- 1Henan International Joint Laboratory for Nuclear Protein Regulation, School of Basic Medical Sciences, Henan University, Kaifeng, China
- 2Henan Technician College of Medicine and Health, Kaifeng, China
Ischemia/reperfusion (I/R) injury is characterized by limiting blood supply to organs, then restoring blood flow and reoxygenation. It leads to many diseases, including acute kidney injury, myocardial infarction, circulatory arrest, ischemic stroke, trauma, and sickle cell disease. Autophagy is an important and conserved cellular pathway, in which cells transfer the cytoplasmic contents to lysosomes for degradation. It plays an important role in maintaining the balance of cell synthesis, decomposition and reuse, and participates in a variety of physiological and pathological processes. Hydrogen sulfide (H2S), along with carbon monoxide (CO) and nitric oxide (NO), is an important gas signal molecule and regulates various physiological and pathological processes. In recent years, there are many studies on the improvement of I/R injury by H2S through regulating autophagy, but the related mechanisms are not completely clear. Therefore, we summarize the related research in the above aspects to provide theoretical reference for future in-depth research.
Introduction
Ischemia/reperfusion (I/R) injury is a term used to represent the functional and structural changes that become obvious during the recovery of blood flow after a period of ischemia. In addition to ischemia, the recovery of blood flow can also lead to potentially very harmful effects, including significant cell swelling, irreversible cell necrosis and uneven blood flow recovery in all parts of the tissue (Peralta et al., 2013; Soares et al., 2019). I/R injury is composed of two important events. The first one refers to the limitation of blood supply to an organ, usually due to the blockage of blood supply in the artery by an embolus. Ischemic are always associated with cell metabolic imbalance and harmful hypoxia. The second one is reperfusion, which may lead to a destructive inflammatory response to further aggravate tissue damage (Yellon and Hausenloy, 2007; Eltzschig and Eckle, 2011). The degree of tissue damage is directly to the degree of blood reduction and the length of ischemia time. Ischemia increases intracellular and mitochondrial calcium levels through disturbing ATPase dependent ion transport. At the same time, the mechanism of cell volume regulation is also destroyed by the lack of ATP, which can induce the dissolution of organelles and plasma membranes. Reperfusion, although rescuing oxygen-starved tissues, promotes the production of reactive oxygen species, isolates the proinflammatory immunocytes in ischemic tissues, and aggravates tissue damage (Kalogeris et al., 2017; Figure 1). I/R injury leads to a lot of serious clinical problems in many pathological processes including acute myocardial infarction, kidney injury (AKI), ischemic stroke, and circulatory arrest (Tang and Zhuang, 2019). Although the roles of I/R injury in many organs have been widely studied, including heart, brain and kidney, the related exact molecular mechanisms are not fully understood (Yellon and Hausenloy, 2007; Hanson et al., 2009; Angeli et al., 2014).
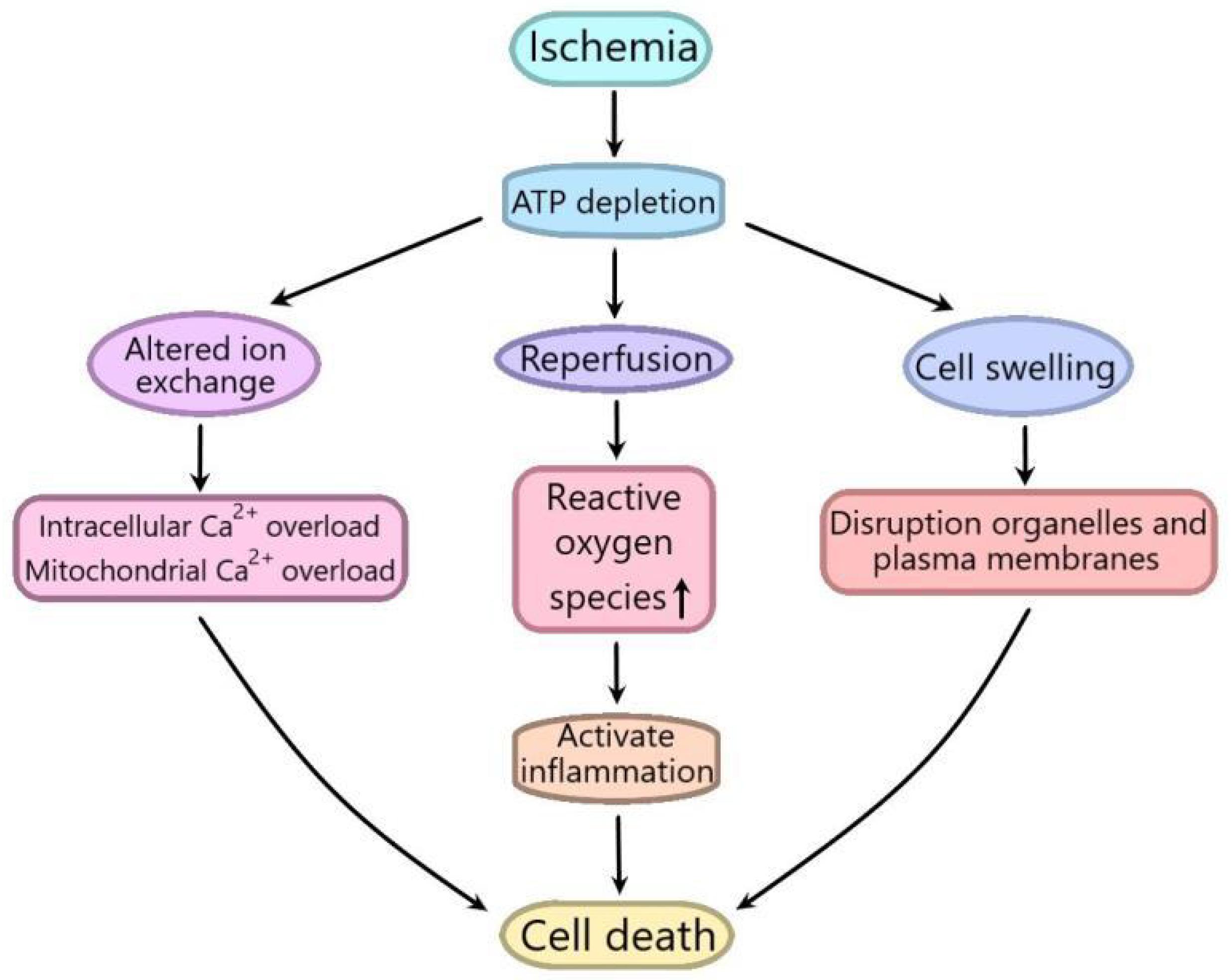
Figure 1. Schematic diagram of the mechanism of ischemia/reperfusion (I/R) injury. Ischemia increases intracellular and mitochondrial calcium levels by interfering with ATPase dependent ion transport. At the same time, the mechanism of cell volume regulation is also destroyed by the lack of ATP, which can cause the dissolution of organelles and plasma membranes. Although reperfusion can save hypoxic tissue, it can promote the production of reactive oxygen species, isolate pro-inflammatory immune cells in ischemic tissue, and aggravate tissue injury.
