- 1Laboratory of Clinical Biochemistry and Metabolism, Department for Pediatrics, Medical Center, University of Freiburg, Freiburg, Germany
- 2Department of Clinical Sciences, University of Bergen, Bergen, Norway
- 3Department of Clinical Biochemistry, Haukeland University Hospital, Bergen, Norway
- 4Department of Cellular and Molecular Medicine, Lerner Research Institute, Cleveland Clinic, Cleveland, OH, USA
Vitamin B12 (cobalamin, Cbl, B12) is an indispensable water-soluble micronutrient that serves as a coenzyme for cytosolic methionine synthase (MS) and mitochondrial methylmalonyl-CoA mutase (MCM). Deficiency of Cbl, whether nutritional or due to inborn errors of Cbl metabolism, inactivate MS and MCM leading to the accumulation of homocysteine (Hcy) and methylmalonic acid (MMA), respectively. In conjunction with total B12 and its bioactive protein-bound form, holo-transcobalamin (holo-TC), Hcy, and MMA are the preferred serum biomarkers utilized to determine B12 status. Clinically, vitamin B12 deficiency leads to neurological deterioration and megaloblastic anemia, and, if left untreated, to death. Subclinical vitamin B12 deficiency (usually defined as a total serum B12 of <200 pmol/L) presents asymptomatically or with rather subtle generic symptoms that oftentimes are mistakenly ascribed to unrelated disorders. Numerous studies have now established that serum vitamin B12 has limited diagnostic value as a stand-alone marker. Low serum levels of vitamin B12 not always represent deficiency, and likewise, severe functional deficiency of the micronutrient has been documented in the presence of normal and even high levels of serum vitamin B12. This review discusses the usefulness and limitations of current biomarkers of B12 status in newborn screening, infant and adult diagnostics, the algorithms utilized to diagnose B12 deficiency and unusual findings of vitamin B12 status in various human disorders.
Vitamin B12 Deficiency
Vitamin B12 (B12 = Cbl, “cobalamin,” the chemical name) is an essential water-soluble micronutrient required by all cells in the body. Humans are unable to synthesize B12 and thus rely on dietary intakes and a complex intracellular route for vitamin processing and delivery to its target destinations (Figure 1) (Hannibal et al., 2009). Vitamin B12 deficiency due to malabsorption and inadequate intake is a public health issue worldwide. It is estimated that 15–20% of the elderly in the United States are B12 deficient (Allen, 2009). In Germany, about 10% of the male elderly population and 26% of the female elderly population present with insufficient levels of vitamin B12 (Hartmann, 2008; Grober et al., 2013). In India, ~75% of the population, i.e., over 650 million people, have B12 deficiency (Antony, 2001; Refsum et al., 2001), which can only be partly ascribed to a vegetarian diet in a substantial portion of the population.
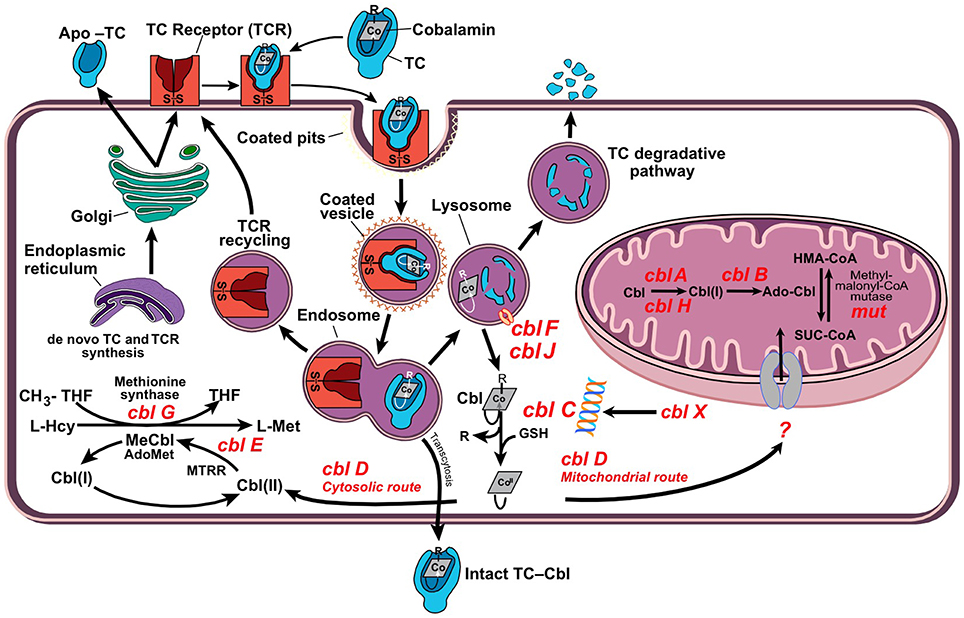
Figure 1. Cellular processing and trafficking of dietary vitamin B12. Cells take up holo-TC via TC-receptor-mediated endocytosis (TCR). In the lysosome, cobalamin (Cbl) is liberated and apo-transcobalamin is degraded, while the TCR is recycled back to the cell surface. Cbl exits the lysosome via transporters cblF/cblJ. In the cytosol, Cbl undergoes processing by enzyme cblC, which catalyzes removal of the upper-axial ligand (R), reduction of the cobalt center and conversion into the base-off configuration. The fate of newly processed B12 is dictated by cblC-cblD interactions, which direct the cofactor into either cytosolic methionine synthase (MS, cblG) or mitochondrial methylmalonyl-CoA mutase (MCM, mut). Nutritional and functional deficiencies of B12 lead to the inactivation of client enzymes MS and MCM with elevations of tHcy and MMA.
Vitamin B12 deficiency is a multifactorial condition caused by insufficient intake (nutritional deficiency) as well as acquired or inherited defects that disrupt B12 absorption, processing and trafficking pathways (functional deficiency). Methylcobalamin (MeCbl) serves as a coenzyme for the biosynthesis of methionine from homocysteine catalyzed by the cytosolic enzyme methionine synthase (MS). This reaction regenerates tetrahydrofolate (THF) from N5-methyl-tetrahydrofolate (N5-CH3-THF), which is essential for the de novo biosynthesis of nucleic acids. Adenosylcobalamin (AdoCbl) is required for the conversion of methylmalonyl-CoA to succinyl-CoA catalyzed by mitochondrial methylmalonyl-CoA synthase (MCM), an anaplerotic reaction that furnishes increased demands for the Krebs cycle and heme biosynthesis precursor succinyl-CoA.
Insufficient supply of B12 and genetic defects impairing its cellular processing and trafficking lead to the accumulation of homocysteine (Hcy) and methylmalonic acid (MMA), which enter circulation and give rise to hyperhomocystinemia and methylmalonic acidemia.
The recommended daily dose of B12 for adults is 2.4 μg per day (Institute of Medicine (US) Standing Committee on the Scientific Evaluation of Dietary Reference Intakes and its Panel on Folate, Other B Vitamins, and Choline, 1998), which is equivalent to the daily intake in the normal western diet. Malabsorption due to aging, poor nutrition and acquired defects in vitamin B12 metabolism are the leading causes of vitamin B12 deficiency worldwide. Inborn errors of vitamin B12 metabolism are rare.
Vitamin B12 deficiency is frequently under-diagnosed in pregnancy and in infants from mothers having insufficient levels of the micronutrient (Wheeler, 2008; Sarafoglou et al., 2011). Ensuring sufficient intake of vitamin B12 during pre-conception, pregnancy, and post-partum is strongly recommended (Bjørke Monsen et al., 2001; Rasmussen et al., 2001; Bjørke-Monsen et al., 2008; Hinton et al., 2010; Dayaldasani et al., 2014). Other populations at risk of developing vitamin B12 deficiency include the elderly, vegetarians and vegans, recipients of bariatric surgery (Majumder et al., 2013; Kwon et al., 2014) as well as those suffering from gastrointestinal diseases featuring ileal resections >20 cm (Battat et al., 2014). Certain medications such as metformin (Greibe et al., 2013b; Aroda et al., 2016) and proton-pump inhibitors (Howden, 2000; Wilhelm et al., 2013) may also transiently induce a status of cobalamin deficiency, which may be reversible upon completion of treatment and/or with oral vitamin B12 supplementation.
Herein, we discuss three aspects of the assessment of B12 status: (1) The utility of metabolites used as biomarkers of vitamin B12 deficiency in neonates and adults, (2) The algorithms employed to predict subclinical and clinical B12 deficiency, and (3) Major challenges and diagnosis of vitamin B12 deficiency in special populations.
Serum Biomarkers of Vitamin B12 Deficiency: Strengths and Limitations
Total Serum Vitamin B12
The most direct assessment and perhaps preferred first-assay to determine vitamin B12 status is the measurement of total serum vitamin B12. This assay is widely available in clinical chemistry laboratories. Ranges for normal (>250 pmol/L), low (150–249 pmol/L), and acute deficiency (<149 pmol/L) vitamin B12 have been defined and are used in most clinical chemistry laboratories worldwide (Clarke et al., 2003; Selhub et al., 2008; Mirkazemi et al., 2012). One limitation of this biomarker is that it assesses total circulating vitamin B12, of which ~80% is bound to haptocorrin, and therefore, not bioavailable for cellular uptake. Another limitation of this assay lies in its unreliability to reflect cellular vitamin B12 status. Results from studies assessing serum and cellular vitamin B12 have shown that the levels of serum B12 do not always represent cellular B12 status (Carmel, 2000; Solomon, 2005; Devalia et al., 2014; Lysne et al., 2016). In particular, patients with inborn errors of vitamin B12 metabolism can present with normal or low serum values of the vitamin, while being deficient at the cellular level. Furthermore, functional vitamin B12 deficiency due to oxidative stress has been identified in elders exhibiting normal values of serum vitamin B12 (Solomon, 2015). Functional deficiency of vitamin B12 was corrected upon supplementation with cyanocobalamin (CNCbl), as judged by reduction in the serum levels of tHcy and MMA (Solomon, 2015). Thus, total serum B12 is not a reliable biomarker of vitamin B12 status when used alone. Nonetheless, this marker should not be considered obsolete as a number of studies show that total serum vitamin B12 may be helpful to predict prognosis and status of diseases featuring abnormally high vitamin B12 levels in serum (>650 pmol/L), such as cancer (Arendt et al., 2016) and autoimmune lymphoproliferative syndrome (ALPS) (Bowen et al., 2012).
Homocysteine
Homocysteine is a metabolite of one-carbon metabolism that is remethylated by MeCbl-dependent MS or betaine-homocysteine methyltransferase as part of the methionine cycle (Finkelstein and Martin, 1984) and degraded by cystathionine β-synthase (CBS) in the transsulfuration pathway (Figure 2). Conversion of Hcy to Met by MS depends on the availability of both vitamin B12 and folate (as N5-CH3-THF), and therefore, nutritional deficiencies in either one of these micronutrients lead to the accumulation of Hcy in serum and urine. Likewise, inborn errors of metabolism that impair the upstream processing and trafficking of B12 or folate lead to elevation of this metabolite, a condition collectively known as hyperhomocystinemia. The normal range of total plasma Hcy (tHcy) in human plasma is 5–15 μmol/L (Ueland et al., 1993) and values >13 μmol/L may be considered elevated in adults (Jacques et al., 1999). Homocysteine levels are always higher in serum compared to plasma due to the release of Hcy bound to cellular components (Jacobsen et al., 1994). Hence, plasma and not serum should be used to determine the levels of tHcy. Although gender and age reference intervals have been established in some studies (Jacobsen et al., 1994; Rasmussen et al., 1996; van Beynum et al., 2005), they are usually ignored in the reporting of tHcy levels. Because of the dual biochemical origin of elevated Hcy, this biomarker is of limited value to assess vitamin B12 status as a stand-alone measurement. This is also true for the newborn screening of inborn errors of vitamin B12 metabolism found in the cblD, cblF, and cblJ (Huemer et al., 2015) disorders.
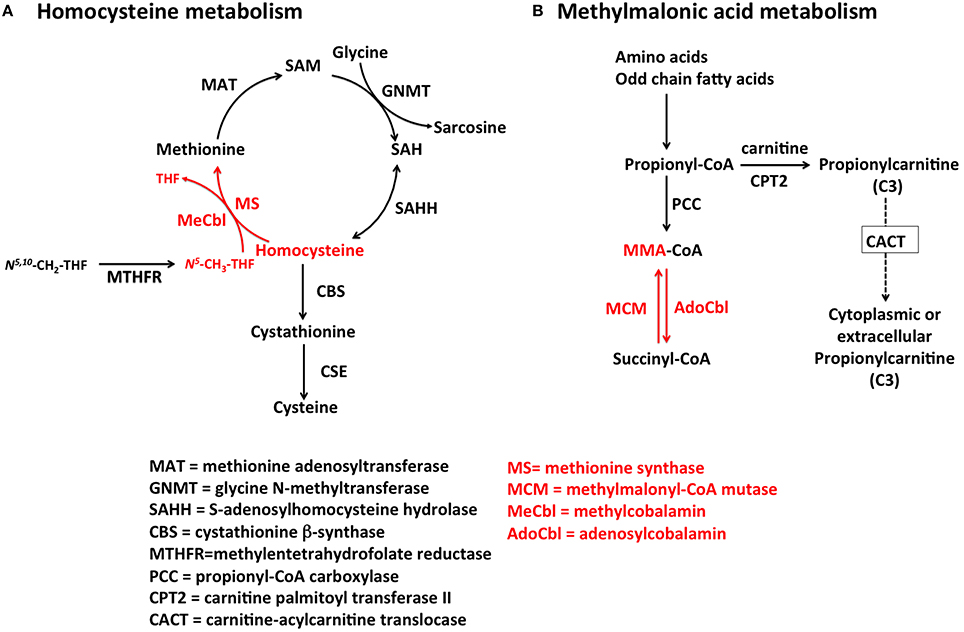
Figure 2. Pathways for Hcy and MMA metabolism in humans. (A) Homocysteine is a branch-point metabolite at the intersection of either the remethylation or the transsulfuration pathways. Thus, Hcy homeostasis relies on three different biochemical reactions [MS, cystathionine β-synthase (CBS) and S-adenosylhomocysteine hydrolase (SAHH)], two of which (CBS and SAHH) are independent of vitamin B12. In addition to nutritional deficiency of vitamin B12, elevation of Hcy in plasma may arise from reduced function of CBS and MTHFR, as well as nutritional deficiencies of folate. (B) MMA is produced during catabolism of odd-chain fatty acids and amino acids in the mitochondrion. Propionyl-CoA is the precursor of MMA in a reaction catalyzed by propionyl-CoA carboxylase (PCC). Inborn errors of PCC lead to propionic acidemia. Likewise, mutations in AdoCbl-dependent MCM lead to a buildup of MMA-CoA and inhibition of PCC that manifests as increased propionyl-CoA and so of propionic acid the circulation. Propionylcarnitine can also be transported out of the cell to reach systemic circulation. Propionylcarnitine is a first-line test in newborn screening.
