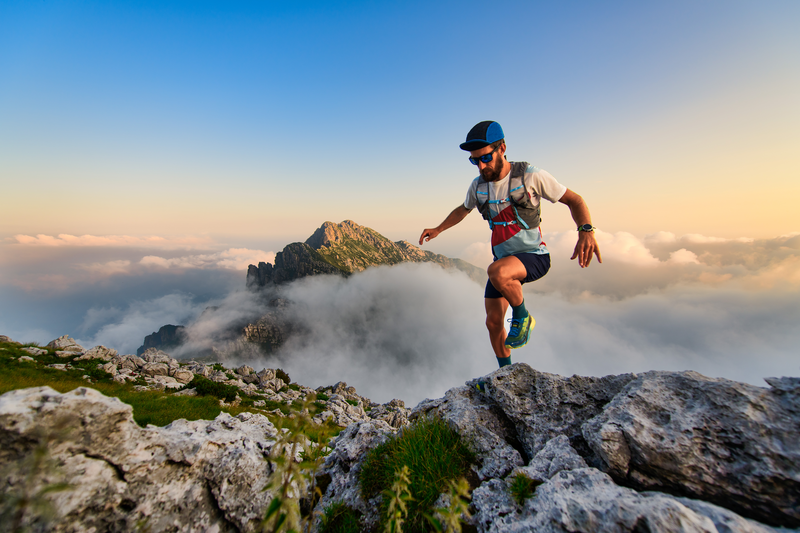
94% of researchers rate our articles as excellent or good
Learn more about the work of our research integrity team to safeguard the quality of each article we publish.
Find out more
REVIEW article
Front. Mol. Biosci. , 07 November 2022
Sec. Molecular Diagnostics and Therapeutics
Volume 9 - 2022 | https://doi.org/10.3389/fmolb.2022.1052878
This article is part of the Research Topic Oxidative Stress in Degenerative Bone and Joint Diseases: Novel Molecular Mechanisms and Therapeutic Potential View all 7 articles
Intervertebral disc degeneration (IDD) is the primary cause of intervertebral disc (IVD) disease. With the increased ageing of society, an increasing number of patients are plagued by intervertebral disc disease. Ageing not only accelerates the decreased vitality and functional loss of intervertebral disc cells but also increases intracellular oxidative stress. Moreover, the speed of intervertebral disc ageing is also linked to high levels of reactive oxygen species (ROS) production. Not only is the production of ROS increased in ageing intervertebral disc cells, but antioxidant levels in degenerative intervertebral discs also decrease. In addition to the intervertebral disc, the structural components of the intervertebral disc matrix are vulnerable to oxidative damage. After chronic mitochondrial dysfunction, ROS can be produced in large quantities, while autophagy can eliminate these impaired mitochondria to reduce the production of ROS. Oxidative stress has a marked impact on the occurrence of IDD. In the future, IDD treatment is aiming to improve oxidative stress by regulating the redox balance in intervertebral disc cells. In summary, ageing and oxidative stress promote the degeneration of IVD, but further basic and clinical trials are needed to determine how to treat oxidative stress. At present, although there are many in-depth studies on the relationship between oxidative stress and degeneration of intervertebral disc cells, the specific mechanism has not been elucidated. In this paper, the main causes of intervertebral disc diseases are studied and summarized, and the impact of oxidative stress on intervertebral disc degeneration is studied.
With the process of increased societal ageing, the number of patients with intervertebral disc disease is increasing worldwide, resulting in a huge social and economic burden (Vos et al., 2012; Knezevic et al., 2021) A large number of studies have shown that Intervertebral disc degeneration (IDD) is the main cause of intervertebral disc disease and that IDD is associated with the severity of the disease (Takatalo et al., 2011). The degenerative process of IDD includes structural damage to the intervertebral disc and changes in various components. The intervertebral disc (IVD) is a fibrocartilaginous structure that not only provides flexibility between the vertebral bodies but also transmits compressive loads. It is very different from the connective tissue in the body; for example, degenerative ageing occurs early in life (Boos et al., 2002). Histological studies revealed reduced blood supply to the vertebral body starting in the second decade of life, and according to current research reports, there is a positive correlation between IDD prevalence and increasing age (Benneker et al., 2005). Relevant studies have shown that the cells in the IVD are closely related to changes in the nutrition of the matrix components, and their survival and nutrition depend on the nutrition in the matrix. The ageing of the IVD has dual hazards: 1. It leads to the loss of intervertebral cell viability and function; and 2. It releases substances such as matrix proteases and chemokines (Khan et al., 2017). The nonvascular characteristics of IVD affect the clearance of ageing IVD cells, inducing inflammation and catabolism, which affect the IVD microenvironment and accelerate IDD (Che et al., 2020). Therefore, understanding the interaction between spinal tissues during degeneration will enhance our understanding of the pathogenesis of this condition and will help to develop new and more effective treatments for IDD.
Oxidative stress (OS) refers to a state of imbalance between oxidation and antioxidant effects in the body. Loss of redox balance can lead to damage to key biological molecules and cells, potentially affecting organisms as a whole (Ďuračková, 2010). According to the latest research, the occurrence of IDD is closely related to OS caused by ROS (Hou et al., 2014; Dimozi et al., 2015). For example, a series of changes that affect gene expression and metabolism and the speed of IVD senescence have also been linked to the levels of ROS production (Vo et al., 2013). Therefore, we discuss the significance of IVD cell senescence in the pathogenesis of IDD and the significance of OS in IDD Elucidating the effects of ageing and OS on IDD will aid in elucidating the pathogenesis of IDD and developing a promising treatment.
The IVD consists of three layers: annulus fibrosus, central nucleus pulposus and endplate (Colombini et al., 2008). The IVD is a vascular-free structure composed of fibrous tissue and cartilage (Boxberger et al., 2009). Cells and matrix are the basis of the normal function of IVD. IDD refers to the functional and structural failure of the IVD, which is related to its cellular pathogenesis and extracellular matrix (ECM) modification, including structural damage to the IVD and changes in cell number and other components, such as decreased elasticity of the IVD and tearing of the fibrous annulus (AF), leading to loss of water in the nucleus pulposus (NP) and calcification of the cartilage endplate (Figure 1). With increasing age, the composition of the ECM of the IVD changes significantly (Roughley, 2004). A variety of interdependent factors, including changes in mechanical load, reduced nutrition supply and genetic factors, are related to the progression of degradation cascades. Changes in ECM components of the IVD with age can be attributed to changes in function and the increase in death of cells that make up the IVD (Stokes and Iatridis, 2004; Battié and Videman, 2006; Zhao et al., 2007).
FIGURE 1. Pathological manifestation and mechanism of intervertebral disc degeneration. Intervertebral disc degeneration (IDD) includes many aspects, such as decreased disc elasticity, cartilage end-plate calcification, reduced moisture in nucleus pulposus, annulus fibrosus tears, and changes in extracellular matrix (ECM) components. Intervertebral disc cell senescence plays a major role in IDD, which can cause the reduction of ECM, and can secrete inflammatory factors leading to the inflammatory state of intervertebral disc microenvironment, thus causing IDD.
Although some mechanical stimulation is necessary for nutrient diffusion and matrix synthesis, excessive mechanical stimulation can lead to tissue loss and further change the strain distribution of the ECM between the vertebrae (Stokes and Iatridis, 2004). The discovery of ageing IVD cells has become the discovery point for further study of the pathogenesis of IDD. On the one hand, ageing IVD cells cannot produce new IVD cells, and the number of functional cells in the disc is reduced due to cell death. On the other hand, catabolic and proinflammatory phenotypes are the primary characteristic phenotypes of the senescence-related secretory phenotype (SASP) of disc cells (Le Maitre et al., 2007a). Ageing IVD cells may change their secretory pattern, thus altering the microenvironment of the IVD (van Deursen, 2014). They reduce the production of ECM in vitro while enhancing its degradation. In addition, the senescence of adjacent intervertebral cells and infiltration of immune cells that enhance inflammation of the degenerative disc microenvironment are due to proinflammatory cytokines secreted by senescent disc cells (Muñoz-Espín and Serrano, 2014).
In conclusion, phenotypic changes in ageing IVD cells, IVD ECM anabolism and catabolism are influencing factors in the IDD process.
