- 1Biomedical Research Center, Qatar University, Doha, Qatar
- 2Department of Drug Design, University of Groningen, Groningen, Netherlands
- 3Qatar Metabolic Institute, Hamad Medical Corporation, Doha, Qatar
- 4Translational Research Institute, Hamad Medical Corporation, Doha, Qatar
- 5Laboratorio Antidoping, Federazione Medico Sportiva Italiana, Rome, Italy
- 6Physical Education Department, College of Education, Qatar University, Doha, Qatar
Introduction: Aerobic exercise activates the complement system in the peripheral blood. However, the effect of age and high intensity endurance training on the levels of circulating complements and sassociated inflammatory cytokines, oxidative stress markers and cellular aging remains unknown.
Methods: In this study, serum samples from 79 elite athletes who belong to high (n = 48) and low/moderate (n = 31) endurance sports and two age groups (below 30 years old, n = 53, and above 30 years old, n = 26) were profiled for 14 complements. Linear models were used to assess differences in complements levels between sport and age groups. Spearmann’s correlation was used to assess the relationship among detected complements and proinflammatory cytokines, oxidative stress markers and telomere lengths.
Results: High endurance elite athletes exhibited significantly lower levels of circulating C2, C3b/iC3b and adipsin complements than their age-matched low/moderate endurance counterparts. Levels of C2, adipsin and C3b/iC3b were positively correlated with most detected complements, the pro-inflammatory cytokines TNF-alpha and IL-22 and the anti-oxidant enzyme catalase. However, they were negatively correlated with telomere length only in younger elite athletes regardless of their sport groups. Furthermore, high endurance elite athletes showed significantly lower concentrations of C3b/iC3b, C4b, C5, C5a, C1q, C3, C4, factor H and properdin in younger athletes compared to their older counterparts.
Conclusion: Our novel data suggest that high endurance elite athletes exhibit age-independent lower levels of circulating C2, C3b/iC3b and adipsin, associated with lower inflammatory, oxidative stress and cellular aging, as well as lower levels of 10 other complements in younger athletes compared to older counterparts. Assessing the effect of various levels of endurance sports on complements-based immune response provides a better understanding of exercise physiology and pathophysiology of elite athletes.
Introduction
As part of the innate immune response, the complement system protects against pathogens in the absence of the adaptive immunity (Medzhitov and Janeway, 1998) and links the innate and acquired immune responses (Carroll, 2000). It offers systemic surveillance and protection by chemotaxis-mediated phagocytosis, scavenging pathogens as well as necrotic and apoptotic debris. Complements can also mediate cell activation, regenerative processes, and humoral and cell-mediated immune responses (Schifferli et al., 1986; Ricklin et al., 2010). The complement system includes 50 proteins that constitute around 15% of the globulin fraction (Carroll, 2000; Walport, 2001). Three main pathways can activate the complement system: classical, lectin, and alternative. The classical pathway is triggered by antigen–antibody immune complexes, apoptotic and necrotic cells and by acute phase proteins such as C-reactive protein. The lectin pathway uses mannose-binding lectins and ficolins to identify patterns of carbohydrate ligands found on the surface of microorganisms. The alternative pathway is constitutively active at low levels in the normal host in preparation for rapid and robust activation upon stimulation (Noris and Remuzzi, 2013).
Each component of the complement system offers a unique function in response to different stimuli. In the classical pathway, C2 is cleaved into C2a and C2b, followed by the fusion between C2a and C4b components, forming the classical-C3 convertase with proteolytic activity (Sarma and Ward, 2011; Prohászka et al., 2018). C3 complement participates in both classic and alternative pathways and its deficiency can cause impairment of the immune response and enhanced risk of infection (Matsuyama et al., 2001). C3 protein cleavage into C3b component is mediated by C3 convertase, which is also shared by the lectin activation pathway. The overstimulation of the activity of the complement system could harm the body, causing serious diseases such as organ rejection, asthma, multiple sclerosis, sepsis, or Alzheimer’s disease (Sarma and Ward, 2011). Previous studies have shown that complement C3 is involved in the aging process (Wu et al., 2020) and that secretion of complement C1q is increased with aging in association with elevated vascular smooth muscle cells proliferation (Hasegawa et al., 2019). The alternative pathway is more dominant compared to the classical one (Sarma and Ward, 2011). Adipsin (complement factor D) is secreted by macrophages, monocytes and adipocytes and controls the alternative complement pathway and the generation of C3a (Gómez-Banoy et al., 2019; Ohtsuki et al., 2019). In obesity, adipsin levels correlate with metabolic disease (Wang et al., 2019) and constitutes a good predictor of death in patients with coronary artery disease (Ohtsuki et al., 2019; Liu et al., 2021) and risk of nonalcoholic fatty liver (Qiu et al., 2019).
The role of the complement system in modulating disease progression in systemic autoimmunity and primary immune-deficiencies is well established (Ricklin et al., 2010; Skattum et al., 2011; De Cordoba et al., 2012; Holers, 2014). Its involvement in post-effort immunity is also documented (Mashiko et al., 2004; Karacabey et al., 2005). However, its role in the chronic exercise immune response is yet to be determined. A recent study has suggested that aerobic exercise can trigger various immune responses in active young men via activating the alternative pathway (Kostrzewa-Nowak et al., 2020). Whereas moderate intensity regular exercise stimulates the immune system, high intensity exercise without sufficient recovery could reduce the immune response and enhance risk of infections (Calabrese and Nieman, 1996; Nieman, 1997; Pedersen and Hoffman-Goetz, 2000; Gleeson et al., 2012; Peake et al., 2017a; Peake et al., 2017b). Hence, intensive physical activity can stimulate or suppress the immune response based on many factors such as age and fitness level, triggering oxidative stress and release of cortisol, catecholamines, insulin like growth factor and heat shock proteins (Nieman, 1997; Liu et al., 2018). The main aim of this study was to examine the impact of two types of endurance sports (low/moderate vs high) in elite athletes on the complement system and post-exercise immune response in younger (less than 30 years old) and older (more than 30 years old) healthy athletes. Profiling complements in elite athletes who belong to different exercise intensity and age groups could help in the understanding the impact of chronic exercise and aging on the immune response.