Methylmalonic Acid
MMA increases upon inactivation of AdoCbl-dependent MCM in the mitochondrion. Nutritional and functional deficiencies of vitamin B12 result in the inactivation of MCM leading to buildup of its substrate methylmalonyl-CoA, which enters circulation as free MMA. The reaction catalyzed by MCM (Figure 2) is not affected by other vitamins of one-carbon metabolism, and therefore, MMA is considered a more specific marker of vitamin B12 deficiency (Clarke et al., 2003). Serum values of MMA, ranging from >260 to 350 nmol/L indicate elevation of this metabolite (Clarke et al., 2003). Nonetheless, there are few pathologies such as renal insufficiency that lead to an increase in MMA (Iqbal et al., 2013). For example, one study showed that 15–30% of individuals with high vitamin B12 concentrations in serum also had elevated MMA concentrations, which may reflect renal dysfunction instead of authentic vitamin B12 deficiency (Clarke et al., 2003). Thus, the utility of this marker should be considered carefully in older patients and patients with suspected or established renal disease. Assessment of a second marker of vitamin B12 status, such as holo-transcobalamin (holo-TC) (Iqbal et al., 2013) should be considered. Another study showed that the clearance of both Hcy and MMA may be compromised in patients with reduced kidney function (Lewerin et al., 2007).
Total Serum Holo-Transcobalamin
Dietary B12 is transported in the digestive system via the use of three protein transporters that bind the micronutrient in a sequential fashion, following the order haptocorrin (HC), intrinsic factor (IF), and transcobalamin (TC) (Fedosov et al., 2007; Fedosov, 2012). After absorption in the intestine, vitamin B12 bound to TC (holo-TC) reaches circulation and it is distributed to every cell in the body. Cells take up holo-TC via receptor-mediated endocytosis, aided by the transcobalamin receptor (TCblR; CD320) (Quadros et al., 2009). Because the only fraction of dietary vitamin B12 that is bioavailable for systemic distribution is in the form of holo-TC (Valente et al., 2011), the level of holo-TC in serum has been successfully utilized as a marker of bioactive vitamin B12 (Nexo et al., 2000, 2002; Valente et al., 2011; Yetley et al., 2011). Holo-TC represents 6–20% of the total vitamin B12 present in serum (Nexo et al., 2000, 2002; Valente et al., 2011; Yetley et al., 2011). This marker is more accurate in assessing the biologically active fraction of vitamin B12 in circulation than serum B12 itself, and its level correlates well with the concentration of vitamin B12 in erythrocytes (Valente et al., 2011). The diagnostic value of holo-TC has proven superior to Hcy and MMA for the assessment of vitamin B12 status in the elderly (Valente et al., 2011). The normal range of holo-TC in healthy subjects is 20–125 pmol/L (Valente et al., 2011). Additional research is needed to elucidate the mechanisms that control holo-TC homeostasis in the normal population and in pathologies that alter vitamin B12 transport and utilization. For example, abnormally low levels of holo-TC have been documented in patients receiving chemotherapy, macrocytosis and in individuals carrying the TC polymporphism 67A>G, without vitamin B12 deficiency (Vu et al., 1993; Wickramasinghe and Ratnayaka, 1996; Riedel et al., 2005, 2011). Insufficient sensitivity (44%) of holo-TC as a marker of vitamin B12 status was noted in a cohort of 218 institutionalized elderly patients (Palacios et al., 2013). At present time, it is unknown whether and how holo-TC levels vary in patients harboring inborn errors affecting intracellular vitamin B12 metabolism (cblA-cblJ). Thus, the diagnostic value of holo-TC as a first line test awaits further investigation.
Propionylcarnitine (C3) and its Ratios with Acetylcarnitine (C3/C2) and Palmitoylcarnitine (C3/C16)
In addition to the four canonical markers discussed above, vitamin B12 deficiency leads to accumulation of propionylcarnitine (C3) (Sarafoglou et al., 2011), which is a marker of methylmalonic aciduria and propionic acidemia. While vitamin B12 deficiency is not a primary test in newborn screening programs, a study showed that markers C3, C3/C2, and C3/C16 exhibit a negative correlation with maternal levels of vitamin B12 in the first trimester of pregnancy, and thus may bear diagnostic value (Dayaldasani et al., 2014). Although this finding requires further investigation with respect to the functional biomarkers Hcy and MMA, the finding opens the possibility of early identification of vitamin B12 insufficiency or deficiency during the first trimester of pregnancy and adequate treatment.
Algorithms for the Diagnosis of Vitamin B12 Deficiency
According to the World Health Organization (WHO), vitamin B12 status in adults is defined by the serum levels of the micronutrient with the following cut-offs and definitions: >221 pmol/L is vitamin “B12 adequacy”; between 148 and 221 pmol/L is “low B12,” and lower than 148 pmol/L is “B12 deficiency” (de Benoist, 2008; Allen, 2009). However, stand-alone markers of B12 status, such as serum B12, have proven insufficient for the unequivocal diagnosis of vitamin B12 deficiency (Fedosov, 2013; Palacios et al., 2013; Remacha et al., 2014; Fedosov et al., 2015). Further, the WHO criterion does not account for age effects. Algorithms that combine a minimum of two biomarkers have been employed worldwide, each exhibiting advantages and disadvantages (Palacios et al., 2013; Remacha et al., 2014).
A study performed on a Swedish population proposed that when physicians request testing for suspected vitamin B12 or folate deficiency, the first-line test of choice should be tHcy, and only when tHcy > 9 μM, should additional markers be tested to discriminate between vitamin B12 and folate deficiencies (Schedvin et al., 2005). This approach proved effective in reducing diagnostic costs by 30% (Schedvin et al., 2005).
Herrmann and Obeid proposed a two-step algorithm for the diagnosis of vitamin B12 in adults that utilizes holo-TC and MMA as biomarkers (Herrmann and Obeid, 2013). Analysis of 1359 samples submitted to the laboratory for total vitamin B12 assessment showed that patients exhibiting holo-TC values between 23 and 75 pmol/L and normal renal function should also be tested for MMA (Herrmann and Obeid, 2013). This guideline was widely recommended in Germany (Herrmann and Obeid, 2008) for the diagnosis of vitamin B12 deficiency in risk groups including infants, unexplained anemia, unexplained neuropsychiatric symptoms, gastrointestinal conditions including stomatitis, anorexia and diarrhea, the elderly, vegetarians and individuals with gastrointestinal diseases such as ilium resection, chronic atrophic gastritis, Crohn's disease and Helicobacter pylori infection and individuals under treatment with proton-pump inhibitors (Herrmann and Obeid, 2008; Hartmann et al., 2009; Koch, 2009; Heinzl, 2014). A cutoff of holo-TC of 50 pmol/L was set to discriminate between individuals unlikely to have vitamin B12 deficiency (holo-TC > 50 pmol/L) vs. those potentially deficient in the micronutrient (holo-TC < 50 pmol/L) (Herrmann and Obeid, 2008). Patients with potential vitamin B12 deficiency were first stratified by holo-TC levels as very low (holo-TC < 35 pmol/L) and low (holo-TC 36–50 pmol/L), and a second-line testing of MMA follows thereafter. Results from MMA lead to a three-block classification of patients, where (a) MMA < 271 nmol/L with holo-TC < 35 pmol/L represents a negative vitamin B12 balance (insufficiency), (b) MMA < 271 nmol/L with holo-TC 36–50 pmol/L suggests the patient is unlikely to be vitamin B12 deficient, and (c) a range of possible vitamin B12deficient patients is characterized by MMA > 271 nmol/L with holo-TC being very low or low (Herrmann and Obeid, 2008).
Berg and Shaw presented a cascade-testing algorithm that stratified patients first by total serum vitamin B12 levels and second by MMA levels, using cutoffs of 118 pmol/L and 0.80 μmol/L, respectively (Berg and Shaw, 2013). The authors encouraged the sequential measurement of these two biomarkers prior to the implementation of vitamin B12 therapy in patients.
Guidelines from the British Committee for Standards in Hematology suggested the use of total serum vitamin B12 as the first-line test, with MMA as the second-line test (Devalia et al., 2014). For the reasons presented in the sections above, this approach would exclude a significant fraction of patients for which serum vitamin B12 does not reflect cellular, genetic, and pharmacological disturbances that lead to functional vitamin B12 deficiency. It was also recommended that individuals classified as having “subclinical deficiency” be provided with empirical therapy with oral CNCbl 50 μg daily for 4 weeks, and have their serum B12 levels re-checked after 3 months (Devalia et al., 2014). Immediate medical attention was recommended for patients with symptoms of neuropathy.
Studies conducted by Palacios et al. on a cohort of 218 institutionalized elderly patients with median age 80 years old showed that an algorithm that combined biochemical, hematological, and morphological data proved more effective for the diagnosis of vitamin B12 than the isolated markers (Palacios et al., 2013). The proposed algorithm combines erythrocyte and serum folate, holo-TC, and MMA, and excludes serum vitamin B12 and tHcy measurements. The biomarkers selected in this algorithm permit the discrimination between isolated folate and vitamin B12 deficiencies as well as the combined deficiency of both vitamins (Palacios et al., 2013).
Fedosov and colleagues developed a series of equations that combine two, three, or four biomarkers of vitamin B12 status in adults. Age effects and folate status, a modifier of vitamin B12 metabolism, are also considered (Fedosov et al., 2015). The combined indicator of vitamin B12 status, cB12, is defined as: cB12 = log10((holo-TC.B12)/(MMA.tHcy))−(age factor) (Fedosov et al., 2015), and thus, it differs from the “if → then” structure of classic diagnostic algorithms. The combined indicator of vitamin B12 status provides five distinct ranges of diagnostic value, which are summarized in Table 1. This classification considers both extremes of vitamin B12 diagnostics, i.e., very low and very high vitamin B12 status. The pathophysiological implications of high vitamin B12 status are poorly understood, but its occurrence has been reviewed in the literature (Arendt and Nexo, 2013). The cB12 quotient has been successfully adopted to investigate the vitamin B12 status of a cohort of healthy Swiss elders (Risch et al., 2015). Results from this study suggest that increased levels of MMA and Hcy in seniors are brought about by a reduced renal function due to aging rather than by an underlying vitamin B12 insufficiency (Risch et al., 2015). Nonetheless, the study identified a clear trend of more prevalent metabolic vitamin B12 deficiency (measured as cB12) with increasing age (Risch et al., 2015). Another study showed that the cB12 indicator was useful not only to identify vitamin B12 deficiency, but also to find interactions with folate status (Brito et al., 2016). Further, the cB12 indicator was effective in identifying vitamin B12 in cancer patients who exhibit normal serum vitamin B12, but increased levels of MMA and Hcy (Vashi et al., 2016). In this study, the cB12 quotient helped to establish that MMA is the most sensitive marker of vitamin B12 in cancer patients, and that total serum B12 has very little diagnostic value (Vashi et al., 2016). None of the studies included a decrease in MMA or tHcy upon B12 administration as a potential marker in the evaluation of potential B12 deficiency.
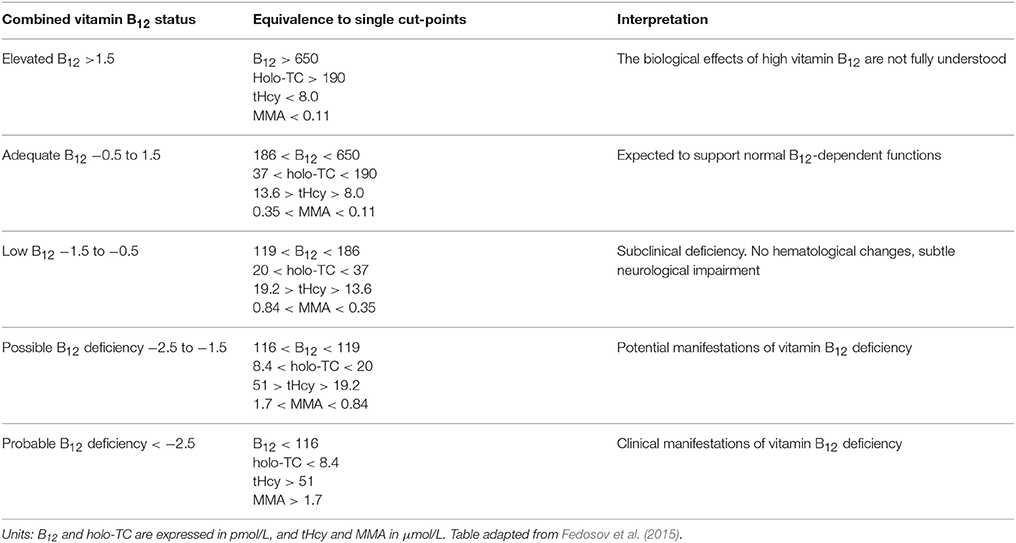
Table 1. Ranges of combined vitamin B12 status, their equivalence to single cut-off values, and clinical interpretation.