The balance between ROS production and removal directly determines intracellular redox homeostasis. ROS are inevitable in the process of cellular aerobic metabolism. When encountering stress, ROS will accumulate in cells, causing oxidative damage to proteins, DNA, and lipids and cell damage. Therefore, it has long been considered that ROS are a class of toxic molecules (Kim and Yim, 2015). As a key part of the antioxidant enzyme system, superoxide dismutase (SOD) is common in animals, plants and microorganisms and is inseparable from the occurrence and development of many diseases (Figure 2). SOD has strong oxidability and can catalyse the conversion of oxygen anion (O2−) to hydrogen peroxide (H2O2) primarily because O2− is produced by the Nox activity of the SOD enzyme and converts to H2O2. Reactive nitrogen species (RNS) are also classified as ROS because they have similar functions to ROS (Gorrini et al., 2013; Filomeni et al., 2015). Mitochondria are the primary production sites of ROS. During the process of electron transport, a small number of electrons leak and reduce O2 to O2− instead of H2O (Gille and Nohl, 2001). In addition, the main factors affecting intracellular redox homeostasis are the balance between ROS production and scavenging of ROS by nonenzymatic and enzymatic antioxidants, including reduced glutathione (GSH), catalase (CAT), glutathione peroxidase, and ascorbic acid (vitamin C) (Jones, 2008; Trachootham et al., 2008). These moieties are distributed in different parts of the cell and can interact with other molecules, including hydrooxidase and tocopherol, to remove ROS. Cellular senescence is an important mechanism that limits the proliferation of potential cancer cells (van Deursen, 2014).
FIGURE 2. Relationship between aging and oxidative stress. Stress-induced premature senescence (SIPS) and natural senescence of cells can cause mitochondrial dysfunction, resulting in the production of a large number of ROS, such as oxygen anion (O2−), hydroxyl radical (OH−), hydrogen peroxide (H2O2), NO and hypochlorite (OCl−). When the production of ROS is greater than its own scavenging capacity, it will cause oxidative stress and cause cell damage and accelerate cell senescence, which further promotes the development of age-related degenerative diseases.
Ageing is a complex process that leads to the functional decline of multiple tissues and organs. The ageing process is closely related to a variety of characteristics at the molecular, cellular and physiological levels, such as changes in the genome and epigenome, loss of protein homeostasis, decline in overall cellular and subcellular functions, and disorder of the signalling system. Another type of cellular senescence, stress-induced premature senescence (SIPS), is a result of the accumulation of genomic and mitochondrial DNA damage (Campisi, 2005; Acosta et al., 2008). In addition, SIPS cells are also related to senescence-related secretory phenotypes and can secrete a large number of inflammatory cytokines and matrix proteases, which decompose neighbouring cells and ECM to promote cellular degeneration (Rodier et al., 2009; Baker et al., 2011). Mitochondrial function decreases with age in various tissues and cell types (Ugalde et al., 2010). Mitochondria are the main source of ROS. Mitochondrial dysfunction increases the production of ROS and causes OS, which leads to cell damage and promotes the progression of ageing. Mitochondrial disease or dysfunction is ultimately an energy production problem because mitochondria are an important energy supply site in cells. Once mitochondria fail, it becomes more common to experience nutrient shortages (Harman, 1956; Jones, 2015). In addition, ageing and chronic degenerative diseases are accompanied by symptoms of mitochondrial dysfunction and inflammation. The former is one cause of ageing. When ageing occurs, mitochondrial DNA suffers oxidative damage to varying degrees, leading to damage to cellular energy metabolism, cell dysfunction, and even death. The relationship between the antioxidant capacity of mitochondria and changing age, as well as the increase in OS, cause physiological cell signal disorder, which damages cellular integrity and accelerates ageing.
Apoptosis is one mechanism of cell death. Apoptosis can reduce the function and the number of surviving IVD cells, which is also the primary factor that induces IDD (Ding et al., 2013; Kepler et al., 2013). ROS are important to OS, and mitochondrial dysfunction is the main cause of excessive ROS. Previous studies have reported a decrease in mitochondria and an increase in mitochondrial respiration in human AF cells with IDD progression (Gruber et al., 2013). In addition, various stimuli, such as hyperoxic tension, high glucose pressure and abnormal mechanical load, have been shown to cause mitochondrial dysfunction in animal IVD cells (Ma et al., 2013; Jiang et al., 2014; Cai et al., 2015). These stimuli promote the production of ROS in NP and AF cells, and apoptosis can be further triggered by mitochondrial apoptosis (Ding et al., 2012). On the other hand, mitochondria are the main targets of ROS. Oxidative damage to mitochondrial DNA and respiratory enzymes further leads to mitochondrial dysfunction, causing a vicious cycle of mitochondrial and OS (Gruber et al., 2011). In addition, according to relevant studies, iron death is mainly an iron-dependent regulatory cell death characterized by lipid peroxidation of the cell membrane. Iron death is a recently discovered type of programmed cell death that plays an important role in tumour biology and therapy. Yang et al. (2021) found that OS can induce iron death in rat NP cells, while inhibition of iron death can slow the process of IDD in vivo (Figure 3).
FIGURE 3. The role of oxidative stress in intervertebral disc cells. Mitochondria are the main source of ROS. Some harmful external factors such as hyperoxia, high glucose pressure and abnormal mechanical load can cause mitochondrial dysfunction in nucleus pulposus (NP) cells. The dysfunction of mitochondria causes a series of elevated levels of ROS, such as oxygen anion (O2−), hydrogen peroxide (H2O2) and NO. Oxidative damage of mitochondrial DNA and respiratory enzymes further lead to mitochondrial apoptosis and further accelerate mitochondrial dysfunction, which leads to a vicious circle between mitochondria and oxidative stress. Apoptosis of NP cells induced by mitochondrial apoptosis or functional impairment will accelerate the progress of IDD. The oxidative stress caused by the increase of ROS level can induce iron death, while iron death can inhibit the progress of IDD.
H2O2 is a member of the ROS family, and it plays a wide role in IVDs. H2O2 can increase the permeability of the lysosomal membrane (Kim et al., 2009). Excessive ROS induces apoptosis of NP cells through the mitochondrial apoptotic pathway. In addition, H2O2 promotes ROS production and DNA damage in human NP cells (Zhou et al., 2016). In addition, overproduction of ROS induced by hyperglycaemia accelerates the senescence of annulus fibrosus and notochord cells in rats through the p16-Rb pathway (Krupkova et al., 2016). In fact, previous studies have reported excessive ROS production in degenerative IVDs, including a significant increase in NO levels in rat degenerative IVDs (Hou et al., 2014). Peroxynitrite is an effective oxidant formed by the interaction of O2 and NO in the body, which will further lead to tyrosine nitrosation and is a sign of excessive ROS (Hogg et al., 1992).
Nitrotyrosine-positive cells in human NP tissue increase with the development of IDD (Poveda et al., 2009). In a previous study, Schleicher et al. (1997) showed that advanced glycosylation end products (AGEs) are products of the oxidative modification of glycosylated proteins, including carboxymethyl lysine (CML) and pentosyl glycosides. CML and pentose accumulate in the degenerative IVD. To a certain extent, the degree of CML enhancement and IDD in the IVD will also increase (Nerlich et al., 1997) In addition, the key influencing factors of OS and apoptosis of human NP cells are the dysfunction of Sirtuin3 (SIRT3) and the mitochondrial antioxidant network. It is reported that oxidative stress induces excessive mitochondrial autophagy and further leads to cell death of human and rat NP cells (Xu et al., 2019). Another study found that OS can lead to mitochondrial dysfunction and mitosis in NP cells, while short hairpin RNA depletion of PINK1 further weakens mitosis and aggravates the senescence of NP cells under OS, indicating that PINK1-mediated mitosis is very important in protecting oxidative stress-related mitochondrial damage and cell senescence (Wang et al., 2018). Further studies have shown that salidroside upregulated Parkin may eliminate damaged mitochondria and promote the survival of NP cells by activating mitosis in vitro. In vivo experiments, Zhang et al. (2018) found that salidroside can inhibit the apoptosis of NP cells and improve the progress of IDD. Another study showed that melatonin alleviates intervertebral disc degeneration through mitochondrial autophagy induction and apoptosis inhibition (Chen et al., 2019). In addition, Urolitin A can induce mitochondrial autophagy and inhibit apoptosis and reduce intervertebral disc degeneration through activation of 5′-monophosphate-activated protein kinase (AMPK) signal pathway (Lin et al., 2020).