Methods
Cohort
Blood samples from 79 consented elite athletes (68 males and 11 females), defined as those participating in national or international sports events and tested for doping abuse by accredited anti-doping laboratories, who belong to different sport disciplines (Table 1) were previously collected for doping analysis of growth factors at anti-doping laboratory in Italy (FMSI). Spare sera collected for anti-doping human growth hormone testing were utilized for complements profiling. Briefly, samples were collected by doping officers in serum separator tubes, then delivered to the anti-doping laboratory within 36 h under cooling conditions. Once received, samples were immediately centrifuged to separate the serum and then stored at −20°C until analysis. Following published criteria (Mitchell et al., 2005; Al-Khelaifi et al., 2018a; Al-Khelaifi et al., 2018b; Al-Khelaifi et al., 2019), participants were divided into two intensity groups based on their sports type: low/moderate endurance sports (n = 48, 43 males and five females) and high endurance sports (n = 31, 25 males and six females). Participants were also divided into two age groups: less than 30 years old (n = 53) and above 30 years old (n = 26). This study is performed in line with the World Medical Association Declaration of Helsinki–Ethical Principles for Medical Research Involving Human Subjects. All protocols were approved by the Institutional Research Board of Qatar University (QU-IRB 1277-E/20). All participants consented for the use of their samples for research.

TABLE 1. Classification of participants (males: M and females: F) according to the endurance intensity of their respective sports.
Human Complement Related Protein Measurements
MILLIPLEX MAP Kit Human Complement Magnetic Bead Panels one and 2 (HCMP1MAG-19K and HCMP2MAG-19K) were used to measure levels of complements in the sera of elite athletes according to manufacturer’s instructions (Merck Millipore, United States). Sensitivity and accuracy levels can be found under the physicochemical information in the manufacturer’s website. Serum samples were diluted 200 times for complement panel one containing C2, C4b, C5, C5a, C9, Factor D, and Mannose-Binding Lectin and 40,000 times for complement panel two containing C1q, C3, C3b/iC3b, C4, Factor B, Factor H and Properdin. Five parameters logistic regression algorithms built into the Bioplex manager six software were used to assess complement levels in reference to standards. Analysis was conducted using a Bioplex-200 instrument according to manufacturer’s instructions (BIO-RAD, Hertfordshire, United Kingdom).
Cytokine profiling
Human CorPlex Cytokine one Array 10-Plex (116-7BF-1-AB) was used to simultaneously profile 10 cytokines, including IL-12p70, IL-1β, IL-4, IL-5, IFN-γ, IL-6, IL-8, IL-22, TNF-α, IL-10, according to manufacturer’s instructions (Quanterix, United States) and recently described (Sellami et al., 2021a).
Measurement of anti-oxidative stress enzymatic activities
Colorimetric activity assays (EIACATC and EIASODC) were used to measure the activities of superoxide dismutase and catalase according to manufacturer’s instructions (ThermoFisher Scientific, United States) and recently described (Sellami et al., 2021a).
Measurement of telomere length
DNA was extracted from whole blood using DNeasy Blood and Tissue kit according to manufacturer’s instructions (Qiagen, Germany). Nanodrop was used to assess the concentration/quality of DNA. The average telomere lengths in extracted DNA samples were assessed using Absolute Human Telomere Length Quantification qPCR Assay Kit according to manufacturer’s instructions (ScienCell, United States). The kit includes telomere primer set that amplifies telomere sequences, a single copy reference region for data normalization and a reference genomic DNA sample with known telomere length as a reference for calculating the telomere length of target samples.
Statistical analysis
Comparisons were performed using t-test, Wilcoxon–Mann–Whitney, 1-way ANOVA, or linear models as appropriate using IBM SPSS statistics 21. Linear regression models were used when analyzing differences in complements levels among different age and intensity groups by considering gender as a potential confounder as females were over represented in the high endurance group. Data were presented as mean ± standard deviation (SD) for parametric data, median (interquartile range, IQR) for non-parametric data verified by skewness and kurtosis test.
Results
Differences in the levels of complements between athletes who belong to low/moderate versus high endurance sports
Among the detected complements, C2, adipsin and C3b/iC3b showed significantly lower levels in athletes who belong to high endurance sports compared to age-matched athletes who belong to low/moderate endurance sports (Table 2; Figure 1). Similarly, there were lower levels of factor B, factor H and properdin in high endurance athletes compared to low/moderate endurance athletes, but differences did not reach statistical significance (p = 0.1).
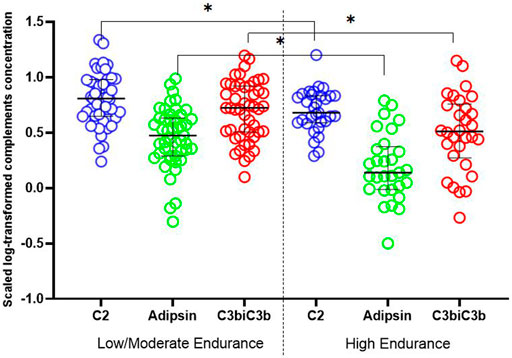
FIGURE 1. Dot plot exhibiting differences in complements levels between high and mild/moderate endurance sports. Independent sample t-test was used to compare scaled log-transformed cytokine levels (y axis) in different sport intensity groups. Data are presented as median and IQR. * <0.05.
Correlations between C2, adipsin and C3b/iC3b complements and other complements, inflammatory cytokines and oxidative stress markers
Levels of inflammatory cytokines and oxidative stress markers were recently published (Sellami et al., 2021b). There were significant positive correlations between C3, adipsin and C3b/iC3b and other measured complements in all participants (n = 79) regardless of sport intensity or age groups (Figure 2A). There were also positive correlations between C2, adipsin and C3b/iC3b complements and the pro-inflammatory cytokines TNF-α and IL-22 and the anti-oxidant enzyme catalase (Figures 2B–D).