Vitamin B12 Deficiency in Infants
Infant cobalamin status at birth depends on maternal cobalamin stores during pregnancy, placental function, gestational age, and birth weight. There is a complex cobalamin metabolism in the placental-fetal compartment, strictly regulating the cobalamin transfer to the fetus (Porck et al., 1983; Miller et al., 1993; Perez-D'gregorio and Miller, 1998; Obeid et al., 2006). Cobalamin and holo-TC levels in the placenta, cord blood, and newborn serum correlate with maternal levels, but are 2–3 fold higher (Luhby et al., 1958; Baker et al., 1975; Giugliani et al., 1985; Frery et al., 1992; Obeid et al., 2006), providing the newborn with a liver store of about 25 μg cobalamin assumed to be sufficient for the first year of life (McPhee et al., 1988).
A wide range of cobalamin levels, varying from 86 to 939 pmol/L (median of 264 pmol/L) (Minet et al., 2000), have been reported for neonates during the first month of life. Maternal cobalamin deficiency, prematurity, and low birth weight are all associated with lower fetal cobalamin stores and an increased risk of deficiency, particularly if the infant is exclusively breastfed for more than 4 months before animal food is introduced (Dror and Allen, 2008; Torsvik et al., 2015). In mainly breastfed infants, the cobalamin level remains at cord blood levels for 5–7 days, then drops sharply, followed by a slow decline, reaching nadir levels at 6–7 months (Minet et al., 2000; Fokkema et al., 2002; Monsen et al., 2003). The decrease in serum cobalamin is accompanied by an increase in the metabolic markers plasma tHcy and MMA (Minet et al., 2000; Bjørke Monsen et al., 2001). This metabolic profile, indicative of cobalamin deficiency (Schneede et al., 1994), is associated with functional motor impairment in infants (Torsvik et al., 2013, 2015). Randomized intervention studies with one-time injection of 400 μg hydroxycobalamin are reported to improve the metabolic pattern and gross motor development compared with infants receiving placebo (Torsvik et al., 2013, 2015).
Reported cobalamin levels in human breast milk vary from 150 to 700 pmol/L (Specker et al., 1990a; Ford et al., 1996; Greibe et al., 2013a; Duggan et al., 2014), depending on the assay used (Black et al., 1994; Lildballe et al., 2009), maternal cobalamin levels and the stage of lactation, as cobalamin levels in breast milk falls progressively during the first months (Greibe et al., 2013a). The cobalamin content of breast milk appears to be lowest at 4 months after birth, and this coincides with a low cobalamin status in the infant (Greibe et al., 2013a). Gradually decreasing cobalamin status with increasing time of exclusively breastfeeding has also been reported in infants with a subnormal birthweight (2–3 kg) during the first 6 months of life (Torsvik et al., 2015). Most commercially prepared infant formulas are enriched with cobalamin up to concentrations of 800–1200 pmol/L, well above that of human milk (Ford et al., 1996), and higher cobalamin and lower tHcy and MMA levels are seen in formula-fed infants (Specker et al., 1990b; Minet et al., 2000; Fokkema et al., 2002; Hay et al., 2008).
After introduction of animal food, the cobalamin level increases and peaks at 3–7 years and then decreases, median plasma tHcy decreases and remains low until 7 years when it starts increasing, whereas median plasma MMA decreases after 12 months and remains low throughout childhood (Monsen et al., 2003). tHcy is considered the best metabolic marker of cobalamin status in infants and toddlers and a plasma tHcy cut-off level of 6.5 μmol/L has been suggested for defining cobalamin deficiency in this age-group (Bjørke-Monsen et al., 2008). This represents the 97.5 percentile in 4 months old infants given a single intramuscular dose of 400 μg hydroxocobalamin at 6 weeks, rendering them to be cobalamin optimized (Bjørke-Monsen et al., 2008). In addition, administration of folinic acid in newborns did not reduce tHcy (Hogeveen et al., 2010). In older children and adults, tHcy is mainly a folate marker, while MMA is considered a good marker for cobalamin status (Bjørke Monsen and Ueland, 2003; Monsen et al., 2003).
Challenges in the Diagnosis of Vitamin B12 Deficiency
Subclinical B12 Deficiency Is Asymptomatic
The diagnosis and management of subclinical vitamin B12 deficiency, which is defined as a total serum B12 concentration of 150–249 pmol/L(Carmel, 2012, 2013) is a matter of great interest, due to its much higher prevalence (up to 40% of the population in western countries) compared to clinical deficiency (Carmel, 2012, 2013). The etiology of subclinical vitamin B12 is unknown (Carmel, 2013). The condition manifests without overt clinical findings, with marginal or no elevation in tHcy and MMA and is typically of non-malabsorptive causes (Carmel, 2013). Subclinical B12 deficiency rarely evolves into clinical deficiency and the need for treatment with B12 has not been fully established in spite of its much higher frequency compared to clinical B12 deficiency (Carmel, 1996, 1998, 1999, 2011, 2013). A study with a population of asymptomatic B12-deficient elderly Chileans showed that patients displayed improved function of myelinated peripheral nerves after vitamin B12 treatment and a positive association with folate status (Brito et al., 2016).
Functional B12 Deficiency: Serum Markers vs. Cellular Status of Vitamin B12
A number of studies point to the lack of correlation between serum and cellular levels of vitamin B12 (Carmel, 2000; Solomon, 2005; Devalia et al., 2014; Lysne et al., 2016), and this is particularly important in the case of inborn errors of cobalamin metabolism whereby serum levels of the micronutrient are within the normal range(Watkins and Rosenblatt, 2013). In this regard, serum vitamin B12 and holo-TC should be avoided as sole markers of B12 deficiency in neonatal screening. Time-consuming metabolic studies to uncover genetic complementation or lack of function continue to be utilized in a very limited number of metabolic centers worldwide (Figure 3). In newborn screenings where biochemical markers are indicative of vitamin B12 deficiency, the diagnosis of inborn errors of vitamin B12 metabolism is performed via functional studies on cultured fibroblasts isolated from skin biopsies (Watkins and Rosenblatt, 2013) or via molecular genetic analysis of putative genes. Functional studies include [14C]-propionate or [14C]-N5−methyl-tetrahydrofolate incorporation into cellular macromolecules, to assess the activities of MCM and MS, respectively, and biosynthesis of MeCbl and AdoCbl upon metabolic labeling with holo-TC made from apo-TC and commercially available [57Co]-vitamin B12 (Watkins and Rosenblatt, 2013). Decreased uptake of [57Co]-vitamin B12 is indicative of impaired receptor-mediated endocytosis of holo-TC by the transcobalamin receptor. Accumulation of [57Co]-vitamin B12 in the lysosome suggests a dysfunctional cblF or cblJ protein unable to mediate exit of B12 from the organelle (Figure 1). Isolated or combined disruption of MeCbl and AdoCbl levels indicates failures in intracellular processing (cblC, cblX), trafficking (cblD), and/or coenzyme biosynthesis and utilization (cblA, cblB, cblG, cblE, mut). When results from [14C]-propionate or [14C]-N5-methyl-tetrahydrofolate incorporation suggest abnormal vitamin B12 metabolism, somatic cell complementation analysis usually follows (Watkins and Rosenblatt, 2013). Cells from patients are fused with cells from other patients with confirmed mutations using polyethylenglycol and the [14C]-propionate or [14C]-N5-methyl-tetrahydrofolate incorporation studies are repeated to interrogate complementation of function. If the incorporation is corrected with respect to control cell lines, then the two cell lines belong to different complementation groups. In contrast, lower than normal [14C]-propionate or [14C]-N5-methyl-tetrahydrofolate incorporation after fusion of cell lines with polyethylenglycol confirms that the two patients possess the same genetic defect (Watkins and Rosenblatt, 2013). Nowadays, the vast majority of genes whose mutation lead to functional vitamin B12 deficiency have been identified, and therefore, sequencing of these genes or next generation sequencing permits unequivocal diagnosis (Pupavac et al., 2016).
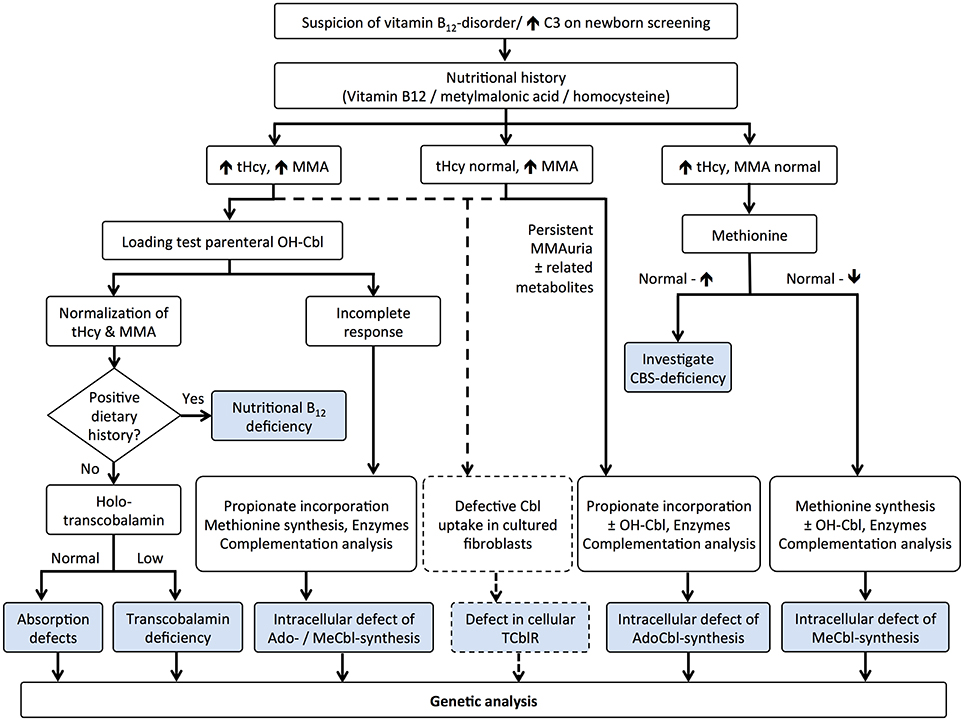
Figure 3. Algorithm for the diagnosis of vitamin B12 deficiency in neonates: metabolomic and functional studies. Elevated propionylcarnitine (C3) prompts the analysis of nutritional history with examination of vitamin B12 and its metabolites, tHcy, and MMA. Combined hyperhomocysteinemia and methylmalonic acidemia may be caused by insufficient B12 intake, defective absorption (intrinsic factor, transcobalamin) and genetic mutations affecting uptake (transcobalamin receptor, TCblR), lysosomal exiting (cblF and cblJ), processing (cblC), and trafficking (cblD). Total or incomplete response to a high dose of HOCbl helps to elucidate the cause of the dysfunction. Importantly, mutations in the TCblR gene may present with either the combined phenotype or with isolated methylmalonic acidemia, which should be considered using flow charts (dotted lines). Classical isolated hyperhomocysteinemia and methylmalonic acidemia are typically confirmed by functional assays, except for CBS deficiency that presents with normal to high methionine levels. Mutations affecting methionine synthase (cblG) and its partner reductase (cblE) lead to isolated hyperhomocysteinemia, whereas mutations affecting MCM (mut) and its associated proteins (cblA, cblB) result in isolated methylmalonic acidemia. Final confirmation of the disease is carried out by gene sequencing. This figure was modified from Baumgartner and Fowler (2014).
Combined B12 and Iron Deficiency
Iron deficiency is a condition that could lead to masking of megaloblastic anemia caused by vitamin B12 and folate deficiencies (Remacha et al., 2013). The combined deficiency of iron and vitamin B12 deficiency is more prominent in individuals aged 60 and older. In general, albeit not always, iron deficiency leads to microcytosis whereas vitamin B12 and folate deficiencies result in macrocytosis (Green, 2012). An algorithm to distinguish between these two large classes of anemia based on a decreased in RBC count, hematocrit and hemoglobin levels has been described (Green, 2012). In addition, an algorithm that introduces age and tHcy levels has been developed to discriminate iron deficiency anemia vs. iron and vitamin B12 combined anemia (Remacha et al., 2013).
Vitamin B12 Status in Special Populations
The Elderly
Despite an apparent sufficient intake, vitamin B12 deficiency is common among the elderly, and the prevalence is shown to increase with age (Allen, 2009; Miles et al., 2015). This is most likely not a physiological change due to aging per se, but a consequence of the elderly being more prone to gastric dysfunction, with variable degrees of gastric atrophy and achlorhydria interfering with the absorption of vitamin B12 from foods (Carmel, 1997). Most cases of low vitamin B12 among the elderly are accompanied by metabolic changes related to deficiency (Carmel, 1997). However, there is limited evidence from observational research linking this to clinical symptoms of deficiency (Miles et al., 2015). Because these metabolic changes usually respond to treatment with oral vitamin B12, low serum concentrations of vitamin B12 in the absence of clinical symptoms should be regarded as a state of subclinical deficiency. An increasing folate status due to folic acid fortification and/or folic acid supplementation has been demonstrated to accelerate and worsen both the metabolic and the clinical consequences of B12 deficiency (Morris et al., 2007; Selhub et al., 2007). Low vitamin B12 levels among the elderly should be considered grounds for concern in countries with mandatory folic acid fortification.