The degradation of IVD mainly involves the reduction of water content and ECM decomposition. It represents the loss of steady-state metabolism, which is primarily caused by an imbalance between anabolism and catabolism, which also demonstrates that anabolism and catabolism play important roles in the IDD process (Horner and Urban, 2001; Sobajima et al., 2005). Moreover, metabolism of the ECM is closely related to the redox state in the IVD (Yang D. et al., 2014). The ECM of IVDs primarily includes the matrix network and collagen (Hwang et al., 2014). This network is essential for the IVD as a shock absorber to resist the mechanical load applied on the spine (Le Maitre et al., 2007b). Posttranslational oxidative modification of the IVD matrix occurs during IDD. Numerous studies have shown that H2O2 can significantly downregulate the expression of type II collagen and proteoglycans in human and rat IVD cells (Wei et al., 2008). Excessive production of ROS induced by anti-inflammatory cytokines or hyperoxia significantly inhibits matrix synthesis and regulates the expression of matrix-degrading proteases in human and rat IVD cells (Scharf et al., 2013) (Figure 4).
FIGURE 4. Oxidative stress in extracellular matrix of intervertebral disc. Piezo1 and high glucose environment can promote oxidative stress to induce more ROS production. Excessive ROS can cause changes in the structure and composition of extracellular matrix (ECM). For example, ROS can lead to a large loss of PG, collagen and fibronectin in intervertebral discs, oxidative damage of collagen I and collagen II lose their natural primary and secondary structures, and excessive ROS can cause the expression of matrix degradation proteases. These changes in ECM can cause reduced moisture and elasticity in the intervertebral disc, thus accelerating the progression of intervertebral disc degeneration (IDD).
Some studies have found that AGEs increase IVD denaturation mainly by promoting cell apoptosis and preventing ECM metabolism. The primary components are pentoside and carboxymethyl lysine (Vistoli et al., 2013). Pentoside is distributed in collagen, and collagen molecules are cross-linked. Collagen stiffness and cartilage biomechanical dysfunction may play an important role with age (DeGroot et al., 1999). The subsequent significant loss of elasticity and stiffness of the IVD damages the mechanical function of the IVD and leads to IDD. The key influencing factor of ageing and apoptosis in human IDD is matrix hardness-activated Piezo1 (Wang et al., 2021). OS induced by high glucose may mediate apoptosis and imbalance of ECM metabolism through p38-MAPK activation in rat NP cells (Cheng et al., 2016).
Inflammation can maintain physiological and pathological homeostasis during infection, but excessive proinflammatory factors can cause damage to the body, with persistent and intense inflammation leading to serious diseases (Risbud and Shapiro, 2014; Žerovnik et al., 2020). Park et al. (2007) found that compared to the control group, patients with lumbar disc herniation exhibited higher levels of serum IL-2, IL-6, IL-8, and tumour necrosis factor. In a pathological model of IDD, it was found that NP cells and fibrocartilage rings (fibrocartilage tissue containing NP) were abnormally regulated by proinflammatory molecules released by macrophages, T cells and neutrophils, indicating that IVD-derived cells can initiate or amplify inflammation (Kraychete et al., 2010). Based on these findings, inflammation plays an important role in the process of IDD. Moreover, chemokines secreted by IVD cells reduce cell vitality and cause functional degradation, resulting in a vicious cycle (Risbud and Shapiro, 2014).
In general, inflammatory tissue is associated with increased levels of active substances (ROS and RNS) produced by immune cells and is essential to help fight foreign pathogens (Lei et al., 2015). These active substances react with various metabolic substances in biological molecules. Nitrosation substances affect cellular signal transduction and regulate the inflammatory response (Chiurchiù and Maccarrone, 2011). Once the cellular pro-oxidation and antioxidation system fails, excessive inflammation will occur, and the production of ROS will increase (Miki and Funato, 2012). Degeneration of IVD is related to local increases in IL-1 β (Le Maitre et al., 2005), which may be because IL-1 β can induce apoptosis through mitochondrial dysfunction and endoplasmic reticulum stress (López-Armada et al., 2006; Caramés et al., 2008; Kim et al., 2010). Elevated ROS activates multiple signalling pathways of intervertebral cells during oxidative stress, including the NF-κB and mitogen-activated protein kinase (MAPK) pathways (Feng et al., 2017). NF-κB is a transcription factor that regulates the gene encoding proinflammatory cytokines and is also a potential therapeutic target for various inflammatory diseases (Wang et al., 2009).
ROS can induce or mediate activation of the MAPK pathway (McCubrey et al., 2006). The metabolism of NF-κB in NP cells is regulated by H2O2 and peroxynitrite (Loukili et al., 2010; Risbud and Shapiro, 2014). Inflammatory factors of receptor heat protein domain related protein 3 (NLRP3), which exert adverse effects on metabolism, aggravate the apoptosis of NP cells and lead to the degeneration of IVD (Tang et al., 2018).
In addition, mitochondrial dysfunction and the production of mitochondrial ROS promote the activation of NLRP3 inflammatory bodies. Mitochondria provide cellular energy and can also regulate cellular functions, such as calcium concentration in the internal cellular environment, signal transduction, and apoptosis. Loss of mitochondrial function and impaired ATP production were found to induce cell death. In the past decade, increasing evidence has demonstrated that mitochondria are involved in NLRP3 inflammasome activation and pyroptosis, playing an important role in this process. Mitochondria participate in the regulation of the natural immune system, which has been an important discovery in recent years (Tang et al., 2018).
Autophagy provides energy by self-digesting damaged or ageing organelles to protect cells from various harmful external stimuli (Kim and Lee, 2014). ROS are produced in the mitochondria, and this process can regulate autophagy. High levels of ROS can be produced after chronic damage to mitochondrial function. Autophagy can eliminate these impaired mitochondria to reduce the production of ROS. Through this mechanism, autophagy can cut off the source of OS and reduce cellular damage (Filomeni et al., 2015).
Many studies have shown that the increase in intracellular H2O2 concentration not only enhances the AMPK and its mediated cellular adaptation but also maintains redox homeostasis to a certain extent (Sandström et al., 2006; Horie et al., 2008; Irrcher et al., 2009). For example, excessive ROS activate the MAPK pathway in plants and animals to induce autophagy, and a large number of studies have experimentally confirmed this (Jalmi and Sinha, 2015; Liu et al., 2020). When cells are exposed to ROS/NOS produced by metabolism or stress, nuclear factor (erythroid-derived-2)-like 2 (Nrf2) can maintain the dynamic balance of cells. A recent study demonstrated that Nrf2 is involved in fine-tuning the autophagic process in response to OS levels and functions in a feedback loop, binding to AMPK, which is essential for the induction of autophagy (Kapuy et al., 2018).
OS increases autophagy and apoptosis of IVD chondrocytes, but with the increase in autophagy in vivo, cartilage apoptosis is prevented, in which OS-induced autophagy of endplate chondrocytes is mTOR-dependent (Chen et al., 2017). There are many relevant studies, and autophagy occurs not only in rats but also in human degenerative IVD cells (Ye et al., 2011; Gruber et al., 2015). Research has demonstrated that autophagy occurs in degenerative IVDs in rats and humans, in which ROS are key regulators of autophagy in vitro. For example, H2O2 promotes autophagy in rat NP cells through the ERK/mTOR signalling pathway, and autophagy inhibition significantly reduces the incidence of apoptosis induced by H2O2. In addition, H2O2 leads to OS and apoptosis of chondrocytes, while Parkin and Nrf2 prevent apoptosis of intervertebral endplate chondrocytes induced by OS by inducing mitochondrial autophagy and antioxidant defence (Kang et al., 2020) (Figure 5).
FIGURE 5. Autophagy and oxidative stress in intervertebral disc degeneration. Mitochondria are not only the place where ROS is produced, but also the organelles that regulate autophagy. Oxidative stress can increase autophagy and apoptosis of intervertebral disc endplate chondrocytes, while the increase of autophagy activity can prevent endplate chondrocyte apoptosis, in which oxidative stress-induced autophagy of endplate chondrocytes is mTOR-dependent. A large amount of ROS was produced after mitochondrial injury, in which the increase of H2O2 concentration led to the activation of 5′-adenosine monophosphate-activated protein kinase (AMPK), while the combination of Nuclear factor (erythroid-derived-2)-like 2 (Nrf2) and AMPK induced autophagy through the down-regulation mechanism of mTOR. In addition, Parkin and Nrf2 can prevent apoptosis of intervertebral endplate chondrocytes induced by oxidative stress by inducing mitochondrial autophagy.
