- 1Department of Medical Biochemistry and Molecular Biology, Faculty of Medicine, Fayoum University, Fayoum, Egypt
- 2Department of Paediatrics, Faculty of Medicine, Fayoum University, Fayoum, Egypt
- 3Department of Physiology, Faculty of Medicine, Zagazig University, Zagazig, Egypt
- 4Department of Medical Physiology, Faculty of Medicine, Fayoum University, Fayoum, Egypt
- 5Department of Clinical and Chemical Pathology, Faculty of Medicine, Cairo University, Cairo, Egypt
- 6Department of Medical Microbiology and Immunology, Faculty of Medicine, Fayoum University, Fayoum, Egypt
Background/aim: IFNG-AS1 is a long noncoding RNA that works as an enhancer for the Interferon-gamma (IFN-γ) transcript. GAS5 (growth arrest-specific 5) is a lncRNA that is associated with glucocorticoid resistance. Aberrant expressions of IFNG-AS1 and GAS5 are directly linked to numerous autoimmune disorders but their levels in childhood ITP are still obscure. This study aims to elucidate expressions of target lncRNAs in childhood ITP and their association with pathophysiology and clinical features of the disease as well as their association with types and treatment responses.
Method: The fold changes of target lncRNAs in blood samples from children with ITP and healthy controls were analyzed using quantitative real-time PCR (qRT-PCR).
Results: There were overexpressed lncRNAs IFNG-AS1 and GAS5 in serum of childhood ITP patients [(median (IQR) = 3.08 (0.2–22.39) and 4.19 (0.9–16.91) respectively, Also, significant higher IFNG-AS1 and GAS5 (p < 0.05) were present in persistent ITP (3–12 months) [ median (IQR) = 4.58 (0.31–22.39) and 3.77 (0.87–12.36) respectively] or chronic ITP (>12 months) [ median (IQR) = 5.6 (0.25–12.59) and 5.61 (1.15–16.91) respectively] when compared to newly diagnosed <3 months patients [IFNG-AS1 median (IQR) = 1.21 (0.2–8.95), and GAS5 median (IQR) = 1.07 (0.09–3.55)]. Also, significant higher lncRNAs IFNG-AS1 and GAS5 were present in patients with partial response to treatment [IFNG-AS1 median (IQR) = 4.15 (0.94–19.25), and GAS5 (median (IQR) = 4.25 (0.81–16.91)] or non-response [IFNG-AS1 median (IQR) = 4.19 (1.25–22.39) and GAS5 median (IQR) = 5.11 (2.34–15.27)] when compared to patients who completely responded to treatment (IFNG-AS1 median (IQR) = 2.09 (0.2–14.58) and GAS5 (median (IQR) = 2.51 (0.09–10.33). In addition, following therapy, the expressions of IFNG-AS1 and GAS5 are significantly negatively correlated with platelet count.
Conclusion: Findings suggest that lncRNAs IFNG-AS1 and GAS5 are novel diagnostic and prognostic genetic markers for childhood ITP that can aid in a precise prediction of the disease’s progress at the time of diagnosis and could be a useful tool for treatment planning.
Introduction
A vast number of non-coding transcripts were discovered during human genome sequencing, they were formerly believed to be non-functional, however recently, scientists discovered that they have regulatory functions on gene expression involving transcriptional, posttranscriptional, and translational levels. Long noncoding RNAs (lncRNAs) are one type of them which defines as RNA transcripts with more than 200 nucleotides that are not translated into protein (Palazzo and Lee, 2015). As more evidence accumulates, lncRNAs appear to have an essential function in immune control, suggesting that aberrant lncRNAs expressions may have played a part in the triggering of autoimmunity comes in a variety of forms (Gao et al., 2018).
IFNG-AS1 (Ifng antisense RNA 1), also known as Tmevpg1 (Theiler’s murine encephalomyelitis virus persistence candidate gene 1), or NeST, (nettoie Salmonella pas Theiler’s), is affiliated as lncRNA transcript which gene is found on the DNA strand opposite the interferon-gamma (IFN- γ) coding gene so hence its name. The coexpression of lncRNA, IFNG-AS1 with T-bet is related to the active transcription of IFN- γ in Th1 cells. Th1 cells are an inflammatory fraction of CD4+ T cells that primarily generate interferon-gamma (IFN-γ), an inflammatory cytokine that protects against intracellular pathogens, and is related to delayed-type hypersensitivity, and autoimmune diseases including ITP (Li et al., 2016), (Collier et al., 2012a).
GAS5 (growth arrest-specific 5) is a lncRNA that was first discovered as a cancer tumor suppressor gene. GAS5 expression has been found to be abnormal in numerous malignancies, and this gene has been linked to cell cycle inhibition and apoptosis (Ji et al., 2019). Recently, scientists discovered that GAS5 dysregulation has been implicated in the development of autoimmunity states; The most effective medications for treating autoimmune illnesses are glucocorticoids (GC). GAS5 interacts with the DNA binding domain of glucocorticoid receptors (GRs), and preventing GRs from connecting with DNA, preventing glucocorticoid activity which is a powerful immunosuppressive and hence contributes to the development of autoimmune disorders (Moharamoghli et al., 2019).
Immune thrombocytopenic purpura (ITP) is a blood disorder characterized by a decreased platelet count below 100×109/L with normal hemoglobin and leucocytes, when there are no additional causes or conditions that could be linked to thrombocytopenia, the condition is called idiopathic or primary ITP. The fundamental immunologic abnormality in ITP is thought to be humoral and cell-mediated antiplatelet antibodies thus leading to enhanced platelet destruction and often inappropriate platelet manufacture in the bone marrow. These phenotypic changes reflected a change in gene regulation (Makis et al., 2017), (Ayoub et al., 2020).
Childhood Immune Thrombocytopenia (ITP) is one of the most frequent autoimmune hemorrhagic diseases affecting 5 to 10 children out of every 100,000. Twenty to thirty percent of cases were shown to be prone to chronicity with increased susceptibility to bleeding, infection, and blood malignancy than healthy children (Beshir et al., 2021) (Li et al., 2020). Previous studies demonstrated that when compared to children who received therapeutic regimens, the no therapy group had a significantly higher recurrence and more serious complications (Makis et al., 2017) (Kühne et al., 2011). The lncRNAs involved in immune mechanisms that play role in the initiation of the disease are the targets of therapy when indicated, Aberrant IFNG-AS1 and GAS5 expressions are linked to numerous autoimmune disorders suggesting the possible role of these genes in the pathogenesis of autoimmunity state (Li et al., 2018).
There are two types of ITP; Adult ITP and childhood ITP (Kühne et al., 2011), although, two separate studies have reported that levels of IFNG-AS1and GAS5 in peripheral blood of adult ITP patients (Li et al., 2016), (Li et al., 2020), the expressions of IFNG-AS1 and GAS5 in childhood ITP are still unknown. Thus, we designated this study to investigate the expressions of lncRNAs IFNG-AS1 and GAS5 in childhood ITP and their relationship to clinical characteristics of the disease, as well as types and treatments.
Materials and methods
Materials
Materials used to execute this work include; one- MiRNeasy Serum/Plasma extraction kit (Qiagen, Valencia, CA, USA) was used for total RNA extraction from serum. Two- The RT2 First Strand Kit (Qiagen, Valencia, CA, USA) was used for reverse transcription to produce cDNA. Three- The RT2 SYBR Green PCR kit (Qiagen, Maryland, USA) was used to perform qRT-PCR along with specific primers supplied by Qiagen, (Valencia, CA, USA); for IFNG-AS1 (Catalog no; 330701LPH20079A, Accession no, ENST00000536914.0) and GAS5 (Catalog no; 330701LPH11340A, Accession no, NR_002578.2) and we used GAPDH primer (Catalog no: 330701 LPH31725A, Accession no: ENST00000496049.0) to standardize the expression pattern and quantify the target long non-coding RNAs.
Patients and controls
This case-control study was comprised of eighty-eight children (1–13 years old) who suffered from primary ITP and eighty-eight healthy age and sex-matched children with no history of any disease. Cases were gathered from the Pediatric Departments’ inpatient and outpatient clinics at Fayoum University Hospital in Egypt over a period of 6 months between Jan 2022 and June 2022.
