- Izmir Institute of Technology, Molecular Biology and Genetics, Izmir, Turkey
Lysosomal serine carboxypeptidase Cathepsin A (CTSA) is a multifunctional enzyme with distinct protective and catalytic function. CTSA present in the lysosomal multienzyme complex to facilitate the correct lysosomal routing, stability and activation of with beta–galactosidase and alpha-neuraminidase. Beside CTSA has role in inactivation of bioactive peptides including bradykinin, substances P, oxytocin, angiotensin I and endothelin-I by cleavage of 1 or 2 amino acid(s) from C-terminal ends. In this study, we aimed to elucidate the regulatory role of CTSA on bioactive peptides in knock-in mice model of CTSAS190A. We investigated the level of bradykinin, substances P, oxytocin, angiotensin I and endothelin-I in the kidney, liver, lung, brain and serum from CTSAS190A mouse model at 3- and 6-months of age. Our results suggest CTSA selectively contributes to processing of bioactive peptides in different tissues from CTSAS190A mice compared to age matched WT mice.
Introduction
Lysosomal CathepsinA (CTSA), belonging to serine proteases family, is a multifunctional glycoprotein with three distinctive hydrolytic activities for deamidase, esterase and carboxypeptidase. It also makes a complex with the two glycosidases, β-galactosidase (β-gal) and α–neuraminidase (Neu1) to protect them against proteolytic degradation in lysosome (reviewed in Bonten et al., 2014). CTSA is necessary for oligomerization and catalytic activation of Neu1 (Itoh et al., 2000). Molecular defect of the CTSA cause autosomal recessively inherited rare lysosomal storage disease; galactosialidosis (D'azzo et al., 1982; Caciotti et al., 2013).
CTSA is widely distributed but differentially expressed in human tissues with the highest expression in the distal and collecting tubular cells of kidney, epithelial cells of lung, liver and large neurons of brain (Satake et al., 1994; Sohma et al., 1999). In mice, the highest level expression of CTSA is observed in kidney, brain, liver and placenta (Galjart et al., 1990). Secreted to the blood plasma by platelets and lymphocytes, CTSA very likely plays an extralysosomal (cell membrane) and/or extracellular regulatory role for a variety of bioactive peptide hydrolyzing them (Jackman et al., 1990).
CTSA is a member of α/β hydrolase fold family and has significant sequence homology in a conserved active side with two yeast enzymes, the KEX1 gene product and carboxypeptidase Y and wheat serine carboxypeptidase suggested the involvement of CTSA in different proteolytic functions in different cellular locations (Galjart et al., 1988; Hiraiwa, 1999). Several studies have shown that in vitro CTSA hydrolyze short regulatory bioactive short peptides including bradykinin, substances P, oxytocin, angiotensin I and endothelin-I (Jackman et al., 1992, 1993; Skidgel and Erdös, 1998). However, the physiological functions of CTSA outside the lysosome have not been explored fully. To elucidate this function, previously we have generated a knock-in mice model of CTSAS190A by replacing the nucleophile of the CTSA active site, Serine at 190 with Alanine. While the mutant CTSA protein retained its ability to form a complex with β-gal and Neu1, it has abolished enzymatic activity. CTSAS190A knock-in mice with inactive CTSA had a significantly induced level of bioactive short peptide endothelin-I resulting increased arterial blood pressure (Seyrantepe et al., 2008). In this study, we aimed to clarify significance of lysosomal CTSA in the regulation of several endogenous bioactive peptides including bradykinin, substances P, oxytocin, angiotensin I as well as endothelin-I in different tissues from CTSAS190A mice model at two different age groups.
Materials and Methods
Animals
CTSAS190A knock-in mice model were previously generated by Dr. Volkan Seyrantepe in Montreal, Canada and donated by Dr. Alexey V. Pshezhetsky (Centre Hospitaliere Universitaire Sainte-Justine, University of Montreal, Montreal, Quebec, Canada). CTSAS190A knock-in male mice was used and breed with C57/BL6 females for at least 10 generation to establish mice colony. All mice were bred and maintained in the Turkish Council on Animal Care (TCAC) accredited animal facility of Izmir Institute of Technology according to the TCAC guidelines. Mice were housed under constant room temperature and humidity on a 12 h light:dark cycle with ad libitum access to food and water. The animal care and the use in the experiments were granted by the Animal Care and Use Committee of Ege University, Izmir, Turkey.