Bariatric Surgery and Gastrointestinal Disorders
The absorption of vitamin B12 is largely dependent on a healthy gastrointestinal tract, including (i) the production of intrinsic factor by parietal cells in the stomach, (ii) the dissociation of B12 from haptocorrin and binding to intrinsic factor in the neutral environment of the duodenum, and (iii) the uptake of vitamin B12 in the ileum. Pernicious anemia, an autoimmune disorder against gastric parietal cells, and bariatric surgery put the patient at risk of developing vitamin B12 deficiency by limiting intestinal absorption and/or by reduction of food intake. In addition to reducing intake of the vitamin itself, bariatric surgery may diminish the secretion of intrinsic factor in the stomach, which is normally produced and released in response to food intake (Marcuard et al., 1989). Finally, limited contact with the pancreatic juice due to a shorter common intestinal tract after surgery may hinder the release from haptocorrin, limiting the intestinal uptake. High prevalence of B12 deficiency has frequently been reported in subjects undergoing bariatric surgery (Sumner et al., 1996; Shah et al., 2006), and low levels of serum cobalamin is reported to be more indicative of true deficiency in bariatric surgery patients compared to healthy controls (Sumner et al., 1996). The malabsorptive procedures have been shown to yield higher risk of B12 deficiency compared to the restrictive procedures (Kwon et al., 2014). In studies where the patients received post-operative intramuscular supplementation of vitamin B12, however, no increased risk of becoming deficient is observed (Kwon et al., 2014). In a study of vitamin B12 status 5 years after surgery, patients who received intramuscular injections of vitamin B12 increased their serum concentration, while the concentration among those not receiving injections decreased (Aaseth et al., 2015). Patients who underwent bariatric surgery would benefit from life-long preventive and maintenance supplementation with vitamin B12. More broadly, gastrointestinal disorders that affect the small intestine (for example, ileal resection) could increase the risk of B12 deficiency through malabsorption (Battat et al., 2014). High prevalence of vitamin B12deficiency has been reported among untreated celiac disease patients (Theethira et al., 2014). Hence, patients newly diagnosed with celiac disease should be screened for B12 deficiency. Vitamin B12 deficiency is also frequently associated with chronic gastritis, which is most often a consequence of H. pylori infection (Varbanova et al., 2014).
Neurological Disorders
The consequences of vitamin B12 deficiency at the onset and progression of neurological impairments are well established (Kumar, 2014). In contrast, the causative role of vitamin B12 deficiency in neurological disorders such as Parkinson's, Alzheimer's, and others is controversial. A review of 43 studies worldwide showed that low serum vitamin B12 levels correlate with increased neurodegenerative disease and cognitive impairment (Moore et al., 2012). While a small subset of dementias examined in some of these studies responded favorably to vitamin B12 supplementation, no benefit was observed in patients with an established, pre-existing deficiency of vitamin B12 (Moore et al., 2012). One explanation may be that at least some of the neurological consequences of vitamin B12 deficiency are irreversible. Another possibility is that the relationship between B12 status and neurological disorders is not causal. A comprehensive analysis of studies performed from 2002 to 2012 examined tHcy levels with respect to dementia and cognitive decline (Health Quality Ontario, 2013). The results were controversial based on sample size and study design. Although the authors described an association between elevated tHcy and the onset of dementia, treatment with vitamin B12 did not improve cognitive function (Health Quality Ontario, 2013). On the other hand, a slower rate of brain atrophy was noted in patients with mild cognitive impairment who have received treatment with vitamin B12 (effective with both oral and parenteral administration) and folate (Health Quality Ontario, 2013). Elevated tHcy and MMA were identified in a small cohort of patients with Parkinson's disease, progressive supranuclear palsy and ammyotrophic lateral sclerosis compared to healthy subjects (Levin et al., 2010). In all cases, patients with these neurological diseases presented serum vitamin B12 and folate comparable to that of healthy controls(Levin et al., 2010), suggesting a functional vitamin deficiency. It is unclear whether increased tHcy and MMA contribute to the onset and progression of neurological disease and whether supplementation with cobalamin, in spite of normal serum levels of the micronutrient, may help to reduce these neurotoxic metabolites. It has also been suggested that treatment of Parkinson's and other neurological diseases with levodopa results in elevation of tHcy through S-adenosylmethionine-dependent methylation of levodopa by catechol O-methyl-transferase (Muller, 2009; Muller et al., 2013). The authors proposed that treatment of these diseases with a combination of levodopa and an inhibitor of O-methyl-transferase may prevent tHcy elevation and oxidative stress (Muller, 2009). Advanced-stage patients with Parkinson's disease typically receive continuous intraduodenal infusion of levodopa or carbidopa intestinal gel (Muller et al., 2013). These patients develop peripheral neuropathies thought to arise from transient deficiency of vitamins B6 and B12 as judged by a favorable response to concomitant supplementation with these micronutrients (Muller et al., 2013). A study performed with 1354 elder Australian subjects showed an association between low vitamin B12 levels and cognitive impairments that was enhanced in the presence of high folate intake (Moore et al., 2014). Furthermore, the study identified that subjects exhibiting normal serum vitamin B12 levels in the presence of high erythrocyte folate were also more likely to develop cognitive impairment (Moore et al., 2014). Unfortunately, this study did not determine metabolic markers of vitamin B12 deficiency such as MMA or tHcy. Altogether, available data suggests that preventing a deficiency of vitamin B12 may afford protection from neurological deterioration, and that some but not all cognitive impairments respond to therapy with vitamin B12. In terms of causation, the contribution of insufficient vitamin B12 metabolism to the onset and progression of unrelated disorders such as Parkinson's and Alzheimer's diseases and other dementias awaits the implementation of large population clinical trials.
Autoimmune Lymphoproliferative Syndrome
ALPS is a genetic disorder leading to defective lymphocyte apoptosis (Oliveira, 2013; Shah et al., 2014). This results in an accumulation of lymphocytes, splenomegaly, multilineage cytopenias, lymphadenopathy, hepatomegaly, and an increased risk of B-cell lymphoma. The disease is accompanied nearly always by autoimmune manifestations (Jackson et al., 1999; Oliveira et al., 2010). Most ALPS patients are affected by heterozygous germline mutations in the Fas cell surface death receptor gene (FAS) via autosomal dominant inheritance (Jackson et al., 1999; Holzelova et al., 2004; Oliveira et al., 2010). Somatic mutations in FAS have also been described albeit less frequently (Jackson et al., 1999; Holzelova et al., 2004; Oliveira et al., 2010). FAS is a member of the tumor necrosis factor receptor family (FAS/CD95/APO-1/TNFRSF6) and it is highly expressed in activated B and T cells (Nagata and Golstein, 1995). FAS-FAS ligand recognition in activated T lymphocytes leads to the activation of the caspase cascade and cellular apoptosis (Nagata and Golstein, 1995). This apoptotic pathway is important for the down-regulation of the immune response, and its disturbance leads to buildup of proliferating lymphocytes resulting in lymphoid hyperplasia and autoimmunity (Rieux-Laucat et al., 2003). Revised diagnostic criteria and classification for the ALPS syndrome were established in 2009 at a US National Institutes of Health International Workshop. Required criteria for ALPS included the presence of lymphadenopathy and/or splenomegaly and elevated TCRαß+-DNT cells. Accessory criteria for ALPS were subdivided into primary (abnormal lymphocyte apoptosis assay and presence of pathogenic mutations in genes of the FAS pathway) and secondary criteria, which included characteristic histopathology, the combined presence of autoimmune cytopenia, polyclonal hypergamma-globulinemia, an ALPS-compatible family history, and the presence of elevated biomarkers, such as interleukin-10, interleukin-18, soluble FAS ligand, and vitamin B12 (Oliveira et al., 2010). These biomarkers are included in the diagnostic criteria because they can predict both germline and somatic FAS mutations with a post-test probability from 85 to 97% (87% for vitamin B12) and because they are accessible to facilities without the ability to do genetic analysis or functional assays (Teachey et al., 2005; Magerus-Chatinet et al., 2009; Caminha et al., 2010; Seif et al., 2010). Very highly elevated median serum vitamin B12 in ALPS Ia and Ia-s (1667 pmol/L; 1220 pmol/L) compared with mutation-negative relatives (350 pmol/L; P < 0.0001) and healthy mutation-positive relatives (421 pmol/L; P < 0.0001) have been reported (Caminha et al., 2010). In particular, serum vitamin B12 median levels in ALPS III and ALPS-Phenotype were substantially elevated (560 and 696 pmol/L) (Caminha et al., 2010). Another group observed significantly higher mean serum vitamin B12 concentrations (3900 pmol/L; controls: 274 pmol/L; p < 0.0001) and significantly higher mean holo-haptocorrin (holo-HC) concentrations (3810 pmol/L; controls: 194 pmol/L; p < 0.0001) in the presence of normal holo-TC concentrations (Bowen et al., 2012). The abnormally high levels of circulating vitamin B12 found in ALPS patients has been ascribed to high expression of HC by lymphocytes isolated from ALPS patients, a characteristic not observed in lymphocytes isolated from healthy control subjects (Bowen et al., 2012). Thus, it would appear that HC expression is upregulated in ALPS. In the Bowen et al. study, the metabolic markers of vitamin B12 status (tHcy and MMA) in ALPS patients were investigated and no differences with respect to healthy controls were found (Bowen et al., 2012). This is consistent with the fact that high serum holo-HC does not impair holo-TC uptake by the cellular TCblR (Quadros et al., 2009, 2010; Jiang et al., 2013; Quadros and Sequeira, 2013) and the downstream intracellular utilization of the micronutrient (Hannibal et al., 2009, 2013). It remains to be determined whether excess serum vitamin B12 found in ALPS patients derives from clearance of liver storages or simply by increased dietary assimilation.
Vegetarians and Vegans
Although a properly planned vegetarian diet is regarded as nutritionally adequate and healthy, unsupplemented populations who actively avoid food of animal origin are at high risk of insufficient dietary intake of vitamin B12. The amount of B12 in vegetarian diets depends on the extent of inclusion of animal products or the use of dietary supplements. Supplementation is recommended to those completely avoiding animal products (strict vegans). Compared to meat eaters, both vegetarians and particularly vegans have a lower intake of vitamin B12 (Davey et al., 2003; Rizzo et al., 2013; Kristensen et al., 2015; Schupbach et al., 2015). This is also reflected by lower serum concentrations (Gilsing et al., 2010), and vitamin B12 deficiency is shown to be common following all types of vegetarian diets (Pawlak et al., 2013, 2014). However, in a study comparing micronutrient status in omnivores, vegetarians and vegans from Switzerland, a low vitamin B12 intake among vegans was not accompanied by low serum concentrations of the micronutrient (Schupbach et al., 2015). This was attributed to widespread use of B12 supplements, which adheres to dietary recommendations for this subpopulation (Pawlak et al., 2014). Infants of vegan mothers not taking supplements are also at increased risk of becoming vitamin B12 deficient, especially so when exclusively breastfed (Dror and Allen, 2008). This is due both to small hepatic stores and low intake through the breast milk, both a result of poor maternal vitamin B12 status. In light of these findings, it is advised that vegetarians and vegans monitor their serum vitamin B12 levels and at least one metabolic marker (tHcy or MMA), or consider taking a B12 supplement as part of their dietary plan.
Medication Affecting Vitamin B12 Status
Besides factors influencing the intake and absorption of vitamin B12, some medications have been shown to interfere with vitamin B12 absorption or metabolism, potentially putting certain patient groups at risk of developing vitamin B12deficiency. Metformin (Glucophage) is regarded a first-line drug in the treatment of type 2 diabetes mellitus. However, it has been shown that treatment with metformin is associated with a decrease in serum vitamin B12 concentrations, which worsens with increasing dose and length of treatment (Ting et al., 2006; de Jager et al., 2010; Liu et al., 2014). The association has been suggested to be due to interference with the calcium dependent intestinal uptake of the vitamin B12-intrinsic factor complex (Bauman et al., 2000). However, although serum B12 is reduced, metformin treatment has been associated with reduction in plasma MMA and indications of improved intracellular B12 status in diabetics (Obeid et al., 2013). Hence, a vitamin B12 resistance phenomenon was suggested in diabetics, and metformin treatment seemed to improve the intracellular vitamin B12 metabolism, contrary to previous beliefs. Two studies found that patients treated with metformin had reduced levels of both serum vitamin B12 and haptocorrin, but treatment was without effect on the levels of holo-TC, the bioactive form of vitamin B12 that is distributed systemically to all cells in the body (Leung et al., 2010; Greibe et al., 2013c). Another study showed that treatment of rats with metformin increased hepatic accumulation of vitamin B12 (Greibe et al., 2013b). This suggests that metformin may alter the homeostasis and tissue distribution of vitamin B12, the consequences of which remain to be investigated. Because not all cases of low serum vitamin B12 imply deficiency, and normal and high serum levels of vitamin B12 are likewise observed in patients with severe functional vitamin B12 deficiency, concern has been raised regarding the interpretation of the effects of metformin on vitamin B12 status (Obeid, 2014). In terms of prevention, diabetics receiving metformin should be regarded at increased risk, and routine screening and supplementation if necessary, should be recommended (Valdes-Ramos et al., 2015).
Proton pump inhibitors or other medications that suppress acid production in the stomach are frequently associated with reduced serum vitamin B12 concentrations (Abraham, 2012). This is due to the acidic environment in the stomach being essential for the release of protein-bound vitamin B12, as well as initial binding to haptocorrin (Stabler, 2012). While serum vitamin B12 is reduced, it usually stays within the normal range (Sheen and Triadafilopoulos, 2011). Hence, the risk of developing overt deficiency due to use of proton pump inhibitors may be low, but it has been suggested that the risk is higher among elderly and malnourished patients receiving long-term treatment (Sheen and Triadafilopoulos, 2011).