IVD compression can accelerate IDD (Desmoulin et al., 2020), and autophagy can be activated by ROS to protect the IVD and delay IDD (Ma et al., 2013). Moreover, OS induced by high glucose levels can also promote mitochondrial damage, leading to autophagy in rat cells (Park and Park, 2013). Tang et al. (2019) showed that excessive OS leads to the upregulation of autophagy, and autophagy acts as an antioxidant response in IDD.
OS has a marked impact on the IDD process. It not only regulates the vitality and function of IVD cells but also play an important role in the ECM structure of IVDs, representing a promising treatment to improve the IDD process by restoring the redox balance of IVD cells.
Studies have found that AGEs accumulate in the IVDs of diabetic mice or rats, which accelerates disc degeneration (Illien-Junger et al., 2013). However, oral pyridoxamine (an AGE inhibitor) can reduce the production and inflammation of ROS in the IVD of diabetic mice, thus slowing the progression of IDD (Fields et al., 2015). GSH is the main antioxidant in living cells. Moreover, antioxidants such as N-acetylcysteine (NAC) eliminate the catabolism of excess ROS and TNF-α in vitro (Suzuki et al., 2015). Oral administration of NAC has been shown to prevent IDD in rat degenerative models. These findings provide new ideas and new targets for continuing to perform research on relevant new mechanisms and precise prevention and treatment based on IDD pathogenesis (Suzuki et al., 2015).
In addition, Lu et al. (2021) found that under OS conditions, NP cell depletion and the pathogenesis of IVD are related to ferroportin (FPN) dysfunction, while ferritin-dependent iron homeostasis has a protective effect on IDD induced by OS. Che et al. (2020) found that p16 plays an important role in the pathogenesis of IVDD, and its deletion weakens IVD by promoting cell cycle and inhibiting SASP, cell senescence and oxidative stress. Xia et al. (2019) found that exocrine bodies derived from mesenchymal stem cells can improve IDD through antioxidant and anti-inflammatory effects.
Some active oxygen scavengers, such as pyrroloquinoline quinone (PQQ) (Zhang et al., 2013), fullerene (Anilkumar et al., 2011) and fullerol (Yang D. et al., 2014), can effectively improve OS induced by excessive ROS. PQQ is not only the primary cofactor of mitochondrial dehydrogenase but also a scavenger of ROS, playing an important role in the production of ROS in mitochondria (Zhang et al., 2013). PQQ performs an antioxidant function and can prevent some OS reactions in mitochondria (Xiong et al., 2011).
In IVD cells, PQQ inhibits the excessive production of ROS induced by H2O2 can prevent the apoptosis of rat NP cells induced by H2O2 in rat NP cells, and then protected rat NP cells from H2O2 induced apoptosis in vitro (Yang et al., 2015). Fullerenes can protect rats from lipid peroxidation in tissues (Vapa et al., 2012). Fullerol, a derivative of fullerene, can reduce the production of ROS in human NP cells and slow IDD by promoting matrix synthesis and inhibiting heterotopic ossification (Yang D. et al., 2014).
Polyphenol is a secondary metabolite produced by plants and has a strong antioxidant capacity, which mainly depends on the ability to express antioxidant genes such as catalase and inhibit the expression of oxidation-promoting genes (Akhtar and Haqqi, 2012). Resveratrol (RSV) is a polyphenol compound that exists in various plants. It has been widely used in many settings (Jiang et al., 2014; Wang et al., 2016). For example, mitochondria are important targets for RSV, and RSV can regulate ROS biosynthesis in mitochondria and regulate energy metabolism through interactions with other genes (Bastin et al., 2011; Zhou et al., 2014).
GSH can inhibit the apoptosis and matrix decomposition of human NP cells induced by H2O2 and reduce the production of ROS in human NP cells (Yang X. et al., 2014). As a precursor of GSH, oral N-acetylcysteine can inhibit OS, matrix catabolism and inflammation of IVDs in rats, delaying the development of IDD (Dimozi et al., 2015). Oestrogen is a steroid hormone primarily produced in the ovary that has been shown to have significant regulatory effects on a variety of human systems (Cauley, 2015). It can also maintain and promote the metabolism of bone matrix, promote calcium and phosphorus salts to undertake in fractures, and maintain normal fracture oestrogen and parathyroid hormone to maintain a balance. With increasing age, if oestrogen levels are low, a series of diseases, such as arteriosclerosis and osteoporosis, can occur (Cauley, 2015). Oestrogen can also regulate IVD metabolism and affect antioxidation (Jin et al., 2018). Insufficient oestrogen can also cause IVD disease and calcification, which significantly aggravate IDD (de Quadros et al., 2019). Oestrogen supplementation can repair these pathological changes and endplate calcification of IVD, promoting the expression of ECM and restoring the hydrophilicity of IVD (Liu et al., 2019).
Several growth factors can protect IVD cells from harm. Bone morphogenetic protein 7 can inhibit the apoptosis-promoting effect of H2O2 on human NP cells in vitro and helps human NP cells maintain matrix synthesis under OS (Wei et al., 2008). Insulin-like growth factor-1 improves H2O2 -induced premature ageing of human atrial fibrillation cells in vitro (Gruber et al., 2008).
The pathological changes of IVD are important to IDD Exploring the effect of OS on IDD provides important research ideas for our future research on the pathogenesis of IDD. With the progress of science and technology, an increasing number of researchers continue to deepen the understanding of the pathogenesis of IDD, although its pathogenesis is not fully understood at present. In vivo and in vitro experiments have made it clear that OS is important to the pathogenesis of IDD, which opens a new way to understand IDD. OS has a great impact on the IDD process. It not only regulates the vitality and function of IVD cells but also plays an important role in the ECM structure of IVDs. Targeting OS is a promising treatment to improve the IDD process through regaining redox balance in IVD cells. Antioxidant therapy is also considered a new and promising treatment for IDD, even though there is not sufficient evidence in vivo that antioxidants delay the establishment of IDD. In short, ageing and OS promote the degeneration of IVD, but additional basic research and clinical trials are needed to determine how to treat OS.
PW: Writing-original draft. BLZ and BFZ: Conceptualization, project administration, and writing-review and editing. TM: Data curation and methodology. LH and YZ: Formal analysis, validation, visualization, and software. All authors contributed to the article and approved the submitted version.
This work was supported by the Key Research and Development Program of Shaanxi Province (Program No. 2022SF-237).
The authors declare that the research was conducted in the absence of any commercial or financial relationships that could be construed as a potential conflict of interest.
All claims expressed in this article are solely those of the authors and do not necessarily represent those of their affiliated organizations, or those of the publisher, the editors and the reviewers. Any product that may be evaluated in this article, or claim that may be made by its manufacturer, is not guaranteed or endorsed by the publisher.