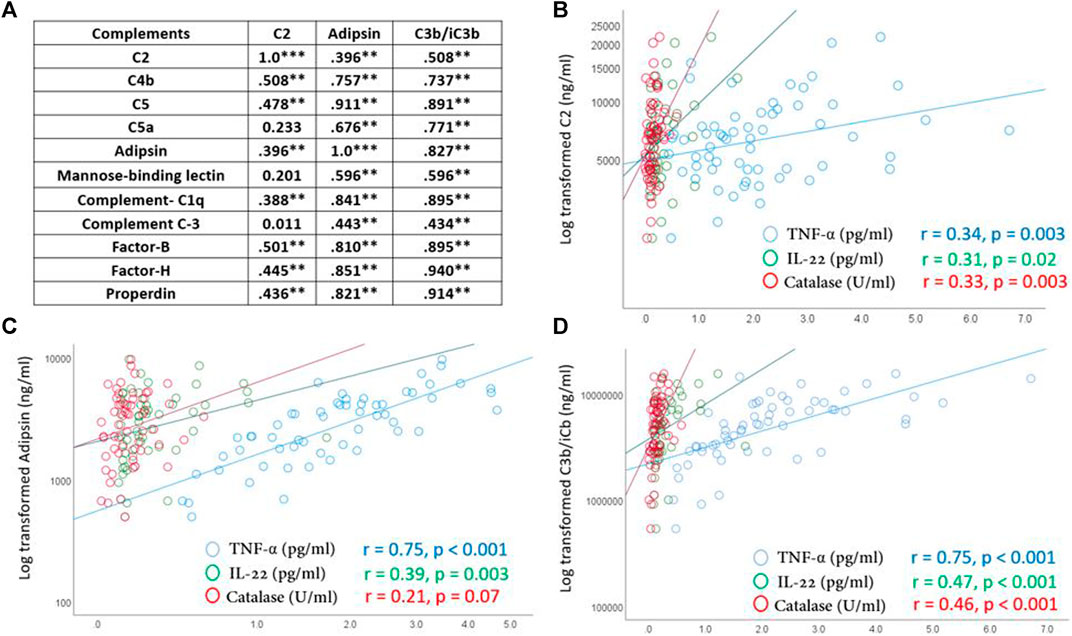
FIGURE 2. Correlations between C2, adipsin and C3b/iC3b complements and other detected complements (A) and inflammatory cytokines and antioxidant enzyme catalase (B–D). Correlations were made using spearman’s correlation analysis. Correlation coefficient (r) and significance (*p ≥ 0.05, **p ≥ 0.01, ***p ≥ 0.001) are indicated.
Differences in complement levels among athletes who belong to different age groups in low/moderate and high endurance elite athletes
Linear models revealed that C3b/iC3b, C4b, C5, C5a, C1q, C3, C4, factor H and properdin were significantly lower in younger high endurance athletes compared to their older counterparts (Table 3; Figure 3). There were no significant differences in the levels of complements in low/moderate elite endurance athletes between the two age groups (Table 3).
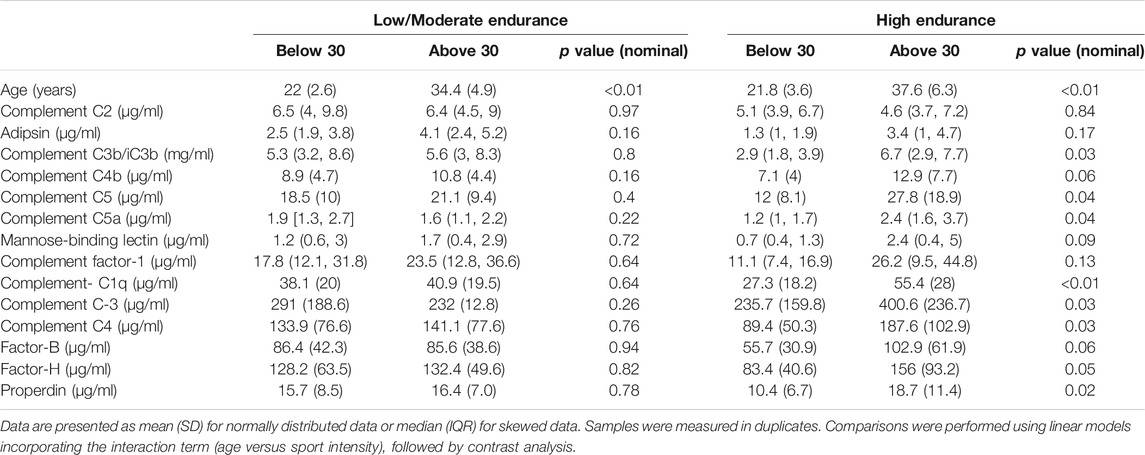
TABLE 3. Comparing complements between different age groups in low/moderate and high endurance elite athletes.
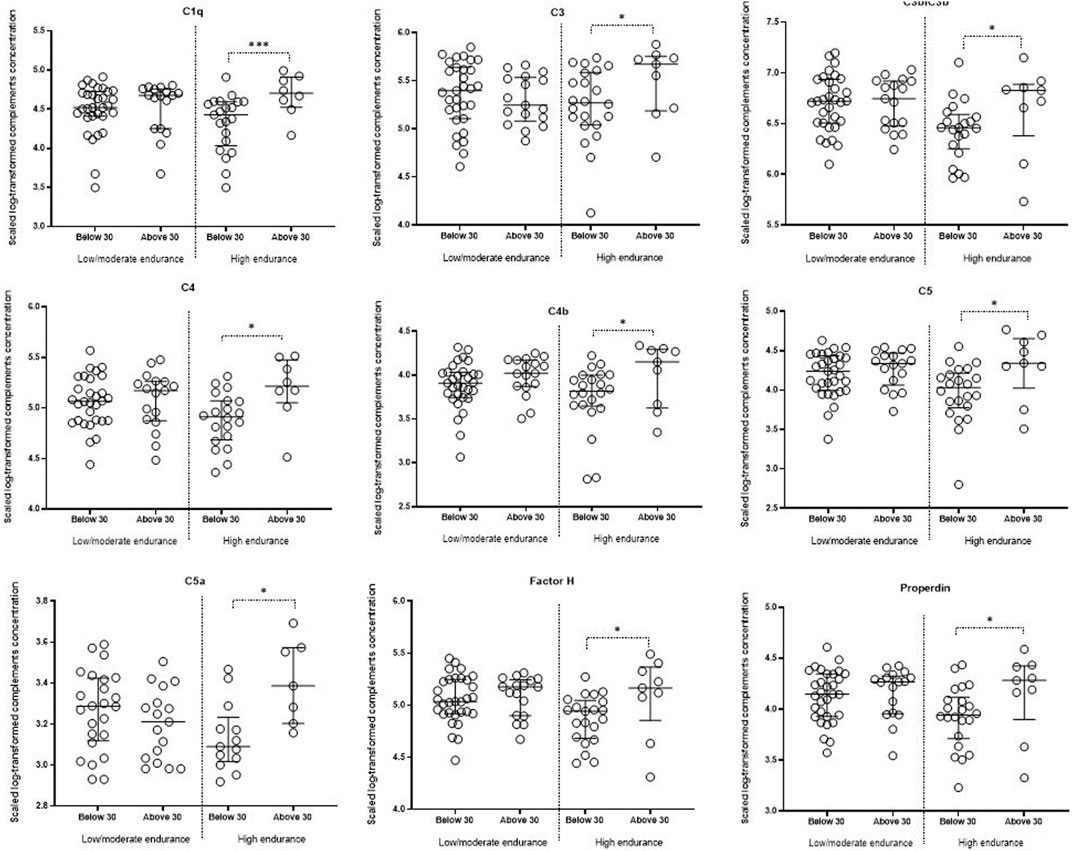
FIGURE 3. Comparing complements between different age groups in low/moderate and high endurance elite athletes. Linear models incorporating the interaction term (age versus sport intensity), followed by contrast analysis, were used to compare scaled log-transformed cytokine levels (y axes) in different sport intensity groups. Data are presented as median and IQR. * <0.05.
