- 1Centre for Translational Microbiome Research (CTMR), Department of Microbiology, Cell and Tumor Biology, Karolinska Institutet, Stockholm, Sweden
- 2Gedea Biotech, Medicon Village, Lund, Sweden
A healthy vaginal microbiome (VMB) is dominated by Lactobacillus spp. and provides the first line of defense against invading pathogens. Vaginal dysbiosis, characterized by the loss of Lactobacillus dominance and increase of microbial diversity, has been linked to an increased risk of adverse genital tract diseases, including bacterial vaginosis, aerobic vaginitis, vulvovaginal candidiasis, sexually transmitted infections, and pregnancy complications such as preterm birth. Currently, antibiotics and antifungals are recommended first-line treatments with high cure rates, but they also can lead to high recurrence and resistance development. As an alternative, lactobacilli have been utilized to restore the vaginal microbiota. In this review article, we discuss vaginal dysbiosis in various gynecological infections and potential interventions based on Live Biotherapeutic Products (LBPs) with a focus on those that use intravaginal treatment modalities to modulate the VMB. Based on these, we provide insights on key factors to consider in designing phenotypic and genotypic screens for selecting bacterial strains for use as vaginally administered microbiome-directed therapeutics. Lastly, to highlight current progress within this field, we provide an overview of LBPs currently being developed with published clinical trial completion for recurrent BV, VVC, and UTI. We also discuss regulatory challenges in the drug development process to harmonize future research efforts in VMB therapy.
Introduction
The vaginal microbiome (VMB) is composed of all the microorganisms inhabiting the vagina. The composition of the vaginal microbiome is dynamic, changing throughout a woman’s lifespan, with notable shifts occurring during hormonal changes, such as menstruation, pregnancy, and menopause. Ravel et al. (2011) performed one of the earliest in-depth analyses of the VMB in reproductive-aged women of varying ethnic groups using next-generation sequencing. They proposed a classification based on Community State Types (CSTs), where the CSTs and their subtypes describe the temporal changes in the composition of the VMB (Ravel et al., 2011; Holm et al., 2023). CSTs I, II, III, and V are characterized by the dominance of different Lactobacillus species, such as L. crispatus, L. gasseri, L. iners, and L. jensenii, respectively. Healthy reproductive-aged females are generally CST-I (L.crispatus-dominated) and CST III (L. iners-dominated). In contrast, CST IV and its subtypes characterize VMBs dominated by facultative anaerobes, such as Gardnerella vaginalis and Fannyhessea vaginae (formerly Atopobium vaginae), resulting in a high-diversity vaginal microbiome with a reduced abundance of lactobacilli. Current prospective studies on reproductive outcomes of young, healthy women confirm the CSTs classification (Haahr et al., 2016; Kroon et al., 2018). However, due to the dynamic nature of the human VMB and the complexity of the various state types, not all women neatly fit into each CST. A modular approach was proposed in a Belgian cohort describing the co-occurrence of bacterial taxa based on network correlation analysis (Lebeer et al., 2023), where common species associated with bacterial vaginosis (BV) and a healthy VMB can be present in asymptomatic women. In addition, some women, especially those of Black or Hispanic background, may have a natural VMB dominated by non-Lactobacillus species. As more research is performed on defining a healthy VMB, more detailed sub-types based on the VAginaL community state typE Nearest CentroId clAssifier (VALENCIA) have been proposed. VALENCIA separates CST III and CST IV groups based on the relative abundance of different bacterial species (France et al., 2020). In addition, metagenomic community state types (mgCSTs) enable the separation of the same species based on the assembled metagenomes and functional diversity (Holm et al., 2023). Nevertheless, in all these findings, it is agreeable that a healthy VMB contributing to positive reproductive outcomes harbors a low-diversity microbial community dominated by different members of the Lactobacillus genus.
Furthermore, as our understanding of the VMB increases, it has been determined that vaginal dysbiosis that progresses from CST IV and its subtypes render women more susceptible to other infection-related issues, including BV (France et al., 2020), aerobic vaginitis (Donders et al., 2017), and sexually transmitted diseases (Edwards et al., 2019; Cheng et al., 2020; Wang et al., 2023). Vaginal dysbiosis also potentially increases the risk of other gynecological conditions associated with adverse pregnancy outcomes, such as miscarriage and preterm birth (Grewal et al., 2022; Gudnadottir et al., 2022).
A deep understanding of the composition and function of the VMB and its interaction with pathogens and the host immune system is necessary for the development of novel treatment options for vaginal dysbiosis. Recently, live biotherapeutic products (LBPs) have emerged as a promising and innovative class of therapeutic agents with broad applications, including addressing issues related to the VMB. The United States Food and Drug Administration (FDA) defines LBPs as biological products containing living organisms, such as bacteria, designed to prevent, treat, and cure human diseases or conditions, excluding vaccines. We aim to highlight key factors that can be important to delineate between probiotics with limited or no therapeutic claims as compared to these LBPs designed as drugs by revisiting the currently known consequences of vaginal dysbiosis that need attention and discuss the recent advancements in the application of LBPs for common gynecological conditions. Further, we outline the desirable characteristics of LBPs in treating vaginal dysbiosis and describe the challenges linked to LBP development for vaginal health.
Vaginal dysbiosis and infectious causes of gynecological inflammation
In a healthy vagina, the vaginal microenvironment harbors immune cells, such as natural killer cells, macrophages, and dendritic cells, abundance of which increases during inflammation (Monin et al., 2020; Anahtar et al., 2015). Lactobacilli are crucial for maintaining the vaginal mucosal layer’s integrity as a physical defense against pathogens. However, vaginal dysbiosis occurs depending on both biological and behavioral factors. Sexual activity may be a primary cause of some of these infections. However, even sexually inactive individuals can experience dysbiosis in their lifetime due to the use of antibiotics, immunosuppression, low estrogen levels, or unsuitable hygiene habits (Figure 1). Vaginal dysbiosis is generally characterized by a long-term high-diversity state, where non-lactobacilli members of the vaginal community flourish. In a dysbiotic vaginal microbiome, increased microbial diversity, along with a decrease in beneficial lactobacilli, is accompanied by immunomodulatory changes that affect the natural barrier and contribute to further alterations in the microbiome, vaginal homeostasis, and host immunity.
