (Figure 2).
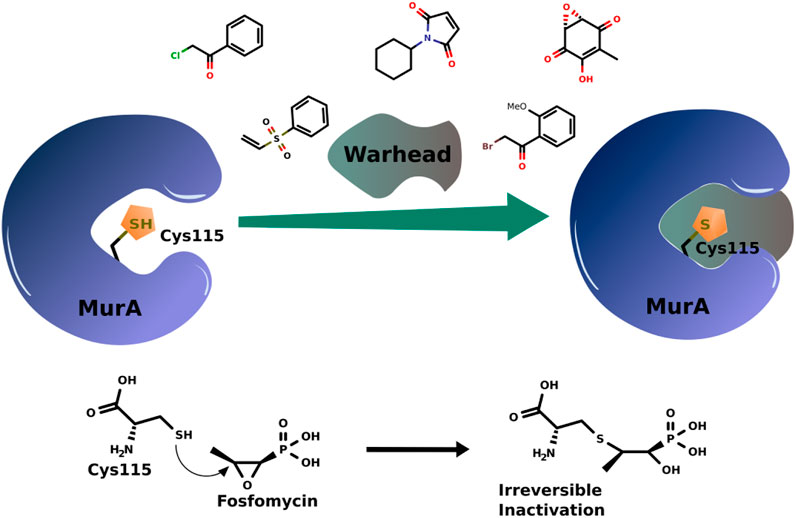
FIGURE 2. Scheme of covalent bond formation in MurA with some examples of warhead covalent inhibitors and the action mechanism of fosfomycin.
Fosfomycin has an oxirane warhead that opens after binding to Cys115 (Figure 2). After years, it is still the most effective and only marketed compound acting on MurA. Thus, the revision presented here aims to give a glimpse of advances in the design of MurA covalent inhibitors.
The problem surrounding fosfomycin, being the only inhibitor commercially available, since its discovery makes increasing number of studies to be carried out to find other covalent inhibitors capable of inhibiting MurA in different types of organisms (Bachelier et al., 2006; Shigetomi et al., 2010; Elsebai et al., 2016; Hamilton et al., 2020; Riyana et al., 2020; Petri et al., 2021). Thus, the enzyme, being one of the main targets of peptidoglycan biosynthesis, has been mostly studied for the development of covalent inhibitors (Table 1).
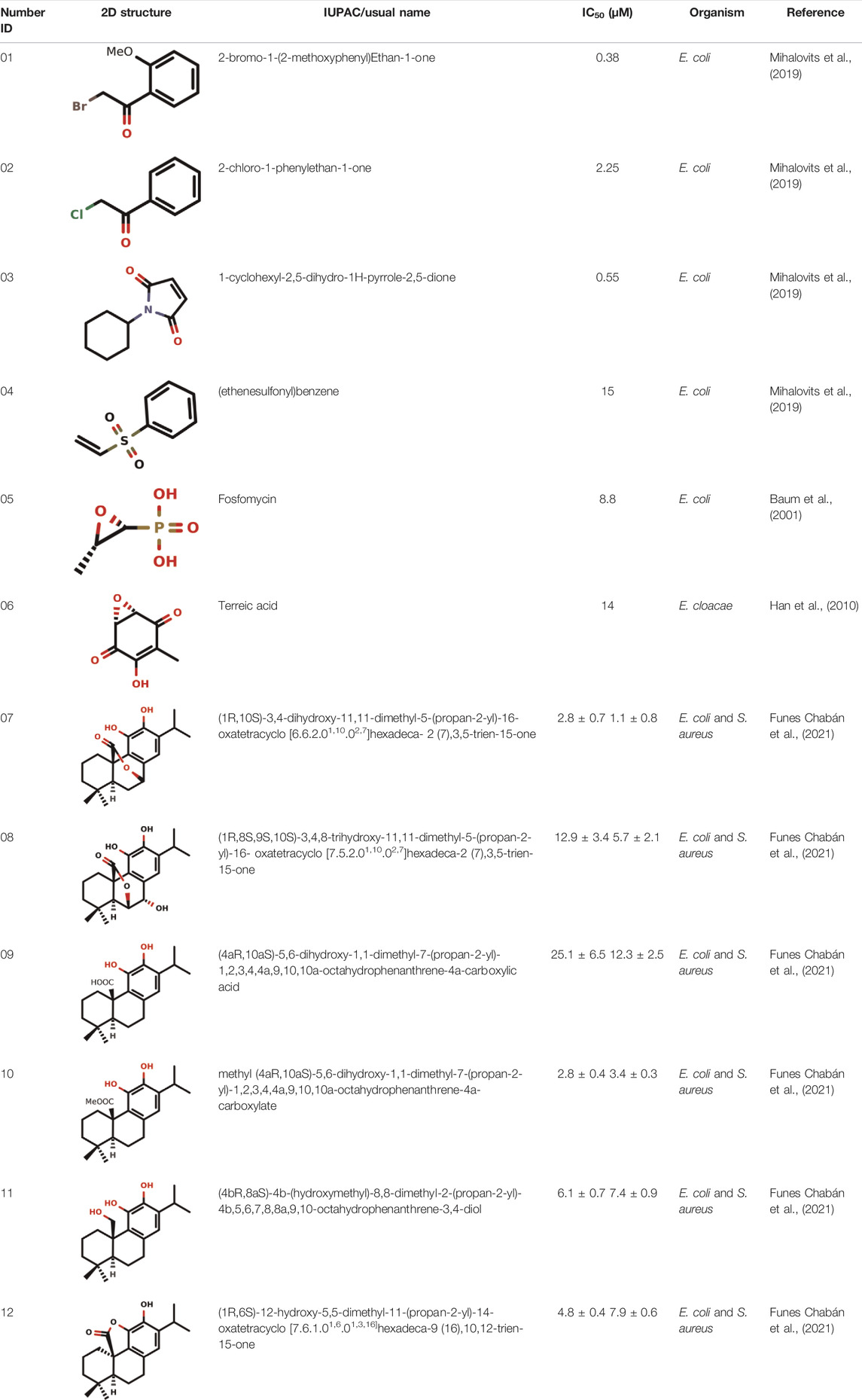
TABLE 1. Some examples of covalent inhibitors against MurA in different organisms, such as Escherichia coli (E. coli), Enterobacter cloacae (E. cloacae) and Staphylococcus aureus (S. aureus).
