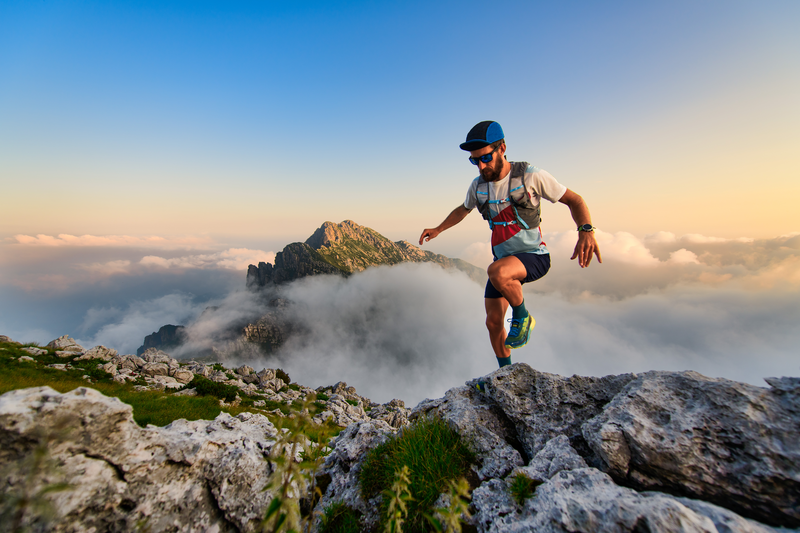
94% of researchers rate our articles as excellent or good
Learn more about the work of our research integrity team to safeguard the quality of each article we publish.
Find out more
ORIGINAL RESEARCH article
Front. Microbiomes
Sec. Host and Microbe Associations
Volume 4 - 2025 | doi: 10.3389/frmbi.2025.1567462
The final, formatted version of the article will be published soon.
You have multiple emails registered with Frontiers:
Please enter your email address:
If you already have an account, please login
You don't have a Frontiers account ? You can register here
Alfalfa forms and rumen degradable starch (RDS) levels in diets can profoundly affect growth performance and rumen fermentation patterns, this influence may result in rumen microbiota varying differently. However, the effects of RDS levels on methanogenic and fungal communities in alfalfa hay (AH) or alfalfa silage (AS) diets, as well as the interaction between methanogens and fungi with growth performance and rumen fermentation patterns, remain unknown. In this study, a 2 × 2 factorial design resulted in four diets: two alfalfa forms (AH; and AS) and two RDS levels (LR: 14.85% DM RDS; and HR: 20.21% DM RDS). We used thirty-two Suffolk female sheep for the experiment. On day 75 (including a 15 days transition period and a 60 days trial period), rumen content was collected after slaughter to examine the ruminal methanogens and fungi. The AHHR diet reduced methanogens Chao 1 index compared to the AS diets (P < 0.05), and the Shannon index was lower than in the ASLR diet (P < 0.05). The fungi Chao 1 index was higher in the AH diets than in the ASHR diet (P < 0.05), and the fungi Shannon index was higher in the LR diets than in the HR diets (P < 0.05). The relative abundance of Aspergillus in the AHLR diet was significantly higher than in the AS diets (P < 0.01), and the relative abundance of Occultifur and Meyerozyma were decreased in the AH than in the AS diets (P < 0.05). The LEfSe analysis showed that Methanobrevibacter_sp_YE315 and Methanobrevibacter_sp_AbM4 were enriched in the ASLR diet, while Methanobrevibacter_millerae was enriched in the ASHR diet. For the fungal biomarkers, the AHLR diet included Aspergillus, Metschnikowia, unclassified_f__Stachybotryaceae, the AHHR diet included stachybotrys, Stemphylium and Cystobasidium, the ASLR diet included unclassified_k__Fungi, Trichothecium, and Psathyrella, and the ASHR diet included Alfaria. Correlation analysis results showed the relative abundance of Methanobrevibacter, Methanoculleus, Penicillium, Cladosporium, and Exophiala and the concentration of isobutyrate and isovalerate, which may provide deeper insights into the previously observed differences.
Keywords: Alfalfa hay, Alfalfa silage, Rumen degradable starch, Rumen, Methanogens, Fungi
Received: 27 Jan 2025; Accepted: 19 Mar 2025.
Copyright: © 2025 Guo, Na, Liu, Li, Du, Zhang, Zhang, Na and Liu. This is an open-access article distributed under the terms of the Creative Commons Attribution License (CC BY). The use, distribution or reproduction in other forums is permitted, provided the original author(s) or licensor are credited and that the original publication in this journal is cited, in accordance with accepted academic practice. No use, distribution or reproduction is permitted which does not comply with these terms.
* Correspondence:
Renhua Na, Inner Mongolia Agricultural University, Hohhot, China
Disclaimer: All claims expressed in this article are solely those of the authors and do not necessarily represent those of their affiliated organizations, or those of the publisher, the editors and the reviewers. Any product that may be evaluated in this article or claim that may be made by its manufacturer is not guaranteed or endorsed by the publisher.
Research integrity at Frontiers
Learn more about the work of our research integrity team to safeguard the quality of each article we publish.