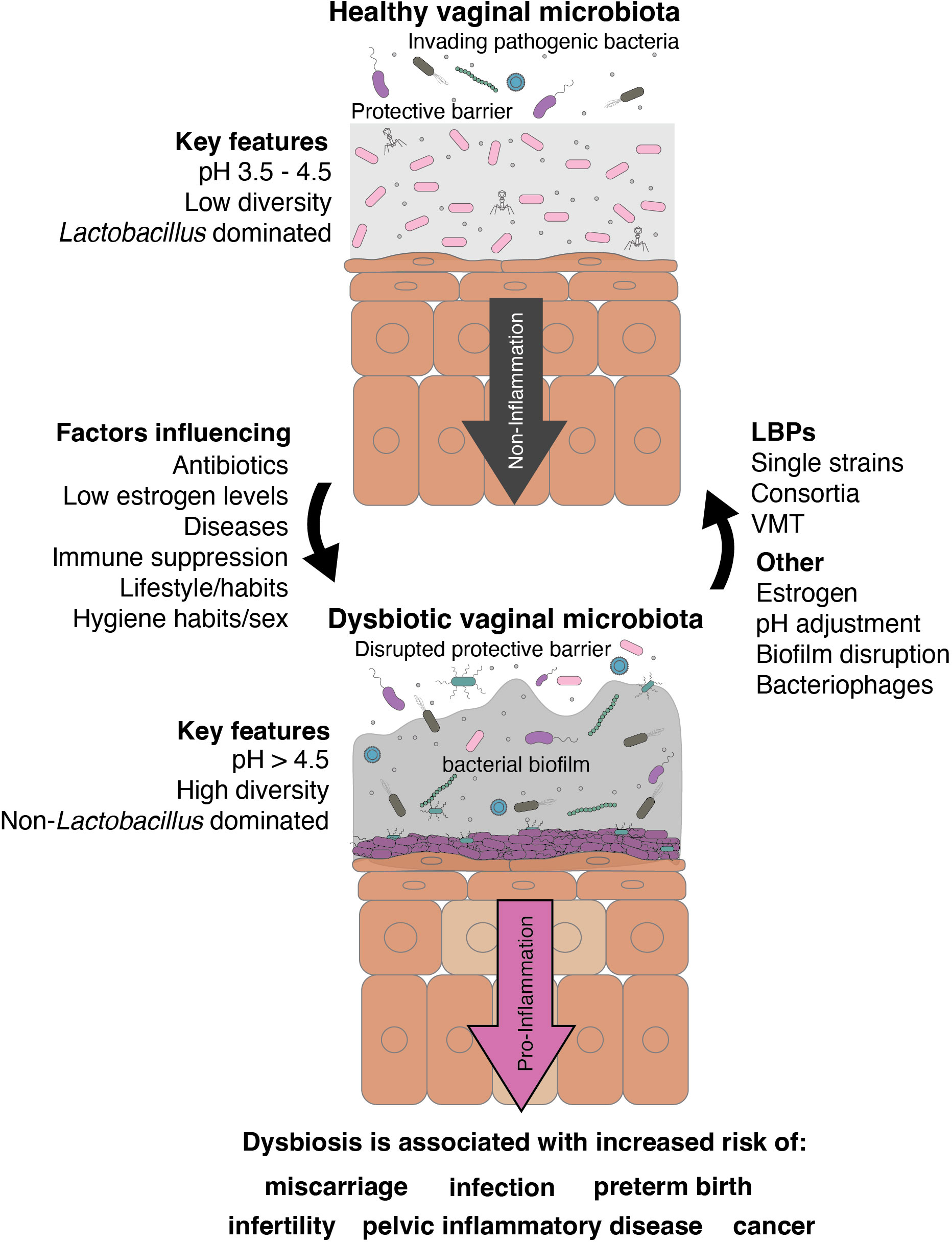
Figure 1. Graphical summary of the role of native lactobacilli in the vaginal tract and their potential role as LBPs in vaginal dysbiosis.
Bacterial vaginosis
The most common dysbiotic state in the vagina occurs with BV, especially in reproductive-aged women worldwide. Its risk for susceptibility to other obstetric and gynecological diseases, such as preterm birth and sexually transmitted infections (STIs), renders a high global economic burden fueling the impetus for screening and therapeutic studies (Peebles et al., 2019). Nugent scoring based on microscopic evaluation of Gram-stained vaginal smear samples provided standardized measures for assessing vaginal health (Nugent et al., 1991; Coleman and Gaydos, 2018). Although the Nugent scoring is proposed as a gold standard and allows comparability between ethnic groups, it requires time and expertise, for which the Amsel criteria is more preferred especially in resource-limited settings (Bhujel et al., 2021). Clinically, the Amsel criteria are more commonly used, where 3 out of the 4 conditions are met for a diagnosis: 1) thin, homogenous discharge; 2) pH of vaginal fluid above 4.5; 3) clue cells on microscopy (more than 20% of epithelial cells); 4) positive KOH test (Amsel et al., 1983; Donders, 2010). However, given the lower specificity and sensitivity of the Amsel criteria (37 to 70%) as compared to Nugent scoring (94-97%) (CDC, 2021), variations in clinical diagnosis can affect the understanding of the contribution of ethnic differences to the prevalence of the condition and the etiology of the disease relevant to the microbiome.
First-line treatment for BV is mainly clindamycin or metronidazole, with cure rates decreasing from >90% (Joesoef et al., 1999) to 50-80% (Eschenbach, 2007) in recent reports of known BV cases, accompanied by high recurrence rates (Bradshaw et al., 2006; Coudray and Madhivanan, 2020; Verwijs et al., 2020). The variability in response to antibiotic treatment may be due to differences in how efficient the inconsistency the BV-associated biofilm is disrupted (Bradshaw and Sobel, 2016; Wu et al., 2022; Sousa et al., 2023). Further, within biofilms, BV-associated bacteria may possess inherent antibiotic resistance mechanisms, such as pumping, chemical neutralization, and matrix barriers (Hardy et al., 2017; Nourbakhsh et al., 2022). This poor clearance can possibly lead to a high recurrence rate, where more than half of the women experience BV symptoms again within one year of the antibiotic treatment and likely enrichment of antibiotic-resistance genes in the vaginal environment (Beigi et al., 2004a; Bradshaw et al., 2006).
During BV, the vagina fosters a pro-inflammatory environment, which can be partly attributed to the immunomodulatory effects of the anaerobic bacteria and their metabolites. Notably, short-chain fatty acids (SCFAs) are elevated during BV and their abundance is associated with increased inflammation (Delgado-Diaz et al., 2020; Schwecht et al., 2023). The presence of SCFAs such as acetate, propionate, butyrate, and succinate combined with biogenic amine production in the absence of lactobacilli increases vaginal pH >4.5 (O’Hanlon et al., 2011), making it favorable for growing anaerobic bacteria that produce virulence factors that can degrade mucin, compromise the vaginal epithelial barrier integrity, and stimulate pro-inflammatory responses (Aldunate et al., 2015). Further, the recognition of bacterial cells by the toll-like receptors (TLRs) on the vaginal epithelial cells launches an inflammatory response (Aldunate et al., 2015; Delgado-Diaz et al., 2020). Aside from the production of amines, recent research found that specific amino acid metabolites secreted by BV-associated bacteria, such as imidazole propionate, can interfere with vaginal epithelial cell function and further contribute to inflammation via the mTOR signaling pathway (Berard et al., 2023).
