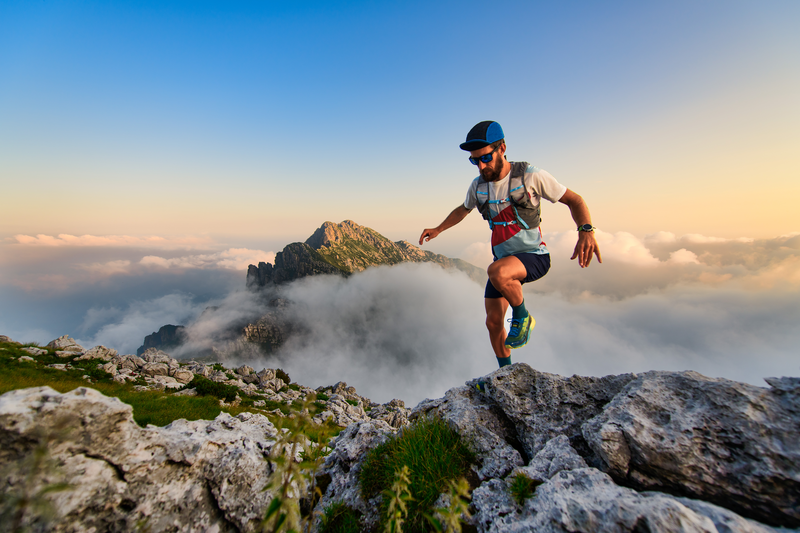
95% of researchers rate our articles as excellent or good
Learn more about the work of our research integrity team to safeguard the quality of each article we publish.
Find out more
ORIGINAL RESEARCH article
Front. Mol. Biosci. , 30 May 2022
Sec. Molecular Recognition
Volume 9 - 2022 | https://doi.org/10.3389/fmolb.2022.831293
This article is part of the Research Topic Interactions Between Proteins and Biomacromolecules: Tools and Applications, Volume II View all 8 articles
Hemocoagulase Agkistrodon halys pallas is a complex mixture composed of snake venom thrombin-like enzymes (svTLEs) and small amounts of thrombokinase-like enzymes. It has been widely used as a hemostatic with rapidly growing marketing due to its advantage of localized clotting fibrinogen other than systemic coagulation. However, svTLEs from different species have various structures, functions, and hemostatic mechanisms. To ensure the efficacy and safety of Hemocoagulase Agkistrodon halys pallas, an exclusive and sensitive method has been developed to identify specific marker peptides based on liquid chromatography-tandem mass spectrometry with multiple reaction monitoring (LC-MS/MS-MRM) mode. By combining transcriptomics and proteomics, a series of species-specific peptides of Agkistrodon halys pallas were predicted and examined by LC-MS/MS. After reduction, alkylation, and tryptic digestion were performed on Hemocoagulase Agkistrodon halys pallas, a target peptide TLCAGVMEGGIDTCNR was analyzed by LC-MS/MS-MRM. It offers a new and effective approach for the quality control of Hemocoagulase Agkistrodon halys pallas products. This method is superior to the current assays in terms of sensitivity, specificity, precision, accuracy, and throughput. The strategy can also be applied in studying other important protein-based medicines.
Snake venom thrombin-like enzymes (svTLEs) are members of snake venom serine proteinases (SVSPs) which have the activity of arginine esterase and acylamidase (Ullah et al., 2018). svTLEs are used as hemostatic drugs, defibrinogenating agents, and antithrombotic and diagnostic agents in clinics because of its ability of clotting fibrinogen (Waheed et al., 2017). In addition, some svTLEs can also activate plasminogen and induce platelet aggregation (Castro et al., 2004; Sajevic et al., 2011). Hemocoagulase atrox for Injection (Reptilase® and Baquting®) isolated from the venom of Bothrops atrox is the earliest and most widely used hemostatic in clinical settings (Funk et al., 1971; Li et al., 2019). Hemocoagulase Agkistrodon for Injection (Suling®) is isolated from the venom of Deinagkistrodon acutus and contains a single thrombin-like component (Li et al., 2018). Hemocoagulase for Injection (Sulejuan®) is isolated from the venom of Vipera russelli siamensis which is composed of batroxobin and active factor X (FXa). The active ingredients of Hemocoagulase Agkistrodon halys pallas for Injection (Bangting®) are thrombin-like enzymes and thrombokinase-like enzymes. The amino acid sequences, glycosylation sites, structures, and hemostatic mechanisms of svTLEs from different species are a little different. Hemocoagulase Agkistrodon halys pallas acts on fibrinogen, which first releases fibrinopeptide B and then releases fibrinopeptide A after a period. The rest act only on the fibrinogen Aα-chain (FPA) (Guan et al., 1984; Zeng et al., 2013). The present qualitative assays of hemocoagulase (including biochemical reaction, chemical chromogenic reaction, liquid chromatography-based peptide mapping, electrophoresis method, and activity measurements) are not accurate enough and the species cannot be identified. Establishing a new strategy to identify specific species is necessary to strengthen quality control and promote the safety of drug use in clinics.
