- 1College of Pharmacy, Anhui University of Chinese Medicine, Hefei, China
- 2Anhui Province Key Laboratory of Pharmaceutical Preparation Technology and Application, Hefei, China
- 3Anhui Province Key Laboratory of Chinese Medicinal Formula, Hefei, China
- 4Bengbu Medical College, Bengbu, China
- 5The First Affiliated Hospital of Bengbu Medical College, Bengbu, China
- 6State Key Laboratory of Quality Research in Chinese Medicine, Macau University of Science and Technology, Macau, China
Extracellular vesicles (EVs) are nanosized particles released by numerous kinds of cells, which are now increasingly considered as essential vehicles of cell-to-cell communication and biomarkers in disease diagnosis and treatment. They contain a variety of biomolecular components, including lipids, proteins and nucleic acids. These functional molecules can be transmitted between tumor cells and other stromal cells such as endothelial cells, fibroblasts and immune cells utilizing EVs. As a result, tumor-derived EVs can deliver molecules to remodel the tumor microenvironment, thereby influencing cancer progression. On the one hand, tumor-derived EVs reprogram functions of endothelial cells, promote cancer-associated fibroblasts transformation, induce resistance to therapy and inhibit the immune response to form a pro-tumorigenic environment. On the other hand, tumor-derived EVs stimulate the immune response to create an anti-tumoral environment. This article focuses on presenting a comprehensive and critical overview of the potential role of tumor-derived EVs-mediated communication in the tumor microenvironment.
Introduction
Extracellular vesicles (EVs), including exosomes and ectosomes, are nanoscale particles released by nearly all types of cells (Théry et al., 2018). Relying on transferring microRNA (miRNA), long noncoding RNA (lncRNA), messenger RNA (mRNA) and proteins, EVs modulate the functions and phenotypes of target cells (Bayraktar et al., 2017; Choi et al., 2017; Gon et al., 2017). For instance, the delivery of miR-330-3p from plasma cells to ovarian cancer cells by EVs induces a mesenchymal phenotype of ovarian cancers (Yang et al., 2021). In addition, EVs isolated from human vascular endothelial cells contain some cardioprotective proteins, which contribute to promoting human myocardium survival after ischemia-reperfusion injury (Yadid et al., 2020). Vesicular miR-21 derived from tubular epithelial cells stimulates fibroblast and subsequently causes renal fibrosis in vivo (Zhao S. et al., 2021). Vesicular lncRNA-SOX2OT from non-small cell lung cancer (NSCLC) cells induces osteoclast differentiation and promotes bone metastasis (Ni et al., 2021).
EVs are closely related to the physical and pathological processes of diseases, especially cancer (Wu et al., 2017; Burnouf et al., 2019; Gamage and Fraser, 2021). Tumor growth requires constant nutrients and oxygen delivered from the vascular network, as they cannot grow above 2 mm2 with an inadequate vascular supply (Small et al., 2014). Thereby, angiogenesis, the growth of new blood vessels from the posterior capillary veins and existing capillaries, is vital for tumor progression. EVs mediate communication between tumor cells and endothelial cells, thereby inducing angiogenesis and promoting tumor growth (Wan et al., 2018; Xu et al., 2018). Besides inducing angiogenesis, tumor-derived EVs can also regulate cancer-associated fibroblasts (CAFs) transformation. Since CAFs can remodel the stromal extracellular matrix (ECM) to facilitate tumor cell migration and invasion, CAFs transformation may promote cancer progression. In addition, EVs released from resistant tumor cells have the ability to induce resistance to cancer therapy, which further facilitates tumor progression. Immune cells such as natural killer (NK) cells, macrophages, T cells and B cells can interact with tumor cells via EVs, thereby causing their functions and phenotypes change. Furthermore, crosstalk between tumor-derived EVs and host immune system regulates immune response, thereby influencing cancer progression. Of note, tumor-derived EVs can be isolated from the conditioned medium of cancer cells but also from various body fluids like blood and ascites of cancer patients (Larrea et al., 2016). Due to their cargo diversity and specificity, tumor-derived EVs are promising biomarkers for cancer diagnosis and treatment to reflect the status of parental cancer cells.
The Biogenesis of EVs
The term EVs is used to describe almost all types of membrane particles secreted from cells. Based on their size and biogenesis, EV subpopulations can be divided into exosomes and ectosomes (Théry et al., 2018). Exosomes are secreted by inward invagination of the plasma membrane (Wolf, 1967; Johnstone et al., 1987). The first invagination of the plasma membrane leads to the generation of an early-sorting endosome that contains fluids, extracellular components and cell surface proteins. The early-sorting endosome undergoes a series of transformations to mature into the late-sorting endosome. The second invagination of the late-sorting endosome results in the formation of multivesicular bodies (MVBs) that contains intraluminal vesicles (ILVs). The MVBs can fuse with the plasma membrane to release exosomes with a size range of 30–150 nm in diameter. The basic mechanisms responsible for exosomes biogenesis have been reported. The endosomal sorting complex required for transport (ESCRT) machinery, containing four protein complexes (ESCRT-0, -I, -II, and -III) along with associated proteins (VTA-1, Alix and VPS4), is closely related to the biogenesis of MVBs and ILVs (Henne et al., 2011). The specific functional components of ESCRT have also been investigated. While the silence of ESCRT-0 and ESCRT-I (HRS) decreases the biogenesis of exosomes, depletion of other ESCRT components exerts no effects or even increases the biogenesis of exosomes (Colombo et al., 2013). ESCRT proteins also play an essential role in specifying the loading of functional cargoes into exosomes. They mediate the sorting of cargo at endosomal plasma and subsequently induce the late-sorting endosomes to release ILVs (later exosomes) with the sorted cargoes. Exosomes biogenesis can operate in an ESCRT-independent manner in some cancer cells, which has been demonstrated by silencing multiple ESCRTs (Stuffers et al., 2009). In addition, some RAB GTPases (RAB27, RAB11, and RAB31) have been found to drive MVBs transport and ILVs biogenesis (Savina et al., 2002; Ostrowski et al., 2010; Wei et al., 2020). For instance, RAB31 can enhance the formation of ILVs and inhibit the degradation of MVBs in an ESCRT-independent manner (Wei et al., 2020). Mechanically, the high level of RAB31 can drive epidermal growth factor receptor into MVBs to generate ILVs and recruit TBC1D2B to prevent MVBs degradation (Wei et al., 2020). However, the upstream of RAB GTPases is not well clarified. Song et al. (2019) found that KIBRA could inhibit the ubiquitination and degradation of RAB27a, thereby contributing to exosomes biogenesis (Song et al., 2019). Phospholipase D2 and its product phosphatidic acid are involved in ILVs biogenesis and exosomes release (Egea-Jimenez and Zimmermann, 2018). Notably, ESCRT-dependent pathways and ESCRT-independent pathways can also jointly drive exosomes biogenesis. Syndecan-syntenin complexes bind ESCRT-I and ESCRT-III via Alix, leading to enhanced ILVs biogenesis (Baietti et al., 2012). Moreover, tyrosine phosphatase Shp2 has been found to inhibit exosomes biogenesis via dephosphorylating syntenin (Zhang Y. et al., 2021). CD63, belonging to tetraspanin family, is associated with sorting cargoes into exosomes (Theos et al., 2006; van Niel et al., 2011).
Ectosomes with a size range of 50–1,000 nm in diameter are released by shedding or outward budding of the plasma membrane. This process is driven by translocating phosphatidylserine to the outer-membrane leaflet (Zwaal and Schroit, 1997). The mechanisms involved in the biogenesis of ectosomes have been studied. Inhibiting VPS4 is shown to impair ectosomes release, suggesting that ESCRT-III is also required for ectosomes biogenesis (Mathieu et al., 2019). Small GTPase RhoA, an essential regulator of actin cytoskeletal remodeling, is closely related to ectosomes biogenesis in different tumor cells (Li et al., 2012). Moreover, RHO-associated protein kinase (ROCK) has been revealed to mediate the function of RhoA in ectosomes biogenesis. Thus, inhibition of ROCK-1 and ROCK-2 by the small molecule Y-27632 can decrease ectosomes biogenesis (Li et al., 2012). Ectosomes are rich in cholesterol, and knockdown of cholesterol can inhibit ectosomes biogenesis (Del Conde et al., 2005). In addition, nSMAse participates in shedding budding of the plasma membrane, and hence controls ectosomes biogenesis (Menck et al., 2017).
Tumor Microenvironment and Tumor-Derived EVs
The TME (tumor microenvironment) comprises tumor cells, endothelial cells, fibroblasts, and immune cells as well as extracellular components such as ECM, cytokines and growth factors (Lv et al., 2012; Jin and Jin., 2020) (Figure 1). The ECM is a highly dynamic three-dimensional network composed of plenty of fibrous proteins and glycoproteins (Mouw et al., 2014). Tumors often exhibit ECM deposition and degradation, and this dysregulation state supports tumorigenesis and metastasis and induces angiogenesis (Sükei et al., 2021). Cytokines are small molecular polypeptides or proteins that serve as immunomodulatory effectors. Overproduction of IL-6 by tumor cells activates STAT-3, a key transcription factor central to immune escape and it is an important regulator in the crosstalk between tumor cells and TME (Lokau et al., 2019). IL-10 is an anti-inflammatory cytokine and its serum levels are negatively related to the tumor prognosis (Pasvenskaite et al., 2021). On the other hand, IL-10 exerts anti-tumor activity by enhancing the immune-stimulatory effect of CD8+ T cell (Naing et al., 2018). Some growth factors in the TME inhibit normal stromal cells proliferation and promote tumor cells metastasis. Transforming growth factor-β (TGF-β), fibroblast growth factor (FGF) and vascular endothelial growth factor (VEGF) form a pro-tumorigenic environment that fosters tumor cell survival, progression and metastasis and directs abnormal vessel growth (Zhou X. et al., 2018; Shang et al., 2020; Zheng et al., 2021). However, TGF-β also shows anti-oncogenic properties in carcinogenesis. TGF-β has been reported to inhibit tumor cell proliferation and induce apoptosis in the early stages of carcinogenesis (Inman, 2011).
As a means of communication between tumor cells and the microenvironment, EVs play an essential role in remodeling the local microenvironment (Milane et al., 2015). Numerous studies have revealed that EVs released by tumor cells contain a variety of biomolecular components, including lipids, proteins and nucleic acids (Taylor et al., 2011; Mathivanan et al., 2012; Agudiez et al., 2020). Especially, those nucleic acids components such as miRNAs and lncRNAs may mediate the formation of a protumoral or an anti-tumoral soil in the microenvironment, thereby influencing tumor progression. Interestingly, hypoxic or metastatic status of tumors appears to a strong force in sorting the loading of composition into EVs, which affects functions of tumor-derived EVs in the TME (Kucharzewska et al., 2013; Yokoi et al., 2017; Chen et al., 2018). Hypoxia is a common feature in most malignant tumors. In hypoxic microenvironment, tumor cells drive glucose mainly into lactate to meet the energy requirements. This phenomenon, called the Warburg effect, is one of the cellular mechanisms by which cancer cells adapt to hypoxic microenvironment and enhance survival (Parks et al., 2017). PKM2, which plays a key role in the Warburg effect, is the enhancer of anaerobic glycolysis. Hypoxic NSCLC cell derived-EVs promote PKM2-dependent glycolysis and subsequently produce metabolites to eliminate ROS, thereby inhibiting tumor apoptosis and promoting tumor growth (Wang et al., 2021). EVs derived from breast cancer enhance the ability of CAFs in response to different metabolic environment by activating MYC signaling pathway in stromal cells resulting in rapid tumor growth (Yan et al., 2018). Moreover, EVs released from Lewis lung carcinoma can induce immunosuppressive macrophages by NF-κB-mediated metabolism reprogramming, leading to tumor metastasis (Morrissey et al., 2021).
Regulation of Protumoral Functions of Endothelial Cells by Tumor-Derived EVs
Tumor-derived EVs are thought to regulate protumoral functions of endothelial cells in numerous types of cancers including hepatocellular carcinoma (HCC) (Lin et al., 2018), colorectal cancer (Huang and Feng, 2017; Zeng et al., 2018; He et al., 2021), cervical cancer (Wu et al., 2019), nasopharyngeal carcinoma (Bao et al., 2018; Tian et al., 2021; Zhang K. et al., 2021), glioma (Ma et al., 2017; Wang Z.-F. et al., 2019), and lung cancer (Hsu et al., 2017). As EVs can be internalized by an endocytic-like process, they may deliver regulatory biomolecules into vascular endothelial cells. Thus, tumor cells can participate in the regulation of endothelial cell proliferation, migration, sprouting, branching, as well as tubular-like structure formation by secreting EVs (Zhuang et al., 2012; French et al., 2017).