Within the context of the vaginal microbiome, the molecular and pathophysiological mechanisms of BV development remain largely unknown. What is known so far is that women suffering from BV are characterized by a dysbiotic vaginal microbiome dominated by a diverse group of anaerobic bacteria, such as Gardnerella vaginalis, Fannyhessea vaginae, Prevotella bivia, and Sneathia amnii (Fredricks et al., 2005; Muzny et al., 2019; Carter et al., 2023). The VMB is also depleted of Lactobacillus spp., except for L. iners, often found adapting and coexisting with BV-associated bacteria (Zheng et al., 2021). G. vaginalis and Prevotella have been touted as primary instigators (Machado et al., 2013; Muzny et al., 2018, 2019; van Teijlingen et al., 2024) of BV and biofilms are formed with other important anaerobic bacteria during pathogenesis (Bradshaw and Sobel, 2016; Hardy et al., 2017). Due to the nature of the polymicrobial infection, metabolic environmental shifts lead to continued host inflammatory responses and disrupted epithelial cell barrier, which become further aggravated, leading to secondary infections and adverse gynecological disease sequelae.
Aerobic vaginitis
Aerobic vaginitis, due to its similarity to BV, can be misdiagnosed and incorrectly treated. It has been associated with complications such as vaginal inflammation, bacteriuria, lower urinary tract infections, and acute pyelonephritis (Kaambo et al., 2018). AV is characterized by an increase in enteric aerobic commensals not generally found in BV, such as group B Streptococcus agalactiae (GBS), Staphylococcus aureus, Escherichia coli, and enterococci, in the vaginal microbiome (Donders et al., 2017). Compared to the normal vaginal flora, these aerobic bacteria increase by 3- to 5-fold and are associated with vaginal mucosal inflammation due to the disruption of Lactobacillus dominance (Fan et al., 2013). The main symptoms are similar to those of BV but present as negative on the KOH test (Donders et al., 2015). Severe inflammation is apparent in vaginal smears, where an increase in intermediate and basal cells occurs. It is accompanied by increased turnover of the superficial epithelial cell layer and possibly epidermal desquamation, vaginal epithelial atrophy, small erosions, and ulceration (Petrova et al., 2015). Immune system dysregulation is the main factor contributing to AV pathogenesis (Oerlemans et al., 2020a). Similarly, reduced estrogen levels and localized immunity make AV prevalent in postmenopausal women (Stika, 2010). Pathogenic members of AV, such as GBS, are an essential focus, as they can occasionally cause morbidity in older adults, pregnant women, and patients with underlying medical conditions (Kwatra et al., 2014; Brigtsen et al., 2015). GBS colonization of pregnant women is considered a significant cause of severe neonatal infections, including neonatal sepsis, meningitis, and pneumonia (Krauss-Silva et al., 2014). In addition to GBS, Enterococcus faecalis has also been associated with preterm birth, low birth weight, and puerperal sepsis, resulting in significant maternal and neonatal morbidity and mortality (Cools et al., 2016; Kim et al., 2014).
As such, preferred treatments for AV are kanamycin and quinolones, which have intrinsic activity against disease-causing pathogenic bacteria while potentially minimizing interference with the vaginal microbiota (Tempera and Furneri, 2010). So far, the use of meclocycline and kanamycin for the treatment of AV allows a focus on Gram-negative bacilli rather than Gram-positive cocci, as these drugs are not absorbed and may leave extra vaginal lactobacilli even in patients with severe AV (Tempera and Furneri, 2010). Likewise, clindamycin for treating BV is also used for AV treatment due to its broad spectrum of activity against several aerobic Gram-positive coccal species; however, infection control with clindamycin is short-lived and may not cover all species associated with AV (Sousa et al., 2023). On the other hand, non-absorbable, broad-spectrum antibiotics such as carbapenems and clavulanic acid-beta-lactam combination (amoxiclav) are very effective for rapid, short-term improvement of severe symptoms from aerobic infections, especially deep cutaneous vulvovaginitis caused by GBS or methicillin-resistant S. aureus (MRSA) (Donders et al., 2015). Some beta-lactam antibiotics, however, are associated with less efficacy in eradicating E. coli vaginal colonization, leading to a high frequency of recurrent urinary tract infections (Stapleton, 2016). Nevertheless, due to significant inflammation in AV patients, including leukocyte and parabasal cell infiltration, frequent and prolonged use of antibiotics may result in adverse side effects. It may need to be accompanied by local administration of estrogens or a combination of probiotics and a very low dose of local estriol for postmenopausal or immunocompromised patients (Donders et al., 2015).
Vulvovaginal candidiasis
Like BV and AV, VVC is another significant public health concern. In the US alone, more than 70% of women experience fungal infection at least once in their life, with inflammation of the vagina and vulva as the primary concern (Nyirjesy et al., 2022). They are commonly colonized in the vagina by Candida albicans and non-albicans species such as C. glabrata, C. tropicalis, C. krusei, and C. parapsilosis. Other risk factors for VVC include antibiotic usage, lifestyle choices such as sexual activity, serum glucose levels in diabetic and pre-diabetic females, estrogen balance, immunoregulatory deficiencies, allergies, and gene polymorphisms (Satora et al., 2023). Thus, VVC classifications help to form treatment modalities based on uncomplicated and complicated VVC. Those with uncomplicated VVC are infrequent, with mild to moderate symptoms. Candida albicans is usually the main culprit in uncomplicated VVC. On the other hand, non-albicans candidiasis mainly occurs in those who are immunocompromised, leading to more severe and recurrent VVC (Nyirjesy et al., 2022).
Immunity against candidiasis is complex and involves multiple processes, including pathogen recognition through receptors such as Toll-like receptors (TLR) and C-type lectin receptors (CLR), active innate and adaptive immune cells such as macrophages, dendritic cells (DC), and T cells, as well as the production of cytokines and chemokines such as IL-4, IL-6, IL-10, IL-12, IFN-γ, and TNF-α (Netea et al., 2015; Pathakumari et al., 2020; Zhou et al., 2021a). A prospective study found higher serum levels of cytokines, including IL-4, IL-6, and IL-10, and lower levels of IFN-γ, TNF-α, and IL-17F in patients with recurrent VVC compared to those with VVC. This indicates that Th1/Th2 immunity may play an essential role in VVC (Ge et al., 2022). The host immune response against candidiasis has been comprehensively summarized in other reviews and will not be discussed in detail in this review (Netea et al., 2015; Pathakumari et al., 2020; Zhou et al., 2021a).