Mass spectrometry plays a key role in the structural characterization of glycans, proteins, glycan–protein interactions, and protein–protein interactions (Britt et al., 2022; Grabarics et al., 2022; Sheng et al., 2021; Zhang and Chi, 2021). Shotgun proteomics based on liquid chromatography−tandem mass spectrometry (LC−MS/MS) and database search are widely used for the qualitative and quantitative analysis of proteins in complex biological samples (Huang and Wang, 2021; Marques et al., 2021). Database search is based on a database of known protein sequences in genomics. Proteogenomics, a combination of proteomics and genomics, is an efficient strategy to detect new proteins and peptides (Lu et al., 2019; Yang et al., 2021). The database derived from transcriptome sequencing was used for the matching of MS/MS data and the proteomic assays were used for the verification of new protein sequences. The development of proteomics and genomics plays a key role in clinical diagnosis and treatment, identifying potential biomarkers, discovery of drug targets, and mechanisms of disease development (Bai et al., 2021; Wang et al., 2021; Yang et al., 2021). In the global healthcare emergency caused by SARS-cov-2 since 2020, genomics, proteomics, and glycoproteomics have played a key role in the development of nucleic acid test kits, variant identification, vaccine development research, developing treatment options, studying infection mechanisms, and biomarkers’ investigation (Alaiya et al., 2021; Wang L. et al., 2021).
Multiple-reaction monitoring (MRM), involving the detection of targeted ion pairs, is a sensitivity and high specificity MS technique and has been extensively used in small molecules and targeted proteomic assays (Shi et al., 2014; Li et al., 2017; Zhang et al., 2020). Identification and validation of unique prototypic peptides by omics technologies and then quantification by liquid chromatography-tandem mass spectrometry-multiple reaction monitoring (LC-MS/MS-MRM) have been widely reported (Zhang et al., 2019; Dong et al., 2021; Suvarna et al., 2021). In an early study, two LC-MS/MS-MRM methods have been built to analyze traditional Chinese medicines, Colla corii asini (CCA) and Compound Xiling Jiedu Capsules (Li et al., 2017; Wang Y. T. et al., 2021). Based on the species-specific peptides, different species of collagen can be identified such as those of a donkey, horse, cattle, and pig. For Compound Xiling Jiedu Capsules, the antelope horn and buffalo horn can also be identified by the LC-MS/MS-MRM method. In this study, a series of specific peptides in Hemocoagulase Agkistrodon halys pallas has also been identified by proteomics. Based on the species-specific peptide, a method for the assay of Hemocoagulase Agkistrodon halys pallas was established using LC-MS/MS-MRM. Compared with previous methods, the new method has several advantages including faster analysis and better specificity, accuracy, and efficiency.
Trypsin (sequencing grade) was purchased from Sigma (United States). Ultrafiltration units with a 3 kDa molecular weight cut-off (MWCO) were purchased from Sartorius (Germany). Guanidine hydrochloride, DL-dithiothreitol (DTT), and iodoacetamide (IA) were purchased from VETEC (United States). Formic acid, acetonitrile [high-performance liquid chromatography (HPLC)-grade] and water (HPLC-grade) were purchased from Thermo Fisher Scientific (United States). All the other chemicals and reagents were of analytical reagent grade or better in the commercial market. Marker peptide (TLCAGVMEGGIDTCNR) standard was synthesized by ChinaPeptides (China). Venom gland and Hemocoagulase Agkistrodon halys pallas stocks were purchased from Avanc Pharma (China). The lyophilized powders of the snake venom of Vipera russelli siamensis, Bothrops atrox, and Deinagkistrodon acutus were purchased from Avanc Pharma (China).
The venom of the snake was drained, then the venom glands of the snake were carefully separated, and the attached connective tissue was carefully dissected. The gland was cut into small pieces and rinsed with precooled PBS solution quickly. After quick-freezing in liquid nitrogen for 1–3 h, the sample was transferred to −80°C dry ice for storage and transportation. The extraction of total RNA was performed by the TRIZOL method according to the manufacturer’s protocol. Second and third-generation sequencing was performed using Illumina NovaSeq6000 and PacBio Sequel II, respectively. The clean data were obtained after filtering and other steps of raw data. Then, the open reading frame (ORF) was analyzed for gene analysis. After the determined transcript, the ORF sequence was translated into the corresponding protein sequence, and used as a transcriptome database for further mass-spectrometry database search.