Autophagy is an evolutionarily conserved process in which the cytoplasm and organelles are isolated in double membrane vesicles, then transported to lysosomes/vacuoles for degradation and recycling of eukaryotic cytoplasmic components (Galluzzi et al., 2017; Guo et al., 2019). According to the differences in cargo specificity and delivery to lysosomes, autophagy is divided into three types: macroautophagy, microautophagy and chaperone-mediated autophagy (Gomes et al., 2017). In macroautophagy (also known as autophagy), cytoplasmic components are engulfed in double membranous vesicles to form autophagosomes. Autophagosomes then fuse with lysosomes to form autolysosomes in which cargoes are degraded or recycled (Mizushima and Komatsu, 2011; Galluzzi et al., 2017; Bonam et al., 2019); Microautophagy refers to the direct invagination of lysosomal membrane and encapsulation of cell contents (Mijaljica et al., 2011); Chaperone-mediated autophagy is a kind of selective autophagy, in which proteins in cells are binded with chaperones, then transported to lysosomal chambers for deradation (Fujiwara et al., 2017; Kaushik and Cuervo, 2018). Autophagy plays an important role in organ homeostasis, anti-aging mechanism, and immune response (Mizushima et al., 2011; Deretic et al., 2013; Kaushik and Cuervo, 2015). Autophagy can be induced by hypoxia, ischemia, pathogen infection, protein misfolding, hormone therapy, nutritional deficiency, and other internal and external factors (Matsui et al., 2007; Ravanan et al., 2017). Abnormal autophagy plays an important role in many pathological processes including ischemia-reperfusion injury (Wang et al., 2019), inflammatory and infectious diseases, obesity and type 2 diabetes, cancer, and neurodegenerative diseases (Choi et al., 2013; Jiang and Mizushima, 2014). The relevant mechanism has not been fully understood.
Hydrogen sulfide (H2S) has always been considered as a toxic pollutant, but recently it, together with nitric oxide (NO) and carbon monoxide (CO), is regarded as a biological signal molecule, namely gas transmitter (Szabo, 2007; Powell et al., 2018). To date, it is generally believed that endogenous H2S is mainly produced by three enzymes, namely cystathionine-β-synthase (CBS), cystathionine-γ-lyase (CSE), as well as 3-mercaptopyruvate sulfurtransferase (3-MST) (Polhemus and Lefer, 2014). H2S has many physiological functions, including anti-inflammatory (Du et al., 2014), anti-apoptotic (Guo et al., 2014), relaxing blood vessels, reducing blood pressure (Yang et al., 2008; Sun et al., 2015) and anti oxidative stress (Zheng et al., 2015). It has been reported that during I/R, the structure of mitochondria is damaged and the production of reactive oxygen species (ROS) increased, H2S can protect the integrity of mitochondria and reduce the production of mitochondrial reactive oxygen species (mtROS), thus alleviating I/R injury (Andreadou et al., 2020). In recent years, there are many studies on the effects of H2S on autophagy to improve I/R injury, but its mechanism is not fully clear. Therefore, we summarize the relevant research in the above aspects to provide theoretical reference for future in-depth research.