Fosfomycin as Food and Drug Administration-Approved Covalent Inhibitor of MurA Enzyme
Fosfomycin, also named monurol or fosfomycin tromethamine, is still the only US Food and Drug Administration-approved (FDA-approved) covalent inhibitor of the MurA enzyme. Since its discovery in 1969, fosfomycin has still been used as a broad-spectrum antibiotic for both Gram-positive and Gram-negative bacteria, and it is currently being used as an alternative agent for the treatment of resistant organisms, such as multidrug-resistant (MDR) bacteria (Falagas et al., 2016; Liu et al., 2020). The structure of fosfomycin contains two key groups: an epoxide and a phosphonic group (Falagas et al., 2019). This antibiotic inhibits the enzymatic reaction catalyzed by MurA involved in the first cytoplasmic step of bacterial wall biosynthesis. The entry of fosfomycin can occur through permeases via two pathways, namely, glucose-6-phosphate (G6P) transporter (UhpT) and glycerol-3-phosphate transporter (GlpT) (Castañ;eda-García et al., 2009; Saiprasad and Krishnaprasad, 2016; Díez-Aguilar and Cantón, 2019; Liu et al., 2020). Fosfomycin inhibits MurA by covalent binding to the thiol group of the key cysteine residue (Cys115 in MurAE.coli) (Zhanel et al., 2018). Consequently, it blocks the formation of UDP-N-acetylMurAmic acid and interrupts the peptidoglycan biosynthetic pathway.
According to the US FDA, the use of fosfomycin (IUPAC name: [(2R,3S)-3-methyloxiran-2-yl]phosphonate) is allowed only for the treatment of patients with uncomplicated cystitis caused by E. coli and Enterococcus faecalis (Baylan, 2010; Silver, 2017). In 1996, this antibiotic was approved for use in acute cystitis treatment in American women (EUA) by single-dose oral therapy of uncomplicated UTIs (Silver, 2017).
Fosfomycin is the unique clinically available inhibitor of MurA acting competitively against phosphoenolpyruvate. Its mode of action is related to the covalent binding to the thiol group of Cys115 residue in the active site of MurA (Kahan et al., 1974; Falagas et al., 2016; Sutanto et al., 2020; Scarpino et al., 2021). Naturally produced by Streptomyces spp. (Falagas et al., 2016), fosfomycin is available in drug formulations for oral and intravenous administration. When combined with other drugs, such as amikacin or ceftazidime, it has already been shown to be effective in cases of urinary tract infections (UTIs) with vesicoureteral reflux (VUR) in children (Wu et al., 2016). Treatment with fosfomycin was also effective against patients with UTIs caused by MDR bacterial strains, thus being the first-choice drug for the treatment (Babiker et al., 2019; Tsegka et al., 2022).
Even though terreic acid in in vitro tests was shown to be an inhibitor of the MurA enzyme by covalently inactivating it through Cys115 residue, Olsen and co-workers showed in vivo that the MurA enzyme is not a molecular target for terreic acid (Olesen et al., 2014). More studies will be necessary for the development of a new antibiotic against the MurA enzyme using the competitive mode of action similar to the fosfomycin.
Covalent Inhibition of MurA and its Different Classes of Inhibitors
Hard and Soft Electrophiles
Electrophiles are electron-deficient chemicals that can react with other compounds which have unshared valence electron pairs called nucleophiles (LoPachin et al., 2019). According to Perry’s 1990 Hard and Soft Acids and Bases (HSAB) theory, these electrophilic and nucleophilic species are named according to their polarizability, classified as “soft” or “hard”, or according to the ease of electron density shift to form a covalent bond (LoPachin et al., 2019).
Nowadays, bromo-cyclobutenaminone derivatives are studied as new covalent inhibitors and electrophilic warheads. These inhibitors have an electrophilic character acting as new warheads for the covalent bonding against the Cys115 residue located in the active site of MurAE.coli (Hamilton et al., 2020).
The development of a heterocyclic electrophilic fragment library revealed their potential as covalent warheads. This library can be used to identify heterocyclic fragments with significant inhibitory potency against the MurA enzyme (Keeley et al., 2018).