Hemocoagulase Agkistrodon halys pallas was resolved in denaturing buffer (0.5 M Tris-HCl, 2.75 mM EDTA, 6 M guanidine hydrochloride, pH 8.1). Then the samples were reduced and alkylated. The reaction reagents were removed by ultrafiltration (MWCO 3 kDa). The samples were digested with trypsin at an enzyme-to-protein ratio of 1/50 (w/w) at 37°C overnight, and then the peptides were recovered by ultrafiltration (MWCO 3 kDa). Nano-LC-MS/MS analysis was performed by using a Thermo Easy-nLC and Orbitrap Fusion mass spectrometer equipped with a nano-spray ion source. The Acclaim PepMap®100 C18 trapping column (100 μm × 2 cm) and Acclaim PepMap®100 C18 analytical column (75 μm × 15 cm) were used to separate the peptides. Mobile phase A was 0.1% formic acid in 2% acetonitrile and mobile phase B was 0.1% formic acid in 98% acetonitrile. A step gradient of B 0–7% for 20 min, 7%–22% for 60 min, 22%–35% for 20 min, and 35%–90% for 10 min was used. The flow rate was set at 0.3 μl/min.
The database search was performed by the Proteome Discoverer 2.1 (Thermo Fisher Scientific). The general settings are as follows: the mass analyzer was orbitrap, the activation type was high energy collision dissociation (HCD), the polarity mode was positive, and the databases were the UniProt thrombin-like enzyme (TLE) database and transcriptome protein database. The enzyme was trypsin, precursor mass tolerance was 10 ppm, fragment mass tolerance was 0.02 Da, dynamic modifications (peptide terminus) were oxidation/+15.995 Da (M), dynamic modifications (protein terminus) were acetyl/+42.011 Da (N-terminus) and carbamido methyl/+57.021 Da (C), and the peptide confidence at least was settled as high. High confidence identification of proteins was ensured by setting the target FDR (strict) at 0.01. After the database search, the TLE sequence of Agkistrodon halys pallas was identified from the transcriptome protein database. The TLEs of Deinagkistrodon acutus and Bothrops atrox were downloaded from the UniProt (https://www.uniprot.org/, Q9I8X2 and P04971). The unique amino acid residues of Agkistrodon halys pallas were spotted using the software BioEdit Sequence Alignment Editor. Combined with database searching results, the suitable marker peptide was screened.
The LC-MS/MS MRM analysis was performed using the AB SCIEX QTRAP® 6500 LC-MS/MS System equipped with an electrospray ionization (ESI) source (AB Sciex, United States). An ACQUITY UPLC BEH C18 (1.7 μm, 2.1 mm × 50 mm) column was used with the mobile phase A of 0.1% formic acid in water and mobile phase B of 0.1% formic acid in acetonitrile. The gradient was mobile phase B 20% for 1 min, 20%–90% for 4 min, 90%–90% for 2 min, and 20% for 1 min. The flow rate was set at 0.2 ml/min. The auto-sampler and the column temperature were maintained at 8°C and 40°C, respectively. The mass spectrometer was in the positive MRM mode and the temperature of the ion source was set at 500°C. The nebulizing gas and drying gas were set at 50 and 60 psi, respectively. The collision energy and declustering potential for the marker peptide were optimized using the peptides synthesized correspondently.
To maximize the market peptide released from the sample, the final concentration of the DTT, enzyme-protein ratio, and enzyme digestion time were optimized. The standard protocol was as follows: the 600 μl Hemocoagulase Agkistrodon halys pallas stock (0.3 μg/μl) was diluted to 10 ml by 25 mmol/L ammonium bicarbonate solution. The 200-μl diluent was reduced by 10 μl 0.2 mol/L DTT and incubated for 1 h at 60°C. After that, 20 μl of 0.1 mol/L IA was added and incubated for 30 min at room temperature in the dark. Then, 25 mmol/L ammonium bicarbonate was added to dilute the sample by ten times, and 4 μg trypsin was added and incubated for 30 min at 37°C.