Exogenous H2S Plays an Important Role Through Regulating Autophagy in Hepatic I/R Injury
Hepatic I/R injury usually occurs in the process of shock and liver transplantation, which is an important clinical problem and a serious threat to human health (Dogan and Aslan, 2011). Therefore, how to improve hepatic I/R injury is increasingly important. Hepatic I/R can induce autophagy, and the suppression of autophagy alleviates hepatic I/R injury (Shen et al., 2013). Exogenous H2S has been reported to play a protective role against hepatic I/R injury (Kang et al., 2010). In order to investigate the effects of exogenous H2S on autophagy in hepatic I/R injury and the related mechanism, Cheng et al. (2014) conducted a series of experiments and found that exogenous H2S improved hepatic I/R injury by notably decreasing I/R-induced serum levels of alanine aminotransferase (ALT), aspartate transaminase (AST) and inflammatory cytokines, improving pathological changes of liver and reducing hepatocyte apoptosis. The mechanism research revealed that autophagy was enhanced in I/R-induced hepatitis, which could be reversed by exogenous H2S. Moreover, exogenous H2S inhibited c-Jun NH2-terminal kinases (JNK) pathway induced by I/R injury through decreasing JNK1 and extracellular signal-regulated kinase (ERK) phosphorylation, while SP600125, a JNK1 inhibitor, strengthened H2S hepatoprotective effects, indicating that autophagy and JNK pathway are involved in the hepatoprotective effect of exogenous H2S in hepatic I/R injury (Cheng et al., 2014). The evidences indicate that the suppression of JNK activation with SP600125 can block autophagy (Rodriguez-Enriquez et al., 2006), so it can be inferred that exogenous H2S improve hepatic I/R injury by inhibiting autophagy through suppressing JNK pathway. Apoptosis interacts with autophagy. The activation of autophagy can inhibit apoptosis, while the inhibition of autophagy can promote cell death and apoptosis (Wang et al., 2012). It has been reported that the upregulation of autophagy can reduce hepatic I/R injury (Cardinal et al., 2009), which is contradicts the above inference that inhibiting autophagy improves hepatic I/R injury. In the above I/R-induced hepatitis, 3-MA, an autophagy inhibitor, further decreased H2S-inhibited autophagy, which abolished the protective effect of exogenous H2S against I/R-induced hepatic injury. Whereas rapamycin, an autophagy enhancer, had the opposite effect. This seems to contradict the previous inference that exogenous H2S improves hepatic I/R injury by inhibiting autophagy (Cheng et al., 2014). The reason may be that the regulation of autophagy by exogenous H2S has two-sided effects in liver I/R injury. The moderate inhibition of autophagy by H2S has cytoprotective effect, while the excessive inhibition of autophagy shows the opposite result. Scavenger receptor A (SRA) is one of the main receptors involved in macrophage-mediated inflammatory response, which plays an important role in cerebral ischemia injury (Ohnishi et al., 2011; Xu et al., 2012). The activation of SRA has been reported to suppress autophagy in macrophages (Huang et al., 2014). Exogenous H2S could improve fatty liver I/R injury through mitigating the pathological changes of liver tissue and decreasing the levels of ALT, AST, and lactate dehydrogenase (LDH). The mechanism research showed that exogenous H2S promoted the autophagy of peritoneal macrophages by increasing the concentration of LC3B particles and the ratio of LC3-II/LC3-I in fatty liver I/R injury. Exogenous H2S also inhibited inflammation, apoptosis and oxidative stress, and decreased the protein expression of SRA in fatty liver I/R injury, suggesting that exogenous H2S alleviated hepatic I/R injury by enhancing autophagy through suppressing SRA pathway, which needed to be further studied by using the related inhibitors (Ruan et al., 2019). SRA pathway may be an important target in the effects of H2S on autophagy in hepatic I/R injury.
Exogenous H2S Plays an Important Role Through Regulating Autophagy in Myocardial I/R Injury
Rapid coronary artery reperfusion is a common treatment for myocardial ischemia. However, reperfusion itself can lead to injury, which is called myocardial I/R injury (Chang et al., 2013). Therefore, it is necessary to find a new way to reduce myocardial I/R injury. Myocardial ischemia enhances Adenosine monophosphate -activated protein kinase (AMPK) activation and induces protective autophagy (Matsui et al., 2007), the mechanism of which is not clear. Hong Xie, et al. found that exogenous H2S could improve myocardial I/R injury by reducing myocardial infarction. Myocardial I/R induced the formation of autophagosome, impaired the clearance of autophagosome, decreased the levels of p-AMPK/AMPK, LC3-II/I and beclin-1, and increased p62 and LAMP-2 protein levels, while exogenous H2S reversed these changes, indicating that exogenous H2S promoted autophagy and activated AMPK pathway. Moreover, the AMPK inhibitor Compound C and autophagy inhibitor CQ abolished the cardioprotective effect of exogenous H2S on myocardial I/R injury, indicating that exogenous H2S improved myocardial I/R injury by promoting AMPK-mediated autophagy impaired by I/R injury (Xie et al., 2015). AMPK-mediated autophagy plays a dual role in the process of myocardial I/R injury. Myocardial ischemia can up- regulate AMPK- mediated autophagy to play a protective role, while subsequent reperfusion has the opposite effect. Contrary to the above conclusion that enhanced autophagy by H2S can ameliorate myocardial I/R injury, enhancing autophagy may lead to myocardial cell death through excessive self-digestion and degradation of essential cell components (Matsui et al., 2007; Hariharan et al., 2011). After myocardial hypoxia/reoxygenation (H/R), autophagy increased significantly, while exogenous H2S could suppress autophagy to protect myocardium. Blocking phosphatidylinositol-3-kinase (PI3K) with LY294002, a PI3K inhibitor, or knocking down Serum- and glucocorticoid-induced kinase 1 (SGK1) with SGK1 siRNA could enhance autophagy and weakened the anti-autophagy and cardioprotection effects of exogenous H2S, while the inhibition of glycogen synthase kinase-3 beta (GSK3β) with tws119, a GSK3β inhibitor, had the opposite effects. Collectively, exogenous H2S plays a cardioprotective role in neonatal rat cardiomyocytes exposed to H/R by inhibiting autophagy through PI3K/SGK1/GSK3β signaling pathway (Jiang et al., 2016).