Some miRNAs, lncRNAs and proteins delivered by EVs have been reported to participate in regulating protumoral functions of endothelial cells (Table 1). miR-210 enriched in EVs of malignant tumors may promote tubular-like structure formation of endothelial cells, leading to pro-angiogenic activities and rapid tumor growth. In HCC, abundant miR-210 can be packed into EVs and transferred to endothelial cells (Lin et al., 2015; Lin et al., 2018). After taking up by human umbilical vein endothelial cells (HUVECs), miR-210 stimulates angiogenesis via down-regulating the expression of SMAD4 and STAT6 (Lin et al., 2018). Vesicular miR-21-5p from colorectal cancer decreases Krev interaction trapped protein 1 expression to activate β-catenin signaling pathway and promote the expression of angiogenesis-related factors like VEGFA, thereby stimulating vascular permeability and angiogenesis (He et al., 2021). In addition, cervical cancer-derived vesicular miR-221-3P promotes angiogenesis by inhibiting the expression of thrombospondin-2 in HUVECs, which consequently enhances tumor growth in vivo (Wu et al., 2019). miR-144 is a key angiogenesis inducer for neo-angiogenesis in nasopharyngeal carcinoma (Tian et al., 2021). Vesicular miR-144 suppresses FBXW7 and increases hypoxia-inducible factor-1α (HIF-1α) and VEGFA in recipient cells, which consequently promotes endothelial cells migration and invasion (Tian et al., 2021). Moreover, the transfer of high mobility group box 3 (HMGB3) from nasopharyngeal carcinoma cells to endothelial cells via EVs induces angiogenesis (Zhang K. et al., 2021). Interestingly, neo-angiogenesis in nasopharyngeal carcinoma facilitates the formation of pre-metastatic niches, which further causes tumor metastasis (Zhang K. et al., 2021). Vesicular miR-26a from glioma down-regulates phosphatase and tensin homolog (PTEN) expression to stimulate PI3k/AKT signaling, thereby contributing to the proliferation of human brain microvascular endothelial cells (HBMECs) (Wang Z.-F. et al., 2019). Ma et al. (2017) believe that the delivery of lncRNA HOTAIR from glioma cancer cells to HBMECs via EVs up-regulates the level of pro-angiogenic factor VEGFA.
It is well-known that disordered vascular distribution and abnormal vascular structure lead to specific hypoxia in many solid tumors. In turn, tumor-derived EVs secreted under hypoxic conditions induce proliferation and migration of endothelial cells, thereby enhancing angiogenesis and tumor growth. For example, EVs isolated from colorectal cancer cells under hypoxia conditions show a more potent pro-angiogenic effect as compared with that from colorectal cancer cells under normoxia conditions (Huang and Feng, 2017). The reason for this phenomenon may be that Wnt4 is highly enriched in hypoxic colorectal cancer-derived EVs, and the increased Wnt4 stimulates the β-catenin signaling pathway in endothelial cells (Yamada, 2017). Similarly, lung cancer-derived vesicular miR-23a under hypoxia conditions enhances the production of HIF-1α in endothelial cells via inhibiting the expression of prolyl hydroxylase 1/2, thereby directly promoting angiogenesis and tumor growth (Hsu et al., 2017).
On the other hand, the emerging evidence has shown that the contents of tumor-derived EVs may be enriched at the metastatic stage during cancer development, and those increased contents can be delivered to endothelial cells to exert biological roles. For example, miR-23a is shown to be significantly higher in nasopharyngeal carcinoma tissues with metastasis than those without metastasis, and its level is associated with angiogenesis (Bao et al., 2018). Furthermore, the molecular mechanism for vesicular miR-23a-mediated angiogenesis may be related to testis-specific gene antigen (Bao et al., 2018). Metastasis-induced vesicular miR-25-3p promotes vascular permeability and angiogenesis, leading to the formation of pre-metastatic niches (Zeng et al., 2018). Mechanically, vesicular miR-25-3p in colorectal cancer can silence Krüppel-like factor 2 and krüppel-like factor 4, thereby enhancing the expression of vascular endothelial growth factor receptor 2 (VEGFR2) and inhibiting the expression of occludin, zonula occludens-1 and Claudin5 (Zeng et al., 2018).
Tumor-derived EVs play an important role in inducing angiogenesis. Similarly, EVs derived from endothelial cell and perivascular cell are also a key player in tumor progression. Anti-angiogenic therapies are thought to improve the prognosis of tumor patients by inhibiting tumor vascularization. However, the outcomes of anti-angiogenic therapies are not ideal for most patients. EVs released from endothelial cells treated with vandetanib enrich VEGF, thus promoting angiogenesis and tumor growth in vivo (Zeng et al., 2019). In addition, EVs released from perivascular cell trigger endothelial progenitor cells recruitment after anti-angiogenic therapy cessation, which contributes to blood vessel regrowth and rapid tumor growth (Huang et al., 2021). Mechanically, Gas6-containing perivascular cell-derived EVs activate Axl signaling and subsequently promote tumor revascularization (Huang et al., 2021).
Regulation of Protumoral Functions of CAFs by Tumor-Derived EVs
As the main contributor to remodel tumor stroma, CAFs are often transformed from resident fibroblasts, mesenchymal stem cells (MSCs) and epithelial-to-mesenchymal transition (EMT) cells after taking up tumor-derived EVs (Figure 2). The active CAFs may enhance angiogenesis and metastasis, thereby contributing to establishing a tumor-promoting environment. Hodgkin lymphoma-derived EVs transform normal fibroblasts into pathological CAFs utilizing the NF-κB signaling pathway, which leads to the release of neo-angiogenesis factors (Dörsam et al., 2018). Notably, many studies have shown that the delivery of functional biomolecules plays a vital role in regulating CAFs transformation (Paggetti et al., 2015; Fang et al., 2018; Giusti et al., 2018; Yang et al., 2018; Wang J. et al., 2018; Zhou Y. et al., 2018). For instance, EVs released from chronic lymphocytic leukemia cells induce fibroblasts transformed to CAFs by the enrichment of some regulatory proteins and miRNAs from parental cells, which consequently causes rapid tumor growth (Paggetti et al., 2015). In addition to regulating the transformation of fibroblasts into a CAF phenotype, tumor-derived EVs have also been demonstrated to play an important role in inducing the transition of MSCs into CAFs. EVs isolated from breast cancer stimulate SMAD-mediated pathway and subsequently increase CAFs marker expression in MSCs, which consequently enhances angiogenesis and metastasis (Cho et al., 2012). Moreover, tumor cells-derived EVs are capable of regulating the transformation of pericytes into a pathological CAFs phenotype. Relying on releasing EVs, gastric cancer cells promote pericytes proliferation and migration, and induce pericytes transformed into CAFs (Ning et al., 2018). Mechanically, gastric cancer cells-derived EVs stimulate PI3k/AKT and MEK/ERK pathways, leading to the up-regulated expression of CAFs markers (Ning et al., 2018).
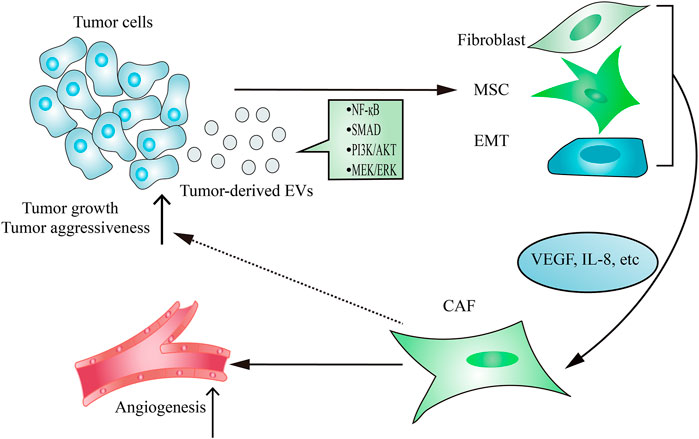
FIGURE 2. Tumor-derived EVs regulate CAFs transformation. The delivery of functional signaling factors from tumor cells to fibroblasts, MSCs or EMT contributes to CAFs transformation, which consequently promotes tumor growth and aggressiveness and induces angiogenesis.
Due to the emerging evidence indicates EVs isolated from tumor cells response to hypoxia, many researchers have investigated the potential of tumor-derived EVs under hypoxia conditions in CAFs transformation (Kucharzewska et al., 2013; Wang et al., 2014; Ramteke et al., 2015). It has been reported that EVs secreted from prostate cancer cells under hypoxia conditions promote CAFs transformation and tumor aggressiveness (Ramteke et al., 2015). Interestingly, EVs derived from tumor cells can not only enrich some proteins, but also load some specific proteins that may induce tumor-promoting microenvironment under hypoxia conditions (Ramteke et al., 2015). This finding suggests that unique components loaded in hypoxia tumor-derived EVs may be helpful to CAFs transformation and tumor progression.
On the other hand, tumor-derived EVs appear to be enriched during fibroblasts reprogramming may be a reaction to the high-metastatic tumor state. Additionally, the functional contents of EVs can also be related to the metastatic status of HCC cells (Fang et al., 2018). The amount of EVs is much higher in high-metastatic cancer cells than that in low-metastatic cancer cells (Fang et al., 2018). This further enhances the regulatory effect of high-metastatic tumor cell-derived EVs on CAFs transformation. Mechanically, elevated miR-1247-3p in EVs isolated from high-metastatic tumor cells that promotes CAFs transformation via inhibiting B4GALT3 expression (Fang et al., 2018).
Tumor-derived EVs play an important role in CAFs transformation, in turn, CAF-derived EVs participate in tumorigenesis. For instance, vesicular miR-92a-3p from CAFs induce EMT, chemoresistance and cancer stemness in colorectal cancer by activating Wnt/β-catenin signaling pathway (Hu et al., 2019). EVs released from CAFs enrich miR-196a by activating heterogeneous nuclear ribonucleoprotein A1, leading to decreased CDKN1B and ING5 in recipient head and neck cancer cancer cells, and ultimately result in enhanced cisplatin resistance and metastasis (Qin et al., 2019). Moreover, ubiquitin-specific protease 7 has been found to inhibit heterogeneous nuclear ribonucleoprotein A1 ubiquitination in CAFs (Zhang et al., 2020). miR-522-bearing EVs released from CAFs inhibit arachidonate lipoxygenase 15 expression and lipid peroxides accumulation, leading to enhanced chemoresistance in gastric cancer (Zhang et al., 2020). Thus, depletion of CAF-derived EVs causes improved chemosensitivity.
Regulation of Resistant Phenotype of Sensitive Cancer Cells by Tumor-Derived EVs
Emerging studies have confirmed that tumor-derived EVs play a vital role in the resistance of tumor cells to cancer therapy, including chemotherapy and radiotherapy (Table 2). Some drug-resistant tumor cells have the ability to confer a drug-resistant phenotype upon sensitive cells in an EVs-dependent manner. This may be due to EVs’ ability to mediate the transfer of miRNA, lncRNA and proteins associated with drug resistance to recipient cells (Corrado et al., 2013). For instance, paclitaxel-resistant gastric cancer cells have been reported to be rich in miR-155-5p (Wang M. et al., 2019). miR-155-5p can be delivered from resistant cancer cells to sensitive cells by EVs, thereby increasing the expression level of miR-155-5p in recipient cells. The increased miR-155-5p confers paclitaxel resistance and induces EMT in gastric cancer cells via inhibiting the expression of GATA3 and TP53INP1 (Wang M. et al., 2019). Similarly, miR-423-5p-bearing EVs induce the transformation of breast cancer cells from sensitive cells to cisplatin-resistant cells (Wang B. et al., 2019). While the above examples show that drug-resistant tumor-derived EVs can disseminate drug resistance via transferring increased miRNAs to recipient cells, it appears that EVs can also induce drug-resistance by decreased miRNAs. EVs released from cisplatin-resistant lung cancer cells down-regulate a total of 11 miRNAs, in which miR-100-5p is the most significantly down-regulated miRNA (Qin et al., 2017). The down-regulated miR-100-5p modulates the expression of the mammalian target of rapamycin in recipient cells, which induces a chemo-resistant phenotype upon NSCLC cells (Qin et al., 2017).
The expression of lncRNA H19 is up-regulated within EVs from gefitinib-resistant NSCLC cells (Lei et al., 2018). Vesicular lncRNA H19 can be transported to sensitive cells to induce gefitinib resistance (Lei et al., 2018). EVs isolated from trastuzumab-resistant HER2+ breast cancer increase the level of lncRNA-SNHG14, which can induce a chemo-resistant phenotype upon sensitive tumor cells (Dong et al., 2018). Besides mediating the transfer of miRNA or lncRNA to induce drug resistance, EVs have also been shown to deliver proteins to target cells to disseminate resistance. For instance, EVs released from patients with a poor response to chemotherapy up-regulate the expression of transient receptor potential channel 5 (TrpC5) (Ma et al., 2014). Relying on EVs, the increased TrpC5 can enter sensitive breast cancer cells to disseminate resistance. In addition, PKM2-bearing EVs from cisplatin-resistant tumor cells induce a chemo-resistant phenotype upon NSCLC cells by reprogramming CAFs transformation (Wang et al., 2021). Recently, vesicular transfer of annexin A6 has been found to confer gemcitabine-resistance in sensitive triple-negative breast cancer cells via suppressing and degrading of epidermal growth factor receptor (Li et al., 2021).