The method for the quantitative analysis of the proposed hemocoagulase was validated in specificity, linearity, limits of detection (LOD), limits of quantification (LOQ), precision, repeatability, recovery, and stability. The sample solution (SS) of Hemocoagulase Agkistrodon halys pallas was prepared by the optimized pretreatment methods. The blank sample (BS) was 25 mmol/L ammonium bicarbonate solution and the control sample (CS) was a synthetic thrombin-like enzymes peptide (STLEP). The other snake venom samples (OSVS) were dissolved to 1 mg/ml using 25 mmol/L ammonium bicarbonate solution. The aforementioned solutions were treated in the same way as described in the Optimization of digestion parameters section. The specificity of the method was evaluated by detecting no peaks at the same retention time in the BS compared with the SS, CS, and OSVS. The CS containing the reference standards of STLEP was prepared and diluted to a series of appropriate concentrations for the LC-MS/MS-MRM analysis. The calibration curves were constructed by plotting the peak area (Y-axis) against the final concentrations of STLEP (X-axis). The LOD and LOQ were determined at a signal-to-noise ratio (S/N) of at least 3 and 10 under the same chromatographic conditions. The solutions of the standards were analyzed for six replicates for the precision test. For the repeatability test, six sample solutions were prepared in parallel for analysis. Stability was assessed by repeatedly determining the SS which was kept in the auto-sampler (8°C) for 0, 2, 4, 8, 10, and 24 h. The accuracy of the method was evaluated by the recovery test. The fixed STLEP content added in the SS was detected and compared with the known content. The spike recovery test was performed using three concentration levels and each set of addition was repeated three times. The mean recovery percentage was calculated by the formula:
MRM, as the most commonly used targeted proteomics assays, is a sensitive and exclusive method to conduct the qualitative and quantitative analysis of known components in a sample with a complex matrix. For Hemocoagulase Agkistrodon halys pallas, the sequence of this thrombin-like enzyme is unattainable. In this study, a combination of transcriptomics and shotgun proteomics assays was built, with the overall workflow shown in Figure 1. The transcriptomics database by transcriptome sequencing and the UniProt database which contained snake thrombin-like enzyme sequences were used as the comprehensive protein database. Then after reduction, alkylation, and digestion of the sample, raw data were obtained by LC-MS/MS analysis. After the database search, the thrombin-like enzyme of Agkistrodon halys pallas was identified by high-quality interpretation of MS/MS data. By sequence alignment of theoretical sequences, the unique amino acid residues of Agkistrodon halys pallas were spotted and a suitable species-specific peptide was screened. Finally, after method development and validation, a highly specific, accurate, and efficient LC-MS/MS-MRM was established.
FIGURE 1. Workflow to discover the marker peptides for analyzing Hemocoagulase Agkistrodon halys pallas.
A suitable marker peptide is critical in developing an LC-MS/MS method for species-specific identification and quantification. After pretreatment of the Hemocoagulase Agkistrodon halys pallas, a shotgun proteomics analysis by nano-LC-MS/MS was performed to find the MS evidence. When the UniProt thrombin-like enzyme database was used, the results of Proteome Discoverer 2.1 showed that the thrombin-like enzyme kangshuanmei (P85109) and snake venom serine protease salmobin (O73800) have high sequence coverage, which were 65% and 53%, respectively. In the absence of the thrombin-like enzyme of Agkistrodon halys pallas in the UniProt database, the transcriptomics database was obtained by mRNA sequencing and the full-length translated mRNA-seq of the venom gland. After raw data were analyzed by Proteome Discoverer 2.1, the TLE sequence of Agkistrodon halys pallas can be identified from the transcriptomics database, with a 99% sequence coverage. The transcript sequences and other available svTLE sequences from different species were aligned and compared using the BioEdit Sequence Alignment Editor software. The partial sequence alignments of svTLEs of Agkistrodon halys pallas, Deinagkistrodon acutus, and Bothrops atrox are shown in Figure 2 as an example. The identical amino acid residues among different species are represented in the same color, and discrepant amino acid residues are represented in different colors for easy identification. Thirty-eight amino acid residues located within this sequence were not identical compared with the other two species. The corresponding peptides containing these amino acid residues were analyzed to find the marker peptides. Four potential species-specific marker peptides of Agkistrodon halys pallas were found: 94LDSPVSNSAHIAPLSLPSSAPSVGSVCR121, 122VMGWGQTTSPQETLPDVPQCANINLFNYSECR153, 165TLCAGVMEGGIDTCNR180, and 181DSGGPLICNGQFQGIVFWGQNLCAQPR207. The blast search revealed that these four peptides were unique in the UniProt database. The presence of these four theoretical marker peptides in Hemocoagulase Agkistrodon halys pallas was also verified by LC-MS/MS as shown in Figure 3. The marker peptides for hemocoagulase were selected based on three criteria. First, the peptide can be detected consistently in both hemocoagulase substances and drug products. Second, the peptide should have a suitable length to make it feasible for the MRM method. Third, the signal intensity of the peptide should match the spectrum of the peptide. Considering the long peptides were difficult and costly to be synthesized, the peptide 165TLCAGVMEGGIDTCNR180 was selected as the marker peptide for quantification.
FIGURE 2. Partial sequence alignments of snake venom thrombin-like enzymes in Deinagkistrodon acutus, Bothrops atrox, and Agkistrodon halys pallas. The unique amino acid residues for Agkistrodon halys pallas are indicated in red boxes. The theoretical tryptic marker peptide which contains the unique amino acid residues studied in this work is in black boxes.
FIGURE 3. MS/MS spectrums of the marker peptides for Agkistrodon halys pallas. (A) Peptide TLCAGVMEGGIDTCNR, m/z = 877.39, double charged. (B) Peptide LDSPVSNSAHIAPLSLPSSAPSVGSVCR, m/z = 935.81, triple charged.