Nitrous oxide, an inhalant used for surgical and dental anesthesia and also as a recreational drug (van Amsterdam et al., 2015), directly affects vitamin B12 metabolism by irreversibly oxidizing the cobalt atom in B12-dependent MS (Guttormsen et al., 1994; Torri, 2010). When subjects with borderline vitamin B12 deficiency are treated with nitrous oxide, rapid onset of neurological symptoms has been reported post treatment (Singer et al., 2008). Patients who are candidates for interventions with nitrous oxide anesthesia may benefit from pre-operatory supplementation with vitamin B12.
Genetic Determinants of Vitamin B12 Status
Aside from inborn errors of metabolism affecting the intracellular pathways of vitamin B12 trafficking and assimilation (for excellent reviews on the topic see Froese and Gravel, 2010; Watkins and Rosenblatt, 2011), mutations in the transcobalamin gene (TCN2) (Keller et al., 2016), high levels of the soluble transcobalamin receptor (sCD320) Hoffmann-Lucke et al., 2013, and polymorphisms in 3-hydroxybutyryl-CoA hydrolase (HIBCH) (Molloy et al., 2016) also affect the serum markers of vitamin B12 status. Mutations in the TCN2 gene can lead to false low levels of circulating holo-TC without effect on other biomarkers of vitamin B12 status (Keller et al., 2016). Increased levels of a heavier variant of the sCD320 led to elevated holo-TC and vitamin B12 in serum, though the reasons for such variations are currently unknown (Hoffmann-Lucke et al., 2013). The larger apparent molecular weight of sCD320 could be due to altered glycation or formation of higher order oligomers of the sCD320 (Hoffmann-Lucke et al., 2013). A genome-wide analysis in 2210 healthy Irish adults identified strong associations between plasma MMA with SNPs in acylCo-A synthetase (ACSF3) and HIBCH, with these loci accounting for 12% of the variance in MMA concentration (Molloy et al., 2016). HIBCH catalyzes a unique step in the degradation of valine, converting 3-hydroxyisobutyric acid-CoA to 3-hydroxyisobutyric acid. The presence of polymorphisms in HIBCH represents an independent determinant of serum MMA concentrations. The molecular mechanism by which polymorphic variants of HIBCH lead to elevated MMA remain to be investigated (Molloy et al., 2016). Transient elevation of MMA has also been documented in patients with mutations in methylmalonate semialdehyde dehydrogenase (Marcadier et al., 2013), and in ACSF3, which possesses malonyl-CoA and methylmalonyl-CoA activity (Alfares et al., 2011; Sloan et al., 2011). Mutations in mitochondrial succinate-CoA ligase (SUCLG1) also lead to elevated MMA (Valayannopoulos et al., 2010).
Mutations that impair the endocytic and lysosomal pathways independently of vitamin B12 metabolism also lead to transient deficiency of the micronutrient, with elevation of tHcy and MMA (Stockler et al., 2014; Dutchak et al., 2015; Zhao et al., 2015). These include abnormal lysosome acidification in a patient with Alzheimer's disease (Zhao et al., 2015), impaired endocytosis due to a mutation in the rabenosyn-5 gene (Stockler et al., 2014; Zhao et al., 2015), and disrupted lysosomal acidification and gene expression in a knock-out mouse model of mTOR inhibitor NPRL2 (Dutchak et al., 2015). This pathway is thought to cause a defective processing of holo-TC in the lysosome (Dutchak et al., 2015).
Upregulation of the expression of hepatic peroxisome proliferator activated receptor alpha (PPAR alpha), has been shown to elevate plasma MMA in male Wistar rats fed a normal high fat diet in the presence of PPAR alpha agonist tetradecylthioacetic acid (TTA) (Lysne et al., 2016). Because the amount of receptor is a limiting factor for PPAR alpha activity, and the amount of PPAR alpha protein strongly correlates with its level of mRNA expression (Lemberger et al., 1996), differential expression of PPAR alpha induced by diet, stress and other factors may represent another vitamin B12-independent determinant of plasma MMA.
Overall, the finding that mutations and polymorphisms in genes peripheral or unrelated to intracellular vitamin B12 pathways affect its marker metabolites, substantiates the need for the combined analysis of at least two biomarkers for the accurate assessment of vitamin B12 status.
Concluding Remarks
The diagnosis of vitamin B12 deficiency both in children and adults requires the use of at least two biomarkers. Among the biomarkers currently available for diagnostic purposes, total serum vitamin B12 is equivocal for the identification of functional deficiencies, such as those caused by inborn errors of metabolism. The use of holo-TC to determine vitamin B12 status is advantageous in that it reflects the biologically active pool of vitamin B12 in serum, but its homeostasis is poorly understood. This is illustrated by the abnormal levels of holo-TC observed under various unrelated disease states and in individuals possessing otherwise normal total serum vitamin B12. tHcy is particularly useful as a marker of vitamin B12 status in the neonate, but its reliability and specificity are equivocal in adulthood because it is influenced by many factors such as folate status. Methylmalonic acid continues to be the most sensitive and specific marker for vitamin B12 status in individuals of all ages with normal renal function. The use of acylcarnitines can be considered ancillary to diagnosis, but certainly not a first-line test. One major challenge lies in identifying early markers of low vitamin B12 status for the timely diagnosis of the clinically and biochemically silent subclinical vitamin B12 deficiency. Likewise, the consequences of insufficient vitamin B12 levels in special populations such as the elderly, vegetarians and vegans, infants, and pregnant women suffer from poor awareness by both patients and clinicians. The finding that low vitamin B12 status is associated with more prominent metabolic markers of vitamin B12 deficiency in the presence of high folic acid concentrations (Miller et al., 2009) points to the importance of nutrient-nutrient interactions, which have only been considered over the past decade and is of particular relevance to countries with ongoing folic acid fortification of foods.
Author Contributions
All authors listed, have made substantial, direct and intellectual contribution to the work, and approved it for publication.
Conflict of Interest Statement
The authors declare that the research was conducted in the absence of any commercial or financial relationships that could be construed as a potential conflict of interest.
References
Aaseth, E., Fagerland, M. W., Aas, A. M., Hewitt, S., Risstad, H., Kristinsson, J., et al. (2015). Vitamin concentrations 5 years after gastric bypass. Eur. J. Clin. Nutr. 69, 1249–1255. doi: 10.1038/ejcn.2015.82
Abraham, N. S. (2012). Proton pump inhibitors: potential adverse effects. Curr. Opin. Gastroenterol. 28, 615–620. doi: 10.1097/MOG.0b013e328358d5b9
Alfares, A., Nunez, L. D., Al-Thihli, K., Mitchell, J., Melancon, S., Anastasio, N., et al. (2011). Combined malonic and methylmalonic aciduria: exome sequencing reveals mutations in the ACSF3 gene in patients with a non-classic phenotype. J. Med. Genet. 48, 602–605. doi: 10.1136/jmedgenet-2011-100230
Allen, L. H. (2009). How common is vitamin B-12 deficiency? Am. J. Clin. Nutr. 89, 693S–696S. doi: 10.3945/ajcn.2008.26947A
Antony, A. C. (2001). Prevalence of cobalamin (vitamin B-12) and folate deficiency in India–audi alteram partem. Am. J. Clin. Nutr. 74, 157–159.
Arendt, J. F., and Nexo, E. (2013). Unexpected high plasma cobalamin: proposal for a diagnostic strategy. Clin. Chem. Lab. Med. 51, 489–496. doi: 10.1515/cclm-2012-0545
Arendt, J. F., Farkas, D. K., Pedersen, L., Nexo, E., and Sorensen, H. T. (2016). Elevated plasma vitamin B12 levels and cancer prognosis: A population-based cohort study. Cancer Epidemiol. 40, 158–165. doi: 10.1016/j.canep.2015.12.007
Aroda, V. R., Edelstein, S. L., Goldberg, R. B., Knowler, W. C., Marcovina, S. M., Orchard, T. J., et al. (2016). Long-term metformin use and Vitamin B12 deficiency in the diabetes prevention program outcomes study. J. Clin. Endocrinol. Metab. 101, 1754–1761. doi: 10.1210/jc.2015-3754
Baker, H., Frank, O., Thomson, A. D., Langer, A., Munves, E. D., De Angelis, B., et al. (1975). Vitamin profile of 174 mothers and newborns at parturition. Am. J. Clin. Nutr. 28, 59–65.
Battat, R., Kopylov, U., Szilagyi, A., Saxena, A., Rosenblatt, D. S., Warner, M., et al. (2014). Vitamin B12 deficiency in inflammatory bowel disease: prevalence, risk factors, evaluation, and management. Inflamm. Bowel Dis. 20, 1120–1128. doi: 10.1097/mib.0000000000000024
Bauman, W. A., Shaw, S., Jayatilleke, E., Spungen, A. M., and Herbert, V. (2000). Increased intake of calcium reverses vitamin B12 malabsorption induced by metformin. Diabetes Care 23, 1227–1231. doi: 10.2337/diacare.23.9.1227
Baumgartner, M. R., and Fowler, B. (2014). Physician's Guide to the Diagnosis, Treatment, and Follow-Up of Inherited Metabolic Diseases. Heidelberg: Springer-Verlag Berlin Heidelberg.
Berg, R. L., and Shaw, G. R. (2013). Laboratory evaluation for vitamin B12 deficiency: the case for cascade testing. Clin. Med. Res. 11, 7–15. doi: 10.3121/cmr.2012.1112
Bjørke Monsen, A. L., and Ueland, P. M. (2003). Homocysteine and methylmalonic acid in diagnosis and risk assessment from infancy to adolescence. Am. J. Clin. Nutr. 78, 7–21.
Bjørke Monsen, A. L., Ueland, P. M., Vollset, S. E., Guttormsen, A. B., Markestad, T., Solheim, E., et al. (2001). Determinants of cobalamin status in newborns. Pediatrics 108, 624–630. doi: 10.1542/peds.108.3.624
Bjørke-Monsen, A. L., Torsvik, I., Sætran, H., Markestad, T., and Ueland, P. M. (2008). Common metabolic profile in infants indicating impaired cobalamin status responds to cobalamin supplementation. Pediatrics 122, 83–91. doi: 10.1542/peds.2007-2716
Black, A. K., Allen, L. H., Pelto, G. H., De Mata, M. P., and Chavez, A. (1994). Iron, vitamin B-12 and folate status in Mexico: associated factors in men and women and during pregnancy and lactation. J. Nutr. 124, 1179–1188.
Bowen, R. A., Dowdell, K. C., Dale, J. K., Drake, S. K., Fleisher, T. A., Hortin, G. L., et al. (2012). Elevated vitamin B(1)(2) levels in autoimmune lymphoproliferative syndrome attributable to elevated haptocorrin in lymphocytes. Clin. Biochem. 45, 490–492. doi: 10.1016/j.clinbiochem.2012.01.016
Brito, A., Verdugo, R., Hertrampf, E., Miller, J. W., Green, R., Fedosov, S. N., et al. (2016). Vitamin B-12 treatment of asymptomatic, deficient, elderly Chileans improves conductivity in myelinated peripheral nerves, but high serum folate impairs vitamin B-12 status response assessed by the combined indicator of vitamin B-12 status. Am. J. Clin. Nutr. 103, 250–257. doi: 10.3945/ajcn.115.116509
Caminha, I., Fleisher, T. A., Hornung, R. L., Dale, J. K., Niemela, J. E., Price, S., et al. (2010). Using biomarkers to predict the presence of FAS mutations in patients with features of the autoimmune lymphoproliferative syndrome. J. Allergy Clin. Immunol. 125, 946–949.e6 doi: 10.1016/j.jaci.2009.12.983
Carmel, R. (1996). Subtle cobalamin deficiency. Ann. Intern. Med. 124, 338–340. doi: 10.7326/0003-4819-124-3-199602010-00010
Carmel, R. (1999). Mild cobalamin deficiency in older Dutch subjects. Am. J. Clin. Nutr. 69, 738–739.
Carmel, R. (2000). Current concepts in cobalamin deficiency. Annu. Rev. Med. 51, 357–375. doi: 10.1146/annurev.med.51.1.357
Carmel, R. (2011). Biomarkers of cobalamin (vitamin B-12) status in the epidemiologic setting: a critical overview of context, applications, and performance characteristics of cobalamin, methylmalonic acid, and holotranscobalamin II. Am. J. Clin. Nutr. 94, 348S–358S. doi: 10.3945/ajcn.111.013441
Carmel, R. (2012). Subclinical cobalamin deficiency. Curr. Opin. Gastroenterol. 28, 151–158. doi: 10.1097/MOG.0b013e3283505852
Carmel, R. (2013). Diagnosis and management of clinical and subclinical cobalamin deficiencies: why controversies persist in the age of sensitive metabolic testing. Biochimie 95, 1047–1055. doi: 10.1016/j.biochi.2013.02.008
Clarke, R., Refsum, H., Birks, J., Evans, J. G., Johnston, C., Sherliker, P., et al. (2003). Screening for vitamin B-12 and folate deficiency in older persons. Am. J. Clin. Nutr. 77, 1241–1247.