Correlations between complements and telomere length in two age groups
There were significant negative correlations between telomere lengths and C2 (R = −0.27, p = 0.05) and C3b/iC3b (R = −0.34, p = 0.02) only in younger athletes (Figure 4), suggesting less cellular aging of blood cells in younger, but not older, high endurance elite athletes.
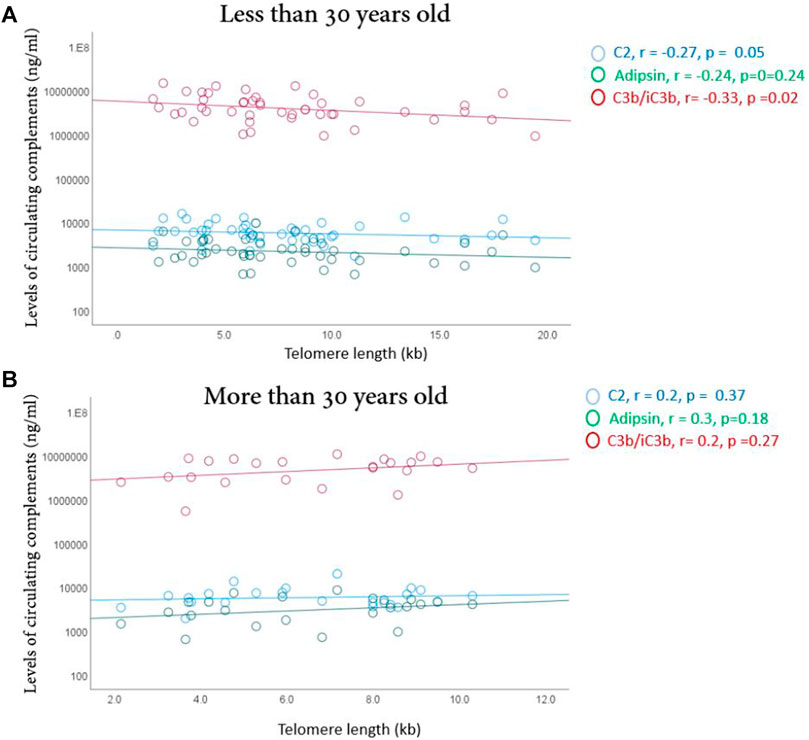
FIGURE 4. Correlation between telomere length and the complements (C2, adipsin and C3b/iC3B) in younger (A) and older (B) elite athletes. Correlations were made using spearman’s correlation analysis. Correlation coefficient (r) and significance are indicated.
Discussion
The effect of physical activity on the immune system varies according to the type, duration and intensity of the exercise (Nieman and Wentz, 2019). These effects are mediated by complex mechanisms that include hormonal, metabolic and psycho-neural changes (Silverman and Deuster, 2014). Short-term low/moderate physical activity with intensity <60% VO2max can modulate the immune response and enhance athlete immunity (Cannon, 1993; Sohail et al., 2020). Conversely, high endurance exercise with intensity greater than >70% VO2max could contribute to lowering the athletes’ immunity (Cannon, 1993; Mackinnon, 1994). As part of the immune response, acute physical activity can also activate the alternative complement pathway in an intensity and duration-dependent manners (Kostrzewa-Nowak et al., 2020), however the effect of long-term exercise, such as the one practiced by elite athletes on circulating complement levels remains to be investigated. In this study, circulating levels of 14 complements were profiled in the serum samples of 79 elite athletes who belong to high and low/moderate endurance sports and two age groups (below and above 30 years old). This age threshold was previously described as a discontinuation stage entailing elite athletes' transition out of competitive sports (Wylleman and Reints, 2010). Our emerging novel data suggest that high endurance elite athletes exhibit age-dependent and independent lower levels of specific complements associated with lower inflammatory, oxidative stress and cellular aging markers.
Low levels of circulating C2, C3b/iC3b and adipsin complements in high endurance athletes
The main finding of this study was that athletes who belong to high or low/moderate endurance sports exhibit a differential profile of complement system. Athletes who belong to high endurance sports showed reduced activation of C2, C3b/iC3b and adipsin compared to the age-matched low/moderate endurance athletes. The complement C2 is a serine protease that provides catalytic activity to the C3 convertase of classical pathway of complements (Figure 5). The lower secretion of C2 in high endurance falls in line with the previously reported lower levels in long distance running athletes compared to volleyball players who belong to moderate endurance sports (Saygin et al., 2006). The complement C3 as well as the breakdown products C3b and iC3b, play a central role in the activation of the complement system, which is required for both classical and alternative complement activation pathways (Figure 5) (Noris and Remuzzi, 2013). Previous studies have demonstrated that acute endurance effort (20 m shuttle run test) triggers a decrease in the post-test C3, but increase in iC3b and C2 in 15–17 year old active males. On the other hand, repeated speed ability test caused a decrease in the post-test C2 for younger participants only, but an increase in the recovery C3a levels (Kostrzewa-Nowak et al., 2020). Differences in C3 responses to endurance between reported data and our study may reflect the difference in the duration (acute or chronic) or intensity (recreational or professional) of exercise between participants of the two studies. However, both data suggest that exercise can indeed trigger alterations in C2, C3b and iC3b levels in an intensity-dependent manner. The impact of lower levels of these complements on the immune response, general health and performance of elite high endurance athletes remains to be determined.