Exogenous H2S Plays an Important Role Through Regulating Autophagy in Cerebral I/R Injury
Cerebral infarction is the leading cause of death and permanent disability of adults all over the world (Yan et al., 2016). The injury of brain cells caused by cerebral ischemia is further aggravated after the recovery of blood supply, which is called cerebral I/R injury (Molina and Alvarez-Sabin, 2009). Autophagy has been reported to be involved in cerebral I/R injury (Fan et al., 2016). The research of Shui et al. (2016) showed that exogenous H2S alleviated the cerebral I/R injury through decreasing cortex infarct in middle cerebral artery occlusion (MCAO) mice, while autophagy activator rapamycin alleviated the protective effects of H2S, and the autophagy inhibitor 3-MA had the similar results to exogenous H2S, suggesting that autophagy was involved in the protective effects of exogenous H2S. Exogenous H2S suppressed the elevation of LC3-II and the decrease of p62, but had no notably effect on Beclin-1 complex in cerebral I/R injury model mice, indicating that exogenous H2S inhibited autophagy through decreasing autophagosome accumulation. From the above, it can be deduced that exogenous H2S reduced cerebral cortex I/R injury through inhibiting autophagy (Shui et al., 2016). The decrease of autophagosome accumulation leaded by exogenous H2S may be due to the decrease of autophagosome synthesis or the acceleration of autophagosome degradation (Klionsky et al., 2008; Bonam et al., 2020), therefore, in order to determine whether exogenous H2S suppresses autophagy by decreasing autophagosome synthesis or increasing autophagosome degradation, Zhu et al. (2017) employed SH-SY5Y cells for the oxygen and glucose deprivation/reoxygenation (OGD/R) and mice for the cerebral I/R to performed in vitro and in vivo experiments, respectively, and found exogenous H2S notably decreased cerebral infarct volume, increased cell viability and downregulated the OGD/R-induced elevation in LC3-II, which was abolished by co-treatment with the autophagy maturation inhibitor bafilomycin A1 (BafA1). Moreover, exogenous H2S had no influences on the -induced upregulation of the ULK1 self-association and reduction of the ATG13 phosphorylation induced by OGD/R, which are vital for the initiation of autophagosome formation. Taken together, it can be inferred that exogenous H2S played neuroprotection against cerebral I/R injury through increasing autophagosome degradation (Zhu et al., 2017). To further prove the protective effect of H2S on cerebral I/R injury by suppressing autophagy, Jiang et al. (2017) exposed PC12 cells to hypoxia glucose deprivation/reoxygenation (OGD/R) in vitro to simulate MCAO and found that exogenous H2S alleviated OGD/R-induced cellular injury of PC12 cells by reducing apoptosis and inhibited autophagy induced by OGD/R by reducing the ratio of LC3-II/I and increasing the expression of p62. Rapamycin abolished the protective effects of exogenous H2S on OGD/R-induced cellular injury of PC12 cells, and the autophagy inhibitor 3-MA had the similar results to H2S, suggesting that exogenous H2S improved the cellular injury of PC12 cells induced by OGD/R through inhibiting autophagy (Jiang et al., 2017). Previous study has shown that exogenous H2S improves cerebral I/R injury through inhibiting oxidative stress and apoptosis (Yu et al., 2015). Whether exogenous H2S alleviates cerebral I/R injury by inhibiting autophagy via suppressing oxidative stress and apoptosis needs to be elucidated.
Exogenous H2S Plays an Important Role Through Regulating Autophagy in Renal I/R Injury
Renal I/R injury is a typical complication of kidney injury after organ transplantation, which leads to accumulation of toxic metabolites, depletion of adenosine triphosphate (ATP), and subsequent tissue injury. In the process of renal transplantation, the transient stop of renal blood flow leads to acute ischemic injury, and the subsequent reperfusion further deepens the functional and structural damage of human kidney (Wang et al., 2004; Wu et al., 2007). At present, it is very urgent to explore the effective prevention of renal I/R injury. Ling et al. (2017) found that in renal I/R injury patients, 24 h after I/R, the levels of IL-6, transforming growth factor-beta (TGF-β), LC3II/I, endoplasmic reticulum stress pathway related genes, apoptotic index and autophagosome number increased significantly, the levels of p62 and SR-A, superoxide dismutase (SOD) and H2S concentration decreased significantly, while exogenous H2S significantly reversed the changes. SR-A gene knockout had the similar effects to exogenous H2S (Ling et al., 2017), Moreover, exogenous H2S alleviated hepatic I/R injury by enhancing autophagy through suppressing SRA pathway (Ruan et al., 2019). Therefore, it can be inferred that exogenous H2S improved renal I/R injury by inhibiting autophagy through suppressing SRA pathway, which needed further studied. In particularly, the mechanism of exogenous H2S regulating SRA pathway remains to be elucidated.
Exogenous H2S Plays an Important Role Through Regulating Autophagy in Spinal Cord I/R Injury
Spinal cord I/R injury is a dynamic process, which often occurs in various clinical conditions, such as thoracoabdominal artery surgery or spinal cord injury. Its pathogenesis is very complex including membrane permeability changes, lipid oxidation and so on. Therefore, it is difficult to achieve the purpose of effective treatment (Ege et al., 2004; Korkmaz et al., 2013). The results of Li et al. (2015) showed that exogenous H2S ameliorated motor function and infarct area, promoted autophagy by upregulating the expression levels of beclin-1 and LC3II, and downregulating miR-30c expression in rat spinal cord I/R injury model, indicating that autophagy and miR-30c were involved in exogenous H2S improvement of spinal cord I/R injury. Exogenous H2S also improved oxygen glucose deprivation (OGD)-induced ischemic injury in SY-SH-5Y cells (Li et al., 2015). It has been reported that miR-30c can regulate autophagy (Wang et al., 2014). The results showed that miR-30c negatively regulated Beclin-1 expression in SY-SH-5Y cells treated with OGD. Moreover, the spinal cord improvement of exogenous H2S was reversed by 3-MA and miR-30c overexpression, suggesting that exogenous H2S ameliorated spinal cord I/R injury by promoting autophagy via suppressing miR-30c, which might have potential clinical value in the treatment of spinal cord I/R injury (Zhu et al., 2017). The regulation of microRNA on autophagy needs to be further studied.