Davey, G. K., Spencer, E. A., Appleby, P. N., Allen, N. E., Knox, K. H., and Key, T. J. (2003). EPIC-Oxford: lifestyle characteristics and nutrient intakes in a cohort of 33 883 meat-eaters and 31 546 non meat-eaters in the UK. Public Health Nutr. 6, 259–269. doi: 10.1079/PHN2002430
Dayaldasani, A., Ruiz-Escalera, J., Rodriguez-Espinosa, M., Rueda, I., Perez-Valero, V., and Yahyaoui, R. (2014). Serum vitamin B12 levels during the first trimester of pregnancy correlate with newborn screening markers of vitamin B12 deficiency. Int. J. Vitam. Nutr. Res. 84, 92–97. doi: 10.1024/0300-9831/a000196
de Benoist, B. (2008). Conclusions of a WHO technical consultation on folate and vitamin B12 deficiencies. Food Nutr. Bull. 29, S238–S244. doi: 10.1177/15648265080292S129
de Jager, J., Kooy, A., Lehert, P., Wulffele, M. G., Van Der Kolk, J., Bets, D., et al. (2010). Long term treatment with metformin in patients with type 2 diabetes and risk of vitamin B-12 deficiency: randomised placebo controlled trial. BMJ 340:c2181. doi: 10.1136/bmj.c2181
Devalia, V., Hamilton, M. S., Molloy, A. M., and British Committee for Standards in Haematology (2014). Guidelines for the diagnosis and treatment of cobalamin and folate disorders. Br. J. Haematol. 166, 496–513. doi: 10.1111/bjh.12959
Dror, D. K., and Allen, L. H. (2008). Effect of vitamin B12 deficiency on neurodevelopment in infants: current knowledge and possible mechanisms. Nutr. Rev. 66, 250–255. doi: 10.1111/j.1753-4887.2008.00031.x
Duggan, C., Srinivasan, K., Thomas, T., Samuel, T., Rajendran, R., Muthayya, S., et al. (2014). Vitamin B-12 supplementation during pregnancy and early lactation increases maternal, breast milk, and infant measures of vitamin B-12 status. J. Nutr. 144, 758–764. doi: 10.3945/jn.113.187278
Dutchak, P. A., Laxman, S., Estill, S. J., Wang, C., Wang, Y., Wang, Y., et al. (2015). Regulation of Hematopoiesis and Methionine Homeostasis by mTORC1 Inhibitor NPRL2. Cell Rep. 12, 371–379. doi: 10.1016/j.celrep.2015.06.042
Fedosov, S. N. (2012). Physiological and molecular aspects of cobalamin transport. Subcell. Biochem. 56, 347–367. doi: 10.1007/978-94-007-2199-9_18
Fedosov, S. N. (2013). Biochemical markers of vitamin B12 deficiency combined in one diagnostic parameter: the age-dependence and association with cognitive function and blood hemoglobin. Clin. Chim. Acta 422, 47–53. doi: 10.1016/j.cca.2013.04.002
Fedosov, S. N., Brito, A., Miller, J. W., Green, R., and Allen, L. H. (2015). Combined indicator of vitamin B12 status: modification for missing biomarkers and folate status and recommendations for revised cut-points. Clin. Chem. Lab. Med. 53, 1215–1225. doi: 10.1515/cclm-2014-0818
Fedosov, S. N., Fedosova, N. U., Krautler, B., Nexo, E., and Petersen, T. E. (2007). Mechanisms of discrimination between cobalamins and their natural analogues during their binding to the specific B12-transporting proteins. Biochemistry 46, 6446–6458. doi: 10.1021/bi062063l
Finkelstein, J. D., and Martin, J. J. (1984). Methionine metabolism in mammals. Distribution of homocysteine between competing pathways. J. Biol. Chem. 259, 9508–9513.
Fokkema, M. R., Woltil, H. A., Van Beusekom, C. M., Schaafsma, A., Dijck-Brouwer, D. A., and Muskiet, F. A. (2002). Plasma total homocysteine increases from day 20 to 40 in breastfed but not formula-fed low-birthweight infants. Acta Paediatr. 91, 507–511. doi: 10.1111/j.1651-2227.2002.tb03268.x
Ford, C., Rendle, M., Tracy, M., Richardson, V., and Ford, H. (1996). Vitamin B12 levels in human milk during the first nine months of lactation. Int. J. Vitam. Nutr. Res. 66, 329–331.
Frery, N., Huel, G., Leroy, M., Moreau, T., Savard, R., Blot, P., et al. (1992). Vitamin B12 among parturients and their newborns and its relationship with birthweight. Eur. J. Obstet. Gynecol. Reprod. Biol. 45, 155–163. doi: 10.1016/0028-2243(92)90076-B
Froese, D. S., and Gravel, R. A. (2010). Genetic disorders of vitamin B(1)(2) metabolism: eight complementation groups–eight genes. Expert Rev. Mol. Med. 12, e37. doi: 10.1017/S1462399410001651
Gilsing, A. M., Crowe, F. L., Lloyd-Wright, Z., Sanders, T. A., Appleby, P. N., Allen, N. E., et al. (2010). Serum concentrations of vitamin B12 and folate in British male omnivores, vegetarians and vegans: results from a cross-sectional analysis of the EPIC-Oxford cohort study. Eur. J. Clin. Nutr. 64, 933–939. doi: 10.1038/ejcn.2010.142
Giugliani, E. R., Jorge, S. M., and Goncalves, A. L. (1985). Serum vitamin B12 levels in parturients, in the intervillous space of the placenta and in full-term newborns and their interrelationships with folate levels. Am. J. Clin. Nutr. 41, 330–335.
Green, R. (2012). Anemias beyond B12 and iron deficiency: the buzz about other B's, elementary, and nonelementary problems. Hematol. Am. Soc. Hematol. Educ. Prog. 2012, 492–498. doi: 10.1182/asheducation-2012.1.492
Greibe, E., Lildballe, D. L., Streym, S., Vestergaard, P., Rejnmark, L., Mosekilde, L., et al. (2013a). Cobalamin and haptocorrin in human milk and cobalamin-related variables in mother and child: a 9-mo longitudinal study. Am. J. Clin. Nutr. 98, 389–395. doi: 10.3945/ajcn.113.058479
Greibe, E., Miller, J. W., Foutouhi, S. H., Green, R., and Nexo, E. (2013b). Metformin increases liver accumulation of vitamin B12 - an experimental study in rats. Biochimie 95, 1062–1065. doi: 10.1016/j.biochi.2013.02.002
Greibe, E., Trolle, B., Bor, M. V., Lauszus, F. F., and Nexo, E. (2013c). Metformin lowers serum cobalamin without changing other markers of cobalamin status: a study on women with polycystic ovary syndrome. Nutrients 5, 2475–2482. doi: 10.3390/nu5072475
Grober, U., Kisters, K., and Schmidt, J. (2013). Neuroenhancement with vitamin B12-underestimated neurological significance. Nutrients 5, 5031–5045. doi: 10.3390/nu5125031
Guttormsen, A. B., Refsum, H., and Ueland, P. M. (1994). The interaction between nitrous oxide and cobalamin. Biochemical effects and clinical consequences. Acta Anaesthesiol. Scand. 38, 753–756. doi: 10.1111/j.1399-6576.1994.tb03996.x
Hannibal, L., Dibello, P. M., and Jacobsen, D. W. (2013). Proteomics of vitamin B12 processing. Clin. Chem. Lab. Med. 51, 477–488. doi: 10.1515/cclm-2012-0568
Hannibal, L., Kim, J., Brasch, N. E., Wang, S., Rosenblatt, D. S., Banerjee, R., et al. (2009). Processing of alkylcobalamins in mammalian cells: A role for the MMACHC (cblC) gene product. Mol. Genet. Metab. 97, 260–266. doi: 10.1016/j.ymgme.2009.04.005
Hartmann, B. (2008). Nationale Verzehrsstudie II (National Nutrition Survey II). Available online at: https://www.mri.bund.de/de/institute/ernaehrungsverhalten/forschungsprojekte/nvsii/ (Accessed June, 2016).
Hartmann, H., Das, A. M., and Lucke, T. (2009). Correspondence (letter to the editor): Risk group includes infants. Dtsch. Arztebl. Int. 106, 290–291; author reply 291. doi: 10.3238/arztebl.2009.0291
Hay, G., Johnston, C., Whitelaw, A., Trygg, K., and Refsum, H. (2008). Folate and cobalamin status in relation to breastfeeding and weaning in healthy infants. Am. J. Clin. Nutr. 88, 105–114.
Health Quality Ontario (2013). Vitamin B12 and cognitive function: an evidence-based analysis. Ont. Health Technol. Assess. Ser. 13, 1–45.
Heinzl, S. (2014). Vitamin-B12-mangel: protonenpumpenhemmer und H2-blocker als auslöser. Dtsch. Arztebl. Int. 111, 353–354.
Herrmann, W., and Obeid, R. (2008). Ursachen und frühzeitige Diagnostik von Vitamin-B12-Mangel. Dtsch Arztebl Int. 105, 680–685.
Herrmann, W., and Obeid, R. (2013). Utility and limitations of biochemical markers of vitamin B12 deficiency. Eur. J. Clin. Invest. 43, 231–237. doi: 10.1111/eci.12034
Hinton, C. F., Ojodu, J. A., Fernhoff, P. M., Rasmussen, S. A., Scanlon, K. S., and Hannon, W. H. (2010). Maternal and neonatal vitamin B12 deficiency detected through expanded newborn screening–United States, 2003-2007. J. Pediatr. 157, 162–163. doi: 10.1016/j.jpeds.2010.03.006
Hoffmann-Lucke, E., Arendt, J. F., Nissen, P. H., Mikkelsen, G., Aasly, J. O., and Nexo, E. (2013). Three family members with elevated plasma cobalamin, transcobalamin and soluble transcobalamin receptor (sCD320). Clin. Chem. Lab. Med. 51, 677–682. doi: 10.1515/cclm-2012-0554
Hogeveen, M., Den Heijer, M., Schonbeck, Y., Ijland, M., Van Oppenraaij, D., Gunnewiek, J. K., et al. (2010). The effect of folinic acid supplementation on homocysteine concentrations in newborns. Eur. J. Clin. Nutr. 64, 1266–1271. doi: 10.1038/ejcn.2010.155
Holzelova, E., Vonarbourg, C., Stolzenberg, M. C., Arkwright, P. D., Selz, F., Prieur, A. M., et al. (2004). Autoimmune lymphoproliferative syndrome with somatic Fas mutations. N. Engl. J. Med. 351, 1409–1418. doi: 10.1056/NEJMoa040036
Howden, C. W. (2000). Vitamin B12 levels during prolonged treatment with proton pump inhibitors. J. Clin. Gastroenterol. 30, 29–33. doi: 10.1097/00004836-200001000-00006
Huemer, M., Kozich, V., Rinaldo, P., Baumgartner, M. R., Merinero, B., Pasquini, E., et al. (2015). Newborn screening for homocystinurias and methylation disorders: systematic review and proposed guidelines. J. Inherit. Metab. Dis. 38, 1007–1019. doi: 10.1007/s10545-015-9830-z
Institute of Medicine (Us) Standing Committee on the Scientific Evaluation of Dietary Reference Intakes Its Panel on Folate, Other B Vitamins, Choline. (1998). Dietary Reference Intakes for Thiamin, Riboflavin, Niacin, Vitamin B6, Folate, Vitamin B12, Pantothenic Acid, Biotin, and Choline. Washington, DC: National Academy Press.
Iqbal, N., Azar, D., Yun, Y. M., Ghausi, O., Ix, J., and Fitzgerald, R. L. (2013). Serum methylmalonic acid and holotranscobalamin-II as markers for vitamin B12 deficiency in end-stage renal disease patients. Ann. Clin. Lab. Sci. 43, 243–249.
Jackson, C. E., Fischer, R. E., Hsu, A. P., Anderson, S. M., Choi, Y., Wang, J., et al. (1999). Autoimmune lymphoproliferative syndrome with defective Fas: genotype influences penetrance. Am. J. Hum. Genet. 64, 1002–1014. doi: 10.1086/302333
Jacobsen, D. W., Gatautis, V. J., Green, R., Robinson, K., Savon, S. R., Secic, M., et al. (1994). Rapid HPLC determination of total homocysteine and other thiols in serum and plasma: sex differences and correlation with cobalamin and folate concentrations in healthy subjects. Clin. Chem. 40, 873–881.
Jacques, P. F., Selhub, J., Bostom, A. G., Wilson, P. W., and Rosenberg, I. H. (1999). The effect of folic acid fortification on plasma folate and total homocysteine concentrations. N. Engl. J. Med. 340, 1449–1454. doi: 10.1056/NEJM199905133401901
Jiang, W., Nakayama, Y., Sequeira, J. M., and Quadros, E. V. (2013). Mapping the functional domains of TCblR/CD320, the receptor for cellular uptake of transcobalamin-bound cobalamin. FASEB J. 27, 2988–2994. doi: 10.1096/fj.13-230185
Keller, P., Rufener, J., Schild, C., Fedosov, S. N., Nissen, P. H., and Nexo, E. (2016). False low holotranscobalamin levels in a patient with a novel TCN2 mutation. Clin. Chem. Lab. Med. doi: 10.1515/cclm-2016-0063. [Epub ahead of print].