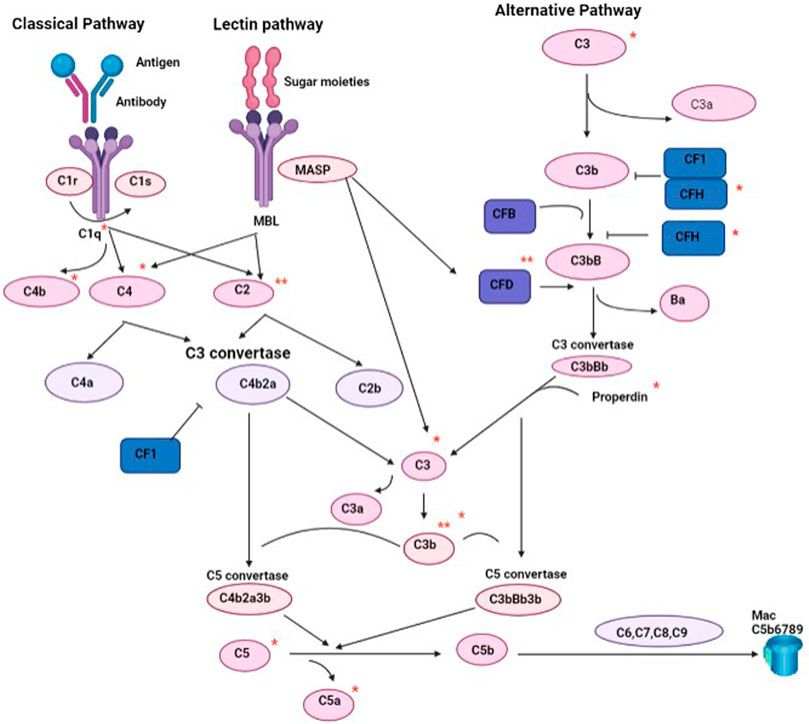
FIGURE 5. A schematic representation of complement pathways highlighting complements that were reduced in high endurance athletes (**) and those reduced in younger high endurance athletes (*). CF (complement factor). CFD (adipsin).
Our data also indicated lower adipsin levels in high endurance athletes. Adipsin, also known as complement factor D, is one of the major proteins secreted by adipocytes for maintaining adipose tissue homeostasis and increasing insulin secretion in response to glucose (Tafere et al., 2020). It also plays a critical role in mediating the rate-limiting step of the alternative pathway of complement activation through the formation of the C5-C9 membrane attack complex and the generation of a number of signaling molecules including the anaphylatoxins C3a and C5a (Figure 5) (Xu et al., 2001; Ricklin et al., 2010). It has been implicated in increased risk of models of ischemia reperfusion (Stahl et al., 2003), and sepsis (Dahlke et al., 2011). Lower plasma adipsin concentrations are described in animals and patients with type 2 diabetes mellitus, since its presence is important for improving hyperglycemia by preserving β-cell survival (Tafere et al., 2020). Therefore, the implication of lower levels of adipsin in high endurance elite athletes could indicate higher risk of metabolic disease as previously suggested (Al-Khelaifi et al., 2019), although further studies are required to investigate the functional relevance of lower adipsin.
Positive correlations between C2, adipsin, C3b/iC3b and other complements, TNF-α, IL-22 and catalase
Significant positive correlations were identified between levels of the three complements that were reduced in high endurance athletes (C2, adipsin and C3b/iC3b) and other detected complements of the classical, lectin, and alternative pathways. These correlations are expected since they could simply reflect the identical nature of these complements caused by their duplicated genes that have diverged in sequence (Janeway et al., 2001). Furthermore, positive correlations were also seen between C2/adipsin/C3b/iC3b and the pro-inflammatory cytokines TNF-α and IL-22. Previous reports have indicated that T helper cell subset 22 (Th22) is characterized by a combinatorial secretion of IL-22 and TNF-α, and that IL-22 increases the TNF-α-dependent induction and secretion of several immune-modulatory molecules including complements (Eyerich et al., 2011). Such synergistic action could explain the positive correlation between these cytokines and C2, adipsin, C3b/iC3b. The production of catalase is increased at higher levels of exercise in order to compensate for the insufficient clearance of hydrogen peroxide by glutathione peroxidase (Finsterer, 2012). The positive correlation between catalase levels and complements could reflect association between inflammation and oxidative stress (Chen et al., 2012; Sellami et al., 2021b); however, the lower levels in younger high endurance athletes may reflect a protective mechanism against oxidative stress with high endurance exercise in younger, but not older, elite athletes.
C2 and C3b/iC3b were negatively correlated with telomere length in younger elite athletes
Telomeres are repetitive nucleotide sequences located at the ends of chromosomes, which prevent loss of genetic information during replication. A shorter telomere length is a strong indicator of cellular ageing and potentially health decline (Shammas, 2011). Studies have shown that that young elite athletes have longer telomeres than their inactive peers (Tafere et al., 2020). Moderate chronic exercise was previously shown to be associated with longer telomeres and better health status and lifespan (Saygin et al., 2006; Wylleman and Reints, 2010). Endurance, but not resistance, training was also suggested to acutely increase telomerase activity and telomere length (Tafere et al., 2020), perhaps due to an acquiring an adaptive mechanism contributing to maintenance of telomeres (Saygin et al., 2006). Moderate exercise was too shown to be associated with changes in lengths of telomeres due to lowering of oxidative stress and inflammation (Sellami et al., 2021b). Furthermore, literature has suggested a strong correlation between VO2max and telomere length (Chen et al., 2012). In order to investigate whether younger elite endurance athletes have less cellular aging compared to their older counterparts, telomere lengths were determined in circulating blood cells. Our data have shown that lower levels of C2 and C3b/iC3b were associated with longer telomeres in younger elite athletes, which could reflect lower chronic inflammation and oxidative stress and elevated telomerase activity in younger elite athletes compared to older counterparts (Samjoo et al., 2013).
Lower C3b/iC3b, C4b, C5, C5a, C1q, C3, C4, factor H and properdin in younger high endurance athletes
Lower levels of complements that belong to classical, alternative and lectin pathways were detected in younger high endurance elite athletes (Figure 5). This may suggest that lower inflammatory and oxidative stress status is associated with endurance training only in young athletes compared to their older counterparts or age-matched peers who belong to lower intensity sports (Samjoo et al., 2013). Our data agree with previous studies suggesting a significant decrease in C3 and C4 proteins in response to physical training in both athletes and non-athletes (Karacabey et al., 2005; Saygin et al., 2006). Indeed, aerobic exercise on a treadmill for 30 min was found to trigger a significant decrease in C3 and C4 proteins (Karacabey et al., 2005) and a similar post-game observation was reported in rugby players (Mashiko et al., 2004). Conversely, other studies have shown that short-term aerobic exercise activated C3 and C4 complement components and subsequently C3a and C4a (Smith et al., 1990). Furthermore, a post-effort increase in C3 and C4 complement components was observed in Marathon runners and their sedentary counterparts performing graded exercise on a mechanical treadmill (Nieman et al., 1989; Mochizuki et al., 1999). A similar trend was also observed in the case of C4 in older participants performing aerobic exercise (Kostrzewa-Nowak et al., 2020). Taken both opposing views into account, it may be concluded that endurance effort exerts alterations in the complement system that may depend on the intensity, duration and type of physical training.