However, Candida spp. has evolved mechanisms for immune evasion and dissemination by which it can hijack macrophages and instigate hyphal escape via pyroptosis (Uwamahoro et al., 2014) and macrophage extracellular trap formation (ETosis) (Netea et al., 2015; Olivier et al., 2022). To escape immune detection, Candida spp. executes morphological switching from non-infectious yeast to hyphae inside the macrophages. It instigates hyphal escape by producing pore-forming proteins such as candidalysin (Olivier et al., 2022), leading to inflammation and macrophage rupture, consequently releasing the pathogen. Further, Candida spp. can neutralize acidic growth environments by releasing ammonia derived from the catabolism of amino acids that may promote evasion and survival, although regulated by mitochondrial respiration (Westman et al., 2018; Silao et al., 2024). Other non-canonical strategies promoting colonization and evasion include β-glucan masking, which is triggered by stressors, nutrients, antifungal drugs, and other factors such as nitrogen availability and quorum sensing molecules (Pradhan et al., 2019). Such adaptations may contribute to the possibility of recurrence aside from other factors that can promote or induce VVC.
Even in healthy females, Candida albicans can co-exist as commensal members of the Lactobacillus-dominated VMB. Vaginal lactobacilli are known to produce anti-Candida factors, specifically with growth inhibition and targeting of hyphal components. Nevertheless, it has been reported that candidiasis occurs more frequently with an L. iners-dominated microbiome (CST III) as compared to an L. crispatus-dominated flora (CST II) (Tortelli et al., 2020). L. crispatus can inhibit the growth of C. albicans through the production of lactic acid and other anti-Candida molecules (Jang et al., 2019) and has the potential to reduce hyphal morphogenesis (Wang et al., 2017). Lactobacilli can further inhibit C. albicans virulence by preventing fungal adhesion to vaginal epithelial cells and hindering biofilm formation (Takano et al., 2023). Candida has been found to co-occur with high abundance of lactobacilli and BV-associated bacteria (Liu et al., 2013). Candida colonization is more commonly asymptomatic and may not always progress to VVC (Tortelli et al., 2020). However, some studies have implicated lactobacilli-rich VMBs associated with symptomatic VVC (Beigi et al., 2004b; McClelland et al., 2009). Nevertheless, with focus on the species level of lactobacilli, it has been more linked to the presence of an L. iners-rich VMB than an L. crispatus-rich VMB (Tortelli et al., 2020). In this context, the exact mechanism leading to the switch between commensal and pathogenic forms of C. albicans is not fully understood, especially in the context of Lactobacillus-Candida interactions in the vaginal environment (Takano et al., 2023; Silao et al., 2024).
Azole antifungal agents are used as first-line treatment, leading to adverse consequences such as the rise in multidrug-resistant Candida spp. Thus, despite standard treatment, the high prevalence of VVC and yeast colonization still puts this high on the World Health Organization’s (WHO) “critical priority” group. A review of evidence conducted by Nyirjesy et al. (2022) identifies the need for novel treatment approaches for recurrent VVC where no alternative treatments, even with oral probiotics, have solid evidence for support yet. Nevertheless, oteseconazole, another antifungal drug that finished a phase 3 study, presents a new option for recurrent VVC with fewer adverse effects that was approved in the USA for women who are not of reproductive potential (Martens et al., 2022; Siddiqui et al., 2023).
Vaginal dysbiosis and the susceptibility to sexually transmitted diseases
A healthy, vaginal epithelium is usually highly protective against STIs caused by viruses such as HPV and HIV, parasites such as Trichomonas vaginalis, and bacteria such as Neisseria gonorrhoeae, Mycoplasma genitalium, and Chlamydia trachomatis. In the study of HIV infection, the vagina has been thought to be a markedly more effective barrier than the rectum. Many layers of stratum corneum cells are shed each day, thereby reducing the ability of pathogens to reach target cells deeper in the epithelium. Further, along with the inhibitory properties of the resident vaginal lactobacilli, epithelial and immune cells constitutively produce low levels of antimicrobial peptides (e.g., SLPI) and cytokines such as the anti-inflammatory IL-1RA that contribute to homeostasis (Cone, 2014; Muzny et al., 2020).
However, BV and microbial diversity can modify the risk of STIs via their interaction with mucosal immunity within the female genital tract and modification of its protective epithelial barrier (Velloza and Heffron, 2017). Dysbiosis-associated bacteria like Gardnerella spp. and Prevotella spp. can break down the mucosal barrier through sialidase production and host cell lysis, making women more susceptible to gynecological infections (France et al., 2022). In vivo, this microbial shift triggers a local immune response and inflammation, reflected in increased pro-inflammatory cytokine production of interleukin (IL)-1α, IL-1β, IL-6 and IL-8 in the vagina with BV-associated bacteria in CST IV such as P. amnii, Sneathia sp. and Mobiluncus mulieris as compared to Lactobacillus-dominated communities (Marconi et al., 2014; Anahtar et al., 2015). In vitro, cervical epithelial cells produce higher concentrations of IL-6 and IL-8 when co-cultured with G. vaginalis, P. bivia, and P. amnii compared to L. crispatus, whereas F. vaginae also elevates IL-6, IL-8, and TNF-α cytokines (Doerflinger et al., 2014).
Human immunodeficiency virus
BV contributes to the proinflammatory cytokines in the vaginal tract of HIV-infected women (Mitchell et al., 2008). However, with these inflammatory states and disrupted barriers, infectious particles such as HIV can traverse through the genital epithelium via tears in the squamous epithelium or transcytosis across the single cell layer of the endocervix, ultimately infecting underlying CD4+ target cells in the submucosa (Vyshenska et al., 2017). The net outcome of these interactions favors HIV infection and replication by attracting target cells, which will subsequently become infected and further propagate the infection.
Recent publications have demonstrated that women with non-Lactobacillus-dominant VMB were at a greater risk of HIV infection, where increased mucosal inflammation enhanced the rate of sexual transmission of HIV in the female genital tract (Vitali et al., 2017; Wessels et al., 2018; Wang et al., 2023). On the other hand, L. crispatus is associated with reduced inflammation due to an immunoregulatory environment, exclusion of BV-associated bacteria, and reduced HIV risk. However, L. iners has been associated with fewer immune benefits and lower HIV protection or exclusion of BV-associated bacteria, possibly providing a more intermediate transitional stage to dysbiosis (Armstrong and Kaul, 2021). It is possible that women can develop a persistent long-term state where BV is frequent and that this state is associated with more frequent HIV infection. BV is more prevalent and persistent among HIV-infected women, particularly among those who are immunocompromised (Jamieson et al., 2001; Schellenberg et al., 2012).
Human papillomavirus
A dysbiotic VMB poses similar risks for the increase in HPV infection and persistence (Mitra et al., 2016; Vyshenska et al., 2017; Muzny et al., 2020). CST-III (L. iners-dominated) and CST-IV (dominated by facultative anaerobes) are suggested to be risk factors for persistent HPV infection (Mei et al., 2022) due to low protective immunity with the loss of healthy vaginal lactobacilli. In addition, HPV proteins E6 and E7 enhance IL-10 expression and macrophage type 2 production (Kremleva and Sgibnev, 2016). Cytokines such as IL-6, IL-8 and TGFβ-1 could also potentially influence the growth of different vaginal microbes (Kremleva and Sgibnev, 2016).