Diagnostic criteria
A detailed history was taken including age, sex, complaint at presentation, disease duration, presence of bleeding manifestations, history of splenectomy, presence of preceding febrile illness, recent vaccination, helicobacter pylori infection history, HCV and/or HBV viral infection. Causes of secondary ITP such as autoimmune rheumatological diseases and the previous treatment regimen. A systematic clinical examination is performed, with special attention paid to clinical symptoms of bleeding such as bruises or purplish spots on the skin, mucous membranes, or gums, and any concomitant splenomegaly. Laboratory tests including complete blood count (CBC) and bone marrow examination to exclude other diseases resulting in thrombocytopenia were done. The definite diagnosis was established as primary idiopathic thrombocytopenia depending on the presence of solitary low platelet count (<100×109/L) in a patient with multiple bruises and/or petechiae.
Types of ITP and response to treatment
The ITP patients were categorized regarding the duration of illness, 42 (47.72%) were newly diagnosed <3 months, 27 (30.86%) with persistent ITP 3–12 months, and 19 (21.60%) had chronic >12 months ITP. Treatment options regimen includes corticosteroids, IVIG, immunosuppressive drugs, and Eltrombopag (detailed treatment regimens are presented in Table 2). Response to treatment; 38 (43.18%) of patients showed complete response to treatment (CR) which is defined as platelet count ≥100×109/L without clinically significant bleeding, 43 (48.87%) showed partial response to treatment (PR); which is a platelet count between 30 and 100×109/L or twice the baseline platelet count without significant bleeding, and 7 (7.95%) with no response (NR) in patients with the present spleen is platelet count <30×109/L in two different measurements or the increase in platelet is less than twice baseline count (Makis et al., 2017)., (Rodeghiero et al., 2009)
Inclusion and exclusion criteria
Children aged 1–13 years, of both sexes, with primary ITP were included in the study while patients with secondary causes for purpura, splenectomy, with concurrent viral, or autoimmune diseases were excluded from the study or with a history of receiving medications cause thrombocytopenia.
Ethical consideration
Before the sample was taken, the study protocols were thoroughly explained to the parents, and they were asked to sign an informed consent form. The Ethics Committee at the Faculty of Medicine in Fayoum University reviewed and approved this work protocol no (R210-89). This work was done per the Declaration of Helsinki’s ethical requirements.
Handling of sample
With the help of skilled medical staff, we withdrew 5 mL of venous blood from each participant into a plain tube for serum preparation and RNA extraction. For serum separation, samples were left on a straight surface for nearly 15 min, supernatants were transferred to clean tubes and centrifuged at 4,000 xg for 10 min to isolate serum which was kept at -80°C until use.
RNA extraction and assessment of its purification
Total RNA was extracted from the serum according to the manufacturer’s instructions using the miRNeasy Serum/Plasma extraction kit (Qiagen, Valencia, CA, USA). The first step is to clean the area of work, pipettes, and centrifuge with prepared ethanol 70%, second step is aimed at lysis of cells and release of nucleic acid so after wearing clean gloves we added 1,000 μl QIAzol lysis reagent to 200 μL sample and incubated the mix after shaking vigorously at room temperature for 5 min. Thirdly, to enhance phase separation so that RNA is purified from DNA and protein debris, we added 200 μl chloroform, vortexed, and put the samples in the freezer for 5 min to avoid RNA destruction then the samples were centrifuged at 12.000 xg for 15 min. Then, we collected the upper aqueous parts in new tubes and added 1.5% of its volume to 100% ethanol to promote RNA precipitation. Afterward, we used the mini spin column in a 2 ml collection tube accompanied RNeasy kits to promote solid phase separation of RNA by adding the samples into two subsequent sessions (750 μl) each with centrifugation at 8,000 g for 15 s, discarded the flow-through and transferred the spin column containing binding RNA to clean 2 ml collection tubes. We used the washing buffers RWT and RPF in the kit; each spin column received 700 μl of RWT buffer, then centrifuged at 8,000 xg for (Fouad et al., 2022a) s, the flow-through was discarded, and the column was reused for the following phase, we next pipetted a 500 μl buffer RPE to the spin column and centrifuged it at 8,000 xg for 15s, discarded the flow-through water, and repeated the previous process. We moved the spin column to a fresh collecting tube and centrifuged it at full speed for 2 min to dehydrate RNA samples. Finally, for elusion, the spin column was transferred to a clean Eppendorf tube and 50 ul Rnase-free water was pipetted directly onto the column before centrifugation at 8,000 xg for 1 min. We next used a NanoDrop 1,000 spectrophotometer (Thermo Scientific, Waltham, MA, USA) to measure the RNA concentration and purification at 260/280 nm A ratio of 2.0 was considered “pure.”
Reverse transcription (RT) of RNA into complementary DNAs (cDNAs)
The RT2 First Strand Kit (Qiagen, Valencia, CA, USA) was used for reverse transcription to produce cDNA, and the manufacturer’s procedure was followed. RT reaction was done in a final volume of 20 μl (10 μl reverse-transcription mix was added to each tube containing genomic DNA elimination mix), Conventional PCR was used to incubate the samples for 60 min at 37°C. followed by incubation for 5 min at 95°C to inactivate reverse transcriptase.
Quantitative real-time PCR (qPCR) for detection of long non-coding RNAs
It has previously been shown that lncRNAs were expressed in serum (Mohammed et al., 2022)– (Visconti et al., 2020). The RT2 SYBR Green PCR kit (Qiagen, Maryland, USA) was used to evaluate the expression of the lncRNAs IFNG-AS1 and GAS5 in serum using specific primers supplied by Qiagen, (Valencia, CA, USA); for IFNG-AS1 (Catalog no; 330701LPH20079A, Accession no, ENST00000536914.0) and GAS5 (Catalog no; 330701LPH11340A, Accession no, NR_002578.2) and we used GAPDH primer (Catalog no: 330701 LPH31725A, Accession no: ENST00000496049.0) to standardize the expression pattern and quantify the target long non-coding RNAs (Li et al., 2016), (Peng et al., 2021). The reaction mix was prepared in a nuclease-free tube according to the manufacturer’s protocol for a 25 μl per well reaction volume. The Rotor-gene Q Real-time PCR system (Qiagen, USA) was used to perform quantitative real-time PCR under the following conditions: 95°C for 10 min, then 45 cycles of 15s at 95 and 60°C for 1 min.
Calculation of results
Melting curve tests were carried out after the PCR cycles were completed to confirm the particular production of the expected PCR result. The cycle threshold (Ct) value is the number of qPCR cycles essential to the fluorescent signal to cross a specific threshold. By deducting the Ct values of GAPDH from those of the target long non-coding RNAs, ΔCt was determined, and by subtracting the ΔCt of the control samples from the ΔCt of the cases samples, ΔΔCt was determined. Then we used the subsequent equation (2−ΔΔCt equation) (Livak and Schmittgen, 2001) to demonstrate the fold change of target genes relative to controls which were set as 1.
Statistical analysis
Data was presented by mean ± SD (Standard Deviation), number and percentage, median and interquartile range; (IQR). SPSS version 22 (SPSS Inc) was used for analyzing data. The mean, SD, median, and range were calculated for the quantitative data. One-way ANOVA test was used for normal distribution data analysis in more than two groups. When variables were not normally distributed, the Mann–Whitney-U test (2 groups) or Kruskal Wallis test (more than two groups) was used in comparing groups. Otherwise, the independent-T test was used. The significance of the qualitative data was detected by chi-square (χ2). Pearson correlation was done to explore the association between IFNG-AS1 and GAS5 and the clinical parameters, treatment, and response to treatment. The sensitivity and specificity of IFNG-AS1 and GAS5 each alone or combined regarding the discrimination between ITP cases and healthy control subjects the receiver operating characteristic (ROC) curve analysis was done. All the results of the tests were interpreted as significant by considering that p ≤ 0.05.
Sample size calculation
The sample size equals 88 cases, to adapt the cost of research without decreasing the validity of results we used the formula;
Necessary sample size =
to get the appropriate sample size involved in this research, confidence levels 95% were converted into Z scores, the standard deviation was 0.5, and the margin of error was 0.05).