Sample Preparation
3- and 6-months-old CTSAS190A and wild-type (WT) littermate male mice were analyzed (n = 3). Blood samples were collected in a centrifuge tube (stabilized with EDTA) after the cardiac puncture under anesthesia. Blood was centrifuged at 4°C; 1600 g for 15 min and serum was stored at −80°C. Kidney, liver, lung and brain were taken, snap freezed with liquid nitrogen and kept in −80°C up to 30 days.
Peptide Isolation
For extraction of peptides, 350 mg of kidney, 1 g of liver, 170 mg of lung and 360 mg of brain from CTSAS190A and WT male mice were homogenized in 5 ml/g cold lysis buffer (10 mM Tris, pH 7.4) using homogenizer (IKA T10 Basic Ultra-Turra-fold). After centrifugation of homogenate (1600 g for 15 min at 4°C), Aprotinin (RK-APRO) was added to all samples including serum at 1/10 (v/v) ratio. Peptides were purified with SEP-Pak C18 column (Phoenix Strata) from homogenized tissues and serum of mice. Eluted samples were first lyophilized (Labconco-Freezone Lyophilizer) and then pellet were re-suspended with ELISA 1-fold Assay Buffer.
ELISA Assays
Peptides from kidney, liver, lung, brain and serum were analyzed using Enzyme Immuno Assay kit (Phoenix Pharmaceutical—Bradykinin, Substances P, Oxytocin, Angiotensin I and Endothelin-I) according to manufacturer's instruction.
Statistical Analysis
Collected data correspond to means of 3 different animals with same sex age and genotype was analyzed using one-way ANOVA. Data were considered significant if P < 0.05.
Results
In the concept of this study, we determined the level of five different endogenous bioactive peptides (bradykinin, substances P, oxytocin, angiotensin I and endothelin-I) in kidney, liver, lung, brain and serum from CTSAS190A and WT male mice at 3- and 6-months-old in order to reveal the importance of lysosomal CTSA on the regulation of bioactive peptides in vivo.
Kidney
CTSAS190A male mice at the age of 3 months had elevated levels of bradykinin (2.2-fold), oxytocin (2.3-fold), angiotensin-I (1.2-fold) and endothelin-I (1.6-fold) compared to WT mice in kidney. However, no differences were detected in the levels of substances P in the same tissue from age matching CTSAS190A and WT male mice (Figure 1). In 6 month old CTSAS190A mice's kidney, the level of bradykinin, oxytocin and angiotensin-I were higher (1.3-fold, 1.2-fold and 1.5-fold, respectively) whereas substances P and endothelin-I levels were similar to age matching WT mice (Figure 1).
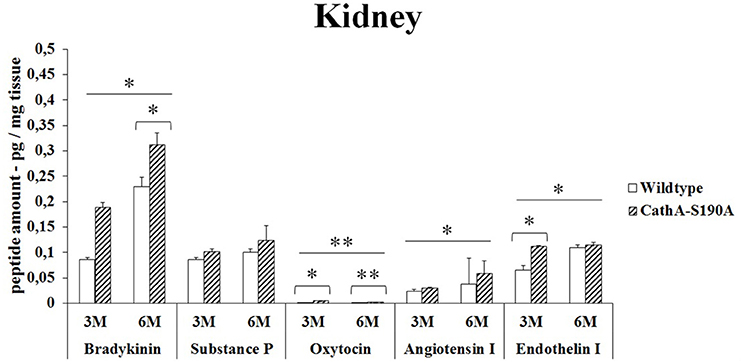
Figure 1. The levels of bradykinin, substance P, oxytocin, angiotensin I and endothelin-I in the kidney of 3-month-old (3M) and 6-month-old (6M) CathAS190A mice and WT littermate mice. Data show mean± SE of measurements in three male mice. Significant levels of Student's t-tests are shown below the boxplots. The symbol “ ____ ” indicates significant levels of bradykinin, substance P, angiotensin I and endothelin I between 3- and 6-month-old CathAS190A mice using the one-way ANOVA (*p < 0.05 and **p < 0.025).
Liver
3 months old CTSAS190A male mice showed significant increase in oxytocin level (5.8 -fold) in liver while other peptides were similar to WT. However, CTSAS190A male mice at the age of 6 month, had higher levels of bradykinin (2.9 -fold) and substances P (1.3-fold) in same tissue compared to WT (Figure 2). Lower oxytocin level was detected in older CTSAS190A however it was higher (1.4-fold) as compared to WT mice.