Additionally, the involvement of EVs in the resistance of tumor cells to radiotherapy has been reported. Early data demonstrated that the protein composition of tumor-derived EVs might be changed when exposed to radiation (Jelonek et al., 2015). Apart from affecting the composition of EVs, radiation has also been shown to affect the functions of EVs on target cells. EVs isolated from irradiated squamous head and neck cancer cells can confer radio-resistance in recipient cells via repairing damaged DNA content (Mutschelknaus et al., 2016). EVs isolated from hypoxic glioma are rich in miR-301a, which is associated with the resistance of tumor cells to radiotherapy (Yue et al., 2019). Mechanically, miR-301a-bearing EVs directly target TCEAL7 gene to induce radio-resistance in glioma cells and this effect can be reversed by inhibiting the Wnt/β-catenin pathway (Yue et al., 2019). Similarly, EVs released from irradiated cells can also reduce the sensitivity of recipient cells to the drug (Wu et al., 2018). Mechanically, EVs released from NSCLC cells can induce anaplastic lymphoma kinase inhibitors-resistant or Ceritinib-resistant phenotype upon target tumor cells via stimulating AKT, STAT3 and ERK pathways (Wu et al., 2018).
Tumor-Derived EVs Modulate the Immune System
Immune cells such as NK cells, macrophages, T cells and B cells can interact with tumor cells, resulting in their functions and phenotypes changes. The emerging report reveals that tumor-derived EVs are involved in remodeling the tumor immune microenvironment (Figure 3). Through releasing EVs, tumor cells can deliver immune-inhibitory and immune-stimulatory signaling biomolecular components to the tumor immune microenvironment, thus creating a protumoral or an anti-tumoral soil to influence cancer progression (Whiteside, 2016).
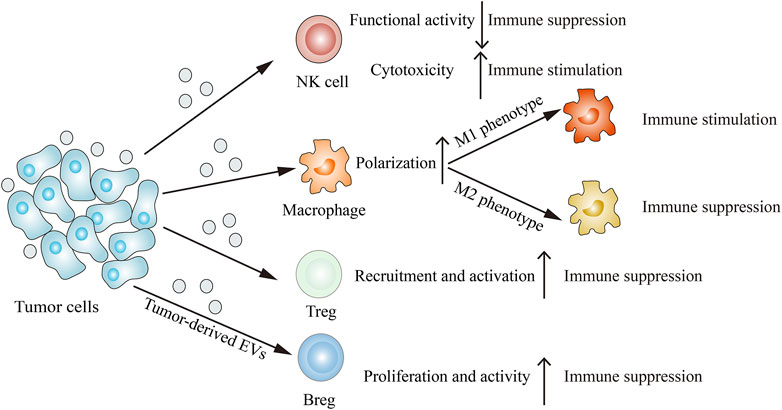
FIGURE 3. Tumor-derived EVs modulate the immune system. Tumor-derived EVs can suppress NK cells functional activity, induce M2 macrophage polarization, recruit Tregs and proliferate Bregs, thus inhibiting immune response. On the other hand, tumor-derived EVs can also enhance NK cells cytotoxicity and induce M1 macrophage polarization, thereby stimulating immune response.
NK Cells
NK cells, which play a key role in cancer immunotherapy, are the important subset of innate immune cells. Early research demonstrated that NK cell activity could be inhibited by breast cancer-derived EVs, which resulted in the accelerated growth of xenograft tumors (Liu et al., 2006). Researchers isolated NK cells from the spleens of BALB/c mice that had been pretreated with purified breast cancer-derived EVs and determined NK cell activity by the chromium release assays (Liu et al., 2006). Trials have shown that NK cell cytolytic activity was inhibited in mice by EVs released from TS/A tumor cells (Liu et al., 2006). Further study has demonstrated that pretreated mice with TS/A tumor cells-derived EVs would lead to a significant decrease in the total number and percentages of NK cells (Liu et al., 2006). Given the accumulating evidence for the role of EVs isolated from tumor cells in response to hypoxia, some researchers have investigated the potential of tumor-derived EVs under hypoxia conditions in reprogramming functions of NK cells. For instance, EVs secreted from tumor cells under hypoxia conditions show a more potent ability to impair the cytotoxicity of NK cells as compared with that from tumor cells under normoxia conditions (Berchem et al., 2016). In addition, the expression levels of functional activity markers such as CD107a and IFN-γ in NK cells pretreated with hypoxic tumor-derived EVs are significantly lower than those in NK cells pretreated with normoxic tumor-derived EVs (Berchem et al., 2016). This could be in part explained by the abundance of miR-23a in hypoxic tumor cells-derived EVs that could function as an additional immunosuppressive activator by directly targeting CD107a in NK cells (Berchem et al., 2016). On the other hand, EVs secreted from pancreatic cancer cells at the high-metastatic state also appear to down-regulate the expression of CD107a and IFN-γ in NK cells (Zhao et al., 2019). Furthermore, EVs isolated from pancreatic cancer patients contain abundant TGF-β1, which can attenuate CD107a and IFN-γ expression in NK cells (Zhao et al., 2019). Mechanically, TGF-β1-bearing EVs activate the TGF β-Smad2/3 pathway in NK cells to impair NK cell-mediated cytotoxicity (Zhao et al., 2019). Apart from inhibiting the cytotoxic activity of NK cells, tumor-derived EVs have also been shown to act as an inducer to stimulate effective NK cell anti-tumor response. For example, EVs isolated from resistant anti-cancer drug-treated HCC cells are able to stimulate the suppressive effects of NK cells on tumor cell proliferation (Lv et al., 2012). The reason for this phenomenon may be that EVs released from HCC cells treated with resistant anti-cancer drugs contain abundant heat shock proteins (HSPs), including HSP60, HSP70 and HSP90 (Lv et al., 2012). Notably, those resistant anti-cancer drugs promote HSP-bearing EVs release, thereby contributing to activating the cytotoxic response of NK cells (Lv et al., 2012).
Macrophages
Macrophages, as one part of innate immune systems, can be affected by many factors to switch their phenotype. Activated macrophages are commonly classified into two phenotypes, classical activation (M1) macrophages and alternative activation (M2) macrophages. M1 macrophages secrete pro-inflammatory cytokines to induce tumoricidal activity, while M2 macrophages secrete anti-inflammatory cytokines to promote tumorigenesis. Tumor-associated macrophages (TAMs) are the main immune cell population in TME, which can be educated by various tumor cells and display an M2-like phenotype to promote the development and progression of tumors. Nowadays, tumor-derived EVs are described as containing a variety of functional components and are now emerging as a key regulator of macrophage polarization. Those components, such as miRNA, lncRNAs and proteins, can be transferred to macrophages via EVs to switch their phenotype.
Epithelial ovarian cancer-derived EVs can induce macrophages to secrete anti-inflammatory cytokine IL-10, leading to enhanced tumor growth and metastasis (Ying et al., 2016). The expression level of vesicular miR-222-3p in epithelial ovarian cancer patients is markedly higher than that in healthy people (Ying et al., 2016). Increased miR-222-3p has the ability to inhibit SOCS3 expression and stimulate the SOCS3/STAT3 pathway in macrophages, thereby inducing TAM-like phenotype macrophages production in vitro and in vivo (Ying et al., 2016). In addition, hypoxic EVs isolated from tumor cells, including pancreatic cancer and glioma, can generate the M2-like phenotype macrophages (Wang et al., 2018b; Qian et al., 2020). miR-301a-3p is highly enriched in EVs isolated from PANC-1 and BxCP-3 pancreatic cancer cells cultured in hypoxia conditions (Wang et al., 2018b). Mechanically, vesicular miR-301a-3p down-regulates PTEN expression and subsequently activates the PI3Kγ pathway, resulting in increased expression of M2 macrophage marker like CD163 (Wang et al., 2018b). Furthermore, the knockdown of HIF-1a and HIF-2a in pancreatic cancer cells revealed that miR-301a-3p expression level under hypoxia conditions relied on HIF-1a and HIF-2a (Wang et al., 2018b). In glioma, miR-1246 is the most prominently increased content in hypoxic tumor-derived EVs as compared with that in normoxic tumor-derived EVs (Qian et al., 2020). The increased miR-1246 is considered as a key regulator in inducing M2 macrophage polarization, since it can activate the STA3T signaling pathway and suppress the NF-κB signaling pathway (Qian et al., 2020). Notably, the M2 macrophage is a pro-tumor phenotype that promotes tumor cells migration and invasion via facilitating the formation of the immunosuppressive microenvironment. Vesicular lncRNA BCRT1 from breast cancer cells enhances tumor cells migration and invasion (Liang et al., 2020). Injection of breast cancer cells into lncRNA BCRT1-overexpressing mice causes more and larger metastatic lung foci (Liang et al., 2020). In addition, M2 markers (CD206 and MRC-2) expression are shown to up-regulate when macrophages stimulated by EVs derived from lncRNA BCRT1-overexpressing breast cancer cells (Liang et al., 2020). Furthermore, the transfer of Rab22a-NeoF1 fusion protein from osteosarcoma cells to negative tumor cells via EVs contributes to the formation of pre-metastatic niche in osteosarcoma (Zhong et al., 2021). Rab22a-NeoF1 fusion protein recruits bone marrow-derived macrophages and subsequently induces M2 macrophage polarization via its binding partner PYK2 (Zhong et al., 2021). Quantitative real-time PCR (RT-qPCR) analysis showed lncRNA TUC339 was significantly enriched in tumor-derived EVs (Li et al., 2018). Knocking out TUC339 in macrophages resulted in elevated pro-inflammatory cytokines IL-1β and TNF-α (Li et al., 2018). In turn, pro-inflammatory cytokine production decreased in TUC339-overexpressing macrophages (Li et al., 2018). This reveals that the lncRNA TUC339 can serve as a regulator to modulate M2 polarization macrophages.
In addition to mediating transmit miRNA or lncRNA to induce M2 polarization macrophages, EVs have also been shown to deliver miRNA or protein to target cells, thus inducing M1 polarization macrophages. For instance, miR-21 is selectively enriched in EVs isolated from colorectal cancer, which correlates with the increased M1/M2 ratio (Shao et al., 2018). In addition, miR-21 mimic causes increased pro-inflammatory cytokine production in macrophage (Shao et al., 2018). Mechanically, vesicular miR-21 up-regulates IL-6 expression level in macrophages by directly binding to toll-like receptor (TLR) 7, thereby contributing to creating an inflammatory TME (Shao et al., 2018). miR-9 has been found to be markedly enriched in HPV + head and neck squamous cell carcinoma (Tong et al., 2020). In addition, vesicular miR-9 down-regulates PPARδ and subsequently induces M1 polarization macrophages, which consequently leads to enhanced tumor radiosensitivity (Tong et al., 2020). Moreover, EVs isolated from oral squamous cell carcinoma significantly up-regulate the expression levels of pro-inflammatory cytokines (IL-6, IL-1β and TNF-α), while exerting no effect on the expression levels of anti-inflammatory cytokines (IL-10, MRC1 and CCL18) (Xiao et al., 2018). This suggests THBS1 expression is closely correlated with the expression levels of M1 related cytokines. Mechanically, EVs isolated from oral squamous cell carcinoma induce M1 polarization macrophages via stimulating p38, AKT and SAPK/JNK signaling pathways (Xiao et al., 2018).