During this time, strict anaerobic bacteria start to colonize the vagina and destroy the protective barrier of the cervical epithelium. This event promotes HPV entry of host cells, paving the way for subsequent integration of the HPV virus into the host DNA and accompanying cell division for virus replication. Nevertheless, a Lactobacillus-dominated VMB, especially with L. crispatus (Cheng et al., 2020), protects against HPV and prevents HPV persistence and subsequent disease development (Zhang et al., 2018; Muzny et al., 2020). In another study, an L. gasseri-dominated microflora (CST II) is also associated with faster HPV clearance (Mei et al., 2022). Without these protective mechanisms in place, the immune system cannot cope with the strong immunological pressure brought about by dysbiosis, allowing tumor evasion strategies that lead to cervical intraepithelial neoplasia or cervical cancer development in unresolved HPV infections (Piersma, 2011; Zhou et al., 2021b).
Trichomonas vaginalis infection
Trichomonas vaginalis infection is associated with an increased risk of HIV infection and cervical and prostate cancer (Dessì et al., 2019; Margarita et al., 2020). The vaginal microbiota also plays a role in mediating susceptibility to T. vaginalis parasite infection, increasing the risk of trichomoniasis among women with BV or a Nugent score > 3 (Hinderfeld et al., 2019; Balkus et al., 2014; Margarita et al., 2020). Here, the loss of lactobacilli leads to an increased pH, creating an environment more favorable for T. vaginalis growth and pathogenicity (Margarita et al., 2020). Studies have also demonstrated an association between prevalent trichomoniasis and detection of Prevotella and Sneathia (non-amnii) species. These bacterial species may mediate susceptibility by producing nicotinamide metabolites that promote T. vaginalis infection (Jarrett et al., 2019). In contrast, suppression of L. iners in the initial interaction with T. vaginalis occurs but shows the possibility of adapting and surviving after longer exposure to T. vaginalis (Chiu et al., 2021). According to this relationship, interventions that decrease BV incidence and promote eubiosis could potentially contribute to reductions in trichomoniasis incidence (Balkus et al., 2014).
Vaginal dysbiosis and its effect on adverse pregnancy outcomes, infertility, and IVF failure
Dysbiosis-induced cervicovaginal inflammation has been strongly linked to adverse pregnancy outcomes such as preterm birth (Fettweis et al., 2019). The most common infectious diseases correlated with pregnancy complications are BV, VVC, and AV (Gao et al., 2021). The mechanism for which VVC during pregnancy is still speculative (Bagga and Arora, 2020), but pregnancy-related factors such as elevated levels of estrogen and progesterone, glycogen deposition, low vaginal pH, and decreased immunity that affect the vaginal milieu remain risk factors for VVC (Tellapragada et al., 2017; Gao et al., 2021). This is observed in a prevalence study of 1119 pregnant women in different regions of the world, of which 30% is colonized by vaginal Candida based on culture (Disha and Haque, 2022). Despite the lack of precise mechanisms, the weakened immunity state of the female cervicovaginal tract during pregnancy remains conducive to Candida infection that can instigate prostaglandin release, leading to uterine contractions and premature birth. However, several large cohort studies and systematic reviews did not show any association between VVC and preterm birth (Schuster et al., 2020; Blomberg et al., 2023; Gigi et al., 2023).
AV, on the other hand, is characterized primarily by a high abundance of GBS and E. coli, which in severe cases may be accompanied by desquamative inflammatory vaginitis, is linked to pregnancy complications such as ascending chorioamnionitis, early membrane rupture, and preterm delivery (Donders et al., 2002, 2011; Ma et al., 2022). A significant reduction in the incidence of preterm birth has been shown in women treated with clindamycin for BV and AV (Lamont et al., 2003; Larsson et al., 2006). However, multiple large, randomized trials did not find reduced incidence of preterm birth after clindamycin treatment (Bellad et al., 2018; Subtil et al., 2018), but preterm birth rates were much higher in the participants who failed antibiotic treatment (McGregor et al., 1994; Kekki et al., 2001; Sabol et al., 2006). In these cases, antibiotic resistance is likely to occur, and the normal vaginal flora is not maintained. Therefore, the current consensus in the field is that there is no significant reduction in the risk for preterm birth with clindamycin therapy (McClure and Goldenberg, 2019; Smaill and Vazquez, 2019). However, the mixed results may still warrant further investigation. Disruption of the vaginal microbiota has been proven to affect the incidence of preterm birth (Jacobsson et al., 2002). More recent studies of the vaginal microbiome of pregnant women with whole genome shotgun sequencing also reveal the similarities of G. vaginalis to the Bifidobacterium genera. In contrast, an L. crispatus-dominated VMB was significantly correlated to full-term birth, along with L. gasseri and Bifidobacterium breve (Feehily et al., 2020). However, current evidence shows that the overgrowth of BV-associated bacteria such as G. vaginalis generates proteolytic enzymes such as sialic acidase and proline aminopeptidase to break down the protective components of the vaginal tract such as mucin and the vaginal secretions and the fetal membranes (Cauci et al., 2008). The loss of protection allows pathogenic adhesion to the vaginal mucosa and invasion of the uterus, whereas the affected elasticity of the fetal membranes potentially leads to early rupture (Gao et al., 2021). Further, the protective low pH in the vaginal microenvironment is disrupted due to the lack of lactobacilli metabolic activity and concurrent biogenic amine production by BV-associated bacteria, possibly allowing more favorable conditions for opportunistic bacteria and pathogens to grow (Nelson et al., 2015; Borgogna et al., 2021). The parasitic T. vaginalis infection occurs in these conditions in symbiotic interactions with Mycoplasma hominis, leading to severe complications such as preterm delivery and low birth weight (Dessì et al., 2019; Margarita et al., 2020).
Continued vaginal dysbiosis can also affect the local immune response, leading to preterm birth. Within the mucosal layer, secretory immunoglobulin A (sIgA) antibodies and immune cells such as macrophages, B and T lymphocytes are present (Gomez-Lopez et al., 2017). However, with the process of infection during upstream movement or within the disrupted amniotic cavity, bacterial endo- and exotoxins generated further aggravate the condition by the stimulation of increased levels of proinflammatory cytokines such as IL-1α, IL-1β and TNF-α (Bogavac et al., 2010; Gao et al., 2021). Such conditions could potentially dysregulate hormonal balance which trigger the body’s synthesis of prostaglandin and matrix metalloenzymes leading to early contractions and dilation of the cervix, and destruction of collagen matrices that maintain the fetal membranes (Bogavac et al., 2010; Triggs et al., 2020; Gao et al., 2021). Incidentally, one bacterium gaining notoriety in association with adverse pregnancy outcomes involves the opportunistic pathogen Fusobacterium nucleatum, as it has been detected in placental and fetal tissues in pregnancy complications involving both ruptured and intact fetal membranes (Han et al., 2010; Bohrer et al., 2012; Wang et al., 2013). Even though it is initially an oral and vaginal commensal, it has been shown to have invasive properties in murine models that bind and infect epithelial and endothelial cells of the fetal-placental units, with TLR4 inflammatory cascades that lead to poor outcomes such as preterm birth, chorioamnionitis, neonatal sepsis, stillbirth, and preeclampsia (Han et al., 2004; Vander Haar et al., 2018). Studies in mice also show potential glycan cross-feeding mechanisms between F. nucleatum and BV-associated bacteria, potentiating sialidase activities in the dysbiotic vaginal microbiome (Agarwal et al., 2020).