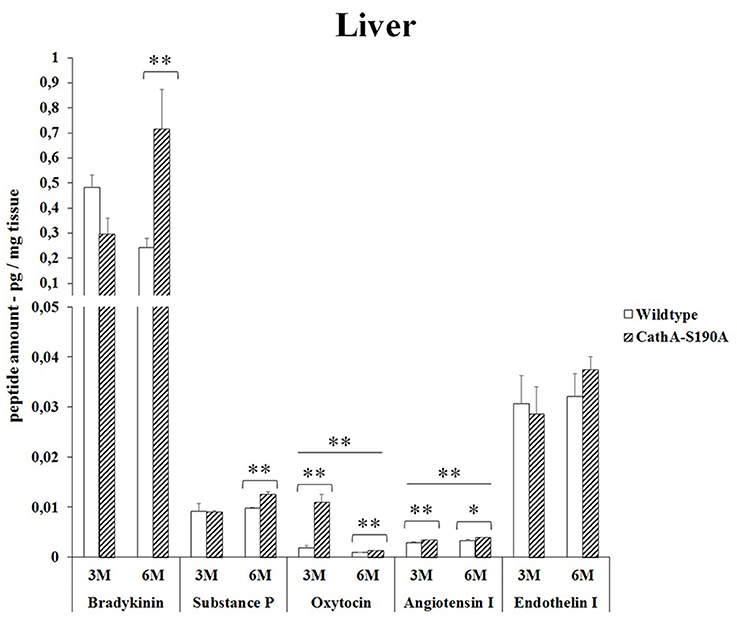
Figure 2. The levels of bradykinin, substance P, oxytocin, angiotensin I and endothelin-I in the liver of 3-month-old (3M) and 6-month-old (6M) CathAS190A mice and WT littermate mice. Data show mean ± SE of measurements in three male mice. Significant levels of Student's t-tests are shown below the boxplots. The symbol “ ____ ” indicates significant level of oxytocin and angiotensin I between 3- and 6-month-old CathAS190A mice using the one-way ANOVA (*p < 0.05 and **p < 0.025).
Lung
Bradykinin (1.5-fold), substances P (1.3-fold), oxytocin (1.6-fold) and endothelin-I (1.4-fold) levels were significantly higher in the lung tissue from 6 months old CTSAS190A male mice but not in younger mice. No difference was detected in the levels of Angiotensin I in the lung from 6 months old CTSAS190A and WT male mice. Only oxytocin level in lung tissue from 3 months old CTSAS190A male mice was higher (2.4-fold) compared to WT male mice (Figure 3).
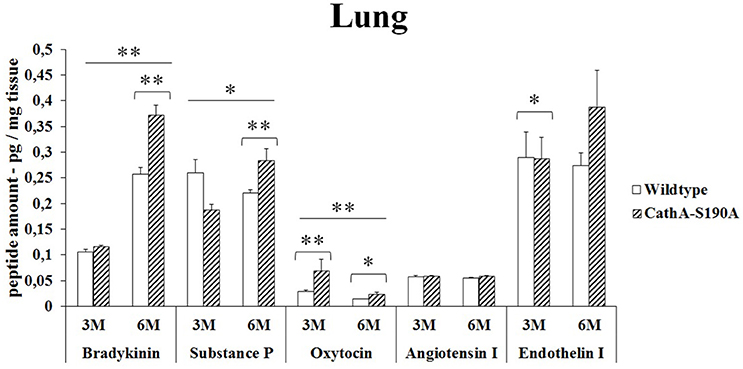
Figure 3. The levels of bradykinin, substance P, oxytocin, angiotensin I and endothelin-I in the lung of 3-month-old (3M) and 6-month-old (6M) CathAS190A and WT littermate mice. Data show mean ± SE of measurements in three male mice. Significant levels of Student's t-tests are shown below the boxplots. The symbol “ ____ ” indicates significant levels of bradykinin, substance P and oxytocin between 3- and 6-month-old CathAS190A mice using the one-way ANOVA (*p < 0.05 and **p < 0.025).
Brain
In the brain, the analysis of the bioactive peptides showed that oxytocin (14.2-fold) and substances P (5.2-fold) level was significantly higher in only 3 months old CTSAS190A mice compared to WT mice. However, no accumulations of other peptides were detected (Figure 4).