Acosta, J. C., O'Loghlen, A., Banito, A., Guijarro, M. V., Augert, A., Raguz, S., et al. (2008). Chemokine signaling via the CXCR2 receptor reinforces senescence. Cell 133 (6), 1006–1018. doi:10.1016/j.cell.2008.03.038
Akhtar, N., and Haqqi, T. M. (2012). Current nutraceuticals in the management of osteoarthritis: A review. Ther. Adv. Musculoskelet. Dis. 4 (3), 181–207. doi:10.1177/1759720x11436238
Anilkumar, P., Lu, F., Cao, L., Luo, P. G., Liu, J. H., Sahu, S., et al. (2011). Fullerenes for applications in biology and medicine. Curr. Med. Chem. 18 (14), 2045–2059. doi:10.2174/092986711795656225
Baker, D. J., Wijshake, T., Tchkonia, T., LeBrasseur, N. K., Childs, B. G., van de Sluis, B., et al. (2011). Clearance of p16Ink4a-positive senescent cells delays ageing-associated disorders. Nature 479 (7372), 232–236. doi:10.1038/nature10600
Bastin, J., Lopes-Costa, A., and Djouadi, F. (2011). Exposure to resveratrol triggers pharmacological correction of fatty acid utilization in human fatty acid oxidation-deficient fibroblasts. Hum. Mol. Genet. 20 (10), 2048–2057. doi:10.1093/hmg/ddr089
Battié, M. C., and Videman, T. (2006). Lumbar disc degeneration: Epidemiology and genetics. J. Bone Jt. Surg. Am. 88 (2), 3–9. doi:10.2106/jbjs.e.01313
Benneker, L. M., Heini, P. F., Alini, M., Anderson, S. E., and Ito, K. (2005). 2004 young investigator award winner: Vertebral endplate marrow contact channel occlusions and intervertebral disc degeneration. Spine (Phila Pa 1976) 30 (2), 167–173. doi:10.1097/01.brs.0000150833.93248.09
Boos, N., Weissbach, S., Rohrbach, H., Weiler, C., Spratt, K. F., and Nerlich, A. G. (2002). Classification of age-related changes in lumbar intervertebral discs: 2002 volvo award in basic science. Spine (Phila Pa 1976) 27 (23), 2631–2644. doi:10.1097/00007632-200212010-00002
Boxberger, J. I., Orlansky, A. S., Sen, S., and Elliott, D. M. (2009). Reduced nucleus pulposus glycosaminoglycan content alters intervertebral disc dynamic viscoelastic mechanics. J. Biomech. 42 (12), 1941–1946. doi:10.1016/j.jbiomech.2009.05.008
Cai, X. Y., Xia, Y., Yang, S. H., Liu, X. Z., Shao, Z. W., Liu, Y. L., et al. (2015). Ropivacaine- and bupivacaine-induced death of rabbit annulus fibrosus cells in vitro: Involvement of the mitochondrial apoptotic pathway. Osteoarthr. Cartil. 23 (10), 1763–1775. doi:10.1016/j.joca.2015.05.013
Campisi, J. (2005). Senescent cells, tumor suppression, and organismal aging: Good citizens, bad neighbors. Cell 120 (4), 513–522. doi:10.1016/j.cell.2005.02.003
Caramés, B., López-Armada, M. J., Cillero-Pastor, B., Lires-Dean, M., Vaamonde, C., Galdo, F., et al. (2008). Differential effects of tumor necrosis factor-alpha and interleukin-1beta on cell death in human articular chondrocytes. Osteoarthr. Cartil. 16 (6), 715–722. doi:10.1016/j.joca.2007.10.006
Cauley, J. A. (2015). Estrogen and bone health in men and women. Steroids 99, 11–15. doi:10.1016/j.steroids.2014.12.010
Che, H., Li, J., Li, Y., Ma, C., Liu, H., Qin, J., et al. (2020). p16 deficiency attenuates intervertebral disc degeneration by adjusting oxidative stress and nucleus pulposus cell cycle. Elife 9, e52570. doi:10.7554/eLife.52570
Chen, K., Lv, X., Li, W., Yu, F., Lin, J., Ma, J., et al. (2017). Autophagy is a protective response to the oxidative damage to endplate chondrocytes in intervertebral disc: Implications for the treatment of degenerative lumbar disc. Oxid. Med. Cell. Longev. 2017, 4041768. doi:10.1155/2017/4041768
Chen, Y., Wu, Y., Shi, H., Wang, J., Zheng, Z., Chen, J., et al. (2019). Melatonin ameliorates intervertebral disc degeneration via the potential mechanisms of mitophagy induction and apoptosis inhibition. J. Cell. Mol. Med. 23 (3), 2136–2148. doi:10.1111/jcmm.14125
Cheng, X., Ni, B., Zhang, F., Hu, Y., and Zhao, J. (2016). High glucose-induced oxidative stress mediates apoptosis and extracellular matrix metabolic imbalances possibly via p38 MAPK activation in rat nucleus pulposus cells. J. Diabetes Res. 2016, 3765173. doi:10.1155/2016/3765173
Chiurchiù, V., and Maccarrone, M. (2011). Chronic inflammatory disorders and their redox control: From molecular mechanisms to therapeutic opportunities. Antioxid. Redox Signal. 15 (9), 2605–2641. doi:10.1089/ars.2010.3547
Colombini, A., Lombardi, G., Corsi, M. M., and Banfi, G. (2008). Pathophysiology of the human intervertebral disc. Int. J. Biochem. Cell Biol. 40 (5), 837–842. doi:10.1016/j.biocel.2007.12.011
de Quadros, V. P., Tobar, N., Viana, L. R., Dos Santos, R. W., Kiyataka, P. H. M., and Gomes-Marcondes, M. C. C. (2019). The 17β-oestradiol treatment minimizes the adverse effects of protein restriction on bone parameters in ovariectomized Wistar rats: Relevance to osteoporosis and the menopause. Bone Jt. Res. 8 (12), 573–581. doi:10.1302/2046-3758.812.bjr-2018-0259.r2
DeGroot, J., Verzijl, N., Bank, R. A., Lafeber, F. P., Bijlsma, J. W., and TeKoppele, J. M. (1999). Age-related decrease in proteoglycan synthesis of human articular chondrocytes: The role of nonenzymatic glycation. Arthritis Rheum. 42 (5), 1003–1009. doi:10.1002/1529-0131(199905)42:5<1003::AID-ANR20>3.0.CO;2-K
Desmoulin, G. T., Pradhan, V., and Milner, T. E. (2020). Mechanical aspects of intervertebral disc injury and implications on biomechanics. Spine (Phila Pa 1976) 45 (8), E457–E464. doi:10.1097/brs.0000000000003291
Dimozi, A., Mavrogonatou, E., Sklirou, A., Kletsas, D., and Sklirou, A. (2015). Oxidative stress inhibits the proliferation, induces premature senescence and promotes a catabolic phenotype in human nucleus pulposus intervertebral disc cells. Eur. Cell. Mat. 30, 89–102. discussion 103. doi:10.22203/ecm.v030a07
Ding, F., Shao, Z. W., and Xiong, L. M. (2013). Cell death in intervertebral disc degeneration. Apoptosis 18 (7), 777–785. doi:10.1007/s10495-013-0839-1
Ding, F., Shao, Z. W., Yang, S. H., Wu, Q., Gao, F., and Xiong, L. M. (2012). Role of mitochondrial pathway in compression-induced apoptosis of nucleus pulposus cells. Apoptosis 17 (6), 579–590. doi:10.1007/s10495-012-0708-3
Ďuračková, Z. (2010). Some current insights into oxidative stress. Physiol. Res. 59 (4), 459–469. doi:10.33549/physiolres.931844
Feng, C., Yang, M., Lan, M., Liu, C., Zhang, Y., Huang, B., et al. (2017). ROS: Crucial intermediators in the pathogenesis of intervertebral disc degeneration. Oxid. Med. Cell. Longev. 2017, 5601593. doi:10.1155/2017/5601593
Fields, A. J., Berg-Johansen, B., Metz, L. N., Miller, S., La, B., Liebenberg, E. C., et al. (2015). Alterations in intervertebral disc composition, matrix homeostasis and biomechanical behavior in the UCD-T2DM rat model of type 2 diabetes. J. Orthop. Res. 33 (5), 738–746. doi:10.1002/jor.22807
Filomeni, G., De Zio, D., and Cecconi, F. (2015). Oxidative stress and autophagy: The clash between damage and metabolic needs. Cell Death Differ. 22 (3), 377–388. doi:10.1038/cdd.2014.150
Gille, L., and Nohl, H. (2001). The ubiquinol/bc1 redox couple regulates mitochondrial oxygen radical formation. Arch. Biochem. Biophys. 388 (1), 34–38. doi:10.1006/abbi.2000.2257
Gorrini, C., Harris, I. S., and Mak, T. W. (2013). Modulation of oxidative stress as an anticancer strategy. Nat. Rev. Drug Discov. 12 (12), 931–947. doi:10.1038/nrd4002