The marker peptides’ m/z was extracted in the raw data by Xcalibur software. The marker peptide (TLCAGVMEGGIDTCNR) standard was synthesized to optimize the method. To develop a rapid method to identify the marker peptide, an LC-MS/MS-MRM method was used to detect the parent and product ions. This method is rapid and high-sensitive, with better results for low-concentration components. From the MS/MS spectrum of TLCAGVMEGGIDTCNR, 877.6 (2+) > 550.3 was chosen as the qualitative ion pair, and 877.6 (2+) > 892.5 as the quantitative ion pair. The optimized gradient and voltage are shown in Table 1. To optimize the digestion conditions, the following parameters were optimized: the final concentration of DTT (5, 10, 20, 50, and 100 mM), enzyme-protein ratio (2:1, 1:1, 1:5, 1:20 and 1:50), and digestion time (0.25, 0.5, 1, 2, 4 and 24 h). As shown in Figure 4, 877.6 (2+) > 892.5 exhibits a higher mass response than 877.6 (2+) > 550.3. When the final concentration of DTT is more than 20 mM, the signal response is significantly decreased. Hence, 10 mM was the optimal final concentration of DTT. A high enzyme-protein ratio (2:1, 1:1) was better in improving the digestion efficiency. To reduce the interference of impurities, 1:1 was chosen as the optimal enzyme-protein ratio. When the enzyme digestion time was changed from 0.25 to 24 h, the signal response was not significantly changed. For complete digestion, 0.5 h is enough.
FIGURE 4. Optimization of digestion conditions. (A) Final concentration of DTT reduction (5, 10, 20, 50, and 100 mM) and alkylation with iodoacetamide (DTT-IA ration 1:2). (B) Enzyme-protein ratio (2:1, 1:1, 1:5, 1:20, and 1:50). (C) Enzyme digestion time (0.25, 0.5, 1, 2, 4, and 24 h) with trypsin at 37°C. Bars are mean ± SE (n = 3).
The LC-MS/MS-MRM method was validated in regard to specificity, linearity and sensitivity, accuracy and precision, recovery, stability, and repeatability. The blank sample, control sample, test sample solution, and the other snake venom samples were prepared with the same pretreatment method and then analyzed by LC-MS/MS-MRM. As shown in Figure 5, the marker peptide was detected in the test and control samples, but not in the blank sample. There were no interference peaks in the spectrum throughout the test. This method can distinguish the analyte from the matrix with good specificity. As for the snake venom of Vipera russelli siamensis, Bothrops atrox, and Deinagkistrodon acutus, in the spectrum, miscellaneous peaks appeared in other retention times with poor peak shape due to many interference factors in complex matrix samples. But there was no chromatographic peak at the same retention time. These results suggest that this method is highly species-specific and can identify Agkistrodon halys pallas from Vipera russelli siamensis, Bothrops atrox, and Deinagkistrodon acutus. The control solution was diluted into a series of appropriate concentrations and analyzed by the LC-MS/MS. The result shows the linear relationship between the peak area and the concentration of the marker peptide. The linear equations was y = 844208x -19,827. R2, with a coefficient of determination of 0.999. The LOD and LOQ were evaluated when the targeted peak intensity was at least 3 and 10 times higher than that in the matrix, which was 2 ng/ml and 6 ng/ml, respectively.
FIGURE 5. MRM investigation of TLCAGVMEGGIDTCNR in the blank sample, control sample, sample solution, and the other snake venom samples. (A) Qualitative ion pair (m/z = 877.6 > 550.3). (B) Quantitative ion pair (m/z = 877.6 > 892.5).
The precision of the method was evaluated by the relative standard deviation (RSD). The sample was injected into the HPLC-MS/MS six times continuously. The mean value was 14.53 μg/ml and the RSD was 2.1%, which shows the excellent precision of the method (Supplementary Table S1). The repeatability of the method was evaluated by preparing samples in sextuplicate. The RSD was 3.2% for the concentration level of 14.40 μg/ml (Supplementary Table S2). These results indicated a good reproducibility of pretreatment procedures. These precision and repeatability results were within the acceptable range, which means the method was precise and repeatable. Some samples may have a low signal or even no signal because of the matrix effect, or something in the matrix had a coincident peak with the targeted peptide, which would affect the accuracy of the result. Analyte recoveries can be used to assess the matrix effect. A certain amount of synthetic peptide was spiked into Hemocoagulase and then detected by LC-MS/MS-MRM. Recovery is defined as the ratio of the actual value of the synthetic peptide measured after the test to the theoretical amount. The quantitative ability of the method can be evaluated using the recovery and the RSD. As shown in Table 2, the recovery of three different concentrations of six replicates ranges from 86.0% to 100.7%. The mean recovery was 93.3% and the RSD was 6.3%, which was acceptable for peptide detection. Sample stability is critical for the long-term analysis of a large number of samples. The sample solution was kept at 8°C in the auto-sampler and injected for detection after 0h, 2h 4, 8, 10, and 24 h. The RSD of the peak area corresponding to the quantitative ion pair of the marker peptide was calculated and the result was <5%, indicating that the marker peptide in the solution was stable within 24 h at 8°C (Supplementary Table S3). The developed LC-MS/MS-MRM method was applied for the quantitative determination of three batches of Hemocoagulase Agkistrodon halys pallas (20190201, 20190202, and 20190203). The results showed that the contents of TLE were 15.33 μg/ml, 14.42 μg/ml, and 15.50 μg/ml, and there were no significant differences among the three batches (Supplementary Table S4).