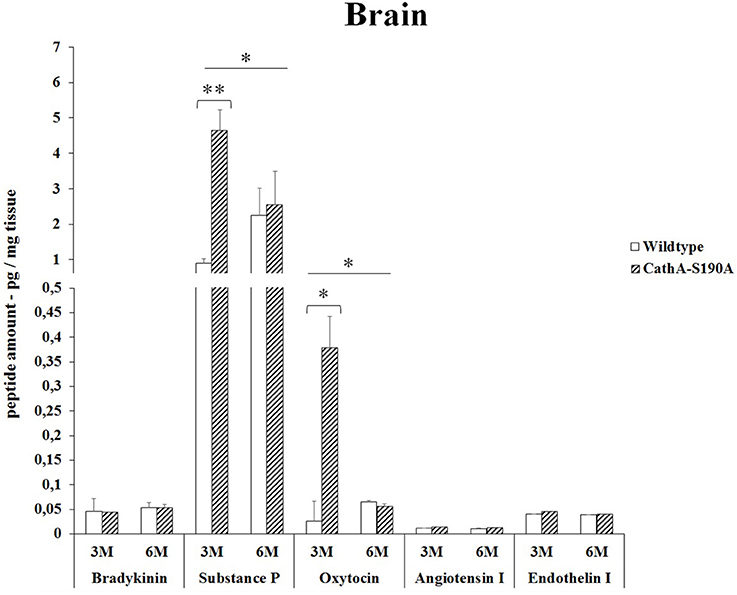
Figure 4. The levels of bradykinin, substance P, oxytocin, angiotensin I and endothelin-I in the brain of 3-month-old (3M) and 6-month-old (6M) CathAS190A and WT littermate mice. Data show mean ± SE of measurements in three male mice. Significant levels of Student's t-tests are shown below the boxplots. The symbol “ ____ ” indicates significant level of substance P and oxytocin between 3- and 6-month-old CathAS190A mice using the one-way ANOVA (*p < 0.05 and **p < 0.025).
Serum
We detected significantly higher level of bradykinin in serum from 3 months old (2.4-fold) as well as 6 months old (1.2-fold) CTSAS190A male mice compared to age-matched WT mice. The level of oxytocin in serum of CTSAS190A mice was higher (4.1-fold) in 3 months old but not in the older mice. On the other hand, the levels angiotensin-I were increased in both 3 and 6 months old CTSAS190A mice's serum as 1.3-fold and 1.6-fold, respectively (Figure 5).
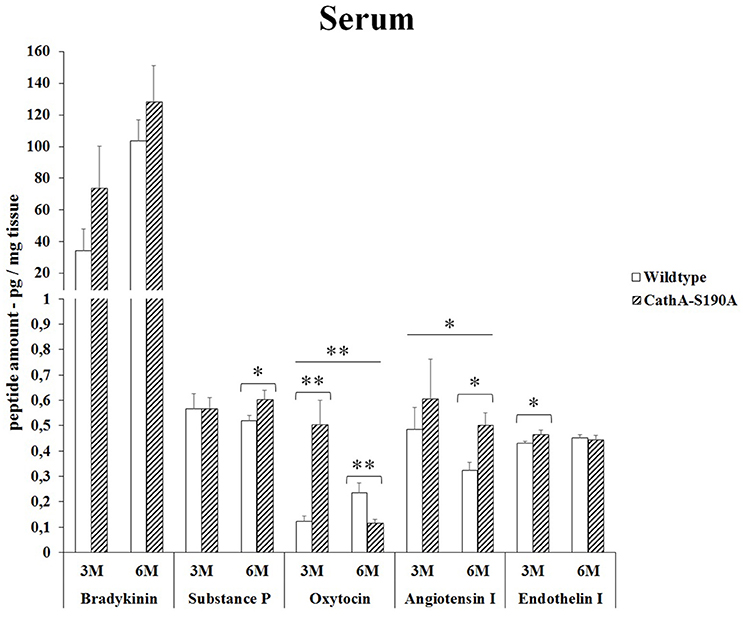
Figure 5. The levels of bradykinin, substance P, oxytocin, angiotensin I and endothelin-I in the serum of 3-month-old (3M) and 6-month-old (6M) CathAS190A and WT littermate mice. Data show mean ± SE of measurements in three male mice. Significant levels of Student's t-tests are shown below the boxplots. The symbol “ ____ ” indicates significant level of oxytocin and angiotensin I between 3-and 6-month-old CathAS190A mice using the one-way ANOVA (*p < 0.05 and **p < 0.025).