Results
Comparison between ITP children and healthy control children regarding gender, age, and some blood smear parameters
A total of 88 ITP children, ranging in age from 1 to 13 years were included in this case-control study with mean ± SD = 4.9 ± 2.15 years and 49 (55.68%) patients were females and 88 healthy children with ages ranging from 2 to 12 years with mean ± SD = 5.1 ± 2.09 years and 45 (51.13%) were females. This study was constructed to see how effective the lncRNAs IFNG-AS1 and GAS5 were at distinguishing between children within ITP and those within the control group and to explore the link between their levels and types of childhood ITP as well as with treatment regimens and response.
Results represented in (Table 1) showed that the studied groups were matched regarding gender (p = 0.254) and age (p = 0.172). Regards CBC picture, ITP patients had lower hemoglobin (HB) (mean ± SD = 9.9 ± 2.19 gm%), lower platelet count (PL) either before (mean ± SD = 9.33 ± 2.51× 103) or after treatment (mean ± SD = 129.14 ± 48.95 × 103) and higher absolute lymphocytic count (ALC) (mean ± SD = 2,509 ± 926/ml) than controls (mean ± SD = 11.01 ± 1.58 gm% for HB, 191.35 ± 30.15 × 103 for PL count, and 1815 ± 875/ml for ALC, p < 0.05).
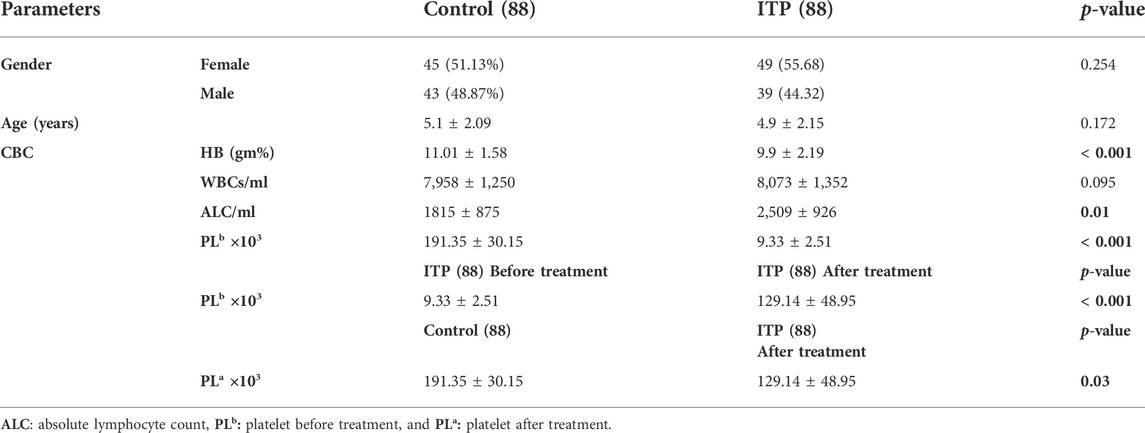
TABLE 1. Comparison between ITP children and healthy control children regarding gender, age, and some blood smear parameters.
There was no significant difference existed between the two groups regards white blood cell count (WBC) (mean ± SD = 8,073 ± 1,352/ml for ITP patients and 7,958 ± 1,250/ml for controls, p = 0.095).
The details of ITP group clinical characters concerning family history, duration of disease, symptoms, treatment, and response to treatment
The patients were divided into groups based on the duration of illness; 42 (47.72%) were newly diagnosed <3 months, 27 (30.86%) with persistent ITP 3–12 months, and 19 (21.60%) had chronic >12 months ITP. Out of 88 patients; 5 (5.68%) with positive family history, 31 (35.23%) with history of bleeding, 59 (67.05%) with preceding febrile illness, 5 (5.68%) with helicobacter infection, and 5 (5.68%) with splenomegaly. All patients had no history of splenectomy, hepatitis B virus (HBV), or hepatitis C virus (HCV) infection. Treatment regimens and responses to treatment are shown in (Table 2).
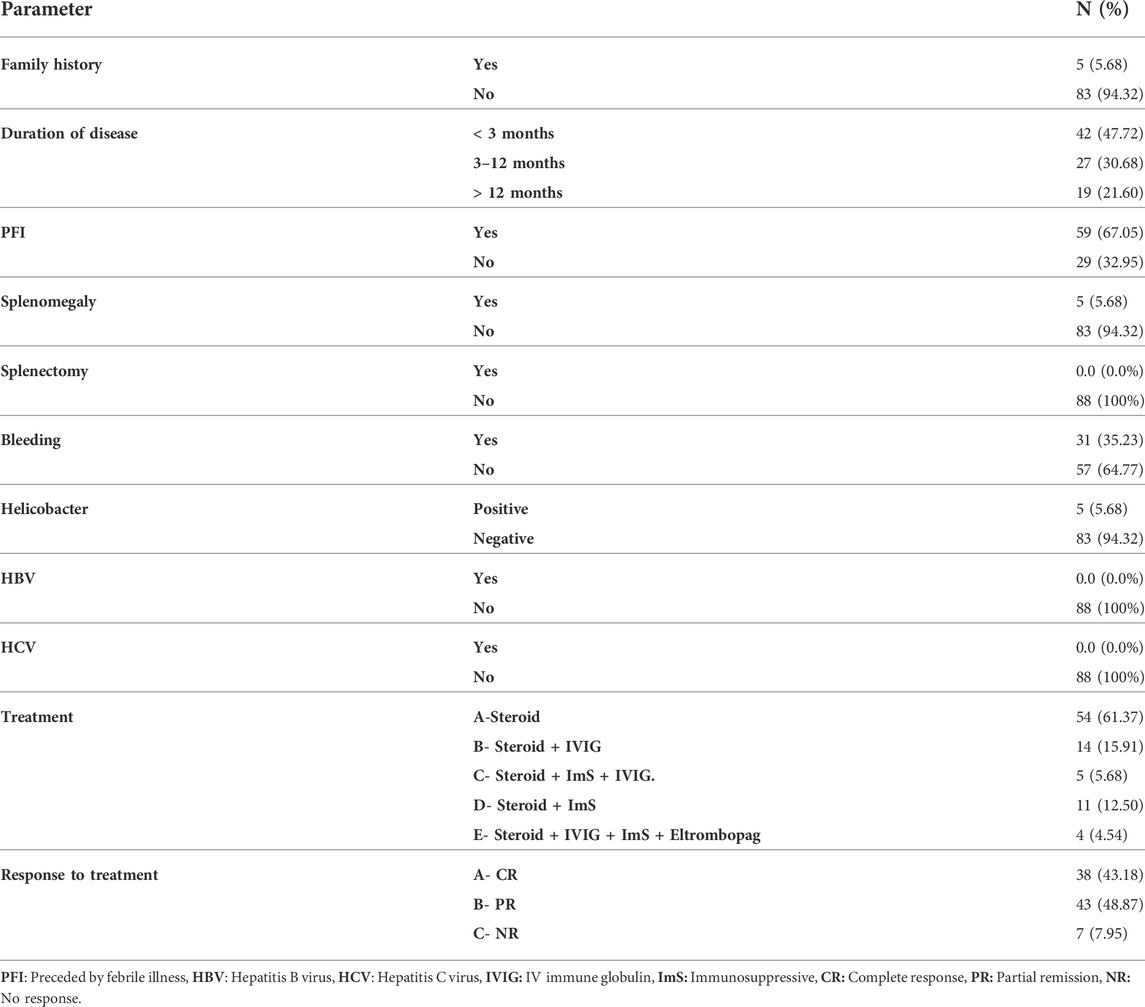
TABLE 2. The details of ITP group clinical characters concerning family history, duration of disease, symptoms, treatment, and response to treatment.
The relative expressions of the serum fold change of target lncRNAs (IFNG-AS1 and GAS5) in ITP patients in comparison with controls
Children with ITP had significantly higher IFNG-AS1 [(median (IQR) = 3.08 (0.2–22.39), mean ± SD = 18.37 ± 19.54] and GAS5 [(median (IQR) = 4.19 (0.9–16.91), mean ± SD = 6.17 ± 11.33)] expressions in serum than that of healthy controls (p < 0.001 each) (Figure 1).