Previous studies have also shown different effect of endurance effort on complement levels in athletes who belong to different age groups. For example, a decrease in the post-test C2 was only seen in younger participants (Kostrzewa-Nowak et al., 2020). It remains to be investigated whether age-related dysregulation of mitochondrial biogenesis, dynamic and autophagy could explain the compromised role of exercise on lowering complements levels in older athletes (Joseph et al., 2016; Sellami et al., 2021b). Comparing exercise-triggered mitochondrial alterations in relation to complement secretion in younger versus older athletes could be instrumental to understand the molecular pathways underlying age-dependent changes in the levels of circulating complements in elite endurance athletes.
Study limitations
The relatively small number of participants and the diversity of their sport groups are main limitations of this study. The overrepresentation of females in the high endurance group represents another limitation of the study, although gender was corrected for in statistical analyses. Additionally, variation in timing of sample collection, transportation and storage could have also affected the results (Shivshankar et al., 2020), although standard operating procedures and shortest duration of sample delivery (within hours of collection) are usually implemented. Furthermore, the limited available information of athletes’ anthropometric, medical history, smoking habits, physiological and nutritional data during sampling as well as the resting time since their last exercise has hindered attempts to consider other important potential confounders such as body mass index and diet in our analysis. However, since samples were selected for doping tests, it suggests that the included participants were active elite athletes, hence unlikely suffering from any inflammatory or metabolic diseases, nor likely to be smokers or under chronic medication. Although various studies have investigated the effect of acute exercise on complement levels and despite all these limitations, our novel data has revealed significant and pronounced alterations in complements in the studied endurance and age groups. However, replication of data in other cohorts with controlled experimental design is warranted.
Conclusion
Understanding the complement system in response to long-term low/moderate and high endurance exercise could provide insight into the mechanisms of exercise immunology and potentially grants a stronger molecular basis for the prevention of exercise-associated injuries and illnesses. The level of complements reported in our study suggest that young high endurance elite athletes exhibit lower levels of circulating complements, associated with lower inflammatory, oxidative stress and cellular aging. Future studies are warranted to investigate the health profile, performance and susceptibility to injuries in this group of elite athletes compared to their older peers or those who belong to lower intensity sports.
Data Availability Statement
The original contributions presented in the study are included in the article/Supplementary Material, further inquiries can be directed to the corresponding author.
Ethics Statement
The studies involving human participants were reviewed and approved by Institutional Research Board of Qatar University (QU-IRB 1277-E/20). The patients/participants provided their written informed consent to participate in this study.
Author Contributions
SM carried out the experimental part and wrote the paper. MR, AD, IB, AA amd MS helped with data analysis and reviewing the paper. FD and FB were involved in study design, sample collection and data analysis. ME was lead principle investigator, designed the experiments, supervised progress, analyzed data and reviewed and approved the final version of the article. ME is responsible for the integrity of the work as a whole. All authors have read and approved the manuscript.
Funding
This research was sponsored by QNRF, Grant no. UREP 26-043-3-018 (MAE, MS)
Conflict of Interest
The authors declare that the research was conducted in the absence of any commercial or financial relationships that could be construed as a potential conflict of interest.
Publisher’s Note
All claims expressed in this article are solely those of the authors and do not necessarily represent those of their affiliated organizations, or those of the publisher, the editors and the reviewers. Any product that may be evaluated in this article, or claim that may be made by its manufacturer, is not guaranteed or endorsed by the publisher.
Acknowledgments
We thank Qatar National Research Fund (QNRF; Grant Nos. UREP 26-043-3-018) for funding this project. The statements made herein are solely the responsibility of the authors.
References
Al-Khelaifi, F., Donati, F., Botrè, F., Latiff, A., Abraham, D., Hingorani, A., et al. (2019). Metabolic Profiling of Elite Athletes with Different Cardiovascular Demand. Scand. J. Med. Sci. Sports 29, 933–943. doi:10.1111/sms.13425
Al-Khelaifi, F., Diboun, I., Donati, F., Botrè, F., Alsayrafi, M., Georgakopoulos, C., et al. (2018). A Pilot Study Comparing the Metabolic Profiles of Elite-Level Athletes from Different Sporting Disciplines. Sports Med. - Open 4, 2. doi:10.1186/s40798-017-0114-z
Al-Khelaifi, F., Diboun, I., Donati, F., Botrè, F., Alsayrafi, M., Georgakopoulos, C., et al. (2018). Metabolomics Profiling of Xenobiotics in Elite Athletes: Relevance to Supplement Consumption. J. Int. Soc. Sports Nutr. 15, 48. doi:10.1186/s12970-018-0254-7
Calabrese, L. H., and Nieman, D. C. (1996). Exercise, Immunity, and Infection. J. Am. Osteopath Assoc. 96, 166. doi:10.7556/jaoa.1996.96.3.166
Cannon, J. G. (1993). Exercise and Resistance to Infection. J. Appl. Physiol. (1985) 74, 973–981. doi:10.1152/jappl.1993.74.3.973
Carroll, M. C. (2000). A Protective Role for Innate Immunity in Autoimmune Disease. Clin. Immunol. 95, S30–S38. doi:10.1006/clim.1999.4813
Chen, S.-J., Yen, C.-H., Huang, Y.-C., Lee, B.-J., Hsia, S., and Lin, P.-T. (2012). Relationships between Inflammation, Adiponectin, and Oxidative Stress in Metabolic Syndrome. PLoS One 7, e45693. doi:10.1371/journal.pone.0045693
Dahlke, K., Wrann, C. D., Sommerfeld, O., Sossdorf, M., Recknagel, P., Sachse, S., et al. (2011). Distinct Different Contributions of the Alternative and Classical Complement Activation Pathway for the Innate Host Response during Sepsis. J.I. 186, 3066–3075. doi:10.4049/jimmunol.1002741
De Cordoba, S. R., Tortajada, A., Harris, C. L., and Morgan, B. P. (2012). Complement Dysregulation and Disease: from Genes and Proteins to Diagnostics and Drugs. Immunobiology 217, 1034–1046. doi:10.1016/j.imbio.2012.07.021
Eyerich, S., Wagener, J., Wenzel, V., Scarponi, C., Pennino, D., Albanesi, C., et al. (2011). IL-22 and TNF-α Represent a Key Cytokine Combination for Epidermal Integrity during Infection with Candida Albicans. Eur. J. Immunol. 41, 1894–1901. doi:10.1002/eji.201041197
Finsterer, J. (2012). Biomarkers of Peripheral Muscle Fatigue during Exercise. BMC Musculoskelet. Disord. 13, 218. doi:10.1186/1471-2474-13-218
Gleeson, M., Walsh, N. P., British Association of, S., and Exercise, S. (2012). The BASES Expert Statement on Exercise, Immunity, and Infection. J. Sports Sci. 30, 321–324. doi:10.1080/02640414.2011.627371
Gómez-Banoy, N., Guseh, J. S., Li, G., Rubio-Navarro, A., Chen, T., Poirier, B., et al. (2019). Adipsin Preserves Beta Cells in Diabetic Mice and Associates with protection from Type 2 Diabetes in Humans. Nat. Med. 25, 1739–1747. doi:10.1038/s41591-019-0610-4
Hasegawa, N., Fujie, S., Horii, N., Uchida, M., Toyama, Y., Inoue, K., et al. (2019). Aging-induced Elevation in Circulating Complement C1q Level Is Associated with Arterial Stiffness. Exp. Gerontol. 124, 110650. doi:10.1016/j.exger.2019.110650
Holers, V. M. (2014). Complement and its Receptors: New Insights into Human Disease. Annu. Rev. Immunol. 32, 433–459. doi:10.1146/annurev-immunol-032713-120154
Janeway, C. A., Travers, P., Walport, M., et al. (2001). Immunobiology: The Immune System in Health and Disease. 5th edition. New York: Garland Science.