Conclusion
In this review, the effects of exogenous H2S on autophagy in I/R injury was summarized as follows: (1) Exogenous H2S improve hepatic I/R injury by inhibit autophagy through suppressing JNK pathway; (2) Exogenous H2S alleviated hepatic I/R injury by enhancing autophagy through suppressing SRA pathway; (3) Exogenous H2S improved myocardial I/R injury by promoting AMPK-mediated autophagy or by inhibiting autophagy through PI3K/SGK1/GSK3β pathway; (4) Exogenous H2S reduced cerebral I/R injury through inhibiting autophagy via promoting autophagosome degradation; (5) Exogenous H2S improved renal I/R injury by inhibiting autophagy through suppressing SRA pathway; and (6) Exogenous H2S ameliorated spinal cord I/R injury by promoting autophagy via suppressing miR-30c (Table 1). It can be concluded that exogenous H2S can improve ischemia-reperfusion injury by inhibiting autophagy or promoting autophagy. This difference may be due to the different basic levels of autophagy in different tissues, which leads to different responses of autophagy to ischemia-reperfusion injury. In addition, the mechanism of the effects of H2S on autophagy and the response mechanism of autophagy to ischemia-reperfusion injury need to be further elucidated.
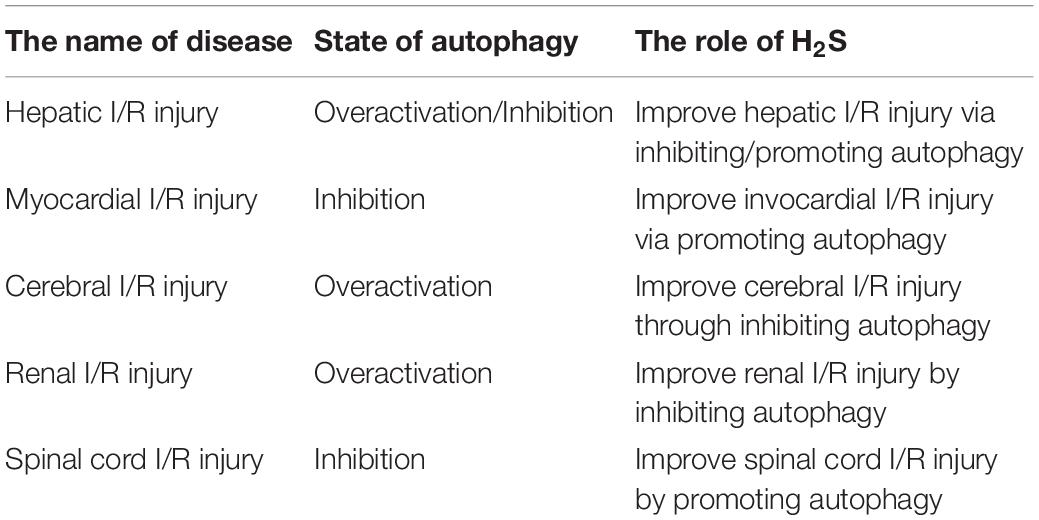
Table 1. The effects of hydrogen sulfide (H2S) on autophagy in different ischemia/reperfusion (I/R) injury.
With the development of research, the effect of exogenous H2S on autophagy may provide a new strategy for the prevention and treatment of ischemia-reperfusion injury.
Author Contributions
HW revised, wrote, and funded the manuscript. SL wrote and funded the manuscript. ZW and JW wrote the manuscript. All authors contributed to the article and approved the submitted version.
Funding
This work was supported by grants from the Key Scientific and Technological Projects in Henan Province, China (Grant No. 202102310153), Natural Science Foundation for Excellent Young Scholars of Henan Province (Grant No. 212300410026), and National Natural Science Foundation of China (Grant No. 81971280).
Conflict of Interest
The authors declare that the research was conducted in the absence of any commercial or financial relationships that could be construed as a potential conflict of interest.
References
Andreadou, I., Schulz, R., Papapetropoulos, A., Turan, B., Ytrehus, K., Ferdinandy, P., et al. (2020). The role of mitochondrial reactive oxygen species, NO and H2S in ischaemia/reperfusion injury and cardioprotection. J. Cell Mol. Med. 24, 6510–6522. doi: 10.1111/jcmm.15279
Angeli, J. P. F., Schneider, M., Proneth, B., Tyurina, Y. Y., Tyurin, V. A., and Hammond, V. J. (2014). Inactivation of the ferroptosis regulator Gpx4 triggers acute renal failure in mice. Nat. Cell Biol. 16, 1180–U1120.
Bonam, S. R., Wang, F., and Muller, S. (2019). Lysosomes as a therapeutic target. Nat. Rev. Drug Discov. 18, 923–948. doi: 10.1038/s41573-019-0036-1
Bonam, S.R., Bayry, J., Tschan, M. P., and Muller, S. (2020). Progress and challenges in the use of MAP1LC3 as a legitimate marker for measuring dynamic autophagy in vivo. Cells 9:1321. doi: 10.3390/cells9051321
Cardinal, J., Pan, P., Dhupar, R., Ross, M., Nakao, A., Lotze, M., et al. (2009). Cisplatin prevents high mobility group box 1 release and is protective in a murine model of hepatic ischemia/reperfusion injury. Hepatology 50, 565–574. doi: 10.1002/hep.23021
Chang, R., Li, Y., Yang, X., Yue, Y., Dou, L., Wang, Y., et al. (2013). Protective role of deoxyschizandrin and schisantherin a against myocardial ischemia-reperfusion injury in rats. PLoS One 8:e61590 doi: 10.1371/journal.pone.0061590
Cheng, P., Wang, F., Chen, K., Shen, M., Dai, W., and Xu, L. (2014). Hydrogen sulfide ameliorates ischemia/reperfusion-induced hepatitis by inhibiting apoptosis and autophagy pathways. Mediators Inflamm. 2014:935251.