Gruber, H. E., Hoelscher, G. L., Ingram, J. A., Bethea, S., and Hanley, E. N. (2008). IGF-1 rescues human intervertebral annulus cells from in vitro stress-induced premature senescence. Growth factors. 26 (4), 220–225. doi:10.1080/08977190802273814
Gruber, H. E., Hoelscher, G. L., Ingram, J. A., Bethea, S., and Hanley, E. N. (2015). Autophagy in the degenerating human intervertebral disc: In vivo molecular and morphological evidence, and induction of autophagy in cultured annulus cells exposed to proinflammatory cytokines-implications for disc degeneration. Spine (Phila Pa 1976) 40 (11), 773–782. doi:10.1097/brs.0000000000000865
Gruber, H. E., Watts, J. A., Hoelscher, G. L., Bethea, S. F., Ingram, J. A., Zinchenko, N. S., et al. (2011). Mitochondrial gene expression in the human annulus: In vivo data from annulus cells and selectively harvested senescent annulus cells. Spine J. 11 (8), 782–791. doi:10.1016/j.spinee.2011.06.012
Gruber, H. E., Watts, J. A., Riley, F. E., Fulkerson, M. B., Norton, H. J., and Hanley, E. N. (2013). Mitochondrial bioenergetics, mass, and morphology are altered in cells of the degenerating human annulus. J. Orthop. Res. 31 (8), 1270–1275. doi:10.1002/jor.22361
Harman, D. (1956). Aging: A theory based on free radical and radiation chemistry. J. Gerontol. 11 (3), 298–300. doi:10.1093/geronj/11.3.298
Hogg, N., Darley-Usmar, V. M., Wilson, M. T., and Moncada, S. (1992). Production of hydroxyl radicals from the simultaneous generation of superoxide and nitric oxide. Biochem. J. 281 (2), 419–424. doi:10.1042/bj2810419
Horie, T., Ono, K., Nagao, K., Nishi, H., Kinoshita, M., Kawamura, T., et al. (2008). Oxidative stress induces GLUT4 translocation by activation of PI3-K/Akt and dual AMPK kinase in cardiac myocytes. J. Cell. Physiol. 215 (3), 733–742. doi:10.1002/jcp.21353
Horner, H. A., and Urban, J. P. (2001). 2001 Volvo Award Winner in Basic Science Studies: Effect of nutrient supply on the viability of cells from the nucleus pulposus of the intervertebral disc. Spine (Phila Pa 1976) 26 (23), 2543–2549. doi:10.1097/00007632-200112010-00006
Hou, G., Lu, H., Chen, M., Yao, H., and Zhao, H. (2014). Oxidative stress participates in age-related changes in rat lumbar intervertebral discs. Arch. Gerontol. Geriatr. 59 (3), 665–669. doi:10.1016/j.archger.2014.07.002
Hwang, P. Y., Chen, J., Jing, L., Hoffman, B. D., and Setton, L. A. (2014). The role of extracellular matrix elasticity and composition in regulating the nucleus pulposus cell phenotype in the intervertebral disc: A narrative review. J. Biomech. Eng. 136 (2), 021010. doi:10.1115/1.4026360
Illien-Junger, S., Grosjean, F., Laudier, D. M., Vlassara, H., Striker, G. E., and Iatridis, J. C. (2013). Combined anti-inflammatory and anti-AGE drug treatments have a protective effect on intervertebral discs in mice with diabetes. PLoS One 8 (5), e64302. doi:10.1371/journal.pone.0064302
Irrcher, I., Ljubicic, V., and Hood, D. A. (2009). Interactions between ROS and AMP kinase activity in the regulation of PGC-1alpha transcription in skeletal muscle cells. Am. J. Physiol. Cell Physiol. 296 (1), C116–C123. doi:10.1152/ajpcell.00267.2007
Jalmi, S. K., and Sinha, A. K. (2015). ROS mediated MAPK signaling in abiotic and biotic stress- striking similarities and differences. Front. Plant Sci. 6, 769. doi:10.3389/fpls.2015.00769
Jiang, W., Zhang, X., Hao, J., Shen, J., Fang, J., Dong, W., et al. (2014). SIRT1 protects against apoptosis by promoting autophagy in degenerative human disc nucleus pulposus cells. Sci. Rep. 4, 7456. doi:10.1038/srep07456
Jin, L. Y., Lv, Z. D., Wang, K., Qian, L., Song, X. X., Li, X. F., et al. (2018). Estradiol alleviates intervertebral disc degeneration through modulating the antioxidant enzymes and inhibiting autophagy in the model of menopause rats. Oxid. Med. Cell. Longev. 2018, 7890291. doi:10.1155/2018/7890291
Jones, D. P. (2008). Radical-free biology of oxidative stress. Am. J. Physiol. Cell Physiol. 295 (4), C849–C868. doi:10.1152/ajpcell.00283.2008
Kang, L., Liu, S., Li, J., Tian, Y., Xue, Y., and Liu, X. (2020). Parkin and Nrf2 prevent oxidative stress-induced apoptosis in intervertebral endplate chondrocytes via inducing mitophagy and anti-oxidant defenses. Life Sci. 243, 117244. doi:10.1016/j.lfs.2019.117244
Kapuy, O., Papp, D., Vellai, T., Bánhegyi, G., and Korcsmáros, T. (2018). Systems-level feedbacks of NRF2 controlling autophagy upon oxidative stress response. Antioxidants (Basel) 7 (3), E39. doi:10.3390/antiox7030039
Kepler, C. K., Ponnappan, R. K., Tannoury, C. A., Risbud, M. V., and Anderson, D. G. (2013). The molecular basis of intervertebral disc degeneration. Spine J. 13 (3), 318–330. doi:10.1016/j.spinee.2012.12.003
Khan, A. N., Jacobsen, H. E., Khan, J., Filippi, C. G., Levine, M., Lehman, R. A., et al. (2017). Inflammatory biomarkers of low back pain and disc degeneration: A review. Ann. N. Y. Acad. Sci. 1410 (1), 68–84. doi:10.1111/nyas.13551
Kim, J., Xu, M., Xo, R., Mates, A., Wilson, G. L., Pearsall, A. W. t., et al. (2010). Mitochondrial DNA damage is involved in apoptosis caused by pro-inflammatory cytokines in human OA chondrocytes. Osteoarthr. Cartil. 18 (3), 424–432. doi:10.1016/j.joca.2009.09.008
Kim, K. A., and Yim, J. E. (2015). Antioxidative activity of onion peel extract in obese women: A randomized, double-blind, placebo controlled study. J. Cancer Prev. 20 (3), 202–207. doi:10.15430/jcp.2015.20.3.202
Kim, K. H., and Lee, M. S. (2014). Autophagy-a key player in cellular and body metabolism. Nat. Rev. Endocrinol. 10 (6), 322–337. doi:10.1038/nrendo.2014.35
Kim, K. W., Chung, H. N., Ha, K. Y., Lee, J. S., and Kim, Y. Y. (2009). Senescence mechanisms of nucleus pulposus chondrocytes in human intervertebral discs. Spine J. 9 (8), 658–666. doi:10.1016/j.spinee.2009.04.018
Knezevic, N. N., Candido, K. D., Vlaeyen, J. W. S., Van Zundert, J., and Cohen, S. P. (2021). Low back pain. Lancet 398 (10294), 78–92. doi:10.1016/s0140-6736(21)00733-9
Kraychete, D. C., Sakata, R. K., Issy, A. M., Bacellar, O., Santos-Jesus, R., and Carvalho, E. M. (2010). Serum cytokine levels in patients with chronic low back pain due to herniated disc: Analytical cross-sectional study. Sao Paulo Med. J. 128 (5), 259–262. doi:10.1590/s1516-31802010000500003
Krupkova, O., Handa, J., Hlavna, M., Klasen, J., Ospelt, C., Ferguson, S. J., et al. (2016). The natural polyphenol epigallocatechin gallate protects intervertebral disc cells from oxidative stress. Oxid. Med. Cell. Longev. 2016, 7031397. doi:10.1155/2016/7031397
Le Maitre, C. L., Freemont, A. J., and Hoyland, J. A. (2007a). Accelerated cellular senescence in degenerate intervertebral discs: A possible role in the pathogenesis of intervertebral disc degeneration. Arthritis Res. Ther. 9 (3), R45. doi:10.1186/ar2198
Le Maitre, C. L., Freemont, A. J., and Hoyland, J. A. (2005). The role of interleukin-1 in the pathogenesis of human intervertebral disc degeneration. Arthritis Res. Ther. 7 (4), R732–R745. doi:10.1186/ar1732
Le Maitre, C. L., Pockert, A., Buttle, D. J., Freemont, A. J., and Hoyland, J. A. (2007b). Matrix synthesis and degradation in human intervertebral disc degeneration. Biochem. Soc. Trans. 35 (4), 652–655. doi:10.1042/bst0350652