Hemocoagulase is one of the most important and indispensable drugs used in surgical operations. Hemocoagulase for Injection is a member of the biological product family, exhibiting a fine hemostasis and coagulation efficacy, which has been widely used as a hemostatic in China (Li et al., 2019). At present, the hemagglutinin drugs on the market in China are mainly isolated from the venom of Bothrops atrox (Baquting®), Deinagkistrodon acutus (Suling®), Vipera russelli siamensis (Sulejuan®), and Agkistrodon halys pallas (Bangting®), and their main active ingredients are svTLEs. However, svTLEs from different snake species vary in structures, hemostatic mechanisms, and pharmacological effects. Therefore, the accurate identification of snake venom species and the content of svTLEs is essential to ensure the quality of this product family. The existing quality control technology has been able to ensure the stability of the product quality, but the species cannot be identified. The identification and protein detection methods on snake venom species are mainly ELISA, liquid chromatography, and electrophoresis, which have disadvantages including complexity, low sensitivity, and poor specificity.
With the rapid development of biological mass spectrometry technology, mass spectrometry-based proteomics study is becoming increasingly mature. The proteomics technology based on peptide biomarkers provides a new idea for food and drug quality control (Huang and Wang, 2021; Marques et al., 2021). A marker peptide refers to an amino acid sequence which is unique for a protein and can be specifically distinguished from other proteins. Because of the differences in the DNA of different species, there should be differences in the amino acid sequences for proteins translated by DNA. Based on this principle, different peptides can be obtained after the hydrolysis of proteins by specific proteases, which can be used as characteristic markers for species identification or qualitative and quantitative analysis of target proteins.
The qualitative and quantitative analysis technology of proteins based on marker peptides has been successfully applied to the identification of meat products, the adulteration screening of traditional Chinese medicines, etc. However, there are few studies in the field of biochemical drugs, and there are no reports on the qualitative and quantitative study of snake venom blood coagulation based on marker peptides. In this study, the sequence of the pit viper thrombin-like enzyme was characterized by combining transcriptomics and proteomic approaches. By the means of bioinformatics, a series of potential marker peptides which can be distinguished from other snake species have been identified. After the method optimization, a rapid LC-MS/MS-MRM method for the qualitative and quantitative analysis of hemocoagulase was established. This study improved the quality standard of Hemocoagulase Agkistrodon halys pallas, which can ensure the safety and effectiveness of clinical medications. The study also provided a new strategy for the quality control of other snake venom products. However, as Hemocoagulase for injection contains a lot of gelatin and other ingredients, a new pre-treatment method needs to be developed to reduce matrix interference in future.
The original contributions presented in the study are included in the article/Supplementary Material; further inquiries can be directed to the corresponding author.
Ethical review and approval were not required for the animal study because Hemocoagulase Agkistrodon halas pallas studied were commercialized and provided by the Avanc Pharma. This study does not involve animal experiments.
RX, FW, and FS contributed to the conception and experimental design. HD provided the venom gland and Hemocoagulase Agkistrodon halys pallas stocks studied in this article. RX and FS provided genome sequencing and ORF sequence translation. CW, RX, and LG performed data generation, analysis, and interpretation of the results. BH, WW, and XZ prepared the figures. All authors drafted the manuscript. FW and FS reviewed and edited the final manuscript.
This study was supported by the Chinese Pharmacopoeia Commission of the “Formulation and Revision of Drug Specifications” research project (Grant No. 2019H04).
HD was employed by the R&D Department, Avanc Pharmaceutical Co., Ltd.
The remaining authors declare that the research was conducted in the absence of any commercial or financial relationships that could be construed as a potential conflict of interest.
All claims expressed in this article are solely those of the authors and do not necessarily represent those of their affiliated organizations or those of the publisher, the editors, and the reviewers. Any product that may be evaluated in this article, or claim that may be made by its manufacturer, is not guaranteed or endorsed by the publisher.
The authors are grateful to Meilian He for her help in the preparation of the figures.