Choi, A. M. K., Ryter, S. W., and Levine, B. (2013). Mechanisms of Disease autophagy in human health and disease. N. Engl. J. Med. 368, 651–662.
Deretic, V., Saitoh, T., and Akira, S. (2013). Autophagy in infection, inflammation and immunity. Nat. Rev. Immunol. 13, 722–737.
Dogan, S., and Aslan, M. (2011). Hepatic ischemia-reperfusion injury and therapeutic strategies to alleviate cellular damage. Hepatol. Res. 41, 103–117. doi: 10.1111/j.1872-034x.2010.00765.x
Du, J., Huang, Y., Yan, H., Zhang, Q., Zhao, M., Zhu, M., et al. (2014). Hydrogen sulfide suppresses oxidized low-density lipoprotein (Ox-LDL)-stimulated Monocyte Chemoattractant Protein 1 generation from Macrophages via the nuclear factor kB (NF- kB) pathway∗. J. Biol. Chem. 289, 9741–9753. doi: 10.1074/jbc.m113.517995
Ege, E., Ilhan, A., Gurel, A., Akyol, O., and Ozen, S. (2004). Erdosteine ameliorates neurological outcome and oxidative stress due to ischemia/reperfusion injury in rabbit spinal cord. Eur. J. Vasc. Endovasc. Surg. 28, 379–386. doi: 10.1016/j.ejvs.2004.06.004
Eltzschig, H. K., and Eckle, T. (2011). Ischemia and reperfusion-from mechanism to translation. Nat. Med. 17, 1391–1401. doi: 10.1038/nm.2507
Fan, T., Huang, Z., Chen, L., Wang, W., Zhang, B., Xu, Y., et al. (2016). Associations between autophagy, the ubiquitin-proteasome system and endoplasmic reticulum stress in hypoxia-deoxygenation or ischemia-reperfusion. Eur. J. Pharmacol. 791, 157–167. doi: 10.1016/j.ejphar.2016.08.026
Fujiwara, Y., Wada, K., and Kabuta, T. (2017). Lysosomal degradation of intracellular nucleic acids-multiple autophagic pathways. J. Biochem. 161, 145–154.
Galluzzi, L., Baehrecke, E. H., Ballabio, A., Boya, P., Pedro, J. M., and Cecconi, F. (2017). Molecular definitions of autophagy and related processes. Embo J. 36, 1811–1836.
Gomes, L. R., Menck, C. F. M., and Cuervo, A. M. (2017). Chaperone-mediated autophagy prevents cellular transformation by regulating MYC proteasomal degradation. Autophagy 13, 928–940. doi: 10.1080/15548627.2017.1293767
Guo, C., Liang, F., Masood, W. S., and Yan, X. (2014). Hydrogen sulfide protected gastric epithelial cell from ischemia/reperfusion injury by Keapl s-sulfhydration, MAPK dependent anti-apoptosis and NF-kappa B dependent anti-inflammation pathway. Eur. J. Pharmacol. 725, 70–78. doi: 10.1016/j.ejphar.2014.01.009
Guo, Y., Zhang, X., Wu, T., Hu, X., Su, J., and Chen, X. (2019). Autophagy in skin diseases. Dermatology 235, 380–389. doi: 10.1159/000500470
Hanson, L. R., Roeytenberg, A., Martinez, P. M., Coppes, V. G., Sweet, D. C., and Rao, R. J. (2009). Intranasal deferoxamine provides increased brain exposure and significant protection in rat ischemic stroke. J. Pharmacol. Exp. Ther. 330, 679–686. doi: 10.1124/jpet.108.149807
Hariharan, N., Zhai, P., and Sadoshima, J. (2011). Oxidative stress stimulates autophagic flux during ischemia/reperfusion. Antioxid. Redox Signal. 14, 2179–2190. doi: 10.1089/ars.2010.3488
Huang, H., Li, X., Zhuang, Y., Li, N., Zhu, X., Hu, J., et al. (2014). Class a scavenger receptor activation inhibits endoplasmic reticulum stress-induced autophagy in macrophage. J. Biomed. Res. 28, 213–221.
Jiang, H., Xiao, J., Kang, B., Zhu, X., Ni, X., and Wang, Z. (2016). PI3K/SGK1/GSK3 beta signaling pathway is involved in inhibition of autophagy in neonatal rat cardiomyocytes exposed to hypoxia/reoxygenation by hydrogen sulfide. Exp. Cell Res. 345, 134–140. doi: 10.1016/j.yexcr.2015.07.005
Jiang, W. -W., Huang, B. S., Han, Y., Deng, L. H., and Wu, L. X. (2017). Sodium hydrosulfide attenuates cerebral ischemia/reperfusion injury by suppressing overactivated autophagy in rats. Febs Open Bio 7, 1686–1695. doi: 10.1002/2211-5463.12301
Kalogeris, T., Baines, C. P., Krenz, M., and Korthuis, R. J. (2017). Ischemia/reperfusion. Compr. Physiol. 7, 113–170.