Lei, Y., Wang, K., Deng, L., Chen, Y., Nice, E. C., and Huang, C. (2015). Redox regulation of inflammation: Old elements, a new story. Med. Res. Rev. 35 (2), 306–340. doi:10.1002/med.21330
Lin, J., Zhuge, J., Zheng, X., Wu, Y., Zhang, Z., Xu, T., et al. (2020). Urolithin A-induced mitophagy suppresses apoptosis and attenuates intervertebral disc degeneration via the AMPK signaling pathway. Free Radic. Biol. Med. 150, 109–119. doi:10.1016/j.freeradbiomed.2020.02.024
Liu, Q., Wang, X., Hua, Y., Kong, G., Wu, X., Huang, Z., et al. (2019). Estrogen deficiency exacerbates intervertebral disc degeneration induced by spinal instability in rats. Spine (Phila Pa 1976) 44 (9), E510–E519. doi:10.1097/brs.0000000000002904
Liu, Z., Huang, Y., Jiao, Y., Chen, Q., Wu, D., Yu, P., et al. (2020). Polystyrene nanoplastic induces ROS production and affects the MAPK-HIF-1/NFkB-mediated antioxidant system in Daphnia pulex. Aquat. Toxicol. 220, 105420. doi:10.1016/j.aquatox.2020.105420
López-Armada, M. J., Caramés, B., Martín, M. A., Cillero-Pastor, B., Lires-Dean, M., Fuentes-Boquete, I., et al. (2006). Mitochondrial activity is modulated by TNFalpha and IL-1beta in normal human chondrocyte cells. Osteoarthr. Cartil. 14 (10), 1011–1022. doi:10.1016/j.joca.2006.03.008
Loukili, N., Rosenblatt-Velin, N., Rolli, J., Levrand, S., Feihl, F., Waeber, B., et al. (2010). Oxidants positively or negatively regulate nuclear factor kappaB in a context-dependent manner. J. Biol. Chem. 285 (21), 15746–15752. doi:10.1074/jbc.M110.103259
Lu, S., Song, Y., Luo, R., Li, S., Li, G., Wang, K., et al. (2021). Ferroportin-dependent iron homeostasis protects against oxidative stress-induced nucleus pulposus cell ferroptosis and ameliorates intervertebral disc degeneration in vivo. Oxid. Med. Cell. Longev. 2021, 6670497. doi:10.1155/2021/6670497
Ma, K. G., Shao, Z. W., Yang, S. H., Wang, J., Wang, B. C., Xiong, L. M., et al. (2013). Autophagy is activated in compression-induced cell degeneration and is mediated by reactive oxygen species in nucleus pulposus cells exposed to compression. Osteoarthr. Cartil. 21 (12), 2030–2038. doi:10.1016/j.joca.2013.10.002
McCubrey, J. A., Lahair, M. M., and Franklin, R. A. (2006). Reactive oxygen species-induced activation of the MAP kinase signaling pathways. Antioxid. Redox Signal. 8 (9-10), 1775–1789. doi:10.1089/ars.2006.8.1775
Miki, H., and Funato, Y. (2012). Regulation of intracellular signalling through cysteine oxidation by reactive oxygen species. J. Biochem. 151 (3), 255–261. doi:10.1093/jb/mvs006
Muñoz-Espín, D., and Serrano, M. (2014). Cellular senescence: From physiology to pathology. Nat. Rev. Mol. Cell Biol. 15 (7), 482–496. doi:10.1038/nrm3823
Nerlich, A. G., Schleicher, E. D., and Boos, N. (1997). 1997 Volvo Award winner in basic science studies. Immunohistologic markers for age-related changes of human lumbar intervertebral discs. Spine (Phila Pa 1976) 22 (24), 2781–2795. doi:10.1097/00007632-199712150-00001
Park, E. Y., and Park, J. B. (2013). High glucose-induced oxidative stress promotes autophagy through mitochondrial damage in rat notochordal cells. Int. Orthop. 37 (12), 2507–2514. doi:10.1007/s00264-013-2037-8
Park, M. S., Lee, H. M., Hahn, S. B., Moon, S. H., Kim, Y. T., Lee, C. S., et al. (2007). The association of the activation-inducible tumor necrosis factor receptor and ligand with lumbar disc herniation. Yonsei Med. J. 48 (5), 839–846. doi:10.3349/ymj.2007.48.5.839
Poveda, L., Hottiger, M., Boos, N., and Wuertz, K. (2009). Peroxynitrite induces gene expression in intervertebral disc cells. Spine (Phila Pa 1976) 34 (11), 1127–1133. doi:10.1097/BRS.0b013e31819f2330
Risbud, M. V., and Shapiro, I. M. (2014). Role of cytokines in intervertebral disc degeneration: Pain and disc content. Nat. Rev. Rheumatol. 10 (1), 44–56. doi:10.1038/nrrheum.2013.160
Rodier, F., Coppé, J. P., Patil, C. K., Hoeijmakers, W. A., Muñoz, D. P., Raza, S. R., et al. (2009). Persistent DNA damage signalling triggers senescence-associated inflammatory cytokine secretion. Nat. Cell Biol. 11 (8), 973–979. doi:10.1038/ncb1909
Roughley, P. J. (2004). Biology of intervertebral disc aging and degeneration: Involvement of the extracellular matrix. Spine (Phila Pa 1976) 29 (23), 2691–2699. doi:10.1097/01.brs.0000146101.53784.b1
Sandström, M. E., Zhang, S. J., Bruton, J., Silva, J. P., Reid, M. B., Westerblad, H., et al. (2006). Role of reactive oxygen species in contraction-mediated glucose transport in mouse skeletal muscle. J. Physiol. 575 (1), 251–262. doi:10.1113/jphysiol.2006.110601
Scharf, B., Clement, C. C., Yodmuang, S., Urbanska, A. M., Suadicani, S. O., Aphkhazava, D., et al. (2013). Age-related carbonylation of fibrocartilage structural proteins drives tissue degenerative modification. Chem. Biol. 20 (7), 922–934. doi:10.1016/j.chembiol.2013.06.006
Schleicher, E. D., Wagner, E., and Nerlich, A. G. (1997). Increased accumulation of the glycoxidation product N(epsilon)-(carboxymethyl)lysine in human tissues in diabetes and aging. J. Clin. Invest. 99 (3), 457–468. doi:10.1172/jci119180
Sobajima, S., Shimer, A. L., Chadderdon, R. C., Kompel, J. F., Kim, J. S., Gilbertson, L. G., et al. (2005). Quantitative analysis of gene expression in a rabbit model of intervertebral disc degeneration by real-time polymerase chain reaction. Spine J. 5 (1), 14–23. doi:10.1016/j.spinee.2004.05.251
Stokes, I. A., and Iatridis, J. C. (2004). Mechanical conditions that accelerate intervertebral disc degeneration: Overload versus immobilization. Spine (Phila Pa 1976) 29 (23), 2724–2732. doi:10.1097/01.brs.0000146049.52152.da
Suzuki, S., Fujita, N., Hosogane, N., Watanabe, K., Ishii, K., Toyama, Y., et al. (2015). Excessive reactive oxygen species are therapeutic targets for intervertebral disc degeneration. Arthritis Res. Ther. 17, 316. doi:10.1186/s13075-015-0834-8
Takatalo, J., Karppinen, J., Niinimäki, J., Taimela, S., Näyhä, S., Mutanen, P., et al. (2011). Does lumbar disc degeneration on magnetic resonance imaging associate with low back symptom severity in young Finnish adults? Spine 36 (25), 2180–2189. doi:10.1097/BRS.0b013e3182077122
Tang, P., Gu, J. M., Xie, Z. A., Gu, Y., Jie, Z. W., Huang, K. M., et al. (2018). Honokiol alleviates the degeneration of intervertebral disc via suppressing the activation of TXNIP-NLRP3 inflammasome signal pathway. Free Radic. Biol. Med. 120, 368–379. doi:10.1016/j.freeradbiomed.2018.04.008
Tang, Z., Hu, B., Zang, F., Wang, J., Zhang, X., and Chen, H. (2019). Nrf2 drives oxidative stress-induced autophagy in nucleus pulposus cells via a Keap1/Nrf2/p62 feedback loop to protect intervertebral disc from degeneration. Cell Death Dis. 10 (7), 510. doi:10.1038/s41419-019-1701-3
Trachootham, D., Lu, W., Ogasawara, M. A., Nilsa, R. D., and Huang, P. (2008). Redox regulation of cell survival. Antioxid. Redox Signal. 10 (8), 1343–1374. doi:10.1089/ars.2007.1957
Ugalde, A. P., Ordóñez, G. R., Quirós, P. M., Puente, X. S., and López-Otín, C. (2010). Metalloproteases and the degradome. Methods Mol. Biol. 622, 3–29. doi:10.1007/978-1-60327-299-5_1
van Deursen, J. M. (2014). The role of senescent cells in ageing. Nature 509 (7501), 439–446. doi:10.1038/nature13193