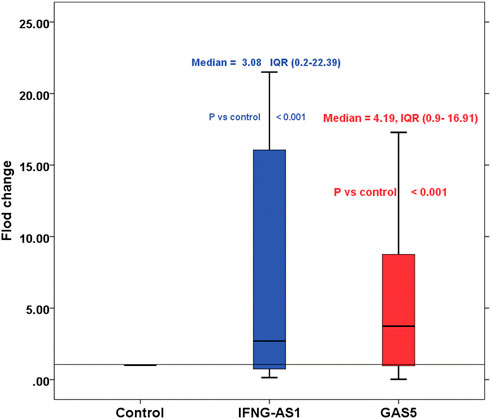
FIGURE 1. Boxplot represents the fold changes of lncRNA IFNG-AS1 and GAS5 in the immune thrombocytopenia (ITP) group in comparison with the healthy control group. IFNG-AS1: Mean ± SD = 18.37 ± 19.54, median (IQR) = 3.08 (0.2–22.39), GAS5: Mean ± SD = 6.17 ± 11.33, median (IQR) = 4.19 (0.9–16.91). Control was set as one according to 2−ΔΔCt equation.
The association between IFNG-AS1 and GAS5 expressions and the clinical signs, treatment, and response to treatment in the ITP group of patients
Regards the association between these genes and clinical characteristics, treatment and response to treatment in cases, we demonstrated that significant higher IFNG-AS1 and GAS5 (p < 0.05) were present in persistent ITP (3–12 months) [ median (IQR) = 4.58 (0.31–22.39) for IFNG-AS1 and 3.77 (0.87–12.36) for GAS5] or chronic ITP (>12 months) [ median (IQR) = 5.6 (0.25–12.59) for IFNG-AS1 and 5.61 (1.15–16.91) for GAS5] when compared to newly diagnosed <3 months patients [IFNG-AS1 median (IQR) = 1.21 (0.2–8.95), and GAS5 median (IQR) = 1.07 (0.09–3.55)]. Also, significant higher lncRNAs IFNG-AS1 and GAS5 were present in patients with partial response to treatment [IFNG-AS1 median (IQR) = 4.15 (0.94–19.25), and GAS5 (median (IQR) = 4.25 (0.81–16.91)] or non-response [IFNG-AS1 median (IQR) = 4.19 (1.25–22.39) and GAS5 median (IQR) = 5.11 (2.34–15.27)] when compared to patients with complete response to treatment (IFNG-AS1 median (IQR) = 2.09 (0.2–14.58) and GAS5 (median (IQR) = 2.51 (0.09–10.33). As well, significant high IFNG-AS1 was detected in patients with positive family history (median (IQR) = 9.09 (2.54–22.39) than in those with negative family history (median (IQR) = 1.98 (0.2–8.04), p = 0.04), just significant p-value = 0.05 was detected between positive family history patients and negative family history patients regards GAS5.
In the cases group, there were no significant variations in target gene expressions in terms of gender, splenomegaly, a history of previous febrile illness or bleeding, helicobacter infection, or treatment regimens (Table 3).
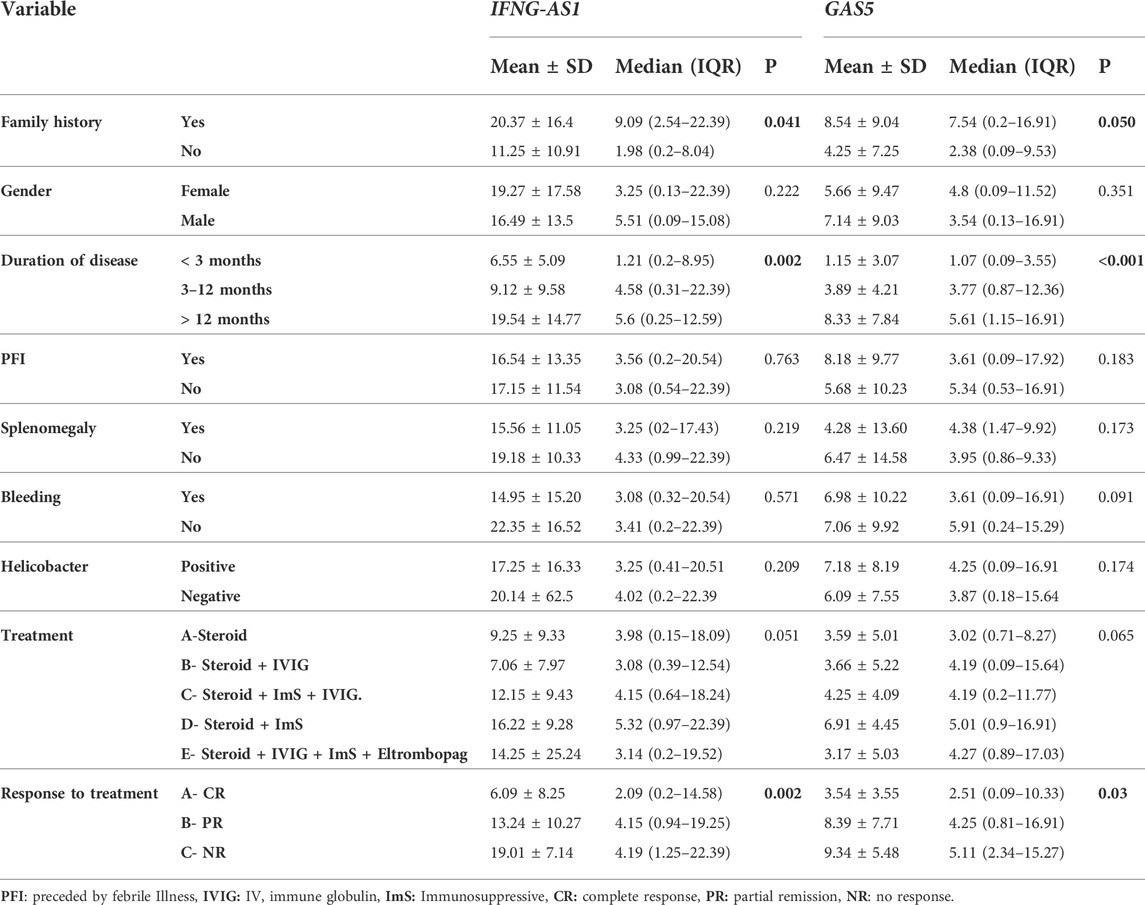
TABLE 3. The association between IFNG-AS1 and GAS5 expressions and the clinical signs, treatment, and response to treatment in the ITP group of patients.
Pearson correlation analysis of the expression of IFNG-AS1 and GAS5 with age and blood smear parameters in cases
Strong significant positive correlation was detected between IFNG-AS1 and GAS5 (r = 0.778, p < 0.001) (Figure 2), while negative correlations were detected between both genes and platelet count after treatment (r = -0.452, p < 0.001 for IFNG-AS1 and r = -0.438, p < 0.001 for GAS5) (Figures 3, 4). There was no correlation between target genes and age, HB concentration, WBCs count, ALC, or PL count before treatment (Table 4).
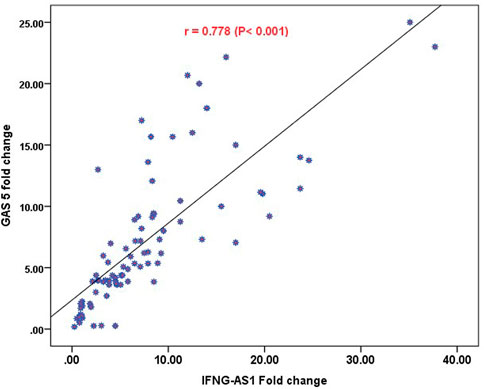
FIGURE 2. Pearson correlation analysis of the expression of IFNG-AS1 and GAS5. A strong significant positive correlation was detected between IFNG-AS1 and GAS5 (r = 0.778, p < 0.001).
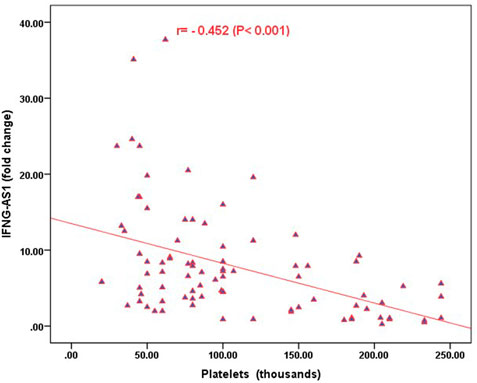
FIGURE 3. Correlation analysis between the expression of IFNG-AS1 and platelet count after treatment. There was a negative correlation between IFNG-AS1 fold change and platelet count after treatment (r = -0.452, p < 0.001).