Joseph, A.-M., Adhihetty, P. J., and Leeuwenburgh, C. (2016). Beneficial Effects of Exercise on Age-Related Mitochondrial Dysfunction and Oxidative Stress in Skeletal Muscle. J. Physiol. 594, 5105–5123. doi:10.1113/jp270659
Karacabey, K., Saygin, O., Ozmerdivenli, R., Zorba, E., Godekmerdan, A., and Bulut, V. (2005). The Effects of Exercise on the Immune System and Stress Hormones in Sportswomen. Neuro Endocrinol. Lett. 26, 361–366. doi:10.1080/13102818.2004.10817142
Kostrzewa-Nowak, D., Kubaszewska, J., Nowakowska, A., and Nowak, R. (2020). Effect of Aerobic and Anaerobic Exercise on the Complement System of Proteins in Healthy Young Males. J. Clin. Med. 9, 2357. doi:10.3390/jcm9082357
Liu, L., Chan, M., Yu, L., Wang, W., and Qiang, L. (2021). Adipsin Deficiency Does Not Impact Atherosclerosis Development in Ldlr−/− Mice. Am. J. Physiology-Endocrinology Metab. 320, E87–E92. doi:10.1152/ajpendo.00440.2020
Liu, X., Zeng, Z., Zhao, L., Xiao, W., and Chen, P. (2018). Changes in Inflammatory and Oxidative Stress Factors and the Protein Synthesis Pathway in Injured Skeletal Muscle after Contusion. Exp. Ther. Med. 15, 2196–2202. doi:10.3892/etm.2017.5625
Mackinnon, L. T. (1994). Current Challenges and Future Expectations in Exercise Immunology: Back to the Future. Med. Sci. Sports Exerc. 26, 191–194. doi:10.1249/00005768-199402000-00009
Mashiko, T., Umeda, T., Nakaji, S., and Sugawara, K. (2004). Position Related Analysis of the Appearance of and Relationship between post-match Physical and Mental Fatigue in university rugby Football Players. Br. J. Sports Med. 38, 617–621. doi:10.1136/bjsm.2003.007690
Matsuyama, W., Nakagawa, M., Takashima, H., Muranaga, F., Sano, Y., and Osame, M. (2001). Molecular Analysis of Hereditary Deficiency of the Third Component of Complement (C3) in Two sisters. Intern. Med. 40, 1254–1258. doi:10.2169/internalmedicine.40.1254
Medzhitov, R., and Janeway, C. A. (1998). An Ancient System of Host Defense. Curr. Opin. Immunol. 10, 12–15. doi:10.1016/s0952-7915(98)80024-1
Mitchell, J. H., Haskell, W., Snell, P., and Van Camp, S. P. (2005). Task Force 8: Classification of Sports. J. Am. Coll. Cardiol. 45, 1364–1367. doi:10.1016/j.jacc.2005.02.015
Mochizuki, M., Suzuki, K., Nakaji, S., Sugawara, K., Totsuka, M., and Sato, K. (1999). Effects of Maximal Exercise on Nonspecific Immunity in Athletes under Trained and Detrained Conditions. Tairyoku Kagaku 48, 147–159. doi:10.7600/jspfsm1949.48.147
Nieman, D. C. (1997). Exercise Immunology: Practical Applications. Int. J. Sports Med. 18 Suppl 1 (Suppl. 1), S91–S100. doi:10.1055/s-2007-972705
Nieman, D. C., and Wentz, L. M. (2019). The Compelling Link between Physical Activity and the Body's Defense System. J. Sport Health Sci. 8, 201–217. doi:10.1016/j.jshs.2018.09.009
Nieman, D., Tan, S., Lee, J., and Berk, L. (1989). Complement and Immunoglobulin Levels in Athletes and Sedentary Controls. Int. J. Sports Med. 10, 124–128. doi:10.1055/s-2007-1024887
Noris, M., and Remuzzi, G. (2013). Overview of Complement Activation and Regulation. Semin. Nephrol. 33, 479–492. doi:10.1016/j.semnephrol.2013.08.001
Ohtsuki, T., Satoh, K., Shimizu, T., Ikeda, S., Kikuchi, N., Satoh, T., et al. (2019). Identification of Adipsin as a Novel Prognostic Biomarker in Patients with Coronary Artery Disease. J. Am. Heart Assoc. 8, e013716. doi:10.1161/JAHA.119.013716
Peake, J. M., Neubauer, O., Della Gatta, P. A., and Nosaka, K. (2017). Muscle Damage and Inflammation during Recovery from Exercise. J. Appl. Physiol. (1985) 122, 559–570. doi:10.1152/japplphysiol.00971.2016
Peake, J. M., Neubauer, O., Walsh, N. P., and Simpson, R. J. (2017). Recovery of the Immune System after Exercise. J. Appl. Physiol. (1985) 122, 1077–1087. doi:10.1152/japplphysiol.00622.2016
Pedersen, B. K., and Hoffman-Goetz, L. (2000). Exercise and the Immune System: Regulation, Integration, and Adaptation. Physiol. Rev. 80, 1055–1081. doi:10.1152/physrev.2000.80.3.1055
Prohászka, Z., Kirschfink, M., and Frazer-Abel, A. (2018). Complement Analysis in the Era of Targeted Therapeutics. Mol. Immunol. 102, 84–88. doi:10.1016/j.molimm.2018.06.001
Qiu, Y., Wang, S.-F., Yu, C., Chen, Q., Jiang, R., Pei, L., et al. (2019). Association of Circulating Adipsin, Visfatin, and Adiponectin with Nonalcoholic Fatty Liver Disease in Adults: A Case-Control Study. Ann. Nutr. Metab. 74, 44–52. doi:10.1159/000495215
Ricklin, D., Hajishengallis, G., Yang, K., and Lambris, J. D. (2010). Complement: a Key System for Immune Surveillance and Homeostasis. Nat. Immunol. 11, 785–797. doi:10.1038/ni.1923
Samjoo, I. A., Safdar, A., Hamadeh, M. J., Raha, S., and Tarnopolsky, M. A. (2013). The Effect of Endurance Exercise on Both Skeletal Muscle and Systemic Oxidative Stress in Previously Sedentary Obese Men. Nutr. Diabetes 3, e88. doi:10.1038/nutd.2013.30
Sarma, J. V., and Ward, P. A. (2011). The Complement System. Cell Tissue Res. 343, 227–235. doi:10.1007/s00441-010-1034-0
Saygin, O., Karacabey, K., Ozmerdivenli, R., Zorba, E., Ilhan, F., and Bulut, V. (2006). Effect of Chronic Exercise on Immunoglobin, Complement and Leukocyte Types in Volleyball Players and Athletes. Neuro Endocrinol. Lett. 27, 271–276.