Discussion
CTSA is a lysosomal protein with dual function: protective and catalytic. CTSA associates with β-galactosidase and α–neuraminidase forming a multi-enzyme complex to protect these two enzymes against lysosomal degradation. Independent from its protective function, CTSA has carboxpeptidase activity at an acidic pH optimum between pH 4.5 and 5.5 as well as deamidase and esterase activities at neutral pH which leads the deamination of peptides (Hiraiwa, 1999).
The carboxyl-terminal end of many physiologically active peptides is blocked by an amide bond (−CONH2) and these form of the peptide are less susceptible to degradation. Several in vitro studies showed that CTSA is involved in activation or inactivation of these peptides by removing last one or two amino acid residues (Skidgel and Erdös, 1998). It has been shown that CTSA hydrolyzes a variety of bioactive peptides including substances P (Jackman et al., 1990). Report on the different percentage of relative carboxypeptidase activity against bioactive peptides such as endothelin I (100%), angiotensin I (9.5%), bradykinin (6.1%), oxytocin (4%), substances P (3.8%) suggests that CTSA may play a role in regulation of bioactive peptide functions (Hiraiwa, 1999).
Previously, it has been demostrated that expression level of CTSA enzyme has a tissue specific pattern in mice (Galjart et al., 1990). Although, kidney has the highest, relatively high levels of expression in brain, liver and lung implicates that CTSA may have different level of contribution to the hydrolysis of endogeneous bioactive peptides in different tissues. To elucidate the in vivo significance of lysosomal CTSA in the regulation of several endogenous bioactive peptides, we analyzed the levels of bradykinin, substances P, oxytocin, angiotensin I as well as endothelin-I in different tissues from knock-in CTSAS190A male mice at two different age groups.
Endothelin-I is a peptide composed of twenty-one amino acids. It is produced mainly in endothelial cells, vascular smooth muscle cells and a lesser extent by astrocytes, neurons, sertoli cells and hepatocytes. Endothelin-1 functions as vasoconstrictor and affects the salt-water equilibrium (Barnes and Turner, 1997). Endothelin-I is the well-known substrate for CTSA (Jackman et al., 1992). Previously we have reported that CTSAS190A mice have an increased level of endothelin-I only in lungs (Seyrantepe et al., 2008). Our current data showed that not only in lungs but also in kidney, there is increased level of endothelin-I in CTSAS190A mice at 6 and 3 months old respectively. Although, the markedly increased endothelin-1-specific immunoreactivity has been demonstrated in CTSA deficient galactosialidosis patient's brain, we did not observe any difference neither in brain nor in liver from CTSAS190A mice using ELISA (Itoh et al., 2000). We also speculate that higher expression of other carboxypeptidases such as Scpep1 may contribute modulating the catabolic proteolysis of Endothelin-I in these tissues (Pshezhetsky and Hinek, 2009; Pan et al., 2014).
Bradykinin is a physiologically and pharmacologically active peptide consists of nine amino acids known to influence the inflammatory process by affecting various tissue reactions including vasodilation, permeability of vessels and coagulation (Maurer et al., 2011). As a neuropeptide, bradykinin in brain chemotactically attracts glioma cells to blood vessels causing their migration and significantly changes the physiological properties of these cells in vivo (Montana and Sontheimer, 2011). We report for the first time that significantly higher levels of bradykinin in kidney (Figure 1), liver (Figure 2), lung (Figure 3) and serum (Figure 5) of the CTSAS190A mice compared to WT mice which indicates CTSA has important role on inactivation of endogenous bradykinin peptide in vivo. Interestingly, the comparisons of bradykinin levels in brain from 3 and 6 months old CTSAS190A and their WT siblings measured by ELISA were not conslusive. Therefore, we suggest that quantitation of bradykinin in brain using 2D-LC-MS/MS assays may be uniquely advantageous for elucidating physiological and biological events.