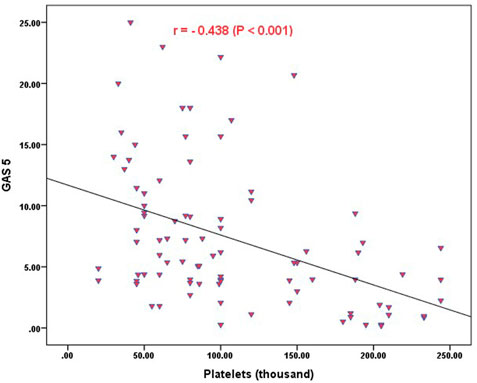
FIGURE 4. Correlation analysis between the expression of GAS5 and platelet count after treatment. There was a negative correlation between GAS5 fold change and platelet count after treatment (r = -0.438, p < 0.001).
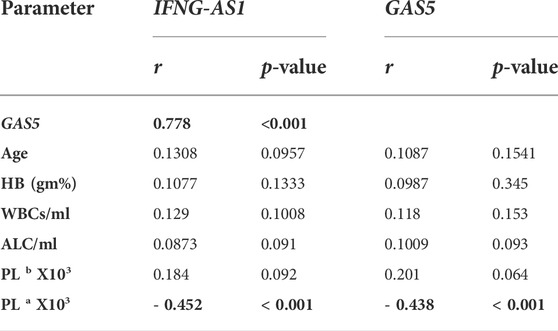
TABLE 4. Pearson correlation analysis of the expression of IFNG-AS1 and GAS5 with age and blood smear parameters.
ROC (Receiver Operating Characteristic) curve analysis to predict diagnostic values of target genes in differentiating ITP patients from controls
Figure 5 and Table 5 are shown the ROC curves of lncRNAs IFNG-AS1 and GAS5 in ITP patients.
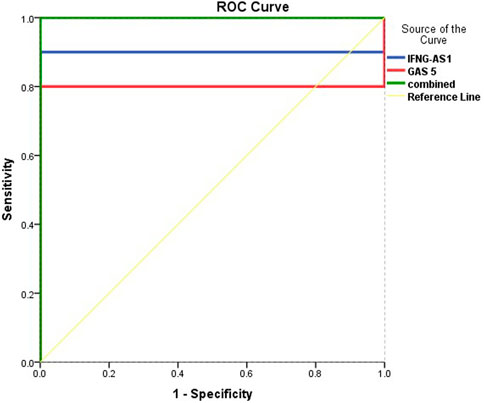
FIGURE 5. ROC curve analysis to predict diagnostic values of target genes in differentiating ITP patients from controls.

TABLE 5. ROC curve analysis to predict diagnostic values of target genes in differentiating ITP patients from controls.
The ROC curves of lncRNAs IFNG-AS1 and GAS5 in ITP patients are demonstrating the diagnostic utility of these markers as predictors in distinguishing between patients with ITP and controls. LncRNA IFNG-AS1; AUC (95% CI) = 0.900 (0.769–0.980), p < 0.001, cut off point 1.38, sensitivity 66.3%, specificity 97.8%, total accuracy, 82.05%. Lnc RNA GAS5, AUC (95% CI) = 0.803 (0.625–0.975), p = 0.001, cut off point 2.58, sensitivity 68.5%, specificity 95.2%, total accuracy 81.85% and their combined expression AUC = 1.000, p < 0.001, sensitivity and specificity 100.0%.
After reviewing the previous literature to explore the functions of target genes in ITP pathogenesis, we designed (Figure 6) to show how these genes could be targets of therapy in childhood ITP.
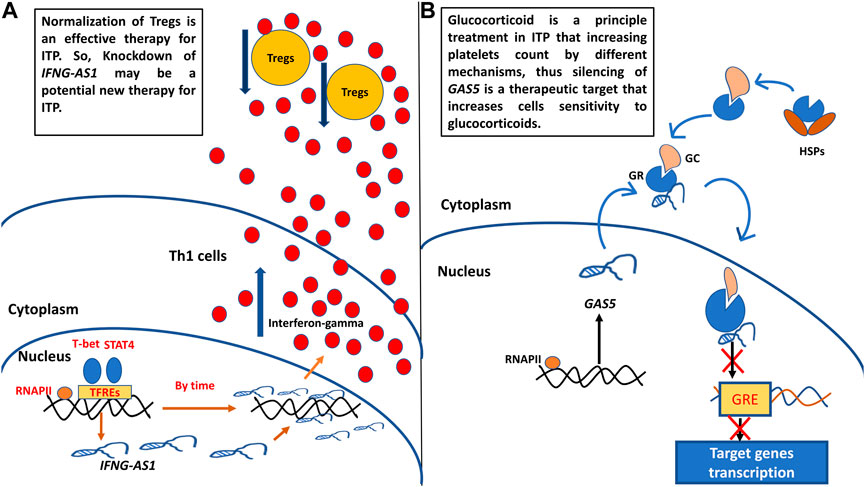
FIGURE 6. (A) Probosed function of IFNG-AS1 in childhood ITP pathogenesis and its supposed role as target of therapy. (B) Proposed function of GAS5 in childhood ITP pathogenesis and its supposed role as target of therapy. Th1: T helper type 1 cells, Tregs: regulatory T cells, RNAPII: RNA polymerase II, TFREs: transcription factors response elements, GC: glucocorticoid, GR: glucocorticoid receptor, GRE: glucocorticoid response element.
Proposed functions of long noncoding RNAs IFNG-AS1 and GAS5 in childhood ITP pathogenesis and their supposed roles as targets of therapy
A. We designed Figure 6 to show the proposed function of target genes IFNG-AS1 and GAS5 in childhood ITP pathogenesis and their potential usage as targets of therapy. IFNG-AS1 is selectively expressed in T helper1 (Th1) cells by the effect of Stat4 and T-bet transcription factors (Collier et al., 2012b), upregulated IFNG-AS1 promoting IFN-γ expression and secretion (Petermann et al., 2019). Excessive IFN-γ decreases CD4+CD25+FoxP3+ Treg cell numbers (the major cells that relate to the maintenance of auto-tolerance) (Zufferey et al., 2017). Normalization of Tregs is an effective therapy for ITP (Semple et al., 2010), So, Knockdown of IFNG-S1 may be a potential new therapy for ITP (Figure 6A).
B. GAS5 is associated with the glucocorticoid receptors (GRs) through hindering binding of GRs to target genes’ glucocorticoid receptor elements (GREs), and repressed GRs transcriptional activity of endogenous glucocorticoid-responsive genes (Wapinski and Chang, 2011; Kino et al., 2010). Hence, the knockdown in GAS5 levels may have an impact on the increased sensitivity of immunosuppressive treatment with glucocorticoid-related drugs (Figure 6B).
Discussion
Childhood Immune Thrombocytopenia (ITP) is typically a benign self-limiting condition that resolves in a few months. However, between 20 and 30% of patients develop chronic ITP, which puts them at risk of serious complications including bleeding such as cerebral hemorrhage and blood cancer (Ayoub et al., 2020). Efficient treatment could decrease the susceptibility of ITP children to these serious complications (Makis et al., 2017) (Kühne et al., 2011). LncRNAs tangled in immune system regulation could be therapeutic targets (Zou and Xu, 2020). Abnormal IFNG-AS1 and GAS5 expression levels have been linked to a number of autoimmune disorders, implying that these genes may play a role in the pathogenesis of autoimmunity and could represent new targets of therapy (Wu et al., 2017), (Peng et al., 2020a).
From the facts that; primary ITP provocation is a result of immune system gene dysregulation, lncRNAs are immune gene regulatory molecules, IFNG-AS1 and GAS5 are two lncRNAs that are directly linked to autoimmune disorders, previously related to adult ITP and no other previous study tested the expression of these genes in childhood ITP, we construct this case-control study to elucidate expressions of target lncRNAs in childhood ITP and their association with pathophysiology and clinical features of the disease as well as their association with types and treatment.