Michael-Type Nucleophilic Addition
Known as 1,4- or conjugate-addition, or Friedel–Crafts Alkylation is one of the most important nucleophilic additions in the formation of carbon-carbon/carbon-heteroatom bonds in organic synthesis (Saracoglu, 2007). Petri et al. (2021) used the Michael-type nucleophilic addition in 13 covalent fragments that represent various warhead chemotypes. The main compounds based on the IC50 value are presented in Table 2. The warhead model 07 (IC50 = 13 ± 2.7 µM) forms a covalent bond with Cys115 in the active site of MurA (Petri et al., 2021).
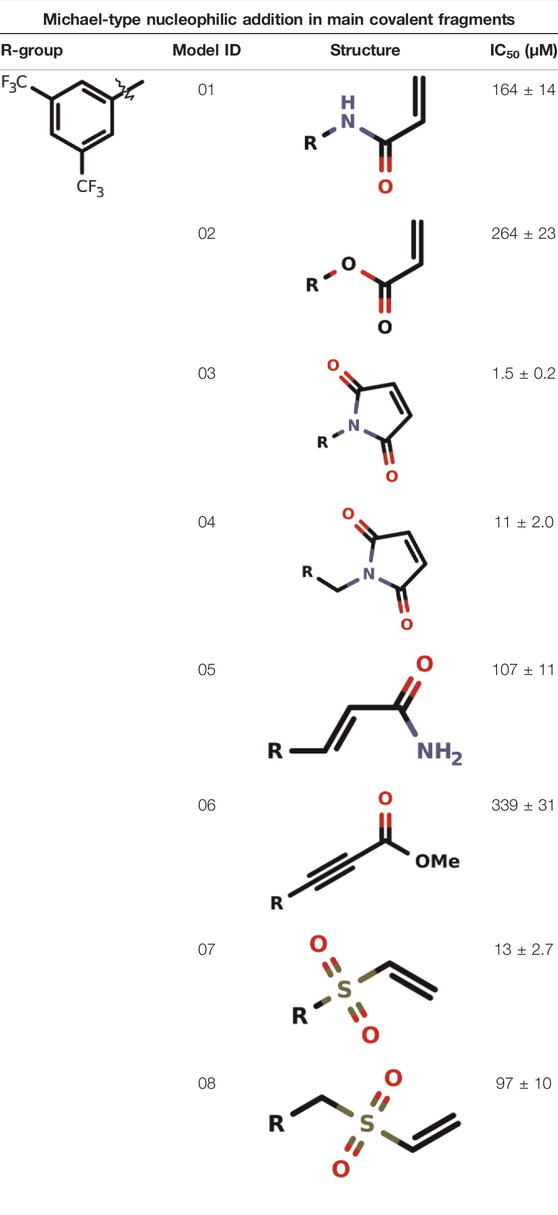
TABLE 2. Michael-type nucleophilic addition in main covalent fragments by Petri et al., 2021.
Recently, Mihalovits et al. (2019) showed that some compounds based on the reaction mechanism for the Michael addition and nucleophilic conjugation against MurA from E. coli as active inhibitors (01–04 in Table 1) react by binding with Cys115 and interacting with histidine residue (His394) in the MurA binding site (Figure 3). The compound (ethenesulfonyl)benzene is an irreversible inhibitor (IC50 = 15 µM), while 1-cyclohexyl-2,5-dihydro-1H-pyrrole-2,5-dione is a reversible inhibitor (IC50 = 0.55 μM), and N,N-dimethylprop-2-enamide was shown to be inactive against the E. coli MurA enzyme (Mihalovits et al., 2019).
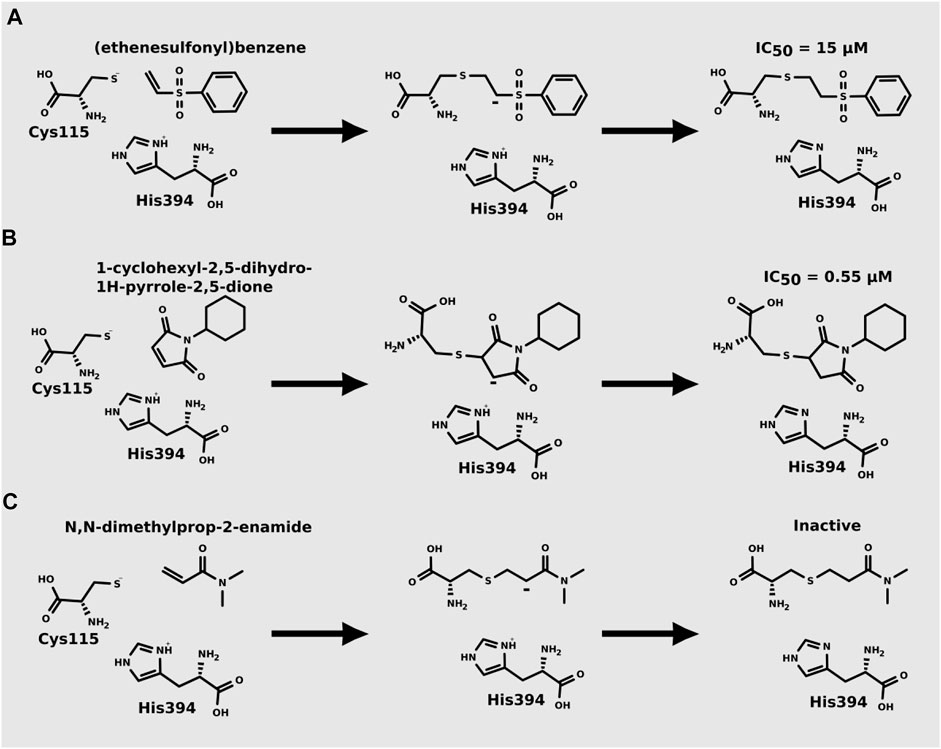
FIGURE 3. Mechanism for the Michael addition of (A) (ethenesulfonyl)benzene (B) N,N-dimethylprop-2-enamide, and (C) 1-cyclohexyl-2,5-dihydro-1H-pyrrole-2,5-dione against MurA (E. coli).