Koch, C. A. (2009). Correspondence (letter to the editor): Gastrin levels in pernicious anemia. Dtsch. Arztebl. Int. 106:290. doi: 10.3238/arztebl.2009.0290a
Kristensen, N. B., Madsen, M. L., Hansen, T. H., Allin, K. H., Hoppe, C., Fagt, S., et al. (2015). Intake of macro- and micronutrients in Danish vegans. Nutr. J. 14, 115. doi: 10.1186/s12937-015-0103-3
Kumar, N. (2014). Neurologic aspects of cobalamin (B12) deficiency. Handb. Clin. Neurol. 120, 915–926. doi: 10.1016/B978-0-7020-4087-0.00060-7
Kwon, Y., Kim, H. J., Lo Menzo, E., Park, S., Szomstein, S., and Rosenthal, R. J. (2014). Anemia, iron and vitamin B12 deficiencies after sleeve gastrectomy compared to Roux-en-Y gastric bypass: a meta-analysis. Surg. Obes. Relat. Dis. 10, 589–597. doi: 10.1016/j.soard.2013.12.005
Lemberger, T., Saladin, R., Vazquez, M., Assimacopoulos, F., Staels, B., Desvergne, B., et al. (1996). Expression of the peroxisome proliferator-activated receptor alpha gene is stimulated by stress and follows a diurnal rhythm. J. Biol. Chem. 271, 1764–1769. doi: 10.1074/jbc.271.3.1764
Leung, S., Mattman, A., Snyder, F., Kassam, R., Meneilly, G., and Nexo, E. (2010). Metformin induces reductions in plasma cobalamin and haptocorrin bound cobalamin levels in elderly diabetic patients. Clin. Biochem. 43, 759–760. doi: 10.1016/j.clinbiochem.2010.02.011
Levin, J., Botzel, K., Giese, A., Vogeser, M., and Lorenzl, S. (2010). Elevated levels of methylmalonate and homocysteine in Parkinson's disease, progressive supranuclear palsy and amyotrophic lateral sclerosis. Dement. Geriatr. Cogn. Disord. 29, 553–559. doi: 10.1159/000314841
Lewerin, C., Ljungman, S., and Nilsson-Ehle, H. (2007). Glomerular filtration rate as measured by serum cystatin C is an important determinant of plasma homocysteine and serum methylmalonic acid in the elderly. J. Intern. Med. 261, 65–73. doi: 10.1111/j.1365-2796.2006.01732.x
Lildballe, D. L., Hardlei, T. F., Allen, L. H., and Nexo, E. (2009). High concentrations of haptocorrin interfere with routine measurement of cobalamins in human serum and milk. A problem and its solution. Clin. Chem. Lab. Med. 47, 182–187. doi: 10.1515/CCLM.2009.047
Liu, Q., Li, S., Quan, H., and Li, J. (2014). Vitamin B12 status in metformin treated patients: systematic review. PLoS ONE 9:e100379. doi: 10.1371/journal.pone.0100379
Luhby, A. L., Cooperman, J. M., Stone, M. L., and Slobody, L. B. (1958). Physiology of vitamin B12 in pregnancy, the placenta, and the newborn. Am. J. Dis. Child 96, 127–128.
Lysne, V., Strand, E., Svingen, G. F. T., Bjørndal, B., Pedersen, E. R., Midttun, Ø., et al. (2016). Peroxisome proliferator-activated receptor activation is associated with altered plasma one-carbon metabolites and B-vitamin status in rats. Nutrients 8:26. doi: 10.3390/nu8010026
Magerus-Chatinet, A., Stolzenberg, M. C., Loffredo, M. S., Neven, B., Schaffner, C., Ducrot, N., et al. (2009). FAS-L, IL-10, and double-negative CD4- CD8- TCR alpha/beta+ T cells are reliable markers of autoimmune lymphoproliferative syndrome (ALPS) associated with FAS loss of function. Blood 113, 3027–3030. doi: 10.1182/blood-2008-09-179630
Majumder, S., Soriano, J., Louie Cruz, A., and Dasanu, C. A. (2013). Vitamin B12 deficiency in patients undergoing bariatric surgery: preventive strategies and key recommendations. Surg. Obes. Relat. Dis. 9, 1013–1019. doi: 10.1016/j.soard.2013.04.017
Marcadier, J. L., Smith, A. M., Pohl, D., Schwartzentruber, J., Al-Dirbashi, O. Y., Consortium, F. C., et al. (2013). Mutations in ALDH6A1 encoding methylmalonate semialdehyde dehydrogenase are associated with dysmyelination and transient methylmalonic aciduria. Orphanet J. Rare Dis. 8:98. doi: 10.1186/1750-1172-8-98
Marcuard, S. P., Sinar, D. R., Swanson, M. S., Silverman, J. F., and Levine, J. S. (1989). Absence of luminal intrinsic factor after gastric bypass surgery for morbid obesity. Dig. Dis. Sci. 34, 1238–1242. doi: 10.1007/BF01537272
McPhee, A. J., Davidson, G. P., Leahy, M., and Beare, T. (1988). Vitamin B12 deficiency in a breast fed infant. Arch. Dis. Child. 63, 921–923. doi: 10.1136/adc.63.8.921
Miles, L. M., Mills, K., Clarke, R., and Dangour, A. D. (2015). Is there an association of vitamin B12 status with neurological function in older people? A systematic review. Br. J. Nutr. 114, 503–508. doi: 10.1017/S0007114515002226
Miller, J. W., Garrod, M. G., Allen, L. H., Haan, M. N., and Green, R. (2009). Metabolic evidence of vitamin B-12 deficiency, including high homocysteine and methylmalonic acid and low holotranscobalamin, is more pronounced in older adults with elevated plasma folate. Am. J. Clin. Nutr. 90, 1586–1592. doi: 10.3945/ajcn.2009.27514
Miller, R. K., Faber, W., Asai, M., D'gregorio, R. P., Ng, W. W., Shah, Y., et al. (1993). The role of the human placenta in embryonic nutrition. Impact of environmental and social factors. Ann. N.Y. Acad. Sci. 678, 92–107. doi: 10.1111/j.1749-6632.1993.tb26112.x
Minet, J. C., Bisse, E., Aebischer, C. P., Beil, A., Wieland, H., and Lutschg, J. (2000). Assessment of vitamin B-12, folate, and vitamin B-6 status and relation to sulfur amino acid metabolism in neonates. Am. J. Clin. Nutr. 72, 751–757.
Mirkazemi, C., Peterson, G. M., Tenni, P. C., and Jackson, S. L. (2012). Vitamin B12 deficiency in Australian residential aged care facilities. J. Nutr. Health Aging 16, 277–280. doi: 10.1007/s12603-011-0348-2
Molloy, A. M., Pangilinan, F., Mills, J. L., Shane, B., O'Neill, M. B., McGaughey, D. M., et al. (2016). A common polymorphism in HIBCH influences methylmalonic acid concentrations in blood independently of cobalamin. Am. J. Hum. Genet. 98, 869–882. doi: 10.1016/j.ajhg.2016.03.005
Monsen, A. L., Refsum, H., Markestad, T., and Ueland, P. M. (2003). Cobalamin status and its biochemical markers methylmalonic acid and homocysteine in different age groups from 4 days to 19 years. Clin. Chem. 49, 2067–2075. doi: 10.1373/clinchem.2003.019869
Moore, E. M., Ames, D., Mander, A. G., Carne, R. P., Brodaty, H., Woodward, M. C., et al. (2014). Among vitamin B12 deficient older people, high folate levels are associated with worse cognitive function: combined data from three cohorts. J. Alzheimers. Dis. 39, 661–668. doi: 10.3233/JAD-131265
Moore, E., Mander, A., Ames, D., Carne, R., Sanders, K., and Watters, D. (2012). Cognitive impairment and vitamin B12: a review. Int. Psychogeriatr. 24, 541–556. doi: 10.1017/S1041610211002511
Morris, M. S., Jacques, P. F., Rosenberg, I. H., and Selhub, J. (2007). Folate and vitamin B-12 status in relation to anemia, macrocytosis, and cognitive impairment in older Americans in the age of folic acid fortification. Am. J. Clin. Nutr. 85, 193–200.
Muller, T. (2009). Possible treatment concepts for the levodopa-related hyperhomocysteinemia. Cardiovasc. Psychiatry Neurol. 2009:969752. doi: 10.1155/2009/969752
Muller, T., Van Laar, T., Cornblath, D. R., Odin, P., Klostermann, F., Grandas, F. J., et al. (2013). Peripheral neuropathy in Parkinson's disease: levodopa exposure and implications for duodenal delivery. Parkinsonism Relat. Disord. 19, 501–507. discussion: 501. doi: 10.1016/j.parkreldis.2013.02.006
Nagata, S., and Golstein, P. (1995). The Fas death factor. Science 267, 1449–1456. doi: 10.1126/science.7533326
Nexo, E., Christensen, A. L., Petersen, T. E., and Fedosov, S. N. (2000). Measurement of transcobalamin by ELISA. Clin. Chem. 46, 1643–1649.
Nexo, E., Hvas, A. M., Bleie, O., Refsum, H., Fedosov, S. N., Vollset, S. E., et al. (2002). Holo-transcobalamin is an early marker of changes in cobalamin homeostasis. A randomized placebo-controlled study. Clin. Chem. 48, 1768–1771.
Obeid, R. (2014). Metformin causing vitamin B12 deficiency: a guilty verdict without sufficient evidence. Diabetes Care 37, e22–e23. doi: 10.2337/dc13-2278
Obeid, R., Jung, J., Falk, J., Herrmann, W., Geisel, J., Friesenhahn-Ochs, B., et al. (2013). Serum vitamin B12 not reflecting vitamin B12 status in patients with type 2 diabetes. Biochimie 95, 1056–1061. doi: 10.1016/j.biochi.2012.10.028
Obeid, R., Morkbak, A. L., Munz, W., Nexo, E., and Herrmann, W. (2006). The cobalamin-binding proteins transcobalamin and haptocorrin in maternal and cord blood sera at birth. Clin. Chem. 52, 263–269. doi: 10.1373/clinchem.2005.057810
Oliveira, J. B. (2013). The expanding spectrum of the autoimmune lymphoproliferative syndromes. Curr. Opin. Pediatr. 25, 722–729. doi: 10.1097/mop.0000000000000032
Oliveira, J. B., Bleesing, J. J., Dianzani, U., Fleisher, T. A., Jaffe, E. S., Lenardo, M. J., et al. (2010). Revised diagnostic criteria and classification for the autoimmune lymphoproliferative syndrome (ALPS): report from the 2009 NIH International Workshop. Blood 116, e35–e40. doi: 10.1182/blood-2010-04-280347
Palacios, G., Sola, R., Barrios, L., Pietrzik, K., Castillo, M. J., and Gonzalez-Gross, M. (2013). Algorithm for the early diagnosis of vitamin B12 deficiency in elderly people. Nutr. Hosp. 28, 1447–1452. doi: 10.3305/nh.2013.28.5.6821
Pawlak, R., Lester, S. E., and Babatunde, T. (2014). The prevalence of cobalamin deficiency among vegetarians assessed by serum vitamin B12: a review of literature. Eur. J. Clin. Nutr. 68, 541–548. doi: 10.1038/ejcn.2014.46
Pawlak, R., Parrott, S. J., Raj, S., Cullum-Dugan, D., and Lucus, D. (2013). How prevalent is vitamin B(12) deficiency among vegetarians? Nutr. Rev. 71, 110–117. doi: 10.1111/nure.12001
Perez-D'gregorio, R. E., and Miller, R. K. (1998). Transport and endogenous release of vitamin B12 in the dually perfused human placenta. J. Pediatr. 132, S35–S42. doi: 10.1016/s0022-3476(98)70526-8
Porck, H. J., Frater-Schroder, M., Frants, R. R., Kierat, L., and Eriksson, A. W. (1983). Genetic evidence for fetal origin of transcobalamin II in human cord blood. Blood 62, 234–237.
Pupavac, M., Tian, X., Chu, J., Wang, G., Feng, Y., Chen, S., et al. (2016). Added value of next generation gene panel analysis for patients with elevated methylmalonic acid and no clinical diagnosis following functional studies of vitamin B12 metabolism. Mol. Genet. Metab. 117, 363–368. doi: 10.1016/j.ymgme.2016.01.008
Quadros, E. V., and Sequeira, J. M. (2013). Cellular uptake of cobalamin: transcobalamin and the TCblR/CD320 receptor. Biochimie 95, 1008–1018. doi: 10.1016/j.biochi.2013.02.004
Quadros, E. V., Lai, S. C., Nakayama, Y., Sequeira, J. M., Hannibal, L., Wang, S., et al. (2010). Positive newborn screen for methylmalonic aciduria identifies the first mutation in TCblR/CD320, the gene for cellular uptake of transcobalamin-bound vitamin B(12). Hum. Mutat. 31, 924–929. doi: 10.1002/humu.21297
Quadros, E. V., Nakayama, Y., and Sequeira, J. M. (2009). The protein and the gene encoding the receptor for the cellular uptake of transcobalamin-bound cobalamin. Blood 113, 186–192. doi: 10.1182/blood-2008-05-158949
Rasmussen, K., Moller, J., Lyngbak, M., Pedersen, A. M., and Dybkjaer, L. (1996). Age- and gender-specific reference intervals for total homocysteine and methylmalonic acid in plasma before and after vitamin supplementation. Clin. Chem. 42, 630–636.
Rasmussen, S. A., Fernhoff, P. M., and Scanlon, K. S. (2001). Vitamin B12 deficiency in children and adolescents. J. Pediatr. 138, 10–17. doi: 10.1067/mpd.2001.112160
Refsum, H., Yajnik, C. S., Gadkari, M., Schneede, J., Vollset, S. E., Orning, L., et al. (2001). Hyperhomocysteinemia and elevated methylmalonic acid indicate a high prevalence of cobalamin deficiency in Asian Indians. Am. J. Clin. Nutr. 74, 233–241.