Our results revealed that the age (mean ± SD) of our patients was 5.1 ± 2·19 years, and there is no sex prediction unlike in adults, the initial platelet count was 9.33 ± 2.51×103/L. these results agree with other studies for age, sex, and initial platelet counts (Talaat et al., 2014), (Diab et al., 2021). In this paper the common clinical presentation was bleeding ∼35% followed by splenomegaly ∼6%, in contrast, a study by Ayoub et al, 2020 showed rates of ∼ 27% for bleeding and ∼ 3% for splenomegaly (Ayoub et al., 2020). We reported that more than 50% of our patients had persistent and chronic ITP while prior studies documented a range between 30% and 40% of patients prone to the persistence of thrombocytopenia after 6 months of diagnosis (Makis et al., 2017), (Ayoub et al., 2020).
It should be stressed that the etiology of ITP is unknown, and on the question of possible precipitating factors, this study found that nearly 6% of our patients have a positive family history, this was consistent with Ayoub et al, 2020 (Ayoub et al., 2020) but less than percentage documented by Diab et al, 2021 which reported 4% of patients had positive family history (Diab et al., 2021). History of helicobacter pylori infection accounted for 6% of our cases; other study reported 10% (Ayoub et al., 2020). While nearly 60% experience a period of febrile illness before disease, this was constant with other studies documented that two-thirds of patients had a preceding infection and febrile illness two to 3 weeks before the disease (Ayoub et al., 2020) (Livak and Schmittgen, 2001).
The most important clinically relevant findings were overexpressed lncRNAs IFNG-AS1 and GAS5 in the serum of childhood ITP patients [(median (IQR) = 3.08 (0.2–22.39), mean ± SD = 18.37 ± 19.54 for IFNG-AS1, and median (IQR) = 4.19 (0.9–16.91) for GAS5 than controls, Also, significant higher IFNG-AS1 and GAS5 were linked to patients with positive family history, patients with persistent or chronic ITP than those who are newly diagnosed, patients with no response or partial response to treatment when compared with patients who completely responded to treatment. In addition, IFNG-AS1 and GAS5 expression were significantly negatively correlated with platelet count after therapy.
Regards IFNG-AS1, our results could be explained by the findings of Collier et al, 2012 who implied that IFNG-AS1 is a part of a larger family of lncRNAs called lincRNAs that positively control gene transcription. They defined IFNG-AS1 as a Th1-specific lincRNA that promotes the transcription and secretion of the IFN-γ (Collier et al., 2012a). IFN-γ expression abnormalities have been linked to a variety of autoimmune disorders. In ITP patients, plasma IFN-γ levels were shown to be higher, which hastens disease development by lowering CD4+CD25+FoxP3+ Treg levels (responsible for preserving self-tolerance by interaction with APC and lowering CD19+ B cell and CD8+ T cell responses) (Zufferey et al., 2017), (Talaat et al., 2014). Many pieces of research have demonstrated that ITP patients have decreased Tregs, and interestingly, several medications that boost platelet cell number in ITP, such as glucocorticoid, rituximab, and intravenous immunoglobulin (IVIG), appear to do so via normalization of Tregs (Zufferey et al., 2017), (Semple et al., 2010), Thus these findings suggesting that silencing of IFNG-AS1 is a potential target of therapy for ITP. The previously mentioned data explain the significantly higher IFNG-AS1 levels presented in the partial response or no response patients group and also explain the significant negative correlation between IFNG-AS1 Levels and platelet number after therapy.
A central contribution to recent work is a study that examined the levels of IFNG-AS1 in adult thrombocytopenic patients and found the transcript level of IFNG-AS1 in peripheral blood mononuclear cells (PBMCs) from active adult ITP patients was lower than in healthy controls. Furthermore, researchers discovered a positive correlation between IFN-γ and IFNG-AS1 transcript levels in PBMCs from healthy controls, as well as a similar change tendency after short-term activation. As a result, researchers speculated that IFNG-AS1 has been shown to enhance IFN-γ transcription. Furthermore, IFN-γ overexpression had a negative feedback effect on IFNG-AS1 expression, resulting in lower IFNG-AS1 levels in adult ITP patients (Li et al., 2016).
Our findings are further supported by studies that investigated IFNG-AS1 in other autoimmune diseases which revealed that IFNG-AS1 expression from PBMCs was elevated in Hashimoto’s thyroiditis patients and contributed to Th1 cell response and IFN-γ expression levels which are implicated in the disease’s etiology (Peng et al., 2015). Likewise, lncRNA signatures in ulcerative colitis colonic tissues revealed increased expression of INFG-AS1 which is function as an enhancer of inflammation through positive regulation of IFN-γ expression. (Padua et al., 2016). In the same way, the transcript level of lncRNA IFNG-AS1 and its target gene IFN-γ were shown to be higher in the peripheral blood of rheumatoid arthritis patients (RA) than in controls and they were positively linked (Peng et al., 2020b). Additionally, Fouad et al, 2022 revealed that the expression level of IFNG-AS1 was upregulated significantly in the serum of patients with Behçet disease compared with controls (Fouad et al., 2022b).
Regarding GAS5, the widely accepted function of GAS5 is that it is a tumor-suppressive lncRNA, which is implicated in a wide range of malignancies. Recently, scientists examined levels of GAS5 in immune-related diseases and elucidated its underlying molecular function, they concluded that the expression level of GAS5 was aberrant in patients with autoimmune disorders when compared to controls, and in addition to its tumor arresting function, GAS5 is a potent repressor of the glucocorticoid receptor (GR) (Mayama et al., 2016).
Glucocorticoids (GCs) are a powerful immunosuppressant and are often the essential treatment of inflammatory and autoimmune illnesses, along with preventing rejection in transplanted patients. Several studies indicated that GAS5 is associated with glucocorticoid resistance through its direct attachment to the GR protein by competing with glucocorticoid receptor element (GRE) and acting as a decoy GRE, preventing glucocorticoid-induced gene transcription upregulation, and decreasing GCs activity so contributing to the development of numerous autoimmune diseases (Wu et al., 2020) (Suo et al., 2018). Corticosteroids, which are therapeutically variants of the glucocorticoids, are the principal therapy in ITP patients especially in chronic and resistant cases by different mechanisms include; raising the number of circulatory Tregs, reviving the Th1/Th2 proportion, restoring the Th17 count, constant with a rise in IL-10 and TGF- β, also, it alters B cell activation by lowering beta-cell stimulator (BlyS) and modulates dendritic cells (DCs) (Zufferey et al., 2017). Hence, the knockdown in GAS5 levels may have an impact on increasing the sensitivity of ITP patients to immunosuppressive treatment with glucocorticoid-related drugs. According to these data, we can interpret the significantly higher GAS5 associated with partial or no response patients group, and the negative correlation between GAS5 expression and platelet number after treatment.
Support for this interpretation comes from Moharamoghli et al, 2019 who found that T cells from RA patients had higher amounts of GAS5 than those from controls (Moharamoghli et al., 2019), Also, Suo et al, 2018 documented that GAS5 and miR21 levels were considerably higher in CD4+ T cells from SLE patients than in control subjects, and GAS5 expression in CD4+ T cells was higher in ulcerated SLE patients than in non-ulcerated SLE patients (Suo et al., 2018). In a similar vein, Gharesouran et al, 2018 found that GAS5 levels were up-regulated in multiple sclerosis patients (Gharesouran, Taheri, Sayad, Ghafouri-Fard, Mazdeh, Davood Omrani).
On the other hand, a recent study documented that GAS5 expression was downregulated in ITP patients’ PBMCs and ITP mice’s spleen tissues, and overexpression of GAS5 suppresses Th17 differentiation in vitro and relieved ITP in vivo via STAT3 reduction. Similarly, Li et al, 2019 concluded that the level of GAS5 was reduced in RA synovial tissues (Li et al., 2020). Furthermore, GAS5 and IL-10 mRNA levels in myasthenia gravis patients’ peripheral blood mononuclear cells (PBMCs) were significantly lower than in healthy controls (Peng and Huang, 2022). This inconsistency of results may be due to variances in samples and disease nature.
A strong positive correlation between both genes was detected that confirms their synergistic effects. The ROC curve analysis for lncRNAs IFNG-AS1 and GAS5 in ITP patients are, demonstrating the diagnostic utility of these markers as predictors in distinguishing between patients with ITP and controls with sensitivity and specificity reached 100.0% in the case of the combination of both genes.