Natural Products as Covalent Inhibitors of MurA
Natural products obtained from different sources have been widely investigated for the development of new drugs (Do Nascimento et al., 2020). It is believed that 60% of drugs available commercially are derived directly or inspired from natural products (De Cesco et al., 2017; Santana et al., 2021).
Diterpenes, secondary metabolites obtained from plants, and their analogs were explored as inhibitors of the MurA enzyme from E. coli and Staphylococcus aureus (Funes Chabán et al., 2021). This research showed that six compounds acted as potential inhibitors of MurA in both microorganisms with IC50 values between 1.1 and 25.1 µM (07–12 in Table 1). The results revealed that main interactions are made with amino acid residues Arg91, Arg120, and Phe328 (Funes Chabán et al., 2021).
Natural products with antibacterial, antifungal and antiviral activities are often produced by microorganisms as a form of defense (De Cesco et al., 2017). Since 1969, after the discovery of fosfomycin, an increased parcel of natural products that can act as covalent inhibitors against MurA has been studied for the development of new antibiotics. Fosfomycin is a well-known natural covalent inhibitor of the MurA enzyme produced by Streptomyces sp. (Kahan et al., 1974). Terreic acid is a covalent inhibitor of MurA in vitro, and it is produced by Aspergillus terreus. Both compounds inactivate MurA through a similar molecular mechanism of action.
The flavonoid compound catechin from Uncaria gambir Roxb. is a potent natural inhibitor of MurA which prevents the growth of E. faecalis and S. sanguinis (Riyana et al., 2020). Six-tuliposide B is a natural product that shows antibiotic activity against bacterial MurA acting via cnicin mechanism (binding to Cys115 of MurA), and sesquiterpene lactone cnicin also has antibiotic activity (Bachelier et al., 2006; Shigetomi et al., 2010).
Cynaropicrin is a natural product that covalently binds to the thiol group of the Cys115 residue in the MurA active site through Michael addition reaction (Elsebai et al., 2016). This compound is a potent irreversible inhibitor of the MurA enzyme with antibacterial, anti-inflammatory and anti-hepatitis C virus activity (Bachelier et al., 2006; Elsebai et al., 2016).
The structure of several natural products has electrophilic moieties, and they react covalently with nucleophilic functional groups when inserted into their targets (Johansson, 2012). When a compound covalently binds to its molecular target it can be reversible or irreversible. This covalent bond can be generated through several forms of different chemical reactions, for example, disulfide bond, Pinner reaction, or Michael addition (1,4-conjugate addition) (Johansson, 2012).
Computational Studies of MurA Covalent Inhibitors
Although about twenty articles previously published in the literature describe in silico studies of MurA inhibitors, only three of them deal specifically with covalent inhibitors (Dunsmore et al., 2008; Miller et al., 2010), and (Mihalovits et al., 2019).
Dunsmore et al. (2008) identified 2-aminotetralones as a new class of MurA inhibitors that act through the formation of a covalent adduct. Docking of 2-(4-methylpiperazin-1-yl)-1,2,3,4-tetrahydronaphthalen-1-one molecule into E. coli MurA showed that keto-group binds close to Cys115 residue and H-bonds with conserved Arg120 residue, which could facilitate thiohemiketal formation and covalent bonding (Dunsmore et al., 2008).
Miller et al. (2010) performed high-throughput screening of a 650 k chemical library against S. aureus MurA and identified benzothioxalone derivatives with IC50 0.25–0.51 µM. Docking studies with MurA–UDP-GlcNAc and 5-hydroxy-2H-1,3-benzoxathiol-2-one suggested that thioxalone resides close to the thiol group of the Cys115 residue, which is necessary for covalent adduct formation. Moreover, docking into MurA containing covalently bound fosfomycin fragment showed altered binding mode for benzothioxalone derivatives, in which carbonyl group of thioxalone goes to the secondary pocket, which might explain why some compounds (e.g., compound 18 from this study) can still bind to MurA pre-treated with fosfomycin (Miller et al., 2010).
Mihalovits et al. (2019) performed QM/MM-based molecular dynamics and docking study using six active and three inactive covalent inhibitors of MurA (Mihalovits et al., 2019). These inhibitors can be divided into three groups: oxiranes [fosfomycin, terreic acid, (S)-3 (R)-3), haloketones (2-bromo-1-(2-methoxyphenyl) etha-1-one, 2-chloro-1-phenylethan-1-one and 2-chloro-N-phenylacetamide), and Michael-acceptors (Figure 3)]. Simulations suggested that the loop closure initiated by UNAG binding brings Cys115 and His394 in a proximity that allows the deprotonation of Cys115 and the formation of the reactive thiolate, which can be involved in various reactions including PEP binding or covalent inhibitor binding. For oxiranes, the key flexible loop can be in a closed (fosfomycin), half-open (fosfomycin) or open (terroic acid) conformation. The reaction was predicted to proceed via a two-step mechanism comprising nucleophilic substitution and subsequent protonation. Haloketones also inhibit MurA via nucleophilic substitution reactions: the formation of the Cys adduct between 2-bromo-1-(2-methoxyphenyl)etha-1-one, 2-chloro-1-phenylethan-1-one and 2-chloro-N-phenylacetamide and the thiolate form of Cys115 proceeds in a single step. For Michael acceptors, the reaction has two steps: first, carbanion intermediate is formed, and then H+ transfer between protonated His394 and the negatively charged carbon takes place. Free energy calculations showed that inhibitory activity was more dependent on the energy barrier height of the chemical reaction of covalent binding than on a non-covalent complex formation prior to the chemical reaction.