Likewise, BV-associated bacteria have been linked to increased susceptibility in acquiring more severe inflammatory infections from sexually transmitted infections affecting the upper female genital tract, including the cervix, uterus, fallopian tubes, and ovaries (Wiesenfeld et al., 2002; Edwards et al., 2019). BV-induced pelvic inflammatory disease is a risk factor for ectopic pregnancies, and chronic infections can severely affect the reproductive organs, resulting in infertility and low success rates with IVF treatment (Haahr et al., 2016). Thus, the endometrial microbiome became another center of focus. Although lower in biomass as compared to the vaginal microbiome, the commensal endometrial microbiome possibly supports the intricate balance that regulates embryo implantation, a natural inflammatory event, and pregnancy development (Odendaal et al., 2024). Despite the technical and sampling limitations, it is necessary to further understand the influence of the cervicovaginal microbiota and utilize restorative LBP therapies such as lactobacilli, to resolve such inflammatory infectious or even non-infectious gynecological conditions, and to improve maternal health by treating vaginal dysbiosis in reproductive-aged women (Haahr et al., 2022).
LBP mechanisms of action and screening for desired characteristics
Beneficial and antipathogenic effects of lactobacilli for LBP use are based on the traditional probiotic traits of lactobacilli such as in the production of lactic acid to maintain a low vaginal pH, production of antimicrobial compounds (i.e., bacteriocins, hydrogen peroxide) and stimulation of the immune system to help to maintain bacterial balance in the vaginal tract (López-Moreno and Aguilera, 2021). In addition, by adhering to the vaginal epithelia, vaginal lactobacilli may inhibit the attachment of pathogenic bacteria and utilize the same nutrients as pathogens, thereby restricting their growth (Lehtoranta et al., 2022). Given so, an understanding of the local vaginal Lactobacillus species and their niche provides insight into the selective criteria for LBP development (Figure 2).
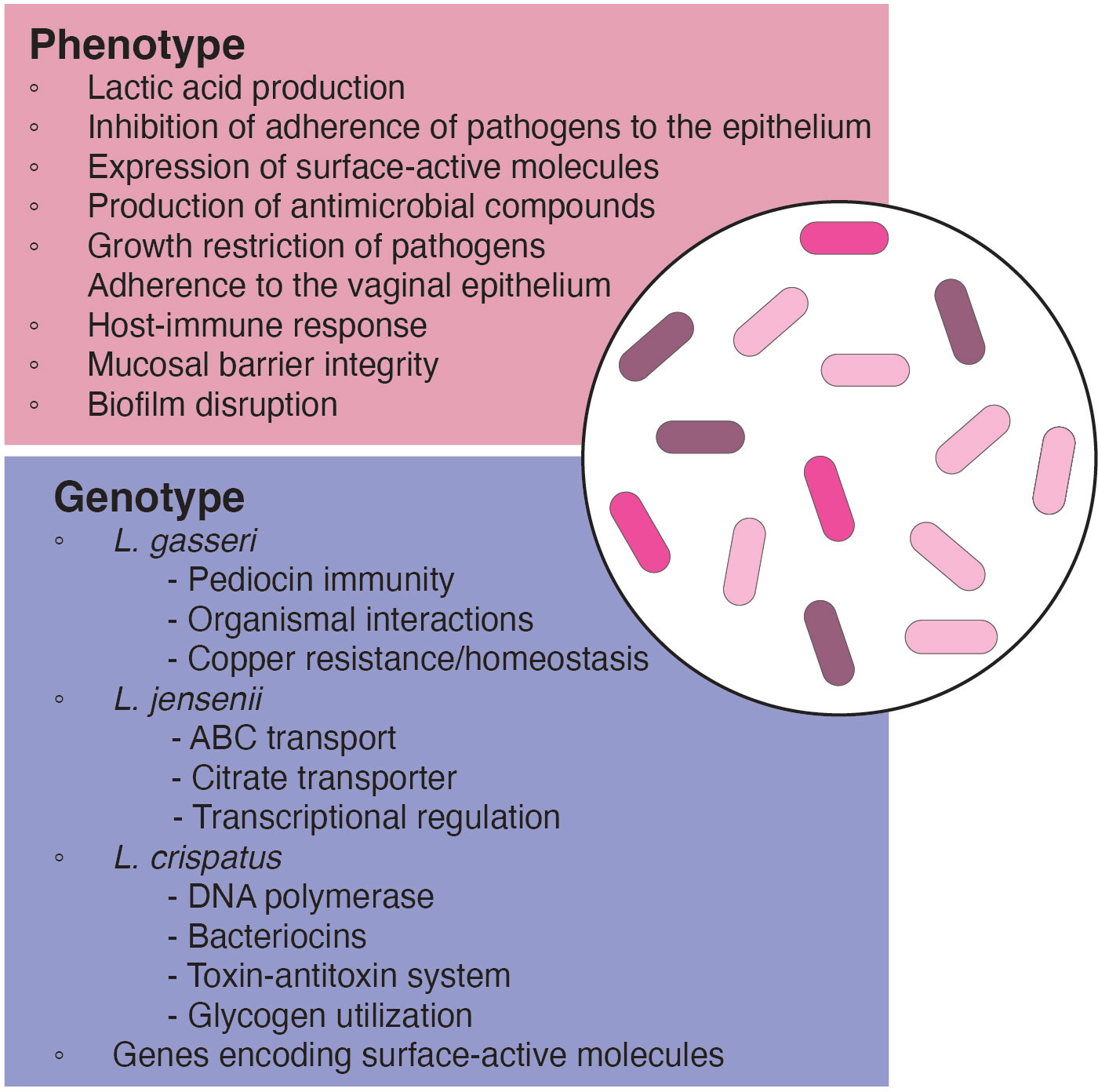
Figure 2. Phenotypic and genotypic characteristics of relevant species and strains for use in LBP development.