Vapa, I., Torres, V. M., Djordjevic, A., Vasovic, V., Srdjenovic, B., Simic, V. D., et al. (2012). Effect of fullerenol C(60)(OH) (24) on lipid peroxidation of kidneys, testes and lungs in rats treated with doxorubicine. Eur. J. Drug Metab. Pharmacokinet. 37 (4), 301–307. doi:10.1007/s13318-012-0092-y
Vistoli, G., De Maddis, D., Cipak, A., Zarkovic, N., Carini, M., and Aldini, G. (2013). Advanced glycoxidation and lipoxidation end products (AGEs and ALEs): An overview of their mechanisms of formation. Free Radic. Res. 47 (1), 3–27. doi:10.3109/10715762.2013.815348
Vo, N., Niedernhofer, L. J., Nasto, L. A., Jacobs, L., Robbins, P. D., Kang, J., et al. (2013). An overview of underlying causes and animal models for the study of age-related degenerative disorders of the spine and synovial joints. J. Orthop. Res. 31 (6), 831–837. doi:10.1002/jor.22204
Vos, T., Flaxman, A. D., Naghavi, M., Lozano, R., Michaud, C., Ezzati, M., et al. (2012). Years lived with disability (YLDs) for 1160 sequelae of 289 diseases and injuries 1990-2010: A systematic analysis for the global burden of disease study 2010. Lancet 380 (9859), 2163–2196. doi:10.1016/s0140-6736(12)61729-2
Wang, B., Ke, W., Wang, K., Li, G., Ma, L., Lu, S., et al. (2021). Mechanosensitive ion channel Piezo1 activated by matrix stiffness regulates oxidative stress-induced senescence and apoptosis in human intervertebral disc degeneration. Oxid. Med. Cell. Longev. 2021, 8884922. doi:10.1155/2021/8884922
Wang, S., Liu, Z., Wang, L., and Zhang, X. (2009). NF-kappaB signaling pathway, inflammation and colorectal cancer. Cell. Mol. Immunol. 6 (5), 327–334. doi:10.1038/cmi.2009.43
Wang, Y., Shen, J., Chen, Y., Liu, H., Zhou, H., Bai, Z., et al. (2018). PINK1 protects against oxidative stress induced senescence of human nucleus pulposus cells via regulating mitophagy. Biochem. Biophys. Res. Commun. 504 (2), 406–414. doi:10.1016/j.bbrc.2018.06.031
Wang, Y., Wu, W., Yao, C., Lou, J., Chen, R., Jin, L., et al. (2016). Elevated tissue Cr levels, increased plasma oxidative markers, and global hypomethylation of blood DNA in male Sprague-Dawley rats exposed to potassium dichromate in drinking water. Environ. Toxicol. 31 (9), 1080–1090. doi:10.1002/tox.22117
Wei, A., Brisby, H., Chung, S. A., and Diwan, A. D. (2008). Bone morphogenetic protein-7 protects human intervertebral disc cells in vitro from apoptosis. Spine J. 8 (3), 466–474. doi:10.1016/j.spinee.2007.04.021
Xia, C., Zeng, Z., Fang, B., Tao, M., Gu, C., Zheng, L., et al. (2019). Mesenchymal stem cell-derived exosomes ameliorate intervertebral disc degeneration via anti-oxidant and anti-inflammatory effects. Free Radic. Biol. Med. 143, 1–15. doi:10.1016/j.freeradbiomed.2019.07.026
Xiong, X. H., Zhao, Y., Ge, X., Yuan, S. J., Wang, J. H., Zhi, J. J., et al. (2011). Production and radioprotective effects of pyrroloquinoline quinone. Int. J. Mol. Sci. 12 (12), 8913–8923. doi:10.3390/ijms12128913
Xu, W. N., Zheng, H. L., Yang, R. Z., Liu, T., Yu, W., Zheng, X. F., et al. (2019). Mitochondrial NDUFA4L2 attenuates the apoptosis of nucleus pulposus cells induced by oxidative stress via the inhibition of mitophagy. Exp. Mol. Med. 51 (11), 1–16. doi:10.1038/s12276-019-0331-2
Yang, D., Wang, D., Shimer, A., Shen, F. H., Li, X., and Yang, X. (2014). Glutathione protects human nucleus pulposus cells from cell apoptosis and inhibition of matrix synthesis. Connect. Tissue Res. 55 (2), 132–139. doi:10.3109/03008207.2013.876421
Yang, L., Rong, Z., Zeng, M., Cao, Y., Gong, X., Lin, L., et al. (2015). Pyrroloquinoline quinone protects nucleus pulposus cells from hydrogen peroxide-induced apoptosis by inhibiting the mitochondria-mediated pathway. Eur. Spine J. 24 (8), 1702–1710. doi:10.1007/s00586-014-3630-2
Yang, R. Z., Xu, W. N., Zheng, H. L., Zheng, X. F., Li, B., Jiang, L. S., et al. (2021). Involvement of oxidative stress-induced annulus fibrosus cell and nucleus pulposus cell ferroptosis in intervertebral disc degeneration pathogenesis. J. Cell. Physiol. 236 (4), 2725–2739. doi:10.1002/jcp.30039
Yang, X., Jin, L., Yao, L., Shen, F. H., Shimer, A. L., and Li, X. (2014). Antioxidative nanofullerol prevents intervertebral disk degeneration. Int. J. Nanomedicine 9, 2419–2430. doi:10.2147/ijn.s60853
Ye, W., Xu, K., Huang, D., Liang, A., Peng, Y., Zhu, W., et al. (2011). Age-related increases of macroautophagy and chaperone-mediated autophagy in rat nucleus pulposus. Connect. Tissue Res. 52 (6), 472–478. doi:10.3109/03008207.2011.564336
Žerovnik, E., Ventura, S., and Kopitar-Jerala, N. (2020). Special issue: "Inflammation, oxidative stress and protein aggregation; any links? Cells 9 (11), E2461. doi:10.3390/cells9112461
Zhang, Q., Ding, M., Cao, Z., Zhang, J., Ding, F., and Ke, K. (2013). Pyrroloquinoline quinine protects rat brain cortex against acute glutamate-induced neurotoxicity. Neurochem. Res. 38 (8), 1661–1671. doi:10.1007/s11064-013-1068-2
Zhang, Z., Xu, T., Chen, J., Shao, Z., Wang, K., Yan, Y., et al. (2018). Parkin-mediated mitophagy as a potential therapeutic target for intervertebral disc degeneration. Cell Death Dis. 9 (10), 980. doi:10.1038/s41419-018-1024-9
Zhao, C. Q., Wang, L. M., Jiang, L. S., and Dai, L. Y. (2007). The cell biology of intervertebral disc aging and degeneration. Ageing Res. Rev. 6 (3), 247–261. doi:10.1016/j.arr.2007.08.001
Zhou, N., Lin, X., Dong, W., Huang, W., Jiang, W., Lin, L., et al. (2016). SIRT1 alleviates senescence of degenerative human intervertebral disc cartilage endo-plate cells via the p53/p21 pathway. Sci. Rep. 6, 22628. doi:10.1038/srep22628
Keywords: ageing, oxidative stress, intervertebral disc degeneration, intervertebral disc, reactive oxygen species
Citation: Wen P, Zheng B, Zhang B, Ma T, Hao L and Zhang Y (2022) The role of ageing and oxidative stress in intervertebral disc degeneration. Front. Mol. Biosci. 9:1052878. doi: 10.3389/fmolb.2022.1052878
Received: 24 September 2022; Accepted: 25 October 2022;
Published: 07 November 2022.
Edited by:
Jialiang Lin, Peking University Third Hospital, ChinaCopyright © 2022 Wen, Zheng, Zhang, Ma, Hao and Zhang. This is an open-access article distributed under the terms of the Creative Commons Attribution License (CC BY). The use, distribution or reproduction in other forums is permitted, provided the original author(s) and the copyright owner(s) are credited and that the original publication in this journal is cited, in accordance with accepted academic practice. No use, distribution or reproduction is permitted which does not comply with these terms.
*Correspondence: Linjie Hao, aGFvbGluamllMDExOEAxMjYuY29t; Yumin Zhang, enltMjY2NkAxNjMuY29t
†These authors have contributed equally to this work
Disclaimer: All claims expressed in this article are solely those of the authors and do not necessarily represent those of their affiliated organizations, or those of the publisher, the editors and the reviewers. Any product that may be evaluated in this article or claim that may be made by its manufacturer is not guaranteed or endorsed by the publisher.
Research integrity at Frontiers
Learn more about the work of our research integrity team to safeguard the quality of each article we publish.