T Cells
T cells, including unactivated naive T cells and effector T cells activated by antigen, are the key regulators in the tumor immunity. Helper T cells and cytotoxic T cells are mainly involved in the tumor immunity, while regulatory T cells (Tregs) are mainly involved in tumor immune escape. Nowadays, tumor-derived EVs have been found to act as an immune suppressor to promote recruitment and activation of Tregs in the TME, thereby creating a pro-tumorigenesis environment for tumor progression. For instance, HCC cells-derived EVs have been demonstrated to mediate the delivery of 14-3-3ζ to tumor-infiltrating T cells, which suppresses the anti-tumor effects of T cells (Wang et al., 2018c). Vesicular 14-3-3ζ inhibits the activity and proliferation of peripheral blood T cells, which consequently contributes to deviating the transformation of naive T cells from effector T cells to Tregs (Wang et al., 2018c). In addition, vesicular miR-208b suppresses programmed cell death factor 4 in recipient CD4+ T cells and subsequently promotes Tregs proliferation, which consequently accelerates tumor growth in colorectal cancer (Ning et al., 2021). Nasopharyngeal carcinoma cells selectively up-regulate the transcription of CCL20, which serves as a Treg attractor (Mrizak et al., 2015). In addition, nasopharyngeal carcinoma-derived EVs have the ability to recruit Tregs into the TME and induce the transformation of T cells into Tregs, resulting in an enhanced immunosuppression effect in a dose-dependent manner (Mrizak et al., 2015). In addition to mediating Tregs recruitment in TME, tumor-derived EVs have also been shown to regulate the expression of the immune-related genes in Tregs. For example, mRNA profiles analysis revealed that EVs isolated from head and neck squamous cell carcinoma could up-regulate the expression of CD25, CD39, CD73 and CD26 in activated Tregs (Muller et al., 2016). Heat map analysis further found that tumor-derived EVs co-cultured with Tregs would lead to higher expression levels of adenosine-pathway genes and lower expression levels of immunoregulatory genes (Muller et al., 2016). Previous studies revealed that the adenosine pathway was one of the key mechanisms utilized by Tregs to function as an immunosuppressor (Whiteside et al., 2012; Whiteside and Jackson, 2013). This suggests tumor-derived EVs promote the suppression functions of Tregs via regulating the expression of adenosine-pathway genes. CD73+ γδT cells, serve as the main Tregs subset in breast cancer, are able to mediate immunosuppressive effect in an adenosine-dependent manner (Ni et al., 2020). In the context of breast cancer, the release of lncRNA SNHG16 from EVs is shown to regulate CD73 expression on γδT cells (Ni et al., 2020). Mechanically, vesicular lncRNA SNHG16 stimulates the TGF-β/SMAD5 pathway by targeting the SMAD5 gene, which up-regulates CD73 expression on γδT cells (Ni et al., 2020).
B Cells
B cells play a key role in humoral immunity on account of their abilities to produce immunoglobulin and present antigens. The regulatory B cells (Bregs) as a subset of B cells are correlated with immunosuppressive response. Similar to T cells, B cells can be induced into Bregs by tumor-derived EVs. For instance, HCC cells-derived EVs can induce TIM-1+ Breg with a high expression level of IL-10 (Ye et al., 2018). T cell co-culture with EVs-induced B cell results in decreased TNF-α and IFN-γ production (Ye et al., 2018). Notably, vesicular HMGB1 can activate the TLR-MAPK signaling pathway, which has been found to play a crucial role in inducing the transition of B cells into Bregs (Ye et al., 2018). Further study has demonstrated that blocking TLR or inhibiting MAPK can significantly suppress the Bregs expansion and up-regulate the production of pro-inflammatory cytokines (Ye et al., 2018). In addition, EVs isolated from esophageal squamous cell carcinoma patients significantly enhance the IL-10+ Breg production (Mao et al., 2019). Correspondingly, flow cytometry analysis showed that the expression levels of IL-10 and PD-1 in B cells were higher when B cells were co-cultured with tumor-derived EVs (Mao et al., 2019). As EVs commonly function via delivering biomolecular components to target cells, researchers further analyzed the mRNAs and lncRNAs composition in EVs. Results revealed that a total of 947 mRNAs and 175 lncRNAs were down-regulated, while a total of 407 mRNAs and 1,331 lncRNAs were up-regulated in EVs released from esophageal squamous cell carcinoma (Mao et al., 2019). Furthermore, EVs derived from head and neck squamous cell carcinoma directly suppress B cell proliferation and activity (Schroeder et al., 2020). Flow cytometry analysis showed that tumor-derived EVs could inhibit the expression of checkpoint receptors (GITR and BTLA) and CD39 on B cells (Schroeder et al., 2020). Notably, as a B cell activation marker, CD39 regulates adenosine production in many immune cells, thus influencing the immunosuppressive effect of B cells (Saze et al., 2013).
Immune Cell-Derived EVs
NK cell-derived EVs significantly enhance the apoptosis of aggressive melanoma (Zhu et al., 2017). Meanwhile, the transfer of miR-186 from NK cell to neuroblastoma cell via EVs inhibits tumor growth and reverses immune escape (Neviani et al., 2018). Most TAMs display an M2-like phenotype, and thus M2 macrophages are the predominant macrophage phenotype in the TME. M2 macrophage derive-EVs (M2-EVs) stimulate PI3k/AKT signaling pathway via enriched apolipoprotein E, leading to cytoskeleton remodeling in gastric cancer cells, and ultimately result in enhanced migration (Zheng et al., 2018). M2-EVs also enrich miR-233 under hypoxia conditions. Vesicular miR-233 affords drug resistance to cDDP in epithelial ovarian cancer cells by activating PTEN-PI3k/AKT signaling pathway (Zhu et al., 2019). Moreover, the transfer of miR-21-5p and miR-155-5p via EVs from M2 macrophages to colon cancer cells down-regulates BRG1 and consequently promotes tumor metastasis (Lan et al., 2018). By contrast, M1 macrophage-derived EVs potentiate therapeutic efficacy of gemcitabine by improving chemosensitivity of resistant pancreatic cancer cells (Zhao Y. et al., 2021). T cell-derived EVs containing programmed cell death 1 inhibit tumor cell immune escape by triggering programmed death-ligand1internalization (Qiu et al., 2021). In addition, CD4+ T cell-derived EVs potentiate vaccine-mediated immune responses by enhancing B cell proliferation and antibodies production (Lu et al., 2019). By contrast, B cell-derived EVs compromise chemotherapeutic effect by attenuating CD8+ T cell response (Zhang F. et al., 2019). Thus, inhibition of B cell-derived EVs release contributes to enhanced post-chemotherapeutic T cell responses (Zhang F. et al., 2019).
Tumor-Derived EVs as Biomarkers in Cancer Diagnosis and Treatment
Due to the lack of ideal biomarkers in the clinic, most cancer patients once diagnosed have been at the advanced stage. Tumor-derived EVs can be released into various body fluids like blood and ascites, which are able to reflect the status of the parental cancer cell. Therefore, tumor-derived EVs are considered as ideal candidates for non-invasive biomarkers in cancer diagnosis. The diversity and specificity of tumor-derived EVs, including miRNA, lncRNA and protein, enable their application in diagnosis (Table 3). For instance, EVs released from acute myeloid leukemia cell selectively enrich let-7a, miR-99b, miR-146a, miR-155, miR-191 and miR-1246 (Hornick et al., 2015). Moreover, RT-qPCR analysis showed that the concentrations of those increased miRNAs were 1000-fold above the cellular level, which may better distinguish acute myeloid leukemia from healthy volunteers with high sensitivity and specificity (Hornick et al., 2015). High levels of circular RNA SETDB1 and miR-31-5P are observed in lung adenocarcinoma patients (Xu et al., 2021; Yu et al., 2021). Serum vesicular circular RNA SETDB1 level is closely correlated with the T stage and lymph node metastasis (Xu et al., 2021). In addition, zinc finger antisense 1 (ZFAS1), belonging to competing endogenous lncRNA, is enriched in the serum EVs of gastric cancer patients (Pan et al., 2017). Highly expressed vesicular ZFAS1 may be related to a higher risk of lymphatic metastasis in gastric cancer patients (Pan et al., 2017). Glypican-1 (GPC1) is specifically up-regulated in tumor-derived EVs, thus detection of serum-derived EVs from pancreatic cancer patients distinguishes healthy individuals and patients with a benign pancreatic cancer from patients with early- and late-stage pancreatic cancer in a GPC1-dependent manner with specificity and sensitivity (Melo et al., 2015). In addition, contactin-1 is selectively elevated in plasma EVs of melanoma cancer patients when compared with EVs of normal volunteers (Pietrowska et al., 2021). This indicates that the detection of these differentially expressed proteins of melanoma cancer-derived EVs may play an essential role in the diagnosis and monitoring of tumors. The expression levels of let-7p-3b, miR-150-3p, miR-145-3p and miR-139-3p in plasma-derived EVs from colon cancer patients are much higher than those in plasma-derived EVs from healthy controls (Min et al., 2019). Moreover, EVs derived miRNAs show a more potent diagnosis efficacy than plasma total miRNAs. Recently, Xia et al. (2020) believe that miR-301a-3p is correlated to gastric cancer development and metastasis. In addition, miR-301a-3p is selectively enriched in serum EVs isolated from gastric cancer with peritoneal metastasis (Xia et al., 2020). Similarly, elevated miR-92a-3p expression level in plasma-derived EVs is related to metastasis of HCC patients (Yang et al., 2020). Also, vesicular lncHILAR expression is markedly higher in renal cancer patients with metastasis than those without metastasis (Hu et al., 2021). Yuan et al. (2021) believe that breast cancer patients with high vesicular miR-21 in serum also have bone metastasis. Furthermore, high vesicular HMGB3 level is observed in nasopharyngeal carcinoma patients, especially those with metastasis (Zhang K. et al., 2021).
In addition to acting as non-invasive biomarkers in cancer diagnosis, EVs have also been shown to serve as “real time” biomarkers during cancer treatment (Table 3). For instance, TrpC5 is a regulator of multidrug transporter P-glycoprotein, which promotes the generation of EVs. The expression level of vesicular TrpC5 in breast cancer patients with low drug sensitivity is significantly higher than that in healthy volunteers (Ma et al., 2014). As a result, detection of TrpC5-bearing EVs in peripheral blood of patients may predict the clinical treatment effect of chemotherapy. Similarly, annexin A6 overexpression relates to poor response to gemcitabine-based chemotherapy (Li et al., 2021). Ning et al. (2021) believe that the elevated miR-208b expression level is associated with oxaliplatin resistance in colorectal cancer patients. In addition, the expression level of vesicular miR-21-5p decreases in colorectal cancer patients after surgical resection (He et al., 2021). Recent studies have found that tumor-derived EVs may be a potential biomarker for cancer prognosis (Table 3). For instance, highly expressed vesicular S100A4 and osteopontin are related to short overall survival rates and disease free survival rates in HCC patients (Sun et al., 2021). Similarly, highly expressed vesicular lncRNA-SOX2OT are also related to short overall survival rates in NSCLC patients (Ni et al., 2021).
To better utilize these biomarkers in clinic, progress in EVs detection should be discussed. The conventional EVs detection methods conclude ultracentrifugation pretreatment and downstream western blotting, ELISA or PCR analysis. However, these techniques are time-consuming and insensitive (Lane et al., 2019). To overcome these limitations, plenty of micro and nano-devices have been exploited for detecting EVs. For example, Lewis et al. (2018) developed an Alternating Current Electrokinetic chip, which could capture and quantify vesicular GPC1 and CD63 within 30 min. Zhang P. et al. (2019) designed a fluorescence-based integrated platform called ExoProfile, which could elucidate the differences between the EVs of ovarian cancer patients and healthy people. Pang et al. (2020) used a surface enhanced Raman scattering method to elucidate the expression level of vesicular PD-L1 between NSCLC patients and healthy people. Recently, Marchisio et al. (2021) used a polychromatic flow cytometry technique to perform the detection of EVs captured by the lipophilic cationic dye. Thakur et al. (2021) presented a localized surface plasmon resonance (LSPR) technique to detect tumor-derived EVs using designed TiN-NH-LSPR biosensor and demonstrated that the label-free LSPR technique can be used for glioblastoma monitoring. Park et al. (2021) proposed a high-throughput electrochemical detection platform called HiMEX, which could distinguish colorectal cancer patients from healthy volunteers with high sensitivity and specificity.
Conclusion
In recent years, numerous studies of EVs have reported their participation in different stages during cancer progression. The delivery of intercellular information from tumor cells to stromal cells via EVs affects the functions and phenotypes of recipient cells, thereby regulating tumor progression. Herein we describe the key findings on how tumor-derived EVs remodel TME to influence tumor progression. In this regard, it is the multiple activators in EVs to create a protumoral or an anti-tumoral soil in the microenvironment. Moreover, such properties also enable their application in cancer diagnosis and treatment as an ideal candidate for non-invasive biomarkers. However, despite significant progress has been made in exploring the role of tumor-derived EVs in TME, many questions remain. Firstly, current studies on tumor-derived EVs use highly heterogeneous cells composed of multiple clones. Therefore, the functions of EVs released from single-cell have yet to be unveiled. Secondly, the enhanced techniques for EVs detection are sensitive and precise, but they also require expensive modification. Thus, highly precise, low-cost and simple techniques for clinical samples detection remain to be exploited. Thirdly, it remains unclear what the main components are at work. This field is in urgent need of more precise characterization of EVs cargo and biology.
Author Contributions
LW, YX, and WC provided the direction and guidance throughout the preparation of this manuscript. QB conducted the literature review and drafted the manuscript. QH, YC, RS, and QW edited the manuscript. All authors have read and agreed to the published version of the manuscript.