Final Considerations
Since its discovery in 1969, fosfomycin remains the only FDA-approved covalent inhibitor used to inhibit the MurA enzyme, being currently available in drug formulations for oral and intravenous administration and being used for the treatment of resistant organisms, such as MDR bacteria. Studies show that fosfomycin combined with other drugs increases the chances of patient cure. Fosfomycin acts through competitive inhibition against PEP, binding the enzyme through the thiol group of Cys115 residue. As the only clinically effective inhibitor available against MurA is a covalent inhibitor, there is an urgent need for more studies to identify molecules that can be used as covalent inhibitors whereas the inhibition of enzyme is a key target for the disruption of the peptidoglycan pathway. Computational methods have been increasingly used in simulations of biological systems, thus, in order to contribute to the development of new drugs, our study demonstrates that new efforts should be made to employ these techniques for the development of MurA covalent inhibitors. Moreover, the present minireview highlightes the recent advances in the development of covalent inhibitors against MurA and the main structural properties associated with its covalent inhibition.
Author Contributions
All authors listed have made a substantial, direct, and intellectual contribution to the work and approved it for publication.
Funding
SV is grateful to the bioinformatics (J.V. Lehtonen), translational activities, and structural biology (FINStruct) infrastructure support from Biocenter Finland and CSC IT Center for Science for computational infrastructure support at the Structural Bioinformatics Laboratory (SBL), Å̊bo Akademi University. AL and KC are grateful to Conselho Nacional de Desenvolvimento Científico e Tecnológico (CNPq, Brazil) and PROPESP/UFPA for the financial support. MDO is grateful to Brazilian funding agency, Coordenação de Aperfeiçoamento de Pessoal de Nível Superior (CAPES, Brazil) for the scholarship.
Conflict of Interest
The authors declare that the research was conducted in the absence of any commercial or financial relationships that could be construed as a potential conflict of interest.
Publisher’s Note
All claims expressed in this article are solely those of the authors and do not necessarily represent those of their affiliated organizations, or those of the publisher, the editors and the reviewers. Any product that may be evaluated in this article, or claim that may be made by its manufacturer, is not guaranteed or endorsed by the publisher.
References
Ábrányi-Balogh, P., Petri, L., Imre, T., Szijj, P., Scarpino, A., Hrast, M., et al. (2018). A Road Map for Prioritizing Warheads for Cysteine Targeting Covalent Inhibitors. Eur. J. Med. Chem. 160, 94–107. doi:10.1016/j.ejmech.2018.10.010
Aghamali, M., Sedighi, M., Zahedi bialvaei, A., Mohammadzadeh, N., Abbasian, S., Ghafouri, Z., et al. (2019). Fosfomycin: Mechanisms and the Increasing Prevalence of Resistance. J. Med. Microbiol. 68, 11–25. doi:10.1099/jmm.0.000874
Araújo, J. D. O., Dos Santos, A. M., Lameira, J., Alves, C. N., and Lima, A. H. (2019). Computational Investigation of Bisphosphate Inhibitors of 3-Deoxy-D-Manno-Octulosonate 8-phosphate Synthase. Molecules 24, 2370. doi:10.3390/molecules24132370
Babiker, A., Clarke, L., Doi, Y., and Shields, R. K. (2019). Fosfomycin for Treatment of Multidrug-Resistant Pathogens Causing Urinary Tract Infection: A Real-World Perspective and Review of the Literature. Diagnostic Microbiol. Infect. Dis. 95, 114856. doi:10.1016/j.diagmicrobio.2019.06.008
Bachelier, A., Mayer, R., and Klein, C. D. (2006). Sesquiterpene Lactones are Potent and Irreversible Inhibitors of the Antibacterial Target Enzyme MurA. Bioorg. Med. Chem. Lett. 16, 5605–5609. doi:10.1016/j.bmcl.2006.08.021
Baum, E. Z., Montenegro, D. A., Licata, L., Turchi, I., Webb, G. C., Foleno, B. D., et al. (2001). Identification and Characterization of New Inhibitors of the Escherichia Coli MurA Enzyme. Antimicrob. Agents Chemother. 45, 3182–3188. doi:10.1128/AAC.45.11.3182-3188.2001
Baylan, O. (2010). Fosfomycin: past, present and future. Mikrobiyol. Bul. 44, 311–321. Available at: http://www.ncbi.nlm.nih.gov/pubmed/20549968.