From the preceding discussion, we speculated that both genes are diagnostic biomarkers for childhood ITP, and the increasing IFNS-AS1 and GAS5 expressions in childhood ITP patients may participate in the progress of the disease, especially its higher levels were significantly associated with persistent and chronic ITP patients than newly diagnosed patients. This finding agreed with Li et al, 2016 who documented associated high IFNG-AS1 with active ITP when compared to inactive ITP patients (Li et al., 2016) Also, high IFNG-AS1 was present in active ulcerative colitis (Padua et al., 2016) and high GAS5 was linked to active SLE (Suo et al., 2018). Thus, IFNG-AS1 and GAS5 may be used as predictors of the course of the disease.
This study confirms that IFNG-AS1 and GAS5 may be potential targets of therapy as we found that higher target genes were detected in patients with no or partial response to treatment when compared to patients who completely responded to treatment, these results further support the idea of GAS5 function as glucocorticoid resistant enhancer (Mayama et al., 2016). In addition, we conveyed that IFNG-AS1 and GAS5 expressions were significantly negatively correlated with platelet count after therapy, this result was consistent with Li et al, 2016 who documented a negative correlation of IFNG-AS1 with platelet count, but they found no correlation with treatment or duration of the disease (Li et al., 2016).
The limitations of the current study include; 1) The sample size of the current study was modest and selected from the same geographical area which provoked the possibility of selection bias. 2) Insufficiency of scientific literature demonstrating the precise function of target lncRNAs in general and in particular to pediatric diseases including childhood ITP. Further multicentric studies are needed to confirm our findings, verify the utility of target genes as targets of therapy, and better understand the molecular role of IFNG-AS1 and GAS5 in the etiology and pathogenesis of childhood ITP.
The current study contains several new and important insights; findings suggest that lncRNAs IFNG-AS1 and GAS5 are novel diagnostic and prognostic biomarkers for childhood ITP that can aid in a precise prediction of the disease’s progress at the time of diagnosis and could be a useful tool for treatment planning, reducing the risk of bleeding while avoiding drug side effects. We speculated that both genes have underlying molecular contributing roles in the development and prognosis of childhood ITP and may be used as targets of therapy to reduce the propagation of the disease to a chronic state.
Conclusion
LncRNAs IFNG-AS1 and GAS5 are novel diagnostic and prognostic genetic markers for childhood ITP.
Data availability statement
The original contributions presented in the study are included in the article/supplementary material, further inquiries can be directed to the corresponding author.
Ethics statement
The studies involving human participants were reviewed and approved by The Ethics Committee at the Faculty of Medicine in Fayoum University reviewed and approved this work protocol no (R210-89). Written informed consent to participate in this study was provided by the participants’ legal guardian/next of kin.
Author contributions
MAA; suppose the hypotheses of the study, shared in gathering blood samples and data from patients’ medical sheets, performed the RNA extraction and PCR tests, interpreted the information, wrote the manuscript, and corrected the last copy of the paper. SKH; collected the patients’ data and samples, executed the clinical examination, and they shared in the method section writing. AAK, AME, MSF, AAI, EAM; shared in writing the application for obtaining the approval of the ethical committee, shared in the collection of blood samples and data from patients, shared in the execution of RT-PCR, and in writing and correcting the paper.
Acknowledgments
All authors acknowledge help from Omayma O. Abdelaleem, Assistant Professor of Biochemistry, Fayoum University, Egypt, for their help in accomplishing this work.
Conflict of interest
The authors declare that the research was conducted in the absence of any commercial or financial relationships that could be construed as a potential conflict of interest.
Publisher’s note
All claims expressed in this article are solely those of the authors and do not necessarily represent those of their affiliated organizations, or those of the publisher, the editors and the reviewers. Any product that may be evaluated in this article, or claim that may be made by its manufacturer, is not guaranteed or endorsed by the publisher.
References
Ayoub, S. E., Hefzy, E. M., Abd El-Hmid, R. G., Ahmed, N. A., Khalefa, A. A., Ali, D. Y., et al. (2020). Analysis of the expression profile of long non-coding RNAs MALAT1 and THRIL in children with immune thrombocytopenia. IUBMB Life 72 (9), 1941–1950. doi:10.1002/iub.2310
Beshir, M. R., Mohamed, Z., Mohamed, A., Esh, H., and Tayib, R. M. (2021). Conditions of the creative commons attribution (CC BY-sa) license prevalence, prognosis, and outcome in Egyptian children with primary immune thrombocytopenia: A single-center experience. Available at: https://ejhm.journals.ekb.eg/.
Collier, S. P., Collins, P. L., Williams, C. L., Boothby, M. R., and Aune, T. M. (2012). Cutting edge: Influence of Tmevpg1 , a long intergenic noncoding RNA, on the expression of ifng by Th1 cells. J. Immunol. 189 (5), 2084–2088. doi:10.4049/jimmunol.1200774
Collier, S. P., Collins, P. L., Williams, C. L., Boothby, M. R., and Aune, T. M. (2012). Cutting edge: Influence of Tmevpg1 , a long intergenic noncoding RNA, on the expression of ifng by Th1 cells. J. Immunol. 189 (5), 2084–2088. doi:10.4049/jimmunol.1200774
Diab, A. M., Abouamer, A. A., Motaleb, G. S. A., Eid, K. A., and Abdelnaiem, H. I. (2021). Prognostic evaluation of immune thrombocytopenia outcomes in Egyptian children: A retrospective single-center experience. Vopr. Gematologii/onkologii. I. Immunopatol. V. Pediatr. 20 (3), 26–30. doi:10.24287/1726-1708-2021-20-3-26-30
Fouad, N. A., Abdelaleem, O. O., Magdy, M. M., and Senara, S. H. (2022). Assessment of long non-coding RNA (THRIL and TMEVPG1) among Behçets’ disease patients. Egypt. Rheumatol. 44 (1), 5–9. doi:10.1016/j.ejr.2021.07.001
Fouad, N. A., Abdelaleem, O. O., Magdy, M. M., and Senara, S. H. (2022). Assessment of long non-coding RNA (THRIL and TMEVPG1) among Behçets’ disease patients. Egypt. Rheumatol. 44 (1), 5–9. doi:10.1016/j.ejr.2021.07.001
Gao, Y., Li, S., Zhang, Z., Yu, X., and Zheng, J. (2018). The role of long non-coding RNAs in the pathogenesis of RA, SLE, and SS. Front. Med. 5. doi:10.3389/fmed.2018.00193
Gharesouran, J., Taheri, M., Sayad, A., Ghafouri-Fard, S., Mazdeh, M., and Davood Omrani, M. The growth arrest-specific transcript 5 (GAS5) and nuclear receptor subfamily 3 group C member 1 (NR3C1): Novel markers involved in multiple sclerosis.