Funding
This project was financially supported by the National Natural Science Foundation of China (81773988, 82073923) and the Project of Natural Science Research in Universities of Anhui Province (KJ 2019A0314).
Conflict of Interest
The authors declare that the research was conducted in the absence of any commercial or financial relationships that could be construed as a potential conflict of interest.
Publisher’s Note
All claims expressed in this article are solely those of the authors and do not necessarily represent those of their affiliated organizations, or those of the publisher, the editors and the reviewers. Any product that may be evaluated in this article, or claim that may be made by its manufacturer, is not guaranteed or endorsed by the publisher.
References
Agudiez, M., Martinez, P. J., Martin-Lorenzo, M., Heredero, A., Santiago-Hernandez, A., Molero, D., et al. (2020). Analysis of Urinary Exosomal Metabolites Identifies Cardiovascular Risk Signatures with Added Value to Urine Analysis. BMC Biol. 18, 192. doi:10.1186/s12915-020-00924-y
Baietti, M. F., Zhang, Z., Mortier, E., Melchior, A., Degeest, G., Geeraerts, A., et al. (2012). Syndecan-Syntenin-ALIX Regulates the Biogenesis of Exosomes. Nat. Cel Biol. 14, 677–685. doi:10.1038/ncb2502
Bao, L., You, B., Shi, S., Shan, Y., Zhang, Q., Yue, H., et al. (2018). Metastasis-Associated miR-23a from Nasopharyngeal Carcinoma-Derived Exosomes Mediates Angiogenesis by Repressing a Novel Target Gene TSGA10. Oncogene 37, 2873–2889. doi:10.1038/s41388-018-0183-6
Bayraktar, R., Van Roosbroeck, K., and Calin, G. A. (2017). Cell‐to‐Cell Communication: microRNAs as Hormones. Mol. Oncol. 11, 1673–1686. doi:10.1002/1878-0261.12144
Berchem, G., Noman, M. Z., Bosseler, M., Paggetti, J., Baconnais, S., Le Cam, E., et al. (2016). Hypoxic Tumor-Derived Microvesicles Negatively Regulate NK Cell Function by a Mechanism Involving TGF-β and miR23a Transfer. Oncoimmunology 5, e1062968. doi:10.1080/2162402x.2015.1062968
Burnouf, T., Agrahari, V., and Agrahari, V. (2019). Extracellular Vesicles as Nanomedicine: Hopes and Hurdles in Clinical Translation. Int. J. Nanomedicine 14, 8847–8859. doi:10.2147/IJN.S225453
Chen, X., Zhou, J., Li, X., Wang, X., Lin, Y., and Wang, X. (2018). Exosomes Derived from Hypoxic Epithelial Ovarian Cancer Cells Deliver microRNAs to Macrophages and Elicit a Tumor-Promoted Phenotype. Cancer Lett. 435, 80–91. doi:10.1016/j.canlet.2018.08.001
Choi, D., Lee, T. H., Spinelli, C., Chennakrishnaiah, S., D’Asti, E., and Rak, J. (2017). Extracellular Vesicle Communication Pathways as Regulatory Targets of Oncogenic Transformation. Semin. Cel Develop. Biol. 67, 11–22. doi:10.1016/j.semcdb.2017.01.003
Colombo, M., Moita, C., van Niel, G., Kowal, J., Vigneron, J., Benaroch, P., et al. (2013). Analysis of ESCRT Functions in Exosome Biogenesis, Composition and Secretion Highlights the Heterogeneity of Extracellular Vesicles. J. Cel Sci. 126, 5553–5565. doi:10.1242/jcs.128868
Corrado, C., Raimondo, S., Chiesi, A., Ciccia, F., De Leo, G., and Alessandro, R. (2013). Exosomes as Intercellular Signaling Organelles Involved in Health and Disease: Basic Science and Clinical Applications. Int. J. Mol. Sci. 14, 5338–5366. doi:10.3390/ijms14035338
Del Conde, I., Shrimpton, C. N., Thiagarajan, P., and López, J. A. (2005). Tissue-factor-bearing Microvesicles Arise from Lipid Rafts and Fuse with Activated Platelets to Initiate Coagulation. Blood 106, 1604–1611. doi:10.1182/blood-2004-03-1095
Dong, H., Wang, W., Chen, R., Zhang, Y., Zou, K., Ye, M., et al. (2018). Exosome-Mediated Transfer of lncRNA-SNHG14 Promotes Trastuzumab Chemoresistance in Breast Cancer. Int. J. Oncol. 53, 1013–1026. doi:10.3892/ijo.2018.4467
Dörsam, B., Bösl, T., Reiners, K. S., Barnert, S., Schubert, R., Shatnyeva, O., et al. (2018). Hodgkin Lymphoma-Derived Extracellular Vesicles Change the Secretome of Fibroblasts Toward a CAF Phenotype. Front. Immunol. 9, 1358. doi:10.3389/fimmu.2018.01358
Egea-Jimenez, A. L., and Zimmermann, P. (2018). Phospholipase D and Phosphatidic Acid in the Biogenesis and Cargo Loading of Extracellular Vesicles. J. Lipid Res. 59, 1554–1560. doi:10.1194/jlr.R083964
Fang, T., Lv, H., Lv, G., Li, T., Wang, C., Han, Q., et al. (2018). Tumor-Derived Exosomal miR-1247-3p Induces Cancer-Associated Fibroblast Activation to foster Lung Metastasis of Liver Cancer. Nat. Commun. 9, 191. doi:10.1038/s41467-017-02583-0
French, K. C., Antonyak, M. A., and Cerione, R. A. (2017). Extracellular Vesicle Docking at the Cellular Port: Extracellular Vesicle Binding and Uptake. Semin. Cel Develop. Biol. 67, 48–55. doi:10.1016/j.semcdb.2017.01.002
Gamage, T. K. J. B., and Fraser, M. (2021). The Role of Extracellular Vesicles in the Developing Brain: Current Perspective and Promising Source of Biomarkers and Therapy for Perinatal Brain Injury. Front. Neurosci. 15, 744840. doi:10.3389/fnins.2021.744840
Giusti, I., Francesco, M. D., Ascenzo, S. D., Palmerini, M. G., Macchiarelli, G., Carta, G., et al. (2018). Ovarian Cancer-Derived Extracellular Vesicles Affect normal Human Fibroblast Behavior. Cancer Biol. Ther. 19, 1–44. doi:10.1080/15384047.2018.1451286
Gon, Y., Maruoka, S., Inoue, T., Kuroda, K., Yamagishi, K., Kozu, Y., et al. (2017). Selective Release of miRNAs via Extracellular Vesicles Is Associated with House-Dust Mite Allergen-Induced Airway Inflammation. Clin. Exp. Allergy 47, 1586–1598. doi:10.1111/cea.13016
He, Q., Ye, A., Ye, W., Liao, X., Qin, G., Xu, Y., et al. (2021). Cancer-Secreted Exosomal miR-21-5p Induces Angiogenesis and Vascular Permeability by Targeting KRIT1. Cell Death Dis 12, 576. doi:10.1038/s41419-021-03803-8
Henne, W. M., Buchkovich, N. J., and Emr, S. D. (2011). The ESCRT Pathway. Develop. Cel 21, 77–91. doi:10.1016/j.devcel.2011.05.015
Hornick, N. I., Huan, J., Doron, B., Goloviznina, N. A., Lapidus, J., Chang, B. H., et al. (2015). Serum Exosome MicroRNA as a Minimally-Invasive Early Biomarker of AML. Sci. Rep. 5, 11295. doi:10.1038/srep11295
Hsu, Y.-L., Hung, J.-Y., Chang, W.-A., Lin, Y.-S., Pan, Y.-C., Tsai, P.-H., et al. (2017). Hypoxic Lung Cancer-Secreted Exosomal miR-23a Increased Angiogenesis and Vascular Permeability by Targeting Prolyl Hydroxylase and Tight junction Protein ZO-1. Oncogene 36, 4929–4942. doi:10.1038/onc.2017.105
Hu, G., Ma, J., Zhang, J., Chen, Y., Liu, H., Huang, Y., et al. (2021). Hypoxia-Induced lncHILAR Promotes Renal Cancer Metastasis via ceRNA for the miR-613/206/1-1-3p/Jagged-1/Notch/CXCR4 Signaling Pathway. Mol. Ther. 29, 2979–2994. doi:10.1016/j.ymthe.2021.05.020
Hu, J. L., Wang, W., Lan, X. L., Zeng, Z. C., Liang, Y. S., Yan, Y. R., et al. (2019). CAFs Secreted Exosomes Promote Metastasis and Chemotherapy Resistance by Enhancing Cell Stemness and Epithelial-Mesenchymal Transition in Colorectal Cancer. Mol. Cancer 18, 91. doi:10.1186/s12943-019-1019-x
Huang, M., Chen, M., Qi, M., Ye, G., Pan, J., Shi, C., et al. (2021). Perivascular Cell‐derived Extracellular Vesicles Stimulate Colorectal Cancer Revascularization after Withdrawal of Antiangiogenic Drugs. J. Extracellular Vesicles 10, e12096. doi:10.1002/jev2.12096
Huang, Z., and Feng, Y. (2017). Exosomes Derived from Hypoxic Colorectal Cancer Cells Promote Angiogenesis Through Wnt4-Induced β-Catenin Signaling in Endothelial Cells. Oncol. Res. 25, 651–661. doi:10.3727/096504016x14752792816791
Inman, G. J. (2011). Switching TGFβ from a Tumor Suppressor to a Tumor Promoter. Curr. Opin. Genet. Develop. 21, 93–99. doi:10.1016/j.gde.2010.12.004
Jelonek, K., Wojakowska, A., Marczak, L., Muer, A., Tinhofer-Keilholz, I., Lysek-Gladysinska, M., et al. (2015). Ionizing Radiation Affects Protein Composition of Exosomes Secreted In Vitro from Head and Neck Squamous Cell Carcinoma. Acta Biochim. Pol. 62, 265–272. doi:10.18388/abp.2015_970
Jin, M.-Z., and Jin, W.-L. (2020). The Updated Landscape of Tumor Microenvironment and Drug Repurposing. Sig Transduct Target. Ther. 5, 166. doi:10.1038/s41392-020-00280-x
Johnstone, R. M., Adam, M., Hammond, J. R., Orr, L., and Turbide, C. (1987). Vesicle Formation during Reticulocyte Maturation. Association of Plasma Membrane Activities with Released Vesicles (Exosomes). J. Biol. Chem. 262, 9412–9420. doi:10.1016/s0021-9258(18)48095-7
Kucharzewska, P., Christianson, H. C., Welch, J. E., Svensson, K. J., Fredlund, E., Ringner, M., et al. (2013). Exosomes Reflect the Hypoxic Status of Glioma Cells and Mediate Hypoxia-dependent Activation of Vascular Cells during Tumor Development. Proc. Natl. Acad. Sci. 110, 7312–7317. doi:10.1073/pnas.1220998110
Lan, J., Sun, L., Xu, F., Liu, L., Hu, F., Song, D., et al. (2018). M2 Macrophage-Derived Exosomes Promote Cell Migration and Invasion in colon Cancer. Cancer Res. 79, 146–158. doi:10.1158/0008-5472.CAN-18-0014
Lane, R. E., Korbie, D., Trau, M., and Hill, M. M. (2019). Optimizing Size Exclusion Chromatography for Extracellular Vesicle Enrichment and Proteomic Analysis from Clinically Relevant Samples. Proteomics 19, e1800156. doi:10.1002/pmic.201800156
Larrea, E., Sole, C., Manterola, L., Goicoechea, I., Armesto, M., Arestin, M., et al. (2016). New Concepts in Cancer Biomarkers: Circulating miRNAs in Liquid Biopsies. Int. J. Mol. Sci. 17, 627. doi:10.3390/ijms17050627
Lee, K., Park, H., Lim, E. H., and Lee, K. W. (2012). Exosomes from Breast Cancer Cells Can Convert Adipose Tissue-Derived Mesenchymal Stem Cells into Myofibroblast-like Cells. Int. J. Oncol. 40, 130–138. doi:10.3892/ijo.2011.1193
Lei, Y., Guo, W., Chen, B., Chen, L., Gong, J., and Li, W. (2018). Tumor-Released lncRNA H19 Promotes Gefitinib Resistance via Packaging into Exosomes in Non-Small Cell Lung Cancer. Oncol. Rep. 40, 3438–3446. doi:10.3892/or.2018.6762
Lewis, J. M., Vyas, A. D., Qiu, Y., Messer, K. S., White, R., and Heller, M. J. (2018). Integrated Analysis of Exosomal Protein Biomarkers on Alternating Current Electrokinetic Chips Enables Rapid Detection of Pancreatic Cancer in Patient Blood. ACS Nano 12, 3311–3320. doi:10.1021/acsnano.7b08199
Li, B., Antonyak, M. A., Zhang, J., and Cerione, R. A. (2012). RhoA Triggers a Specific Signaling Pathway that Generates Transforming Microvesicles in Cancer Cells. Oncogene 31, 4740–4749. doi:10.1038/onc.2011.636
Li, T., Tao, Z., Zhu, Y., Liu, X., Wang, L., Du, Y., et al. (2021). Exosomal Annexin A6 Induces Gemcitabine Resistance by Inhibiting Ubiquitination and Degradation of EGFR in Triple-Negative Breast Cancer. Cel Death Dis 12, 684. doi:10.1038/s41419-021-03963-7
Li, X., Lei, Y., Wu, M., and Li, N. (2018). Regulation of Macrophage Activation and Polarization by HCC-Derived Exosomal lncRNA TUC339. Int. J. Mol. Sci. 19, 2958. doi:10.3390/ijms19102958
Liang, Y., Song, X., Li, Y., Chen, B., Zhao, W., Wang, L., et al. (2020). LncRNA BCRT1 Promotes Breast Cancer Progression by Targeting miR-1303/PTBP3 Axis. Mol. Cancer 19, 85. doi:10.1186/s12943-020-01206-5
Lin, X.-J., Chong, Y., Guo, Z.-W., Xie, C., Yang, X.-J., Zhang, Q., et al. (2015). A Serum microRNA Classifier for Early Detection of Hepatocellular Carcinoma: A Multicentre, Retrospective, Longitudinal Biomarker Identification Study with a Nested Case-Control Study. Lancet Oncol. 16, 804–815. doi:10.1016/S1470-2045(15)00048-0
Lin, X.-J., Fang, J.-H., Yang, X.-J., Zhang, C., Yuan, Y., Zheng, L., et al. (2018). Hepatocellular Carcinoma Cell-Secreted Exosomal MicroRNA-210 Promotes Angiogenesis In Vitro and In Vivo. Mol. Ther. - Nucleic Acids 11, 243–252. doi:10.1016/j.omtn.2018.02.014
Liu, C., Yu, S., Zinn, K., Wang, J., Zhang, L., Jia, Y., et al. (2006). Murine Mammary Carcinoma Exosomes Promote Tumor Growth by Suppression of NK Cell Function. J. Immunol. 176, 1375–1385. doi:10.4049/jimmunol.176.3.1375
Lokau, J., Schoeder, V., Haybaeck, J., and Garbers, C. (2019). Jak-Stat Signaling Induced by Interleukin-6 Family Cytokines in Hepatocellular Carcinoma. Cancers 11, 1704. doi:10.3390/cancers11111704
Lu, J., Wu, J., Xie, F., Tian, J., Tang, X., Guo, H., et al. (2019). CD4 + T Cell‐Released Extracellular Vesicles Potentiate the Efficacy of the HBsAg Vaccine by Enhancing B Cell Responses. Adv. Sci. 6, 1802219. doi:10.1002/advs.201802219
Lv, L.-H., Wan, Y.-L., Lin, Y., Zhang, W., Yang, M., Li, G.-L., et al. (2012). Anticancer Drugs Cause Release of Exosomes with Heat Shock Proteins from Human Hepatocellular Carcinoma Cells that Elicit Effective Natural Killer Cell Antitumor Responses In Vitro. J. Biol. Chem. 287, 15874–15885. doi:10.1074/jbc.M112.340588
Ma, X., Li, Z., Li, T., Zhu, L., Li, Z., and Tian, N. (2017). Long Non-Coding RNA HOTAIR Enhances Angiogenesis by Induction of VEGFA Expression in Glioma Cells and Transmission to Endothelial Cells via Glioma Cell Derived-Extracellular Vesicles. Am. J. Transl. Res. 9, 5012–5021.