Kang, K., Jiang, H. C., Zhao, M. Y., Sun, X. Y., and Pan, S. H. (2010). Protection of CSE/H2S system in hepatic ischemia reperfusion injury in rats. Zhonghua wai ke za zhi 48, 924–928.
Kaushik, S., and Cuervo, A. M. (2018). The coming of age of chaperone-mediated autophagy. Nat. Rev. Mol. Cell Biol. 19, 365–381. doi: 10.1038/s41580-018-0001-6
Klionsky, D. J., Abeliovich, H., Agostinis, P., Agrawal, D. K., Aliev, G., and Askew, D. S. (2008). Guidelines for the use and interpretation of assays for monitoring autophagy in higher eukaryotes. Autophagy 4, 151–175.
Korkmaz, K., Gedik, H. S., Budak, A. B., Erdem, H., Lafci, G., Karakilic, E., et al. (2013). Effect of heparin on neuroprotection against spinal cord ischemia and reperfusion in rats. Eur. Rev. Med. Pharmacol. Sci. 17, 522–530.
Li, L., Jiang, H. K., Li, Y. P., and Guo, Y. P. (2015). Hydrogen sulfide protects spinal cord and induces autophagy via miR-30c in a rat model of spinal cord ischemia-reperfusion injury. J. Biomed. Sci. 22:50.
Ling, Q., Yu, X., Wang, T., Wang, S. G., Ye, Z. Q., and Liu, J. H. (2017). Roles of the Exogenous H2S-Mediated SR-A signaling pathway in renal ischemia/reperfusion injury in regulating endoplasmic reticulum stress-induced Autophagy in a rat model. Cell Physiol. Biochem. 41, 2461–2474. doi: 10.1159/000475915
Matsui, Y., Takagi, H., Qu, X., Abdellatif, M., Sakoda, H., Asano, T., et al. (2007). Distinct roles of autophagy in the heart during ischemia and reperfusion - roles of AMP-activated protein kinase and Beclin 1 in mediating autophagy. Circ. Res. 100, 914–922. doi: 10.1161/01.res.0000261924.76669.36
Mijaljica, D., Prescott, M., and Devenish, R. J. (2011). Microautophagy in mammalian cells revisiting a 40-year-old conundrum. Autophagy 7, 673–682. doi: 10.4161/auto.7.7.14733
Mizushima, N., and Komatsu, M. (2011). Autophagy: renovation of cells and tissues. Cell 147, 728–741. doi: 10.1016/j.cell.2011.10.026
Mizushima, N., Yoshimori, T., and Ohsumi, Y. (2011). The role of atg proteins in autophagosome formation. Annu. Rev. Cell Dev. Biol. 27, 107–132 doi: 10.1146/annurev-cellbio-092910-154005
Molina, C. A., and Alvarez-Sabin, J. (2009). Recanalization and reperfusion therapies for acute Ischemic stroke. Cerebrovasc. Dis. 27, 162–167. doi: 10.1159/000200455
Ohnishi, K., Komohara, Y., Fujiwara, Y., Takemura, K., Lei, X., Nakagawa, T., et al. (2011). Suppression of TLR4-mediated inflammatory response by macrophage class a scavenger receptor (CD204). Biochem. Biophys. Res. Commun. 411, 516–522. doi: 10.1016/j.bbrc.2011.06.161
Peralta, C., Jimenez-Castro, M. B., and Gracia-Sancho, J. (2013). Hepatic ischemia and reperfusion injury: effects on the liver sinusoidal milieu. J. Hepatol. 59, 1094–1106. doi: 10.1016/j.jhep.2013.06.017
Polhemus, D. J., and Lefer, D. J. (2014). Emergence of hydrogen sulfide as an endogenous gaseous signaling molecule in cardiovascular disease. Circ. Res. 114, 730–737. doi: 10.1161/circresaha.114.300505
Powell, C. R., Dillon, K. M., and Matson, J. B. (2018). A review of hydrogen sulfide (H2S) donors: chemistry and potential therapeutic applications. Biochem. Pharmacol. 149, 110–123. doi: 10.1016/j.bcp.2017.11.014
Ravanan, P., Srikumar, I. F., and Talwar, P. (2017). Autophagy: the spotlight for cellular stress responses. Life Sci. 188, 53–67. doi: 10.1016/j.lfs.2017.08.029
Rodriguez-Enriquez, S., Kim, I., Currin, R. T., and Lemasters, J. J. (2006). Tracker dyes to probe mitochondrial autophagy (mitophagy) in rat hepatocytes. Autophagy 2, 39–46. doi: 10.4161/auto.2229
Ruan, Z., Liang, M., Deng, X., Lai, M., Shang, L., and Su, X. (2019). Exogenous hydrogen sulfide protects fatty liver against ischemia-reperfusion injury by regulating endoplasmic reticulum stress-induced autophagy in macrophage through mediating the class a scavenger receptor pathway in rats. Cell Biology International. doi: 10.1002/cbin.11234 Online ahead of print.
Shen, M., Lu, J., Dai, W., Wang, F., Xu, L., and Chen, K. (2013). Ethyl Pyruvate Ameliorates Hepatic Ischemia-Reperfusion injury by inhibiting intrinsic pathway of apoptosis and autophagy. Mediators Inflamm. 2016:5434275.