Substance P consists of eleven amino acids which is released from both the central and peripheral endings of neurons and functions as a neurotransmitter which is involved in vasodilation (reviewed in Harrison and Geppetti, 2001). Substances P plays role in inflammation through astrocyte and leukocyte activation at the site of injury (Lorente et al., 2015). A number of enzymes are required in the metabolism of substances P, including: neutral endopeptidase (NEP), angiotensin-converting enzyme (ACE), substance-P-degrading enzyme, cathepsin-D and cathepsin-E. In our study, among the studied tissues, a significant accumulation of substances P (5-fold) was observed in the brain of CTSAS190A mice (Figure 4). This accumulation in the absence of active CTSA shows that beside other enzymes CTSA is one of the functional enzymes that are involved in the metabolism of substances P, especially in mouse brain.
The role of CTSA in conversion of angiotensin-I to angiotensin-II suggests that CTSA may be an important player of the renin-angiotensin system regulating blood pressure and water balance (Miller et al., 1988). In the classic renin-angiotensin system enzymatic cascade angiotensinogen is cleaved by renin and converted to angiotensin I which is further processed by angiotensin converting enzyme (ACE) to form angiotensin II (Lavoie et al., 2004). Similar to ACE, CTSA is more likely to be involved in the in vivo conversion of angiotensin I into angiotensin II in the human heart (Jackman et al., 2002). In our results it was showed that elevated levels of angiotensin-I in kidney (Figure 1) and serum (Figure 5) but not in liver (Figure 2), lung (Figure 3) and brain (Figure 4) of CTSAS190A mice. Since Angiotensin-I is an intermediary peptide found most abundant as inactive form in blood (Lavoie et al., 2004), it is not surprising that we were able to detect the increased level of angiotensin I in blood as well as kidney where the cleavage of angiotensinogen takes place. Our results clearly implicate that CTSA is important member of renin-angiotensin system, however, more precise study including in situ analysis showing the expression level of CTSA and Angiotensin-I in particular tissues such as renal proximal tubules is required.
Oxytocin is a mammalian, 9-amino acid cyclic peptide which is synthesized in biologically active form in the paraventricular and supra-optic nuclei of the hypothalamus and is released into the central nervous system as well as the bloodstream (reviewed in Lee et al., 2009). In addition to brain, oxytocin and oxytocin receptors are found in several peripheral organs including heart, kidney, pancreas, breast, thymus, non-pregnant uterus, bowel and testis of the rat and human (Vargas-Martínez et al., 2014). Although, the highest densities of oxytocin receptors were observed in infant rat brain especially in cortex and spinal cord, low or undetectable numbers of oxytocin receptors were reported in the adult rat brain. It has been shown that aging decreases the number of oxytocin binding sites in the other part of brain (Arsenijevic et al., 1995). Besides its best known roles in parturition and lactation, oxytocin also plays important regulatory role in variety of physiological conditions such as social memory and attachment, sex and maternal behavior, and human bonding and trust (Gimpl and Fahrenholz, 2001; Lee et al., 2009). Oxytocin and oxytocin receptor expression is effected by steroid hormones and gender and it is usually higher in females (Lee et al., 2009). Therefore, in our research only male mice were investigated to detect the regulatory function of CTSA on cleavage off oxytocin peptide in vivo, kidney, liver, lung, brain and serum. Our results showed that the levels of oxytocin peptide were significantly higher in kidney (2.3-fold), liver (5.8-fold), lung (2.4-fold), brain (14.2-fold) and serum (4.1-fold) of 3 months old CTSAS190A mice compared to age matched WT mice respectively. Our data clearly suggests the role of CTSA on the cut off oxytocin in different tissues. The decrease in the oxytocin level in serum and other tissues of 6 months of WT and CTSAS190A mice as compared to that of 3 months may be related to the decreased level of oxytocin receptors in the aging brain.
Over all, our data clearly indicates that CTSA contributes on the hydrolysis of bioactive peptides in different mouse tissues including kidney, liver, lung, brain and serum. However, we expect that further analysis of aging mice (12 months old or older) which either can be feed with high-salt diet or normal diet may provide more insights regarding CTSA catalytic activity on bioactive peptides and its physiological role outside of lysosomes. Additionally, high-throughput and selective 2D-LC-MS/MS bioanalytical methods rather than sensitive radioimmunoassay (RIA) or ELISA can be applied to validate the physiological role of this unique enzyme in the regulation of bioactive peptides as well as determination of its unknown substrates.
Author Contributions
All authors listed, have made substantial, direct and intellectual contribution to the work, and approved it for publication.