Ecological fitness, competence and growth
Lactobacilli display distinct strain differentiation in both genotypic and phenotypic characteristics within the same species. Within vaginal species, each genome encodes species-specific protein families. Among the most common vaginal lactobacilli—L. crispatus, L. gasseri, L. iners, and L. jensenii—L. iners exhibited the smallest average genome size at 1.3 Mbp, lacking integral membrane proteins, transcriptional regulators, acetyltransferase GNAT family members, and certain ABC transport proteins. However, they possessed unique ABC transporter permeases, thiol-activated cytolysin (inerolysin), and other protein families absent in other Lactobacillus species (Rampersaud et al., 2011; Macklaim et al., 2013). On the other hand, L. crispatus, known for its extensive genome size among vaginal lactobacilli, exhibited unique proteins such as DNA polymerase, bacteriocins, and toxin-antitoxin systems, absent in other vaginal strains. Generally, L. crispatus harbored all common protein families with the other Lactobacillus species, but no protein families were significantly more abundant in L. crispatus (Damelin et al., 2011). For L. gasseri, proteins related to copper resistance/homeostasis, toxin-antitoxin systems, and pediocin immunity were exclusive across all tested strains. In the case of L. jensenii, protein families involving families associated with ABC transport, transcriptional regulation, and a citrate transporter were unique. However, certain protein families such as the glucitol/sorbitol phosphotransferase system were present in other vaginal lactobacilli but were missing in L. jensenii (Merhej et al., 2009; Mendes-Soares et al., 2014).
Pan et al. (2020) also compared phenotypic assays of L. crispatus and L. gasseri in healthy vaginal microbiomes and human gastrointestinal tracts. Variations in the growth patterns and fermentative capacities are observed within the same species, where vaginal lactobacilli performed well in a simulated vaginal fluid, demonstrating higher lactic acid resistance at a later growth phase was necessary for the maintenance of low vaginal pH. Their findings underscored that the isolation source of a strain could influence its potential probiotic functionality, emphasizing its consideration in probiotic formulation (Pan et al., 2020). Similarly, clinical evidence shows that specific oral probiotic strains or their combinations may elevate vaginal lactobacilli counts in healthy women or women with BV and/or VVC and support natural VMB during/after recovery from antibiotics/antifungal treatment (De Alberti et al., 2015; Heczko et al., 2015; Mezzasalma et al., 2017). Nevertheless, current clinical research also shows that this has not always been the case in other BV, GBS infection and VVC cohorts, where there was no consistent improvement and vaginal colonization of orally administered probiotic strains (Hanson et al., 2014; Husain et al., 2020; Farr et al., 2020). As far, it seems that given the longer length, higher dosing, and necessity for lactobacilli to withstand gastric and bile acids for treatment via the oral route, the direct intravaginal route of antibiotic and LBP therapy is deemed more effective, relevant to the source of the strain.
Analysis of L. crispatus strains from various environments revealed genetic adaptations of strains to their ecological niches. Certain well-adapted metabolic and growth capacities to the vaginal tract can provide an advantageous basis for colonization and provide means for restoration of the natural vaginal lactobacilli. Notably, a vaginal isolate, L. crispatus PRL2021, demonstrates superior ecological competence in a simulated vaginal fluid compared to other vaginal Lactobacillus species, indicating its potential in higher growth performance in the vaginal microbiome and competitive mechanisms (Mancabelli et al., 2021). L. crispatus strains from Lactobacillus-dominated and dysbiotic vaginal microbiomes were also studied, but with no phenotypic distinction observed between strains, specifically with organic acid production (Abdelmaksoud et al., 2016; van der Veer et al., 2019). However, they observed a disproportionally higher abundance of gene fragments encoding for glycosyltransferases among strains isolated from dysbiotic microbiomes, suggesting a role for cell surface glycoconjugates in shaping vaginal microbiota-host interactions. Additionally, they noted the ability of L. crispatus to grow on glycogen, correlating with the presence of a full-length pullulanase type I gene (van der Veer et al., 2019). In all studies, glycogen utilization deemed favorable for selection as it is the most abundant carbohydrate source in the vaginal milieu, supporting both growth and lactic acid production.
Antimicrobial production
Lactic acid has both antimicrobial and immune modulatory functions. It does not only acidify the vaginal environment but is also associated with lower levels of pro-inflammatory cytokines (e.g., IL-1α, IL-6, IL-8, and TNFα), which help to generate a homeostatic environment (Aldunate et al., 2015). Several in vitro studies have demonstrated that lactic acid is a potential antiviral and bactericidal compound, inhibiting replication/growth of genital STI pathogens and pathobionts, including C. trachomatis, N. gonorrhoeae, GBS, HPV and HIV (Muzny et al., 2020). In this context, acidity and protonated lactic acid are responsible for the anti-trichomonadal effects of L. gasseri (Pradines et al., 2022) alongside other lactobacillar bacteriostatic and bactericidal compounds in the vagina (Brotman et al., 2012). Other antimicrobial compounds such as hydrogen peroxide-producing enzymes are common among vaginal L. crispatus strains (van der Veer et al., 2019). The rationality of hydrogen peroxide production as a significant factor in vaginal health has been debated due to the lack of oxygen in the healthy vaginal tract (Tachedjian et al., 2018), and that physiological concentrations of hydrogen peroxide (<100 uM) do not affect BV bacteria (O’Hanlon et al., 2011). Nevertheless, given certain physiological factors causing vaginal dysbiosis such as in AV and VVC, it may still be relevant as the natural vaginal strains still maintain the core functional genes. In this context, vaginal L. crispatus supernatants are strongly inhibiting of Candida albicans growth, virulence gene expression and hyphal formation (Wang et al., 2017). Furthermore, bacteriocins have also been found in certain vaginal lactobacilli. Lactocin 160 from a vaginal L. rhamnosus, induces transient pore formation via disruption of the chemiosmotic potential on the cytoplasmic membrane of G. vaginalis (Turovskiy et al., 2009), whereas Gassericin E from L. gasseri EV1461 affects both related and non-related strains, inhibiting the growth of BV pathogens (Maldonado-Barragán et al., 2016).
Modulation of host innate and adaptive immune responses
Further, LBP host colonization and the inhibition of pathogen adhesion are important selective traits for maintaining mucosal barrier integrity (Boris et al., 1998; Osset et al., 2001; Delgado-Diaz et al., 2022). Lactobacilli can induce host defense against pathogens through the formation of microcolonies that attach to epithelial cell receptors and form a physical barrier to pathogen attachment (Lebeer et al., 2010; Petrova et al., 2013). For instance, L. gasseri inhibits T. vaginalis colonization of vaginal epithelial cells through the action of its cells and a cell-surface aggregation-promoting factor (Pradines et al., 2022). Similarly, genomic studies of L. crispatus also found genes encoding fibronectin-binding L. crispatus protein (LACT01268) and L. crispatus adhesins (LACT01712 and LACT02327) that have been found to interfere with fibronectin-binding and pilus components of G. vaginalis (Koumans et al., 2007; Yeoman et al., 2010). One such fibronectin-binding protein encoded by L. crispatus the LEA protein, which can potentially exert inhibitory effects by competing with the same attachment sites as the pili of G. vaginalis (Edelman et al., 2012; Ojala et al., 2014). Core proteins of L. crispatus may play a crucial role in protecting the vagina from pathogens and bacterial vaginosis and highlight intricate mechanisms through which L. crispatus maintains vaginal health.