Burschowsky, D., Thorbjørnsrud, H. V., Heim, J. B., Fahrig-Kamarauskaitė, J., Würth-Roderer, K., Kast, P., et al. (2018). Inter-Enzyme Allosteric Regulation of Chorismate Mutase in Corynebacterium Glutamicum: Structural Basis of Feedback Activation by Trp. Biochemistry 57, 557–573. doi:10.1021/acs.biochem.7b01018
Castañeda-Garcìa, A., Rodriìguez-Rojas, A., Guelfo, J. R., and Blàzquez, J. (2009). The Glycerol-3-Phosphate Permease GlpT is the Only Fosfomycin Transporter in Pseudomonas Aeruginosa. J. Bacteriol. 191, 6968–6974. doi:10.1128/JB.00748-09
De Cesco, S., Kurian, J., Dufresne, C., Mittermaier, A. K., and Moitessier, N. (2017). Covalent Inhibitors Design and Discovery. Eur. J. Med. Chem. 138, 96–114. doi:10.1016/j.ejmech.2017.06.019
De Oliveira, M. D., Araújo, J. D. O., Galúcio, J. M. P., Santana, K., and Lima, A. H. (2020). Targeting Shikimate Pathway: In Silico Analysis of Phosphoenolpyruvate Derivatives as Inhibitors of EPSP Synthase and DAHP Synthase. J. Mol. Graph. Model. 101, 107735. doi:10.1016/j.jmgm.2020.107735
Díez-Aguilar, M., and Cantón, R. (2019). New Microbiological Aspects of Fosfomycin. Rev. Esp. Quimioter. 32 (Suppl. 1), 8–18. Available at: http://www.ncbi.nlm.nih.gov/pubmed/31131587.
Diniz do Nascimento, L., Moraes, A. A. B., Costa, K. S., Pereira Galúcio, J. M., Taube, P. S., Costa, C. M. L., et al. (2020). Bioactive Natural Compounds and Antioxidant Activity of Essential Oils from Spice Plants: New Findings and Potential Applications. Biomolecules 10 (7), 988–1037. doi:10.3390/biom10070988
Dunsmore, C. J., Miller, K., Blake, K. L., Patching, S. G., Henderson, P. J. F., Garnett, J. A., et al. (2008). 2-Aminotetralones: Novel Inhibitors of MurA and MurZ. Bioorg. Med. Chem. Lett. 18, 1730–1734. doi:10.1016/j.bmcl.2008.01.089
Egan, A. J. F., Errington, J., and Vollmer, W. (2020). Regulation of Peptidoglycan Synthesis and Remodelling. Nat. Rev. Microbiol. 18, 446–460. doi:10.1038/s41579-020-0366-3
Elsebai, M. F., Mocan, A., and Atanasov, A. G. (2016). Cynaropicrin: A Comprehensive Research Review and Therapeutic Potential as an Anti-Hepatitis C Virus Agent. Front. Pharmacol. 7, 472. doi:10.3389/fphar.2016.00472
Evangelina, I. A., Herdiyati, Y., Laviana, A., Rikmasari, R., Zubaedah, C., Anisah, , et al. (2021). Bio-Mechanism Inhibitory Prediction of β-Sitosterol from Kemangi (Ocimum Basilicum L.) as an Inhibitor of MurA Enzyme of Oral Bacteria: In Vitro and In Silico Study. Adv. Appl. Bioinform. Chem. 14, 103–115. doi:10.2147/AABC.S301488
Falagas, M. E., Athanasaki, F., Voulgaris, G. L., Triarides, N. A., and Vardakas, K. Z. (2019). Resistance to Fosfomycin: Mechanisms, Frequency and Clinical Consequences. Int. J. Antimicrob. Agents 53, 22–28. doi:10.1016/j.ijantimicag.2018.09.013
Falagas, M. E., Vouloumanou, E. K., Samonis, G., and Vardakas, K. Z. (2016). Fosfomycin. Clin. Microbiol. Rev. 29, 321–347. doi:10.1128/CMR.00068-15
Funes Chabán, M., Hrast, M., Frlan, R., Graikioti, D. G., Athanassopoulos, C. M., and Carpinella, M. C. (2021). Inhibition of MurA Enzyme from Escherichia Coli and Staphylococcus Aureus by Diterpenes from Lepechinia Meyenii and Their Synthetic Analogs. Antibiotics 10, 1535. doi:10.3390/antibiotics10121535
Gupta, R. S. (2011). Origin of Diderm (Gram-Negative) Bacteria: Antibiotic Selection Pressure Rather Than Endosymbiosis Likely Led to the Evolution of Bacterial Cells with Two Membranes. Ant. Van Leeuwenhoek 100, 171–182. doi:10.1007/s10482-011-9616-8
Hamilton, D. J., Ábrányi-Balogh, P., Keeley, A., Petri, L., Hrast, M., Imre, T., et al. (2020). Bromo-Cyclobutenaminones as New Covalent UDP-N-Acetylglucosamine Enolpyruvyl Transferase (MurA) Inhibitors. Pharmaceuticals 13, 362. doi:10.3390/ph13110362
Han, H., Yang, Y., Olesen, S. H., Becker, A., Betzi, S., and Schönbrunn, E. (2010). The Fungal Product Terreic Acid is a Covalent Inhibitor of the Bacterial Cell Wall Biosynthetic Enzyme UDP-N-Acetylglucosamine 1-Carboxyvinyltransferase (MurA). Biochemistry 49, 4276–4282. doi:10.1021/bi100365b
Hsu, Y.-P., Booher, G., Egan, A., Vollmer, W., and VanNieuwenhze, M. S. (2019). D-Amino Acid Derivatives as in Situ Probes for Visualizing Bacterial Peptidoglycan Biosynthesis. Acc. Chem. Res. 52, 2713–2722. doi:10.1021/acs.accounts.9b00311
Johansson, M. H. (2012). Reversible Michael Additions: Covalent Inhibitors and Prodrugs. Mini. Rev. Med. Chem. 12, 1330–1344. doi:10.2174/13895575112091330
Kahan, F. M., Kahan, J. S., Cassidy, P. J., and Kropp, H. (1974). The Mechanism of Action of Fosfomycin (Phosphonomycin). Ann. N. Y. Acad. Sci. 235, 364–386. doi:10.1111/j.1749-6632.1974.tb43277.x