Ma, X., Chen, Z., Hua, D., He, D., Wang, L., Zhang, P., et al. (2014). Essential Role for TrpC5-Containing Extracellular Vesicles in Breast Cancer with Chemotherapeutic Resistance. Proc. Natl. Acad. Sci. 111, 6389–6394. doi:10.1073/pnas.1400272111
Mao, Y., Wang, Y., Dong, L., Zhang, Q., Wang, C., Zhang, Y., et al. (2019). Circulating Exosomes from Esophageal Squamous Cell Carcinoma Mediate the Generation of B10 and PD ‐1 High Breg Cells. Cancer Sci. 110, 2700–2710. doi:10.1111/cas.14122
Marchisio, M., Simeone, P., Bologna, G., Ercolino, E., Pierdomenico, L., Pieragostino, D., et al. (2021). Flow Cytometry Analysis of Circulating Extracellular Vesicle Subtypes from Fresh Peripheral Blood Samples. Int. J. Mol. Sci. 22, 48. doi:10.3390/ijms22010048
Mathieu, M., Martin-Jaular, L., Lavieu, G., and Théry, C. (2019). Specificities of Secretion and Uptake of Exosomes and Other Extracellular Vesicles for Cell-To-Cell Communication. Nat. Cel Biol. 21, 9–17. doi:10.1038/s41556-018-0250-9
Mathivanan, S., Fahner, C. J., Reid, G. E., and Simpson, R. J. (2012). ExoCarta 2012: Database of Exosomal Proteins, RNA and Lipids. Nucleic Acids Res. 40, D1241–D1244. doi:10.1093/nar/gkr828
Melo, S. A., Luecke, L. B., Kahlert, C., Fernandez, A. F., Gammon, S. T., Kaye, J., et al. (2015). Glypican-1 Identifies Cancer Exosomes and Detects Early Pancreatic Cancer. Nature 523, 177–182. doi:10.1038/nature14581
Menck, K., Sönmezer, C., Worst, T. S., Schulz, M., Dihazi, G. H., Streit, F., et al. (2017). Neutral Sphingomyelinases Control Extracellular Vesicles Budding from the Plasma Membrane. J. Extracellular Vesicles 6, 1378056. doi:10.1080/20013078.2017.1378056
Milane, L., Singh, A., Mattheolabakis, G., Suresh, M., and Amiji, M. M. (2015). Exosome Mediated Communication within the Tumor Microenvironment. J. Controlled Release 219, 278–294. doi:10.1016/j.jconrel.2015.06.029
Min, L., Zhu, S., Chen, L., Liu, X., Wei, R., Zhao, L., et al. (2019). Evaluation of Circulating Small Extracellular Vesicles Derived miRNAs as Biomarkers of Early colon Cancer: a Comparison with Plasma Total miRNAs. J. Extracellular Vesicles 8, 1643670. doi:10.1080/20013078.2019.1643670
Morrissey, S. M., Zhang, F., Ding, C., Montoya-Durango, D. E., Hu, X., Yang, C., et al. (2021). Tumor-derived Exosomes Drive Immunosuppressive Macrophages in a Pre-Metastatic Niche through Glycolytic Dominant Metabolic Reprogramming. Cel Metab. 33, 2040–2058. doi:10.1016/j.cmet.2021.09.002
Mouw, J. K., Ou, G., and Weaver, V. M. (2014). Extracellular Matrix Assembly: A Multiscale Deconstruction. Nat. Rev. Mol. Cel Biol. 15, 771–785. doi:10.1038/nrm3902
Mrizak, D., Martin, N., Barjon, C., Jimenez-Pailhes, A.-S., Mustapha, R., Niki, T., et al. (2015). Effect of Nasopharyngeal Carcinoma-Derived Exosomes on Human Regulatory T Cells. J. Natl. Cancer Inst. 107, 363. doi:10.1093/jnci/dju363
Muller, L., Mitsuhashi, M., Simms, P., Gooding, W. E., and Whiteside, T. L. (2016). Tumor-Derived Exosomes Regulate Expression of Immune Function-Related Genes in Human T Cell Subsets. Sci. Rep. 6, 20254. doi:10.1038/srep20254
Mutschelknaus, L., Peters, C., Winkler, K., Yentrapalli, R., Heider, T., Atkinson, M. J., et al. (2016). Exosomes Derived from Squamous Head and Neck Cancer Promote Cell Survival after Ionizing Radiation. PLoS ONE 11, e0152213. doi:10.1371/journal.pone.0152213
Naing, A., Infante, J. R., Papadopoulos, K. P., Chan, I. H., Shen, C., Ratti, N. P., et al. (2018). PEGylated IL-10 (Pegilodecakin) Induces Systemic Immune Activation, CD8+ T Cell Invigoration and Polyclonal T Cell Expansion in Cancer Patients. Cancer Cell 34, 775–791. doi:10.1016/j.ccell.2018.10.007
Neviani, P., Wise, P. M., Murtadha, M., Liu, C. W., Wu, C.-H., Jong, A. Y., et al. (2018). Natural Killer-Derived Exosomal miR-186 Inhibits Neuroblastoma Growth and Immune Escape Mechanisms. Cancer Res. 79, 1151–1164. doi:10.1158/0008-5472.CAN-18-0779
Ni, C., Fang, Q.-Q., Chen, W.-Z., Jiang, J.-X., Jiang, Z., Ye, J., et al. (2020). Breast Cancer-Derived Exosomes Transmit lncRNA SNHG16 to Induce CD73+γδ1 Treg Cells. Sig Transduct Target. Ther. 5, 41. doi:10.1038/s41392-020-0129-7
Ni, J., Zhang, X., Li, J., Zheng, Z., Zhang, J., Zhao, W., et al. (2021). Tumour-Derived Exosomal lncRNA-Sox2ot Promotes Bone Metastasis of Non-Small Cell Lung Cancer by Targeting the miRNA-194-5p/RAC1 Signalling axis in Osteoclasts. Cel Death Dis 12, 662. doi:10.1038/s41419-021-03928-w
Ning, T., Li, J., He, Y., Zhang, H., Wang, X., Deng, T., et al. (2021). Exosomal miR-208b Related with Oxaliplatin Resistance Promotes Treg Expansion in Colorectal Cancer. Mol. Ther. 29, 2723–2736. doi:10.1016/j.ymthe.2021.04.028
Ning, X., Zhang, H., Wang, C., and Song, X. (2018). Exosomes Released by Gastric Cancer Cells Induce Transition of Pericytes into Cancer-Associated Fibroblasts. Med. Sci. Monit. 24, 2350–2359. doi:10.12659/msm.906641
Ostrowski, M., Carmo, N. B., Krumeich, S., Fanget, I., Raposo, G., Savina, A., et al. (2010). Rab27a and Rab27b Control Different Steps of the Exosome Secretion Pathway. Nat. Cel Biol. 12, 19–30. doi:10.1038/ncb2000
Paggetti, J., Haderk, F., Seiffert, M., Janji, B., Distler, U., Ammerlaan, W., et al. (2015). Exosomes Released by Chronic Lymphocytic Leukemia Cells Induce the Transition of Stromal Cells into Cancer-Associated Fibroblasts. Blood 126, 1106–1117. doi:10.1182/blood-2014-12-618025
Pan, L., Liang, W., Fu, M., Huang, Z.-h., Li, X., Zhang, W., et al. (2017). Exosomes-Mediated Transfer of Long Noncoding RNA ZFAS1 Promotes Gastric Cancer Progression. J. Cancer Res. Clin. Oncol. 143, 991–1004. doi:10.1007/s00432-017-2361-2
Pang, Y., Shi, J., Yang, X., Wang, C., Sun, Z., and Xiao, R. (2020). Personalized Detection of Circling Exosomal PD-L1 Based on Fe3O4@TiO2 Isolation and SERS Immunoassay. Biosens. Bioelectron. 148, 111800. doi:10.1016/j.bios.2019.111800
Park, J., Park, J. S., Huang, C.-H., Jo, A., Cook, K., Wang, R., et al. (2021). An Integrated Magneto-Electrochemical Device for the Rapid Profiling of Tumour Extracellular Vesicles from Blood Plasma. Nat. Biomed. Eng. 5, 678–689. doi:10.1038/s41551-021-00752-7
Parks, S. K., Cormerais, Y., and Pouysségur, J. (2017). Hypoxia and Cellular Metabolism in Tumour Pathophysiology. J. Physiol. 595, 2439–2450. doi:10.1113/JP273309
Pasvenskaite, A., Liutkeviciene, R., Gedvilaite, G., Vilkeviciute, A., Liutkevicius, V., and Uloza, V. (2021). Impact of IL-10 Promoter Polymorphisms and IL-10 Serum Levels on Advanced Laryngeal Squamous Cell Carcinoma and Survival Rate. Cancer Genomics Proteomics 18, 53–65. doi:10.21873/cgp.20241
Pietrowska, M., Zebrowska, A., Gawin, M., Marczak, L., Sharma, P., Mondal, S., et al. (2021). Proteomic Profile of Melanoma Cell‐derived Small Extracellular Vesicles in Patients' Plasma: a Potential Correlate of Melanoma Progression. J. Extracellular Vesicles 10, e12063. doi:10.1002/jev2.12063
Qian, M., Wang, S., Guo, X., Wang, J., Zhang, Z., Qiu, W., et al. (2020). Hypoxic Glioma-Derived Exosomes Deliver microRNA-1246 to Induce M2 Macrophage Polarization by Targeting TERF2IP via the STAT3 and NF-Κb Pathways. Oncogene 39, 428–442. doi:10.1038/s41388-019-0996-y
Qin, X., Guo, H., Wang, X., Zhu, X., Yan, M., Wang, X., et al. (2019). Exosomal miR-196a Derived from Cancer-Associated Fibroblasts Confers Cisplatin Resistance in Head and Neck Cancer through Targeting CDKN1B and ING5. Genome Biol. 20, 12. doi:10.1186/s13059-018-1604-0
Qin, X., Yu, S., Zhou, L., Shi, M., Hu, Y., Xu, X., et al. (2017). Cisplatin-Resistant Lung Cancer Cell–Derived Exosomes Increase Cisplatin Resistance of Recipient Cells in Exosomal miR-100–5p-Dependent Manner. Int. J. Nanomedicine 12, 3721–3733. doi:10.2147/ijn.s131516
Qiu, Y., Yang, Y., Yang, R., Liu, C., Hsu, J.-M., Jiang, Z., et al. (2021). Activated T Cell-Derived Exosomal PD-1 Attenuates PD-L1-Induced Immune Dysfunction in Triple-Negative Breast Cancer. Oncogene 40, 4992–5001. doi:10.1038/s41388-021-01896-1
Ramteke, A., Ting, H., Agarwal, C., Mateen, S., Somasagara, R., Hussain, A., et al. (2015). Exosomes Secreted under Hypoxia Enhance Invasiveness and Stemness of Prostate Cancer Cells by Targeting Adherens Junction Molecules. Mol. Carcinog. 54, 554–565. doi:10.1002/mc.22124
Savina, A., Vidal, M., and Colombo, M. I. (2002). The Exosome Pathway in K562 Cells Is Regulated by Rab11. J. Cel Sci. 115, 2505–2515. doi:10.1242/jcs.115.12.2505
Saze, Z., Schuler, P. J., Hong, C.-S., Cheng, D., Jackson, E. K., and Whiteside, T. L. (2013). Adenosine Production by Human B Cells and B Cell-Mediated Suppression of Activated T Cells. Blood 122, 9–18. doi:10.1182/blood-2013-02-482406