Conflict of Interest Statement
The authors declare that the research was conducted in the absence of any commercial or financial relationships that could be construed as a potential conflict of interest.
Acknowledgments
This work was supported by TUBITAK (The Scientific and Technological Research Council of Turkey) grant 111T896.
References
Arsenijevic, Y., Dreifuss, J. J., Vallet, P., Marguerat, A., and Tribollet, E. (1995). Reduced binding of o-foldytocin in the rat brain during aging. Brain Res. 698, 275–279. doi: 10.1016/0006-8993(95)01020-V
Barnes, K., and Turner, A. J. (1997). The endothelin system and endothelin-converting enzyme in the brain: molecular and cellular studies. Neurochem Res. 1033–40.
Bonten, E. J., Annunziata, I., and d'Azzo, A. (2014). Lysosomal multienzyme complex: pros and cons of working together. Cell Mol. Life Sci. 71, 2017–2032. doi: 10.1007/s00018-013-1538-3
Caciotti, A., Catarzi, S., Tonin, R., Lugli, L., Perez, C. R., Michelakakis, H., et al. (2013). Galactosialidosis: review and analysis of CTSA gene mutations. Orphanet J. Rare Dis. 8:114. doi: 10.1186/1750-1172-8-114
D'azzo, A., Hoogeveen, A., Reuser, A. J., Robinson, D., and Galjart, H. (1982). Molecular defect in combined β-galactosidase and neuraminidase deficiency in man. Proc. Natl. Acad. Sci. U.S.A. 79, 4535–4539.
Galjart, N. J., Gillemans, N., Harris, A., van der Horst, G. T., Verheijen, F. W., Galjaard, H., et al. (1988). Expression of cDNA encoding the human “protective protein” associated with lysosomal beta-galactosidase and neuraminidase: homology to yeast proteases. Cell 54, 755–764.
Galjart, N. J., Gillemans, N., Meijer, D., and d'Azzo, A. (1990). Mouse “Protective Protein” cDNA cloning, sequence comparison, and expression. J. Biol. Chem. 265, 4678–4684.
Gimpl, G., and Fahrenholz, F. (2001). The oxytocin receptor system : structure, function, and regulation. Physiol. Rev. 81, 629–683.
Harrison, S., and Geppetti, P. (2001). Substance p. Int. J. Biochem. Cell Biol. 33, 555–576. doi: 10.1016/S1357-2725(01)00031-0
Hiraiwa, M. (1999). Cathepsin A/protective protein: an unusual lysosomal multifunctional protein. Cell Mol. Life Sci. 56, 894–907.
Itoh, K., Oyanagi, K., Takahashi, H., Sato, T., Hashizume, Y., Shimmoto, M., et al. (2000). Endothelin-1 in the brain of patients with galactosialidosis: its abnormal increase and distribution pattern. Ann. Neurol. 47, 122–126. doi: 10.1002/1531-8249(200001)47:1<122::AID-ANA21>3.0.CO;2-9
Jackman, H. J., Morris, P. W., Deddish, P. A., Skidgel, R. A., and Erdös, E. G. (1992). Inactivation of endotelin I by deamidase (lysosomal protective protein). J. Biol. Chem. 267, 2872–2875.
Jackman, H. J., Tan, F., Tamei, H., Beurling-Harbury, C., Li, X. Y., Skidgel, R. A., et al. (1990). A peptidase in human platelets that deamidates tachkinins: probable identity with the lysosomal protective protein. J. Biol. Chem. 265, 11265–11272.
Jackman, H. L., Massad, M. G., Sekosan, M., Tan, F., Brovkovych, V., Marcic, B. M., et al. (2002). Angiotensin 1–9 and 1–7 release in human heart role of cathepsin, A. Hypertension 39, 976–981. doi: 10.1161/01.HYP.0000017283.67962.02
Jackman, H. L., Morris, P. W., Rabito, S. F., Johansson, G. B., Skidgel, R. A., and Erdös, E. G. (1993). Inactivation of endothelin-1 by an enzyme of the vascular endothelial cells. Hypertension 21(6 Pt 2), 925–928.