Keeley, A., Ábrányi-Balogh, P., Hrast, M., Imre, T., Ilaš, J., Gobec, S., et al. (2018). Heterocyclic Electrophiles as New MurA Inhibitors. Arch. Pharm. Chem. Life Sci. 351, 1800184. doi:10.1002/ardp.201800184
Kumar, P., Saumya, K. U., and Giri, R. (2020). Identification of Peptidomimetic Compounds as Potential Inhibitors against MurA Enzyme of Mycobacterium Tuberculosis. J. Biomol. Struct. Dyn. 38, 4997–5013. doi:10.1080/07391102.2019.1696231
Laddomada, F., Miyachiro, M., and Dessen, A. (2016). Structural Insights into Protein-Protein Interactions Involved in Bacterial Cell Wall Biogenesis. Antibiotics 5, 14. doi:10.3390/antibiotics5020014
Liu, P., Chen, S., Wu, Z.-Y., Qi, M., Li, X.-Y., and Liu, C.-X. (2020). Mechanisms of Fosfomycin Resistance in Clinical Isolates of Carbapenem-Resistant Klebsiella Pneumoniae. J. Glob. Antimicrob. Resist. 22, 238–243. doi:10.1016/j.jgar.2019.12.019
LoPachin, R. M., Geohagen, B. C., and Nordstroem, L. U. (2019). Mechanisms of Soft and Hard Electrophile Toxicities. Toxicology 418, 62–69. doi:10.1016/j.tox.2019.02.005
Martin, J. S., MacKenzie, C. J., Fletcher, D., and Gilbert, I. H. (2019). Characterising Covalent Warhead Reactivity. Bioorg. Med. Chem. 27, 2066–2074. doi:10.1016/j.bmc.2019.04.002
Megrian, D., Taib, N., Witwinowski, J., Beloin, C., and Gribaldo, S. (2020). One or Two Membranes? Diderm Firmicutes Challenge the Gram‐Positive/Gram‐Negative Divide. Mol. Microbiol. 113, 659–671. doi:10.1111/mmi.14469
Mihalovits, L. M., Ferenczy, G. G., and Keserű, G. M. (2019). Catalytic Mechanism and Covalent Inhibition of UDP-N-Acetylglucosamine Enolpyruvyl Transferase (MurA): Implications to the Design of Novel Antibacterials. J. Chem. Inf. Model. 59, 5161–5173. doi:10.1021/acs.jcim.9b00691
Mihalovits, L. M., Ferenczy, G. G., and Keserű, G. M. (2021). The Role of Quantum Chemistry in Covalent Inhibitor Design. Int J Quantum Chem. 122, 26768. doi:10.1002/qua.26768
Miller, K., Dunsmore, C. J., Leeds, J. A., Patching, S. G., Sachdeva, M., Blake, K. L., et al. (2010). Benzothioxalone Derivatives as Novel Inhibitors of UDP-N-Acetylglucosamine Enolpyruvyl Transferases (MurA and MurZ). J. Antimicrob. Chemother. 65, 2566–2573. doi:10.1093/jac/dkq349
Olesen, S. H., Ingles, D. J., Yang, Y., and Schönbrunn, E. (2014). Differential Antibacterial Properties of the MurA Inhibitors Terreic Acid and Fosfomycin. J. Basic Microbiol. 54, 322–326. doi:10.1002/jobm.201200617
Pazos, M., and Peters, K. (2019). Peptidoglycan. Pept. Subcell. Biochem. 92, 127–168. doi:10.1007/978-3-030-18768-2_5
Petri, L., Àbrányi‐Balogh, P., Tímea, I., Pálfy, G., Perczel, A., Knez, D., et al. (2021). Assessment of Tractable Cysteines for Covalent Targeting by Screening Covalent Fragments. ChemBioChem 22, 743–753. doi:10.1002/cbic.202000700
Pham, H. T., Shi, W., Xiang, Y., Foo, S. Y., Plan, M. R., Courtin, P., et al. (2021). Cyclic Di-AMP Oversight of Counter-Ion Osmolyte Pools Impacts Intrinsic Cefuroxime Resistance in Lactococcus Lactis. MBio 12, e00324–21. doi:10.1128/mBio.00324-21
Popović, V., Morrison, E., Rosanally, A. Z., Balachandran, N., Senson, A. W., Szabla, R., et al. (2019). NeuNAc Oxime: A Slow-Binding and Effectively Irreversible Inhibitor of the Sialic Acid Synthase NeuB. Biochemistry 58, 4236–4245. doi:10.1021/acs.biochem.9b00654
Ray, S., and Murkin, A. S. (2019). New Electrophiles and Strategies for Mechanism-Based and Targeted Covalent Inhibitor Design. Biochemistry 58, 5234–5244. doi:10.1021/acs.biochem.9b00293
Riyana, B., Huspa, D. H. P., Satari, M. H., and Kurnia, D. (2020). The Potency of Catechin from Gambir (Uncaria Gambir Roxb.) as a Natural Inhibitor of MurA (1UAE) Enzyme: In Vitro and In Silico Studies. Lddd 17, 1531–1537. doi:10.2174/1570180817999200714104737
Saiprasad, P., and Krishnaprasad, K. (2016). Exploring the Hidden Potential of Fosfomycin for the Fight against Severe Gram-Negative Infections. Indian J. Med. Microbiol. 34, 416–420. doi:10.4103/0255-0857.195379
Santana, K., do Nascimento, L. D., Lima e Lima, A., Damasceno, V., Nahum, C., Braga, R. C., et al. (2021). Applications of Virtual Screening in Bioprospecting: Facts, Shifts, and Perspectives to Explore the Chemo-Structural Diversity of Natural Products. Front. Chem. 9, 662688. doi:10.3389/fchem.2021.662688
Saracoglu, N. (2007). “Functionalization of Indole and Pyrrole Cores via Michael-Type Additions,” in Bioactive Heterocycles V (Berlin, Heidelberg: Springer Berlin Heidelberg), 11, 1–61.