Schroeder, J. C., Puntigam, L., Hofmann, L., Jeske, S. S., Beccard, I. J., Doescher, J., et al. (2020). Circulating Exosomes Inhibit B Cell Proliferation and Activity. Cancers 12, 2110. doi:10.3390/cancers12082110
Shang, A., Gu, C., Wang, W., Wang, X., Sun, J., Zeng, B., et al. (2020). Exosomal circPACRGL Promotes Progression of Colorectal Cancer via the miR-142-3p/miR-506-3p- TGF-β1 axis. Mol. Cancer 19, 117. doi:10.1186/s12943-020-01235-0
Shao, Y., Chen, T., Zheng, X., Yang, S., Xu, K., Chen, X., et al. (2018). Colorectal Cancer-Derived Small Extracellular Vesicles Establish an Inflammatory Premetastatic Niche in Liver Metastasis. Carcinogenesis 39, 1368–1379. doi:10.1093/carcin/bgy115
Small, H. Y., Montezano, A. C., Rios, F. J., Savoia, C., and Touyz, R. M. (2014). Hypertension Due to Antiangiogenic Cancer Therapy with Vascular Endothelial Growth Factor Inhibitors: Understanding and Managing a New Syndrome. Can. J. Cardiol. 30, 534–543. doi:10.1016/j.cjca.2014.02.011
Song, L., Tang, S., Han, X., Jiang, Z., Dong, L., Liu, C., et al. (2019). KIBRA Controls Exosome Secretion via Inhibiting the Proteasomal Degradation of Rab27a. Nat. Commun. 10, 1639. doi:10.1038/s41467-019-09720-x
Stuffers, S., Sem Wegner, C., Stenmark, H., and Brech, A. (2009). Multivesicular Endosome Biogenesis in the Absence of ESCRTs. Traffic 10, 925–937. doi:10.1111/j.1600-0854.2009.00920.x
Sükei, T., Palma, E., and Urbani, L. (2021). Interplay between Cellular and Non-Cellular Components of the Tumour Microenvironment in Hepatocellular Carcinoma. Cancers 13, 5586. doi:10.3390/cancers13215586
Sun, H., Wang, C., Hu, B., Gao, X., Zou, T., Luo, Q., et al. (2021). Exosomal S100A4 Derived from Highly Metastatic Hepatocellular Carcinoma Cells Promotes Metastasis by Activating STAT3. Sig Transduct Target. Ther. 6, 187. doi:10.1038/s41392-021-00579-3
Taylor, D. D., Zacharias, W., and Gercel-Taylor, C. (2011). Exosome Isolation for Proteomic Analyses and RNA Profiling. Methods Mol. Biol. 728, 235–246. doi:10.1007/978-1-61779-068-3_15
Thakur, A., Xu, C., Li, W. K., Qiu, G., He, B., Ng, S.-P., et al. (2021). In Vivo liquid Biopsy for Glioblastoma Malignancy by the AFM and LSPR Based Sensing of Exosomal CD44 and CD133 in a Mouse Model. Biosens. Bioelectron. 191, 113476. doi:10.1016/j.bios.2021.113476
Theos, A. C., Truschel, S. T., Tenza, D., Hurbain, I., Harper, D. C., Berson, J. F., et al. (2006). A Lumenal Domain-dependent Pathway for Sorting to Intralumenal Vesicles of Multivesicular Endosomes Involved in Organelle Morphogenesis. Develop. Cel 10, 343–354. doi:10.1016/j.devcel.2006.01.012
Théry, C., Witwer, K. W., Aikawa, E., Alcaraz, M. J., Anderson, J. D., Andriantsitohaina, R., et al. (2018). Minimal Information for Studies of Extracellular Vesicles 2018 (MISEV2018): a Position Statement of the International Society for Extracellular Vesicles and Update of the MISEV2014 Guidelines. J. Extracell. Vesicles 7, 1535750. doi:10.1080/20013078.2018.1535750
Tian, X., Liu, Y., Wang, Z., and Wu, S. (2021). miR-144 Delivered by Nasopharyngeal Carcinoma-Derived EVs Stimulates Angiogenesis through the FBXW7/HIF-1α/VEGF-A Axis. Mol. Ther. - Nucleic Acids 24, 1000–1011. doi:10.1016/j.omtn.2021.03.016
Tong, F., Mao, X., Zhang, S., Xie, H., Yan, B., Wang, B., et al. (2020). HPV + HNSCC-Derived Exosomal miR-9 Induces Macrophage M1 Polarization and Increases Tumor Radiosensitivity. Cancer Lett. 478, 34–44. doi:10.1016/j.canlet.2020.02.037
van Niel, G., Charrin, S., Simoes, S., Romao, M., Rochin, L., Saftig, P., et al. (2011). The Tetraspanin CD63 Regulates ESCRT-Independent and -Dependent Endosomal Sorting during Melanogenesis. Develop. Cel 21, 708–721. doi:10.1016/j.devcel.2011.08.019
Wan, Z., Gao, X., Dong, Y., Zhao, Y., Chen, X., Yang, G., et al. (2018). Exosome-Mediated Cell-Cell Communication in Tumor Progression. Am. J. Cancer Res. 8, 1661–1673.
Wang, B., Zhang, Y., Ye, M., Wu, J., Ma, L., and Chen, H. (2019a). Cisplatin-Resistant MDA-MB-231 Cell-Derived Exosomes Increase the Resistance of Recipient Cells in an Exosomal miR-423-5p-Dependent Manner. Curr. Drug Metab. 20, 804–814. doi:10.2174/1389200220666190819151946
Wang, D., Zhao, C., Xu, F., Zhang, A., Jin, M., Zhang, K., et al. (2021). Cisplatin-Resistant NSCLC Cells Induced by Hypoxia Transmit Resistance to Sensitive Cells through Exosomal PKM2. Theranostics 11, 2860–2875. doi:10.7150/thno.51797
Wang, J., Guan, X., Zhang, Y., Ge, S., Zhang, L., Li, H., et al. (2018a). Exosomal miR-27a Derived from Gastric Cancer Cells Regulates the Transformation of Fibroblasts into Cancer-Associated Fibroblasts. Cell Physiol. Biochem. 49, 869–883. doi:10.1159/000493218
Wang, M., Qiu, R., Yu, S., Xu, X., Li, G., Gu, R., et al. (2019b). Paclitaxel-Resistant Gastric Cancer MGC-803 Cells Promote Epithelial-to-Mesenchymal Transition and Chemoresistance in Paclitaxel-Sensitive Cells via Exosomal Delivery of miR-155-5p. Int. J. Oncol. 54, 326–338. doi:10.3892/ijo.2018.4601
Wang, T., Gilkes, D. M., Takano, N., Xiang, L., Luo, W., Bishop, C. J., et al. (2014). Hypoxia-Inducible Factors and RAB22A Mediate Formation of Microvesicles that Stimulate Breast Cancer Invasion and Metastasis. Proc. Natl. Acad. Sci. 111, E3234–E3242. doi:10.1073/pnas.1410041111
Wang, X., Luo, G., Zhang, K., Cao, J., Huang, C., Jiang, T., et al. (2018b). Hypoxic Tumor-Derived Exosomal miR-301a Mediates M2 Macrophage Polarization via PTEN/PI3Kγ to Promote Pancreatic Cancer Metastasis. Cancer Res. 78, 4586–4598. doi:10.1158/0008-5472.can-17-3841
Wang, X., Shen, H., Zhangyuan, G., Huang, R., Zhang, W., He, Q., et al. (2018c). 14-3-3ζ Delivered by Hepatocellular Carcinoma-Derived Exosomes Impaired Anti-tumor Function of Tumor-Infiltrating T Lymphocytes. Cel Death Dis 9, 159. doi:10.1038/s41419-017-0180-7
Wang, Z.-F., Liao, F., Wu, H., and Dai, J. (2019c). Glioma Stem Cells-Derived Exosomal miR-26a Promotes Angiogenesis of Microvessel Endothelial Cells in Glioma. J. Exp. Clin. Cancer Res. 38, 201. doi:10.1186/s13046-019-1181-4
Wei, D., Zhan, W., Gao, Y., Huang, L., Gong, R., Wang, W., et al. (2020). RAB31 marks and Controls an ESCRT-Independent Exosome Pathway. Cell Res 31, 157–177. doi:10.1038/s41422-020-00409-1
Whiteside, T. L. (2016). Exosomes and Tumor-Mediated Immune Suppression. J. Clin. Invest. 126, 1216–1223. doi:10.1172/jci81136
Whiteside, T. L., and Jackson, E. K. (2013). Adenosine and prostaglandin e2 production by human inducible regulatory T cells in health and disease. Front. Immunol. 4, 212. doi:10.3389/fimmu.2013.00212
Whiteside, T. L., Schuler, P., and Schilling, B. (2012). Induced and Natural Regulatory T Cells in Human Cancer. Expert Opin. Biol. Ther. 12, 1383–1397. doi:10.1517/14712598.2012.707184
Wolf, P. (1967). The Nature and Significance of Platelet Products in Human Plasma. Br. J. Haematol. 13, 269–288. doi:10.1111/j.1365-2141.1967.tb08741.x
Wu, H., Zeng, C., Ye, Y., Liu, J., Mu, Z., Xie, Y., et al. (2018). Exosomes from Irradiated Nonsmall Cell Lung Cancer Cells Reduced Sensitivity of Recipient Cells to Anaplastic Lymphoma Kinase Inhibitors. Mol. Pharmaceutics 15, 1892–1900. doi:10.1021/acs.molpharmaceut.8b00059
Wu, K., Xing, F., Wu, S.-Y., and Watabe, K. (2017). Extracellular Vesicles as Emerging Targets in Cancer: Recent Development from Bench to Bedside. Biochim. Biophys. Acta (Bba) - Rev. Cancer 1868, 538–563. doi:10.1016/j.bbcan.2017.10.001
Wu, X.-G., Zhou, C.-F., Zhang, Y.-M., Yan, R.-M., Wei, W.-F., Chen, X.-J., et al. (2019). Cancer-Derived Exosomal miR-221-3p Promotes Angiogenesis by Targeting THBS2 in Cervical Squamous Cell Carcinoma. Angiogenesis 22, 397–410. doi:10.1007/s10456-019-09665-1
Xia, X., Wang, S., Ni, B., Xing, S., Cao, H., Zhang, Z., et al. (2020). Hypoxic Gastric Cancer-Derived Exosomes Promote Progression and Metastasis via MiR-301a-3p/PHD3/HIF-1α Positive Feedback Loop. Oncogene 39, 6231–6244. doi:10.1038/s41388-020-01425-6
Xiao, M., Zhang, J., Chen, W., and Chen, W. (2018). M1-Like Tumor-Associated Macrophages Activated by Exosome-Transferred THBS1 Promote Malignant Migration in Oral Squamous Cell Carcinoma. J. Exp. Clin. Cancer Res. 37, 143. doi:10.1186/s13046-018-0815-2
Xu, L., Liao, W.-L., Lu, Q.-J., Zhang, P., Zhu, J., and Jiang, G.-N. (2021). Hypoxic Tumor-Derived Exosomal Circular RNA SETDB1 Promotes Invasive Growth and EMT via the miR-7/Sp1 axis in Lung Adenocarcinoma. Mol. Ther. - Nucleic Acids 23, 1078–1092. doi:10.1016/j.omtn.2021.01.019
Xu, R., Rai, A., Chen, M., Suwakulsiri, W., Greening, D. W., and Simpson, R. J. (2018). Extracellular Vesicles in Cancer - Implications for Future Improvements in Cancer Care. Nat. Rev. Clin. Oncol. 15, 617–638. doi:10.1038/s41571-018-0036-9