The Supplementary Material for this article can be found online at: https://www.frontiersin.org/articles/10.3389/fmolb.2022.831293/full#supplementary-material
Alaiya, A., Alshukairi, A., Shinwari, Z., Al-Fares, M., Alotaibi, J., AlOmaim, W., et al. (2021). Alterations in the Plasma Proteome Induced by SARS-CoV-2 and MERS-CoV Reveal Biomarkers for Disease Outcomes for COVID-19 Patients. Jir 14, 4313–4328. doi:10.2147/JIR.S322430
Bai, S., Ye, R., Wang, C., Sun, P., Wang, D., Yue, Y., et al. (2021). Identification of Proteomic Signatures in Chronic Obstructive Pulmonary Disease Emphysematous Phenotype. Front. Mol. Biosci. 8, 650604. doi:10.3389/fmolb.2021.650604
Britt, H. M., Cragnolini, T., and Thalassinos, K. (2022). Integration of Mass Spectrometry Data for Structural Biology. Chem. Rev 122, 7952–7986. doi:10.1021/acs.chemrev.1c00356
Castro, H. C., Zingali, R. B., Albuquerque, M. G., Pujol-Luz, M., and Rodrigues, C. R. (2004). Snake Venom Thrombin-like Enzymes: from Reptilase to Now. Cell. Mol. Life Sci. (CMLS) 61, 843–856. doi:10.1007/s00018-003-3325-z
Dong, X., Sun, J., Miao, W., Chang, C.-e. A., and Wang, Y. (2021). Proteome-Wide Characterizations of N6-Methyl-Adenosine Triphosphate- and N6-Furfuryl-Adenosine Triphosphate-Binding Capabilities of Kinases. Anal. Chem. 93, 13251–13259. doi:10.1021/acs.analchem.1c02565
Funk, C., Gmür, J., Herold, R., and Straub, P. W. (1971). Reptilase-R-A New Reagent in Blood Coagulation. Br. J. Haematol. 21, 43–52. doi:10.1111/j.1365-2141.1971.tb03415.x
Grabarics, M., Lettow, M., Kirschbaum, C., Greis, K., Manz, C., and Pagel, K. (2022). Mass Spectrometry-Based Techniques to Elucidate the Sugar Code. Chem. Rev. 122, 7840–7908. doi:10.1021/acs.chemrev.1c00380
Guan, L.-f., Chi, C.-w., and Yuan, M. (1984). Study on the Thrombin-like Enzyme Preferentially Releasing Fibrinopeptide B from the Snake Venom of agkistrodon Halys Pallas. Thrombosis Res. 35, 301–310. doi:10.1016/0049-3848(84)90361-x
Huang, M., and Wang, Y. (2021). Global and Targeted Profiling of Gtp‐binding Proteins in Biological Samples by Mass Spectrometry. Mass Spec. Rev. 40, 215–235. doi:10.1002/mas.21637
Li, B.-H., Yu, Z.-J., Wang, C.-Y., Zi, H., Li, X.-D., Wang, X.-H., et al. (2019). A Preliminary, Multicenter, Prospective and Real World Study on the Hemostasis, Coagulation, and Safety of Hemocoagulase Bothrops Atrox in Patients Undergoing Transurethral Bipolar Plasmakinetic Prostatectomy. Front. Pharmacol. 10, 1426. doi:10.3389/fphar.2019.01426
Li, H., Huang, Y., Wu, X., Wu, T., Cao, Y., Wang, Q., et al. (2018). Effects of Hemocoagulase agkistrodon on the Coagulation Factors and its Procoagulant Activities. Dddt 12, 1385–1398. doi:10.2147/DDDT.S159210
Li, X., Shi, F., Gong, L., Hang, B., Li, D., and Chi, L. (2017). Species-specific Identification of Collagen Components in Colla Corii Asini Using a Nano-Liquid Chromatography Tandem Mass Spectrometry Proteomics Approach. Ijn 12, 4443–4454. doi:10.2147/IJN.S136819
Lu, S., Zhang, J., Lian, X., Sun, L., Meng, K., Chen, Y., et al. (2019). A Hidden Human Proteome Encoded by 'non-Coding' Genes. Nucleic Acids Res. 47, 8111–8125. doi:10.1093/nar/gkz646
Marques, T. M., van Rumund, A., Kersten, I., Bruinsma, I. B., Wessels, H. J. C. T., Gloerich, J., et al. (2021). Identification of Cerebrospinal Fluid Biomarkers for Parkinsonism Using a Proteomics Approach. npj Park. Dis. 7, 107. doi:10.1038/s41531-021-00249-9
Sajevic, T., Leonardi, A., and Križaj, I. (2011). Haemostatically Active Proteins in Snake Venoms. Toxicon 57, 627–645. doi:10.1016/j.toxicon.2011.01.006
Sheng, A., Chen, Q., Yu, M., Xiao, R., Zhang, T., Wang, Z., et al. (2021). Coupling Liquid Chromatography and Tandem Mass Spectrometry to Electrophoresis for In-Depth Analysis of Glycosaminoglycan Drugs: Heparin and the Multicomponent Sulodexide. Anal. Chem. 93, 1433–1442. doi:10.1021/acs.analchem.0c03330
Shi, F., Guo, C., Gong, L., Li, J., Dong, P., Zhang, J., et al. (2014). Application of a High Resolution Benchtop Quadrupole-Orbitrap Mass Spectrometry for the Rapid Screening, Confirmation and Quantification of Illegal Adulterated Phosphodiesterase-5 Inhibitors in Herbal Medicines and Dietary Supplements. J. Chromatogr. A 1344, 91–98. doi:10.1016/j.chroma.2013.12.030