Ji, J., Dai, X., Yeung, S. C. J., and He, X. (2019). The role of long non-coding RNA GAS5 in cancers,” Cancer Management and Research. Dove Med. Press Ltd. 11, 2729–2737. doi:10.2147/CMAR.S189052
Kino, T., Hurt, D. E., Ichijo, T., Nader, N., and Chrousos, G. P. (2010). Noncoding RNA Gas5 is a growth arrest- and starvation-associated repressor of the glucocorticoid receptor. Sci. Signal. 3 (107), ra8. doi:10.1126/scisignal.2000568
Kühne, T., Berchtold, W., Michaels, L. A., Wu, R., Donato, H., Espina, B., et al. (2011). Newly diagnosed immune thrombocytopenia in children and adults: A comparative prospective observational registry of the intercontinental cooperative immune thrombocytopenia study group. Haematologica 96 (12), 1831. doi:10.3324/haematol.2011.050799
Li, H., Hao, Y., Zhang, D., Fu, R., Liu, W., Zhang, X., et al. (2016). Aberrant expression of long noncoding RNA TMEVPG1 in patients with primary immune thrombocytopenia. Autoimmunity 49 (7), 496–502. doi:10.3109/08916934.2016.1167192
Li, J., Tian, J., Lu, J., Wang, Z., Ling, J., Wu, X., et al. (2020). LncRNA GAS5 inhibits Th17 differentiation and alleviates immune thrombocytopenia via promoting the ubiquitination of STAT3. Int. Immunopharmacol. 80, 106127. doi:10.1016/j.intimp.2019.106127
Li, T., Gu, M., Liu, P., Liu, Y., Guo, J., Zhang, W., et al. (2018). Abnormal expression of long noncoding RNAs in primary immune thrombocytopenia: A microarray related study. Cell. Physiol. biochem. 48 (2), 618–632. doi:10.1159/000491890
Livak, K. J., and Schmittgen, T. D. (2001). Analysis of relative gene expression data using real-time quantitative PCR and the 2(-Delta Delta C(T)) Method. Methods 25 (4), 402–408. doi:10.1006/meth.2001.1262
Makis, A., Gkoutsias, A., Palianopoulos, T., Pappa, E., Papapetrou, E., Tsaousi, C., et al. (2017). Prognostic factors for immune thrombocytopenia outcome in Greek children: A retrospective single-centered analysis. Adv. Hematol. 2017, 7878605. doi:10.1155/2017/7878605
Mayama, T., Marr, A. K., and Kino, T. (2016). Differential expression of glucocorticoid receptor noncoding RNA repressor Gas5 in autoimmune and inflammatory diseases. Hormone Metabolic Res. 48 (8), 550–557. doi:10.1055/s-0042-106898
Mohammed, S. R., Abdelaleem, O. O., Ahmed, F. A., Abdelaziz, A. A., Hussein, H. A., Eid, H. M., et al. (2022). Expression of lncRNAs NEAT1 and lnc-DC in serum from patients with behçet’s disease can Be used as predictors of disease. Front. Mol. Biosci. 8, 797689. doi:10.3389/fmolb.2021.797689
Moharamoghli, M., Hassan-Zadeh, V., Dolatshahi, E., Alizadeh, Z., and Farazmand, A. (2019). The expression of GAS5, THRIL, and RMRP lncRNAs is increased in T cells of patients with rheumatoid arthritis. Clin. Rheumatol. 38 (11), 3073–3080. doi:10.1007/s10067-019-04694-z
Padua, D., Mahurkar-Joshi, S., Law, I. K. M., Polytarchou, C., Vu, J. P., Pisegna, J. R., et al. (2016). A long noncoding RNA signature for ulcerative colitis identifies IFNG-AS1 as an enhancer of inflammation. Am. J. Physiol. Gastrointest. Liver Physiol. 311, 446–457. doi:10.1152/ajpgi.00212.2016
Palazzo, A. F., and Lee, E. S. (2015). Non-coding RNA: What is functional and what is junk? Front. Genet. 5. doi:10.3389/fgene.2015.00002
Peng, H., Liu, Y., Tian, J., Ma, J., Tang, X., Rui, K., et al. (2015). The long noncoding RNA IFNG-AS1 promotes T helper type 1 cells response in patients with Hashimoto’s thyroiditis. Sci. Rep. 5, 17702. doi:10.1038/srep17702
Peng, H., Ren, S., Liu, Y., Zhou, H., Tang, X., Yang, J., et al. Elevated expression of the long noncoding RNA IFNG-AS1 in the peripheral blood from patients with rheumatoid arthritis. J. Immunol. Res. 2020, 6401978. doi:10.1155/2020/6401978
Peng, H., Ren, S., Liu, Y., Zhou, H., Tang, X., Yang, J., et al. Elevated expression of the long noncoding RNA IFNG-AS1 in the peripheral blood from patients with rheumatoid arthritis. J. Immunol. Res. 2020, 6401978. doi:10.1155/2020/6401978
Peng, S., and Huang, Y. (2022). LncRNA GAS5 positively regulates IL-10 expression in patients with generalized myasthenia gravis. Brain Behav. 12 (1), e2457. doi:10.1002/brb3.2457
Peng, T., Ji, D., and Jiang, Y. (2021). Long non-coding RNA GAS5 suppresses rheumatoid arthritis progression via miR-128-3p/HDAC4 axis. Mol. Cell. Biochem. 476 (6), 2491–2501. doi:10.1007/s11010-021-04098-1
Petermann, F., Pekowska, A., Johnson, C. A., Jankovic, D., Shih, H. Y., Jiang, K., et al. (2019). The magnitude of IFN-γ responses is fine-tuned by DNA architecture and the non-coding transcript of ifng-as1. Mol. Cell 75 (6), 1229–1242. e5, Sep. 2019. doi:10.1016/j.molcel.2019.06.025
Rodeghiero, F., Stasi, R., Gernsheimer, T., Michel, M., Provan, D., Arnold, D. M., et al. (2009). Standardization of terminology, definitions and outcome criteria in immune thrombocytopenic purpura of adults and children: Report from an international working group. Blood 113, 2386. doi:10.1182/blood-2008-07-162503
Semple, J. W., Provan, D., Garvey, M. B., and Freedman, J. (2010). Recent progress in understanding the pathogenesis of immune thrombocytopenia. Curr. Opin. Hematol. 17 (6), 590–595. doi:10.1097/MOH.0b013e32833eaef3
Suo, Q. F., Sheng, J., Qiang, F. Y., Tang, Z. S., and Yang, Y. Y. (2018). Association of long non-coding RNA GAS5 and miR-21 levels in CD4+ T cells with clinical features of systemic lupus erythematosus. Exp. Ther. Med. 15 (1), 345–350. doi:10.3892/etm.2017.5429
Talaat, R. M., Elmaghraby, A. M., Barakat, S. S., and El-Shahat, M. (2014). Alterations in immune cell subsets and their cytokine secretion profile in childhood idiopathic thrombocytopenic purpura (ITP). Clin. Exp. Immunol. 176 (2), 291–300. doi:10.1111/cei.12279
Visconti, V. V., Fittipaldi, S., Ciuffi, S., Marini, F., Isaia, G., D'Amelio, P., et al. (2020). Circulating long non-coding rna gas5 is overexpressed in serum from osteoporotic patients and is associated with increased risk of bone fragility. Int. J. Mol. Sci. 21 (18), 69300–E7012. doi:10.3390/ijms21186930
Wapinski, O., and Chang, H. Y. (2011). Long noncoding RNAs and human disease. Trends Cell Biol. 21 (6), 354–361. doi:10.1016/j.tcb.2011.04.001
Wu, D., Gu, B., Qian, Y., Sun, Y., Chen, Y., Mao, Z. D., et al. (2020). Long non-coding RNA growth arrest specific-5: A potential biomarker for early diagnosis of severe asthma. J. Thorac. Dis. 12 (5), 1960. doi:10.21037/jtd-20-213
Wu, G.-C., Li, J., Leng, R. X., Li, X. P., Li, X. M., Wang, D. G., et al. (2017). Identification of long non-coding RNAs GAS5, linc0597 and lnc-DC in plasma as novel biomarkers for systemic lupus erythematosus. Oncotarget 8 (14), 23650–23663. doi:10.18632/oncotarget.15569
Zou, Y., and Xu, H. (2020). Involvement of long noncoding RNAs in the pathogenesis of autoimmune diseases. J. Transl. Autoimmun. 3, 01. doi:10.1016/j.jtauto.2020.100044
Keywords: IFNG-AS1, GAS5, childhood ITP, qRT-PCR, diagnosis, prognosis, biomarkers
Citation: Ali MA, Hussein SK, Khalifa AA, El Amin Ali AM, Farhan MS, Ibrahim Amin AA and Mohamed EA (2022) The Ifng antisense RNA 1 (IFNG-AS1) and growth arrest-specific transcript 5 (GAS5) are novel diagnostic and prognostic markers involved in childhood ITP. Front. Mol. Biosci. 9:1007347. doi: 10.3389/fmolb.2022.1007347
Received: 30 July 2022; Accepted: 12 September 2022;
Published: 12 October 2022.
Edited by:
Xin Zhang, Jiangmen Central Hospital, ChinaReviewed by:
Reza Gheitasi, University Hospital Jena, GermanySeyed Mohammad Gheibihayat, Shahid Sadoughi University of Medical Sciences and Health Services, Iran
Copyright © 2022 Ali, Hussein, Khalifa, El Amin Ali, Farhan, Ibrahim Amin and Mohamed. This is an open-access article distributed under the terms of the Creative Commons Attribution License (CC BY). The use, distribution or reproduction in other forums is permitted, provided the original author(s) and the copyright owner(s) are credited and that the original publication in this journal is cited, in accordance with accepted academic practice. No use, distribution or reproduction is permitted which does not comply with these terms.
*Correspondence: Marwa A. Ali, dr_marwag@yahoo.com, Mag04@fayoum.edu.eg