Yadid, M., Lind, J. U., Ardoña, H. A. M., Sheehy, S. P., Dickinson, L. E., Eweje, F., et al. (2020). Endothelial Extracellular Vesicles Contain Protective Proteins and rescue Ischemia-Reperfusion Injury in a Human Heart-On-Chip. Sci. Transl. Med. 12, eaax8005. doi:10.1126/scitranslmed.aax8005
Yamada, N. O. (2017). Extracellular Vesicles: Emerging Mediators of Intercellular Communication and Tumor Angiogenesis. Ann. Transl. Med. 5, 59. doi:10.21037/atm.2017.01.14
Yan, W., Wu, X., Zhou, W., Fong, M. Y., Cao, M., Liu, J., et al. (2018). Cancer-Cell-Secreted Exosomal miR-105 Promotes Tumour Growth through the MYC-dependent Metabolic Reprogramming of Stromal Cells. Nat. Cel Biol. 20, 597–609. doi:10.1038/s41556-018-0083-6
Yang, B., Feng, X., Liu, H., Tong, R., Wu, J., Li, C., et al. (2020). High-metastatic Cancer Cells Derived Exosomal miR92a-3p Promotes Epithelial-Mesenchymal Transition and Metastasis of Low-Metastatic Cancer Cells by Regulating PTEN/Akt Pathway in Hepatocellular Carcinoma. Oncogene 39, 6529–6543. doi:10.1038/s41388-020-01450-5
Yang, Z., Jin, P., Xu, S., Zhang, T., Yang, X., Li, X., et al. (2018). Dicer Reprograms Stromal Fibroblasts to a Pro-Inflammatory and Tumor-Promoting Phenotype in Ovarian Cancer. Cancer Lett. 415, 20–29. doi:10.1016/j.canlet.2017.11.026
Yang, Z., Wang, W., Zhao, L., Wang, X., Gimple, R. C., Xu, L., et al. (2021). Plasma Cells Shape the Mesenchymal Identity of Ovarian Cancers through Transfer of Exosome-Derived microRNAs. Sci. Adv. 7, eabb0737. doi:10.1126/sciadv.abb0737
Ye, L., Zhang, Q., Cheng, Y., Chen, X., Wang, G., Shi, M., et al. (2018). Tumor-Derived Exosomal HMGB1 Fosters Hepatocellular Carcinoma Immune Evasion by Promoting TIM-1+ Regulatory B Cell Expansion. J. Immunotherapy Cancer 6, 145. doi:10.1186/s40425-018-0451-6
Ying, X., Wu, Q., Wu, X., Zhu, Q., Wang, X., Jiang, L., et al. (2016). Epithelial Ovarian Cancer-Secreted Exosomal miR-222-3p Induces Polarization of Tumor-Associated Macrophages. Oncotarget 7, 43076–43087. doi:10.18632/oncotarget.9246
Yokoi, A., Yoshioka, Y., Yamamoto, Y., Ishikawa, M., Ikeda, S.-I., Kato, T., et al. (2017). Malignant Extracellular Vesicles Carrying MMP1 mRNA Facilitate Peritoneal Dissemination in Ovarian Cancer. Nat. Commun. 8, 14470. doi:10.1038/ncomms14470
Yu, F., Liang, M., Huang, Y., Wu, W., Zheng, B., and Chen, C. (2021). Hypoxic Tumor-Derived Exosomal miR-31-5p Promotes Lung Adenocarcinoma Metastasis by Negatively Regulating SATB2-Reversed EMT and Activating MEK/ERK Signaling. J. Exp. Clin. Cancer Res. 40, 179. doi:10.1186/s13046-021-01979-7
Yuan, X., Qian, N., Ling, S., Li, Y., Sun, W., Li, J., et al. (2021). Breast Cancer Exosomes Contribute to Pre-Metastatic Niche Formation and Promote Bone Metastasis of Tumor Cells. Theranostics 11, 1429–1445. doi:10.7150/thno.45351
Yue, X., Lan, F., and Xia, T. (2019). Hypoxic Glioma Cell-Secreted Exosomal miR-301a Activates Wnt/β-Catenin Signaling and Promotes Radiation Resistance by Targeting TCEAL7. Mol. Ther. 27, 1939–1949. doi:10.1016/j.ymthe.2019.07.011
Zeng, Y., Yao, X., Liu, X., He, X., Li, L., Liu, X., et al. (2019). Anti-Angiogenesis Triggers Exosomes Release from Endothelial Cells to Promote Tumor Vasculogenesis. J. Extracellular Vesicles 8, 1629865. doi:10.1080/20013078.2019.1629865
Zeng, Z., Li, Y., Pan, Y., Lan, X., Song, F., Sun, J., et al. (2018). Cancer-Derived Exosomal miR-25-3p Promotes Pre-Metastatic Niche Formation by Inducing Vascular Permeability and Angiogenesis. Nat. Commun. 9, 5395. doi:10.1038/s41467-018-07810-w
Zhang, F., Li, R., Yang, Y., Shi, C., Shen, Y., Lu, C., et al. (2019a). Specific Decrease in B-Cell-Derived Extracellular Vesicles Enhances Post-Chemotherapeutic CD8+ T Cell Responses. Immunity 50, 738–750. doi:10.1016/j.immuni.2019.01.010
Zhang, H., Deng, T., Liu, R., Ning, T., Yang, H., Liu, D., et al. (2020). CAF Secreted miR-522 Suppresses Ferroptosis and Promotes Acquired Chemo-Resistance in Gastric Cancer. Mol. Cancer 19, 43. doi:10.1186/s12943-020-01168-8
Zhang, K., Liu, D., Zhao, J., Shi, S., He, X., Da, P., et al. (2021a). Nuclear Exosome HMGB3 Secreted by Nasopharyngeal Carcinoma Cells Promotes Tumour Metastasis by Inducing Angiogenesis. Cel Death Dis 12, 554. doi:10.1038/s41419-021-03845-y
Zhang, P., Zhou, X., and Zeng, Y. (2019b). Multiplexed Immunophenotyping of Circulating Exosomes on Nano-Engineered ExoProfile Chip towards Early Diagnosis of Cancer. Chem. Sci. 10, 5495–5504. doi:10.1039/c9sc00961b
Zhang, Y., Li, Y., Liu, P., Gong, D., Zhou, H., Li, W., et al. (2021b). Phosphatase Shp2 Regulates Biogenesis of Small Extracellular Vesicles by Dephosphorylating Syntenin. J. Extracellular Vesicles 10, e12078. doi:10.1002/jev2.12078
Zhao, J., Schlößer, H. A., Wang, Z., Qin, J., Li, J., Popp, F., et al. (2019). Tumor-Derived Extracellular Vesicles Inhibit Natural Killer Cell Function in Pancreatic Cancer. Cancers 11, 874. doi:10.3390/cancers11060874
Zhao, S., Li, W., Yu, W., Rao, T., Li, H., Ruan, Y., et al. (2021a). Exosomal miR-21 from Tubular Cells Contributes to Renal Fibrosis by Activating Fibroblasts via Targeting PTEN in Obstructed Kidneys. Theranostics 11, 8660–8673. doi:10.7150/thno.62820
Zhao, Y., Zheng, Y., Zhu, Y., Zhang, Y., Zhu, H., and Liu, T. (2021b). M1 Macrophage-Derived Exosomes Loaded with Gemcitabine and Deferasirox against Chemoresistant Pancreatic Cancer. Pharmaceutics 13, 1493. doi:10.3390/pharmaceutics13091493
Zheng, H., Chen, C., Luo, Y., Yu, M., He, W., An, M., et al. (2021). Tumor‐derived Exosomal BCYRN1 Activates WNT5A/VEGF‐C/VEGFR3 Feedforward Loop to Drive Lymphatic Metastasis of Bladder Cancer. Clin. Translational Med. 11, e497. doi:10.1002/ctm2.497
Zheng, P., Luo, Q., Wang, W., Li, J., Wang, T., Wang, P., et al. (2018). Tumor-associated Macrophages-Derived Exosomes Promote the Migration of Gastric Cancer Cells by Transfer of Functional Apolipoprotein E. Cel Death Dis 9, 434. doi:10.1038/s41419-018-0465-5
Zhong, L., Liao, D., Li, J., Liu, W., Wang, J., Zeng, C., et al. (2021). Rab22a-NeoF1 Fusion Protein Promotes Osteosarcoma Lung Metastasis through its Secretion into Exosomes. Sig Transduct Target. Ther. 6, 59. doi:10.1038/s41392-020-00414-1
Zhou, X., Yan, T., Huang, C., Xu, Z., Wang, L., Jiang, E., et al. (2018a). Melanoma Cell-Secreted Exosomal miR-155-5p Induce Proangiogenic Switch of Cancer-Associated Fibroblasts via SOCS1/JAK2/STAT3 Signaling Pathway. J. Exp. Clin. Cancer Res. 37, 242. doi:10.1186/s13046-018-0911-3
Zhou, Y., Ren, H., Dai, B., Li, J., Shang, L., Huang, J., et al. (2018b). Hepatocellular Carcinoma-Derived Exosomal miRNA-21 Contributes to Tumor Progression by Converting Hepatocyte Stellate Cells to Cancer-Associated Fibroblasts. J. Exp. Clin. Cancer Res. 37, 324. doi:10.1186/s13046-018-0965-2
Zhu, L., Kalimuthu, S., Gangadaran, P., Oh, J. M., Lee, H. W., Baek, S. H., et al. (2017). Exosomes Derived from Natural Killer Cells Exert Therapeutic Effect in Melanoma. Theranostics 7, 2732–2745. doi:10.7150/thno.18752
Zhu, X., Shen, H., Yin, X., Yang, M., Wei, H., Chen, Q., et al. (2019). Macrophages Derived Exosomes Deliver miR-223 to Epithelial Ovarian Cancer Cells to Elicit a Chemoresistant Phenotype. J. Exp. Clin. Cancer Res. 38, 81. doi:10.1186/s13046-019-1095-1
Zhuang, G., Wu, X., Jiang, Z., Kasman, I., Yao, J., Guan, Y., et al. (2012). Tumour-secreted miR-9 Promotes Endothelial Cell Migration and Angiogenesis by Activating the JAK-STAT Pathway. EMBO J. 31, 3513–3523. doi:10.1038/emboj.2012.183
Keywords: extracellular vesicles, tumor microenvironment, angiogenesis, resistance, immune cells, biomarkers
Citation: Bao Q, Huang Q, Chen Y, Wang Q, Sang R, Wang L, Xie Y and Chen W (2022) Tumor-Derived Extracellular Vesicles Regulate Cancer Progression in the Tumor Microenvironment. Front. Mol. Biosci. 8:796385. doi: 10.3389/fmolb.2021.796385
Received: 16 October 2021; Accepted: 08 December 2021;
Published: 04 January 2022.
Edited by:
Jian-ye Zhang, Guangzhou Medical University, ChinaReviewed by:
Daniele Vergara, University of Salento, ItalyDwijendra K. Gupta, Jai Prakash Vishwavidyalaya, India
Manuel Varas-Godoy, San Sebastián University, Chile
Copyright © 2022 Bao, Huang, Chen, Wang, Sang, Wang, Xie and Chen. This is an open-access article distributed under the terms of the Creative Commons Attribution License (CC BY). The use, distribution or reproduction in other forums is permitted, provided the original author(s) and the copyright owner(s) are credited and that the original publication in this journal is cited, in accordance with accepted academic practice. No use, distribution or reproduction is permitted which does not comply with these terms.
*Correspondence: Lei Wang, wanglei@ahtcm.edu.cn; Ying Xie, yxie@must.edu.mo; Weidong Chen, wdchen@ahtcm.edu.cn