Suvarna, K., Salkar, A., Palanivel, V., Bankar, R., Banerjee, N., Gayathri J Pai, M., et al. (2021). A Multi-Omics Longitudinal Study Reveals Alteration of the Leukocyte Activation Pathway in COVID-19 Patients. J. Proteome Res. 20, 4667–4680. doi:10.1021/acs.jproteome.1c00215
Ullah, A., Masood, R., Ali, I., Ullah, K., Ali, H., Akbar, H., et al. (2018). Thrombin-like Enzymes from Snake Venom: Structural Characterization and Mechanism of Action. Int. J. Biol. Macromol. 114, 788–811. doi:10.1016/j.ijbiomac.2018.03.164
Waheed, H., Moin, S. F., and Choudhary, M. I. (2017). Snake Venom: From Deadly Toxins to Life-Saving Therapeutics. Cmc 24, 1874–1891. doi:10.2174/0929867324666170605091546
Wang, L., Zhou, T., Zhang, Y., Yang, E. S., Schramm, C. A., Shi, W., et al. (2021). Antibodies with Potent and Broad Neutralizing Activity against Antigenically Diverse and Highly Transmissible SARS-CoV-2 Variants. bioRxiv [Preprint]. doi:10.1101/2021.02.25.432969
Wang, W., Wu, J., Liu, P., Tang, X., Pang, H., Xie, T., et al. (2021). Urinary Proteomics Identifying Novel Biomarkers for the Diagnosis and Phenotyping of Carotid Artery Stenosis. Front. Mol. Biosci. 8, 714706. doi:10.3389/fmolb.2021.714706
Wang, Y. T., Zhang, H. L., and Chen, X. (2021). Qualitative Identification of Horns in Compound Xiling Jiedu Capsules by Mass Spectrometry. Drug Stand. China 22, 337–340. doi:10.19778/j.chp.2021.04.009
Yang, X.-L., Shi, Y., Zhang, D.-D., Xin, R., Deng, J., Wu, T.-M., et al. (2021). Quantitative Proteomics Characterization of Cancer Biomarkers and Treatment. Mol. Ther. - Oncolytics 21, 255–263. doi:10.1016/j.omto.2021.04.006
Zeng, F., Shen, B., Zhu, Z., Zhang, P., Ji, Y., Niu, L., et al. (2013). Crystal Structure and Activating Effect on RyRs of AhV_TL-I, a Glycosylated Thrombin-like Enzyme from Agkistrodon Halys Snake Venom. Arch. Toxicol. 87, 535–545. doi:10.1007/s00204-012-0957-5
Zhang, B., and Chi, L. (2021). Chondroitin Sulfate/Dermatan Sulfate-Protein Interactions and Their Biological Functions in Human Diseases: Implications and Analytical Tools. Front. Cell Dev. Biol. 9, 693563. doi:10.3389/fcell.2021.693563
Zhang, B., Whiteaker, J. R., Hoofnagle, A. N., Baird, G. S., Rodland, K. D., and Paulovich, A. G. (2019). Clinical Potential of Mass Spectrometry-Based Proteogenomics. Nat. Rev. Clin. Oncol. 16, 256–268. doi:10.1038/s41571-018-0135-7
Keywords: hemocoagulase, snake venom thrombin-like enzymes, marker peptide, mass spectrometry, proteomics, MRM
Citation: Xian R, Wang C, Gong L, Hang B, Wang W, Zhang X, Du H, Wang F and Shi F (2022) A Species-Specific Strategy for the Identification of Hemocoagulase Agkistrodon halys pallas Based on LC-MS/MS-MRM. Front. Mol. Biosci. 9:831293. doi: 10.3389/fmolb.2022.831293
Received: 08 December 2021; Accepted: 25 April 2022;
Published: 30 May 2022.
Edited by:
Qunye Zhang, Shandong University, ChinaReviewed by:
Chao Cai, Ocean University of China, ChinaCopyright © 2022 Xian, Wang, Gong, Hang, Wang, Zhang, Du, Wang and Shi. This is an open-access article distributed under the terms of the Creative Commons Attribution License (CC BY). The use, distribution or reproduction in other forums is permitted, provided the original author(s) and the copyright owner(s) are credited and that the original publication in this journal is cited, in accordance with accepted academic practice. No use, distribution or reproduction is permitted which does not comply with these terms.
*Correspondence: Feng Shi, MTM5NTMxNzUzMjFAMTM5LmNvbQ==
†These authors have contributed equally to this work
Disclaimer: All claims expressed in this article are solely those of the authors and do not necessarily represent those of their affiliated organizations, or those of the publisher, the editors and the reviewers. Any product that may be evaluated in this article or claim that may be made by its manufacturer is not guaranteed or endorsed by the publisher.
Research integrity at Frontiers
Learn more about the work of our research integrity team to safeguard the quality of each article we publish.