Shui, M., Liu, X., Zhu, Y., and Wang, Y. (2016). Exogenous hydrogen sulfide attenuates cerebral ischemia-reperfusion injury by inhibiting autophagy in mice. Can. J. Physiol. Pharmacol. 94, 1187–1192. doi: 10.1139/cjpp-2016-0100
Soares, R. O. S., Losada, D. M., Jordani, M. C., Evora, P., and Castro-E-Silva, O. (2019). Ischemia/reperfusion injury revisited: an overview of the latest pharmacological strategies. Int. J. Mol. Sci. 20:5034. doi: 10.3390/ijms20205034
Sun, Y., Huang, Y., Zhang, R., Chen, Q., Chen, J., and Zong, Y. (2015). Hydrogen sulfide upregulates K-ATP channel expression in vascular smooth muscle cells of spontaneously hypertensive rats. J. Mol. Med. 93, 439–455. doi: 10.1007/s00109-014-1227-1
Szabo, C. (2007). Hydrogen sulphide and its therapeutic potential. Nat. Rev. Drug Discov. 6, 917–935. doi: 10.1038/nrd2425
Tang, J., and Zhuang, S. (2019). Histone acetylation and DNA methylation in ischemia/reperfusion injury. Clin. Sci. 133, 597–609. doi: 10.1042/cs20180465
Wang, D., Ma, Y., Li, Z., Kang, K., Sun, X., and Pan, S. (2012). The role of AKT1 and autophagy in the protective effect of hydrogen sulphide against hepatic ischemia/reperfusion injury in mice. Autophagy 8, 954–962. doi: 10.4161/auto.19927
Wang, J., Wu, D., and Wang, H. (2019). Hydrogen sulfide plays an important protective role by influencing autophagy in diseases. Physiol. Res. 68, 335–345.
Wang, P., Liang, J., Li, Y., Li, J., Yang, X., and Zhang, X. (2014). Down-regulation of miRNA-30a Alleviates cerebral ischemic injury through enhancing Beclin 1-mediated autophagy. Neurochem. Res. 39, 1279–1291. doi: 10.1007/s11064-014-1310-6
Wang, Z. H., Zahedi, K., Barone, S., Tehrani, K., Rabb, H., Matlin, K., et al. (2004). Overexpression of SSAT in kidney cells recapitulates various phenotypic aspects of kidney ischemia-reperfusion injury. J. Am. Soc. Nephrol. 15, 1844–1852. doi: 10.1097/01.asn.0000131525.77636.d5
Wu, H., Chen, G., Wyburn, K. R., Yin, J., Bertolino, P., Eris, J. M., et al. (2007). TLR4 activation mediates kidney ischemia/reperfusion injury. J. Clin. Investig. 117, 2847–2859. doi: 10.1172/jci31008
Xie, H., Xu, Q., Jia, J., Ao, G., Sun, Y., and Hu, L. (2015). Hydrogen sulfide protects against myocardial ischemia and reperfusion injury by activating AMP-activated protein kinase to restore autophagic flux. Biochem. Biophys. Res. Commun. 458, 632–638. doi: 10.1016/j.bbrc.2015.02.017
Xu, Y., Qian, L., Zong, G., Ma, K., Zhu, X., and Zhang, H. (2012). class a scavenger receptor promotes cerebral ischemic injury by pivoting microglia/macrophage polarization. Neuroscience 218, 35–48. doi: 10.1016/j.neuroscience.2012.05.036
Yan, A., Zhang, T., Yang, X., Shao, J., Fu, N., Shen, F., et al. (2016). Thromboxane A2 receptor antagonist SQ29548 reduces ischemic stroke-induced microglia/macrophages activation and enrichment, and ameliorates brain injury. Sci. Rep. 6:35885.
Yang, G., Wu, L., Jiang, B., Yang, W., Qi, J., and Cao, K. (2008). H2S as a physiologic vasorelaxant: hypertension in mice with deletion of cystathionine gamma-lyase. Science 322, 587–590. doi: 10.1126/science.1162667
Yellon, D. M., and Hausenloy, D. J. (2007). Mechanisms of disease: Myocardial reperfusion injury. N. Engl. J. Med. 357, 1121–1135.
Yu, Q., Lu, Z., Tao, L., Yang, L., Guo, Y., Yang, Y., et al. (2015). ROS-dependent neuroprotective effects of NaHS in Ischemia brain injury involves the PARP/AIF pathway. Cell Physiol. Biochem. 36, 1539–1551. doi: 10.1159/000430317
Zheng, J., Zhao, T., Yuan, Y., Hu, N., and Tang, X. (2015). Hydrogen sulfide (H2S) attenuates uranium-induced acute nephrotoxicity through oxidative stress and inflammatory response via Nrf2-NF-kappa B pathways. Chem. Biol. Interact 242, 353–362. doi: 10.1016/j.cbi.2015.10.021
Keywords: hydrogen sulfide, autophagy, ischemia/reperfusion injury, apoptosis, oxidative stress
Citation: Lv S, Wang Z, Wang J and Wang H (2021) Exogenous Hydrogen Sulfide Plays an Important Role Through Regulating Autophagy in Ischemia/Reperfusion Injury. Front. Mol. Biosci. 8:681676. doi: 10.3389/fmolb.2021.681676
Received: 17 March 2021; Accepted: 19 April 2021;
Published: 13 May 2021.
Edited by:
Sandra Donnini, University of Siena, ItalyReviewed by:
Srinivasa Reddy Bonam, Institut National de la Santé et de la Recherche Médicale (INSERM), FranceClaudia Penna, University of Turin, Italy
Copyright © 2021 Lv, Wang, Wang and Wang. This is an open-access article distributed under the terms of the Creative Commons Attribution License (CC BY). The use, distribution or reproduction in other forums is permitted, provided the original author(s) and the copyright owner(s) are credited and that the original publication in this journal is cited, in accordance with accepted academic practice. No use, distribution or reproduction is permitted which does not comply with these terms.
*Correspondence: Honggang Wang, whg197167@vip.henu.edu.cn