Remacha, A. F., Sarda, M. P., Canals, C., Queralto, J. M., Zapico, E., Remacha, J., et al. (2013). Combined cobalamin and iron deficiency anemia: a diagnostic approach using a model based on age and homocysteine assessment. Ann. Hematol. 92, 527–531. doi: 10.1007/s00277-012-1634-8
Remacha, A. F., Sarda, M. P., Canals, C., Queralto, J. M., Zapico, E., Remacha, J., et al. (2014). Role of serum holotranscobalamin (holoTC) in the diagnosis of patients with low serum cobalamin. Comparison with methylmalonic acid and homocysteine. Ann. Hematol. 93, 565–569. doi: 10.1007/s00277-013-1905-z
Riedel, B. M., Molloy, A. M., Meyer, K., Fredriksen, A., Ulvik, A., Schneede, J., et al. (2011). Transcobalamin polymorphism 67A->G, but not 776C->G, affects serum holotranscobalamin in a cohort of healthy middle-aged men and women. J. Nutr. 141, 1784–1790. doi: 10.3945/jn.111.141960
Riedel, B., Bjørke Monsen, A. L., Ueland, P. M., and Schneede, J. (2005). Effects of oral contraceptives and hormone replacement therapy on markers of cobalamin status. Clin. Chem. 51, 778–781. doi: 10.1373/clinchem.2004.043828
Rieux-Laucat, F., Fischer, A., and Deist, F. L. (2003). Cell-death signaling and human disease. Curr. Opin. Immunol. 15, 325–331. doi: 10.1016/S0952-7915(03)00042-6
Risch, M., Meier, D. W., Sakem, B., Medina Escobar, P., Risch, C., Nydegger, U., et al. (2015). Vitamin B12 and folate levels in healthy Swiss senior citizens: a prospective study evaluating reference intervals and decision limits. BMC Geriatr. 15:82. doi: 10.1186/s12877-015-0060-x
Rizzo, N. S., Jaceldo-Siegl, K., Sabate, J., and Fraser, G. E. (2013). Nutrient profiles of vegetarian and nonvegetarian dietary patterns. J. Acad. Nutr. Diet. 113, 1610–1619. doi: 10.1016/j.jand.2013.06.349
Sarafoglou, K., Rodgers, J., Hietala, A., Matern, D., and Bentler, K. (2011). Expanded newborn screening for detection of vitamin B12 deficiency. JAMA 305, 1198–1200. doi: 10.1001/jama.2011.310
Schedvin, G., Jones, I., Hultdin, J., and Nilsson, T. K. (2005). A laboratory algorithm with homocysteine as the primary parameter reduces the cost of investigation of folate and cobalamin deficiency. Clin. Chem. Lab. Med. 43, 1065–1068. doi: 10.1515/CCLM.2005.186
Schneede, J., Dagnelie, P. C., Van Staveren, W. A., Vollset, S. E., Refsum, H., and Ueland, P. M. (1994). Methylmalonic acid and homocysteine in plasma as indicators of functional cobalamin deficiency in infants on macrobiotic diets. Pediatr. Res. 36, 194–201. doi: 10.1203/00006450-199408000-00010
Schupbach, R., Wegmüller, R., Berguerand, C., Bui, M., and Herter-Aeberli, I. (2015). Micronutrient status and intake in omnivores, vegetarians and vegans in Switzerland. Eur. J. Nutr. doi: 10.1007/s00394-015-1079-7. [Epub ahead of print].
Seif, A. E., Manno, C. S., Sheen, C., Grupp, S. A., and Teachey, D. T. (2010). Identifying autoimmune lymphoproliferative syndrome in children with Evans syndrome: a multi-institutional study. Blood 115, 2142–2145. doi: 10.1182/blood-2009-08-239525
Selhub, J., Jacques, P. F., Dallal, G., Choumenkovitch, S., and Rogers, G. (2008). The use of blood concentrations of vitamins and their respective functional indicators to define folate and vitamin B12 status. Food Nutr. Bull. 29, S67–S73. doi: 10.1177/15648265080292S110
Selhub, J., Morris, M. S., and Jacques, P. F. (2007). In vitamin B12 deficiency, higher serum folate is associated with increased total homocysteine and methylmalonic acid concentrations. Proc. Natl. Acad. Sci. U.S.A. 104, 19995–20000. doi: 10.1073/pnas.0709487104
Shah, M., Simha, V., and Garg, A. (2006). Review: long-term impact of bariatric surgery on body weight, comorbidities, and nutritional status. J. Clin. Endocrinol. Metab. 91, 4223–4231. doi: 10.1210/jc.2006-0557
Shah, S., Wu, E., Rao, V. K., and Tarrant, T. K. (2014). Autoimmune lymphoproliferative syndrome: an update and review of the literature. Curr. Allergy Asthma Rep. 14, 462. doi: 10.1007/s11882-014-0462-4
Sheen, E., and Triadafilopoulos, G. (2011). Adverse effects of long-term proton pump inhibitor therapy. Dig. Dis. Sci. 56, 931–950. doi: 10.1007/s10620-010-1560-3
Singer, M. A., Lazaridis, C., Nations, S. P., and Wolfe, G. I. (2008). Reversible nitrous oxide-induced myeloneuropathy with pernicious anemia: case report and literature review. Muscle Nerve 37, 125–129. doi: 10.1002/mus.20840
Sloan, J. L., Johnston, J. J., Manoli, I., Chandler, R. J., Krause, C., Carrillo-Carrasco, N., et al. (2011). Exome sequencing identifies ACSF3 as a cause of combined malonic and methylmalonic aciduria. Nat. Genet. 43, 883–886. doi: 10.1038/ng.908
Solomon, L. R. (2005). Cobalamin-responsive disorders in the ambulatory care setting: unreliability of cobalamin, methylmalonic acid, and homocysteine testing. Blood 105, 978–985; author reply 1137. doi: 10.1182/blood-2004-04-1641
Solomon, L. R. (2015). Functional cobalamin (vitamin B12) deficiency: role of advanced age and disorders associated with increased oxidative stress. Eur. J. Clin. Nutr. 69, 687–692. doi: 10.1038/ejcn.2014.272
Specker, B. L., Black, A., Allen, L., and Morrow, F. (1990a). Vitamin-B-12: low milk concentrations are related to low serum concentrations in vegetarian women and to methylmalonic aciduria in their Infants. Am. J. Clin. Nutr. 52, 1073–1076.
Specker, B. L., Brazerol, W., Ho, M. L., and Norman, E. J. (1990b). Urinary methylmalonic acid excretion in infants fed formula or human milk. Am. J. Clin. Nutr. 51, 209–211.
Stabler, S. P. (2012). “Vitamin B12,” in Present Knowledge in Nutrition, eds J. W. Erdman, I. A. Macdonald, and S. H. Zeisel (Oxford, UK: Wiley-Blackwell), 343–358.
Stockler, S., Corvera, S., Lambright, D., Fogarty, K., Nosova, E., Leonard, D., et al. (2014). Single point mutation in Rabenosyn-5 in a female with intractable seizures and evidence of defective endocytotic trafficking. Orphanet J. Rare Dis. 9:141. doi: 10.1186/s13023-014-0141-5
Sumner, A. E., Chin, M. M., Abrahm, J. L., Berry, G. T., Gracely, E. J., Allen, R. H., et al. (1996). Elevated methylmalonic acid and total homocysteine levels show high prevalence of vitamin B12 deficiency after gastric surgery. Ann. Intern. Med. 124, 469–476. doi: 10.7326/0003-4819-124-5-199603010-00002
Teachey, D. T., Manno, C. S., Axsom, K. M., Andrews, T., Choi, J. K., Greenbaum, B. H., et al. (2005). Unmasking Evans syndrome: T-cell phenotype and apoptotic response reveal autoimmune lymphoproliferative syndrome (ALPS). Blood 105, 2443–2448. doi: 10.1182/blood-2004-09-3542
Theethira, T. G., Dennis, M., and Leffler, D. A. (2014). Nutritional consequences of celiac disease and the gluten-free diet. Expert Rev. Gastroenterol. Hepatol. 8, 123–129. doi: 10.1586/17474124.2014.876360
Ting, R. Z., Szeto, C. C., Chan, M. H., Ma, K. K., and Chow, K. M. (2006). Risk factors of vitamin B(12) deficiency in patients receiving metformin. Arch. Intern. Med. 166, 1975–1979. doi: 10.1001/archinte.166.18.1975
Torsvik, I. K., Ueland, P. M., Markestad, T., Midttun, O., and Monsen, A. L. (2015). Motor development related to duration of exclusive breastfeeding, B vitamin status and B12 supplementation in infants with a birth weight between 2000-3000 g, results from a randomized intervention trial. BMC Pediatr. 15:218. doi: 10.1186/s12887-015-0533-2
Torsvik, I., Ueland, P. M., Markestad, T., and Bjørke-Monsen, A. L. (2013). Cobalamin supplementation improves motor development and regurgitations in infants: results from a randomized intervention study. Am. J. Clin. Nutr. 98, 1233–1240. doi: 10.3945/ajcn.113.061549
Ueland, P. M., Refsum, H., Stabler, S. P., Malinow, M. R., Andersson, A., and Allen, R. H. (1993). Total homocysteine in plasma or serum: methods and clinical applications. Clin. Chem. 39, 1764–1779.
Valayannopoulos, V., Haudry, C., Serre, V., Barth, M., Boddaert, N., Arnoux, J. B., et al. (2010). New SUCLG1 patients expanding the phenotypic spectrum of this rare cause of mild methylmalonic aciduria. Mitochondrion 10, 335–341. doi: 10.1016/j.mito.2010.02.006
Valdes-Ramos, R., Guadarrama-Lopez, A. L., Martinez-Carrillo, B. E., and Benitez-Arciniega, A. D. (2015). Vitamins and type 2 diabetes mellitus. Endocr. Metab. Immune Disord. Drug Targets 15, 54–63. doi: 10.2174/1871530314666141111103217
Valente, E., Scott, J. M., Ueland, P. M., Cunningham, C., Casey, M., and Molloy, A. M. (2011). Diagnostic accuracy of holotranscobalamin, methylmalonic acid, serum cobalamin, and other indicators of tissue vitamin B(1)(2) status in the elderly. Clin. Chem. 57, 856–863. doi: 10.1373/clinchem.2010.158154
van Amsterdam, J., Nabben, T., and Van Den Brink, W. (2015). Recreational nitrous oxide use: Prevalence and risks. Regul. Toxicol. Pharmacol. 73, 790–796. doi: 10.1016/j.yrtph.2015.10.017
van Beynum, I. M., den Heijer, M., Thomas, C. M., Afman, L., Oppenraay-van Emmerzaal, D., and Blom, H. J. (2005). Total homocysteine and its predictors in Dutch children. Am. J. Clin. Nutr. 81, 1110–1116.
Varbanova, M., Frauenschlager, K., and Malfertheiner, P. (2014). Chronic gastritis - an update. Best Pract. Res. Clin. Gastroenterol. 28, 1031–1042. doi: 10.1016/j.bpg.2014.10.005
Vashi, P., Edwin, P., Popiel, B., Lammersfeld, C., and Gupta, D. (2016). Methylmalonic acid and homocysteine as indicators of vitamin B-12 deficiency in cancer. PLoS ONE 11:e0147843. doi: 10.1371/journal.pone.0147843
Vu, T., Amin, J., Ramos, M., Flener, V., Vanyo, L., and Tisman, G. (1993). New assay for the rapid determination of plasma holotranscobalamin II levels: preliminary evaluation in cancer patients. Am. J. Hematol. 42, 202–211. doi: 10.1002/ajh.2830420212
Watkins, D., and Rosenblatt, D. S. (2011). Inborn errors of cobalamin absorption and metabolism. Am. J. Med. Genet. C Semin. Med. Genet. 157C, 33–44. doi: 10.1002/ajmg.c.30288
Watkins, D., and Rosenblatt, D. S. (2013). Lessons in biology from patients with inborn errors of vitamin B12 metabolism. Biochimie 95, 1019–1022. doi: 10.1016/j.biochi.2013.01.013
Wheeler, S. (2008). Assessment and interpretation of micronutrient status during pregnancy. Proc. Nutr. Soc. 67, 437–450. doi: 10.1017/S0029665108008732
Wickramasinghe, S. N., and Ratnayaka, I. D. (1996). Limited value of serum holo-transcobalamin II measurements in the differential diagnosis of macrocytosis. J. Clin. Pathol. 49, 755–758. doi: 10.1136/jcp.49.9.755
Wilhelm, S. M., Rjater, R. G., and Kale-Pradhan, P. B. (2013). Perils and pitfalls of long-term effects of proton pump inhibitors. Expert Rev. Clin. Pharmacol. 6, 443–451. doi: 10.1586/17512433.2013.811206
Yetley, E. A., Pfeiffer, C. M., Phinney, K. W., Bailey, R. L., Blackmore, S., Bock, J. L., et al. (2011). Biomarkers of vitamin B-12 status in NHANES: a roundtable summary. Am. J. Clin. Nutr. 94, 313S–321S. doi: 10.3945/ajcn.111.013243
Keywords: vitamin B12, cobalamin, homocysteine, methylmalonic acid, holo-transcobalamin, diagnostic algorithm, functional deficiency of B12
Citation: Hannibal L, Lysne V, Bjørke-Monsen A-L, Behringer S, Grünert SC, Spiekerkoetter U, Jacobsen DW and Blom HJ (2016) Biomarkers and Algorithms for the Diagnosis of Vitamin B12 Deficiency. Front. Mol. Biosci. 3:27. doi: 10.3389/fmolb.2016.00027
Received: 15 April 2016; Accepted: 07 June 2016;
Published: 27 June 2016.
Edited by:
Thomas Nägele, University of Vienna, AustriaReviewed by:
Andrea Fuso, Sapienza University of Rome, ItalyMatej Oresic, University of Turku, Finland
Copyright © 2016 Hannibal, Lysne, Bjørke-Monsen, Behringer, Grünert, Spiekerkoetter, Jacobsen and Blom. This is an open-access article distributed under the terms of the Creative Commons Attribution License (CC BY). The use, distribution or reproduction in other forums is permitted, provided the original author(s) or licensor are credited and that the original publication in this journal is cited, in accordance with accepted academic practice. No use, distribution or reproduction is permitted which does not comply with these terms.
*Correspondence: Luciana Hannibal, bHVjaWFuYS5oYW5uaWJhbEB1bmlrbGluaWstZnJlaWJ1cmcuZGU=