Lavoie, J. L., Lake-Bruse, K. D., and Sigmund, C. D. (2004). Increased blood pressure in transgenic mice expressing both human renin and angiotensinogen in the renal pro-foldimal tubule. Am. J. Physiol. Renal. Physiol. 286, F965–F71. doi: 10.1152/ajprenal.00402.2003
Lee, H. J., Macbeth, A. H., Pagani, J., and Young, W. S. III. (2009). Oxytocin: the great facilitator of life. Prog. Neurobiol. 88, 127–151. doi: 10.1016/j.pneurobio.2009.04.001
Lorente, L., Martin, M. M., Almeida, T., Hernández, M., Ramos, L., Argueso, M., et al. (2015). Serum substance P levels are associated with severity and mortality in patients with severe traumatic brain injury. Crit. Care 19:192. doi: 10.1186/s13054-015-0911-z
Maurer, M., Bader, M., Bas, M., Bossi, F., Cicardi, M., Cugno, M., et al. (2011). New topics in bradykinin research. Allergy 66, 1397–406. doi: 10.1111/j.1398-9995.2011.02686.x
Miller, J. J., Changaris, D. G., and Levy, R. S. (1988). Conversion of angiotensin I to angiotensin II by cathepsin A isozymes of porcine kidney. Biochem. Biophys. Res. Commun. 154, 1122–1129. doi: 10.1016/0006-291X(88)90257-4
Montana, V., and Sontheimer, H. (2011). Bradykinin promotes the chemotactic invasion of primary brain tumors. J. Neurosci. 31, 4858–4867. doi: 10.1523/JNEUROSCI.3825-10.2011
Pan, X., Grigoryeva, L., Seyrantepe, V., Peng, J., Kollmann, K., Tremblay, J., et al. (2014). Serine carboxypeptidase SCPEP1 and Cathepsin A play complementary roles in regulation of vasoconstriction via inactivation of endothelin-1. PLoS Genet. 10:e1004146. doi: 10.1371/journal.pgen.1004146
Pshezhetsky, A. V., and Hinek, A. (2009). Serine carboxypeptidases in regulation of vasoconstriction and elastogenesis. Trends Cardiovasc Med. 19, 11–17. doi: 10.1016/j.tcm.2009.03.002
Satake, A., Itoh, K., Shimmoto, M., Saido, T. C., Sakuraba, H., and Suzuki, Y. (1994). Distribution of lysosomal protective protein in human tissues. Biochem. Biophys. Res. Commun. 205, 38–43.
Seyrantepe, V., Hinek, A., Peng, J., Fedjaev, M., Ernest, S., Kadota, Y., et al. (2008). Enzymatic activity of lysosomal carboxypeptitase (cathepsin) A is required for proper elastic fiber formation and inactivation of endotelin-1. Circulation 117, 1973–1981. doi: 10.1161/CIRCULATIONAHA.107.733212
Skidgel, R. A., and Erdös, E. G. (1998). Cellular carboxypeptidases. Immunol. Rev. 161, 129–141. doi: 10.1111/j.1600-065X.1998.tb01577.x
Sohma, O., Mizuguchi, M., Takashima, S., Satake, A., Itoh, K., Sakuraba, H., et al. (1999). Expression of protective protein in human tissue. Pediatr. Neurol. 20, 210–214.
Keywords: Cathepsin A, bioactive peptid, mouse, lysosome, regulation
Citation: Timur ZK, Akyildiz Demir S and Seyrantepe V (2016) Lysosomal Cathepsin A Plays a Significant Role in the Processing of Endogenous Bioactive Peptides. Front. Mol. Biosci. 3:68. doi: 10.3389/fmolb.2016.00068
Received: 29 July 2016; Accepted: 07 October 2016;
Published: 25 October 2016.
Edited by:
Saleh AlGhamdi, King Abdullah International Medical Research Center, Saudi ArabiaReviewed by:
Juan Ricardo Rodrigues, Central University of Venezuela, VenezuelaSuliman Abdallah Alsagaby, Majmaah University, Saudi Arabia
Alessandra D'Azzo, St. Jude Children's Research Hospital, USA
Copyright © 2016 Timur, Akyildiz Demir and Seyrantepe. This is an open-access article distributed under the terms of the Creative Commons Attribution License (CC BY). The use, distribution or reproduction in other forums is permitted, provided the original author(s) or licensor are credited and that the original publication in this journal is cited, in accordance with accepted academic practice. No use, distribution or reproduction is permitted which does not comply with these terms.
*Correspondence: Volkan Seyrantepe, volkanseyrantepe@iyte.edu.tr