Schifferli, J. A., Ng, Y. C., and Peters, D. K. (1986). The Role of Complement and its Receptor in the Elimination of Immune Complexes. N. Engl. J. Med. 315, 488–495. doi:10.1056/NEJM198608213150805
Sellami, M., Al-Muraikhy, S., Al-Jaber, H., Al-Amri, H., Al-Mansoori, L., Mazloum, N. A., et al. (2021). Age and Sport Intensity-dependent Changes in Cytokines and Telomere Length in Elite Athletes. Antioxidants (Basel) 10, 1035. doi:10.3390/antiox10071035
Sellami, M., Bragazzi, N. L., Aboghaba, B., and Elrayess, M. A. (2021). The Impact of Acute and Chronic Exercise on Immunoglobulins and Cytokines in Elderly: Insights from a Critical Review of the Literature. Front. Immunol. 12, 631873. doi:10.3389/fimmu.2021.631873
Shammas, M. A. (2011). Telomeres, Lifestyle, Cancer, and Aging. Curr. Opin. Clin. Nutr. Metab. Care 14, 28–34. doi:10.1097/mco.0b013e32834121b1
Shivshankar, P., Fekry, B., Eckel-Mahan, K., and Wetsel, R. A. (2020). Circadian Clock and Complement Immune System-Complementary Control of Physiology and Pathology? Front. Cel. Infect. Microbiol. 10, 418. doi:10.3389/fcimb.2020.00418
Silverman, M. N., and Deuster, P. A. (2014). Biological Mechanisms Underlying the Role of Physical Fitness in Health and Resilience. Interf. Focus. 4, 20140040. doi:10.1098/rsfs.2014.0040
Skattum, L., van Deuren, M., van der Poll, T., and Truedsson, L. (2011). Complement Deficiency States and Associated Infections. Mol. Immunol. 48, 1643–1655. doi:10.1016/j.molimm.2011.05.001
Smith, J. K., Chi, D. S., Krish, G., Reynolds, S., and Cambron, G. (1990). Effect of Exercise on Complement Activity. Ann. Allergy 65, 304–310.
Sohail, M. U., Al-Mansoori, L., Al-Jaber, H., Georgakopoulos, C., Donati, F., Botrè, F., et al. (2020). Assessment of Serum Cytokines and Oxidative Stress Markers in Elite Athletes Reveals Unique Profiles Associated with Different Sport Disciplines. Front. Physiol. 11, 600888. doi:10.3389/fphys.2020.600888
Stahl, G. L., Xu, Y., Hao, L., Miller, M., Buras, J. A., Fung, M., et al. (2003). Role for the Alternative Complement Pathway in Ischemia/reperfusion Injury. Am. J. Pathol. 162, 449–455. doi:10.1016/s0002-9440(10)63839-4
Tafere, G. G., Wondafrash, D. Z., Zewdie, K. A., Assefa, B. T., and Ayza, M. A. (2020). Plasma Adipsin as a Biomarker and its Implication in Type 2 Diabetes Mellitus. Diabetes Metab. Syndr. Obes. 13, 1855–1861. doi:10.2147/dmso.s253967
Wang, Y., Zheng, X., Xie, X., Qian, W., Zhang, L., and Ren, W. (2019). Correlation of Increased Serum Adipsin with Increased Cardiovascular Risks in Adult Patients with Growth Hormone Deficiency. Endocr. Pract. 25, 446–453. doi:10.4158/ep-2018-0541
Wu, X., Lin, L., Cui, J., Chen, Y., Yang, L., and Wan, J. (2020). Complement C3 Deficiency Ameliorates Aging Related Changes in the Kidney. Life Sci. 260, 118370. doi:10.1016/j.lfs.2020.118370
Wylleman, P., and Reints, A. (2010). A Lifespan Perspective on the Career of Talented and Elite Athletes: Perspectives on High-Intensity Sports. Scand. J. Med. Sci. Sports 20 (Suppl. 2), 88–94. doi:10.1111/j.1600-0838.2010.01194.x
Keywords: complements, endurance, elite athletes, aging, inflammatory cytokines, telomere length
Citation: Al-Muraikhy S, Ramanjaneya M, Dömling AS, Bettahi I, Donati F, Botre F, Abou-Samra A-B, Sellami M and Elrayess MA (2021) High Endurance Elite Athletes Show Age-dependent Lower Levels of Circulating Complements Compared to Low/Moderate Endurance Elite Athletes. Front. Mol. Biosci. 8:715035. doi: 10.3389/fmolb.2021.715035
Received: 26 May 2021; Accepted: 06 September 2021;
Published: 23 September 2021.
Edited by:
Silas Granato Villas-Boas, Luxembourg Institute of Science and Technology, LuxembourgReviewed by:
Katsuhiko Suzuki, Waseda University, JapanRodrigo Vanerson Passos Neves, Universidade Católica de Brasília (UCB), Brazil
Copyright © 2021 Al-Muraikhy, Ramanjaneya, Dömling, Bettahi, Donati, Botre, Abou-Samra, Sellami and Elrayess. This is an open-access article distributed under the terms of the Creative Commons Attribution License (CC BY). The use, distribution or reproduction in other forums is permitted, provided the original author(s) and the copyright owner(s) are credited and that the original publication in this journal is cited, in accordance with accepted academic practice. No use, distribution or reproduction is permitted which does not comply with these terms.
*Correspondence: Mohamed A Elrayess, bS5lbHJheWVzc0BxdS5lZHUucWE=