Scarpino, A., Petri, L., Knez, D., Imre, T., Ábrányi-Balogh, P., Ferenczy, G. G., et al. (2021). WIDOCK: A Reactive Docking Protocol for Virtual Screening of Covalent Inhibitors. J. Comput. Aided. Mol. Des. 35, 223–244. doi:10.1007/s10822-020-00371-5
Shigetomi, K., Shoji, K., Mitsuhashi, S., and Ubukata, M. (2010). The Antibacterial Properties of 6-Tuliposide B. Synthesis of 6-Tuliposide B Analogues and Structure-Activity Relationship. Phytochemistry 71, 312–324. doi:10.1016/j.phytochem.2009.10.008
Silver, L. L. (2017). Fosfomycin: Mechanism and Resistance. Cold Spring Harb. Perspect. Med. 7, a025262. doi:10.1101/cshperspect.a025262
Simon, M. A., Ongpipattanakul, C., Nair, S. K., and van der Donk, W. A. (2021). Biosynthesis of Fosfomycin in Pseudomonads Reveals an Unexpected Enzymatic Activity in the Metallohydrolase Superfamily. Proc. Natl. Acad. Sci. U. S. A. 118, e2019863118. doi:10.1073/pnas.2019863118
Sutanto, F., Konstantinidou, M., and Dömling, A. (2020). Covalent Inhibitors: A Rational Approach to Drug Discovery. RSC Med. Chem. 11, 876–884. doi:10.1039/D0MD00154F
Tsegka, K. G., Voulgaris, G. L., Kyriakidou, M., Kapaskelis, A., and Falagas, M. E. (2022). Intravenous Fosfomycin for the Treatment of Patients with Bone and Joint Infections: A Review. Expert Rev. Anti-Infective Ther. 20, 33–43. doi:10.1080/14787210.2021.1932463
Vainer, R., Belakhov, V., Rabkin, E., Baasov, T., and Adir, N. (2005). Crystal Structures of Escherichia Coli KDO8P Synthase Complexes Reveal the Source of Catalytic Irreversibility. J. Mol. Biol. 351, 641–652. doi:10.1016/j.jmb.2005.06.021
Wu, T.-H., Huang, F.-L., Fu, L.-S., Chou, C.-M., Chien, Y.-L., Huang, C.-M., et al. (2016). Treatment of Recurrent Complicated Urinary Tract Infections in Children with Vesicoureteral Reflux. J. Microbiol. Immunol. Infect. 49, 717–722. doi:10.1016/j.jmii.2014.08.024
Keywords: covalent inhibitors, bacterial resistance, fosfomycin, peptidoglycan, MurA enzyme
Citation: de Oliveira MVD, Furtado RM, da Costa KS, Vakal S and Lima AH (2022) Advances in UDP-N-Acetylglucosamine Enolpyruvyl Transferase (MurA) Covalent Inhibition. Front. Mol. Biosci. 9:889825. doi: 10.3389/fmolb.2022.889825
Received: 04 March 2022; Accepted: 17 June 2022;
Published: 20 July 2022.
Edited by:
Carlos Montanari, University of São Paulo, BrazilReviewed by:
Marina Borisova, University of Tübingen, GermanyCopyright © 2022 de Oliveira, Furtado, da Costa, Vakal and Lima. This is an open-access article distributed under the terms of the Creative Commons Attribution License (CC BY). The use, distribution or reproduction in other forums is permitted, provided the original author(s) and the copyright owner(s) are credited and that the original publication in this journal is cited, in accordance with accepted academic practice. No use, distribution or reproduction is permitted which does not comply with these terms.
*Correspondence: Anderson H. Lima, YW5kZXJzb25AdWZwYS5icg==
†ORCID: Maycon Vinicius Damasceno de Oliveira, orcid.org/0000-0003-2263-2124; Renan Machado Furtado, orcid.org/0000-0003-0709-0304; Kauê S. da Costa, orcid.org/0000-0002-2735-8016; Serhii Vakal, orcid.org/0000-0002-1549-0394; Anderson H. Lima, orcid.org/0000-0002-8451-9912