At the same time, potential LBPs demonstrate the ability to improve host innate immune responses. TLRs on vaginal epithelial cells regulate the production of cytokines by responding to molecular patterns (MAMPs) present on the bacterial surface. For instance, Gardnerella can induce an epithelial cell response through nuclear factor kappa B (NF-κB) activation via a TLR2-dependent signaling pathway (Anton et al., 2022). In AV, inflammatory responses can also be induced through TLR4 activation of NF-κB (Mirmonsef et al., 2011; Rose et al., 2012). Given this, TLR2/TLR6 modulation has been suggested to play an anti-inflammatory role in certain situations (Li et al., 2013). To ameliorate the low activation of TLR2/TLR6 typically observed in an inflammatory state, Lactobacillus-dominated commensal communities may be an important part of maintaining homeostasis and inducing an immunomodulatory role to reduce vaginal inflammation (Nasu and Narahara, 2010; Hearps et al., 2017). Surface active molecules such as bacterial exopolysaccharides (EPS) and peptidoglycan on the cell surfaces are also present in L. crispatus (Ojala et al., 2014; Chee et al., 2020). EPS can enhance the ability of vaginal VK2 cells in producing anti-candidal human defensin-2 protein, and likewise improving colonization potential (Donnarumma et al., 2014). In vitro evidence also shows the ability of the vaginal L. crispatus peptidoglycan to stimulate CD207 expression in Langerhans cells, the antigen presenting dendritic cells in vagina, to reduce HIV receptor entry (Song et al., 2018).
Lastly, it has been demonstrated that certain Lactobacillus species such as L. delbrueckii and L. rhamnosus taken as oral probiotics may dampen lipopolysaccharide (LPS)-induced expression of HLA-DR, CD86, CD80, CD83, and IL-12 in human dendritic cells (Esmaeili et al., 2018; Odendaal et al., 2024). Although it is not yet well understood, it may be a process through which the microbiota modulates immunotolerance in early pregnancy (Inversetti et al., 2023; Odendaal et al., 2024). Nevertheless, further investigation is needed to explore the mechanisms associated with local immunomodulation within the cervicovaginal microbiome, and their relevance to the implantation regulation and pregnancy development.
Currently available LBPs and ongoing developments
Traditional probiotics vs. LBPs
In the US, alternative treatment options are classified only as vaginal probiotics, due to the more straightforward track for getting them out to the market. Currently, there are seven different vaginal probiotics available for BV support. The AZO Complete Feminine Balance™ and Jarro-Dophilus® Women contain similar strains of L. crispatus LbV 88, L. jensenii LbV 116, L. gasseri LbV 150N, and L. rhamnosus LbV 96, which are a mix of the most common Lactobacillus spp. found in the vagina and has been documented to have increased recovery rates from BV (Laue et al., 2018). On the other hand, Bio-K+® Women’s Health, Jarrow Formulas® Fem-Dophilus® 1 Billion, Jarrow Formulas® Fem-Dophilus® 5 Billion, RepHresh™ Pro-B™ Probiotic, and UltraFlora® Women’s utilize strains isolated from the urogenital tract, such as L. reuteri RC-14 and L. rhamnosus GR-1, which did not improve BV cure rates as a dietary supplement, but can contribute to improved vaginal flora composition (Hummelen et al., 2010).
Moving forward, it is crucial to consistently differentiate between using Lactobacillus strains as a traditional probiotic and its use as an LBP. More relevant regulatory functions and expectations are necessary for the drug development track, considering that the target populations are diseased individuals who may be immune-sensitive (Cordaillat-Simmons et al., 2020). As far, Lactobacillus bacteremia is rare and mainly correlated to intestinal translocation in severely immunocompromised patients (i.e. uncontrolled diabetes mellitus, malignancy or have undergone organ transplantation), urogenital pathologies, congenital and valvular heart diseases or those who have undergone invasive procedures, that may be more susceptible to probiotic use (Salminen et al., 2004; Rossi et al., 2022; Salminen et al., 2006; Bapna et al., 2023; Kullar et al., 2023). There are currently no clinical reports of Lactobacillus bacteremia with intravaginally administered LBP strains, but vaginal lactobacilli, specifically L. jensenii, has been reported in polymicrobial bacteremia with Veillonella montpellierensis during pregnancy in an immunocompetent but anemic patient at 33-weeks of gestation (Toprak et al., 2022). Another recent case involves L. jensenii and P. bivia polymicrobial bacteremia leading to renal and perinephric abscesses with an otherwise immunocompetent patient but has undergone ureteral stent procedure (Mohan et al., 2020). Other case reports of L. jensenii bacteremia within obstetrics and gynecology that have occurred after vaginal delivery (Marciniak et al., 2014) and elective abortion using dilatation and curettage (Suárez-García et al., 2012) with patients who presented with infective endocarditis.
Nevertheless, the use of lactobacilli as a biotherapeutic drug is still justifiable due to its lower risk of use as a “generally regarded as safe” (GRAS) microorganism and its positive benefit-risk ratio compared to antibiotic usage in patients suffering from recurrent vaginal infections. Likewise, such side effects and contraindications can be averted with a well-characterized LBP strain lacking pathogenic factors and a defined antimicrobial sensitivity spectrum. These LBP candidates should be further explored within the ongoing clinical trials. Nevertheless, same as other drugs, high doses should also be used with caution, and monitored carefully by the administering physician along with any presenting underlying illnesses.
Thus, vaginal lactobacilli developed as intravaginally administered LBPs are meant to undergo further trait selection and safety testing, including considerations of the proper dosing, delivery form and optimal administration frequency and route, therapeutic indications, and expected side effects. Further, strain-specific properties and treatments prior to LBP administration is crucial (Wu et al., 2022). Based on this, various treatment modalities have been considered, such as the use of single lactobacilli strains, multi-strain lactobacilli combinations, and vaginal microbiome transplantation (VMT), with varying levels of efficacy specifically against BV, with or without antibiotic therapy prior to LBP treatment.
Single strain LBPs vs. multi-strain LBPs in recurrent BV and VVC
Substantial research has explored LBPs for treating recurrent BV and VVC, but they are not yet a standard clinical approach due to differences in trial size, methods, and uses. Recurrent BV has been the most impactful in the health and economic sectors, especially with its associations with diseases such as HPV, HIV and Trichomonas infection. Thus, clinical trials utilizing strains native to vaginal microbiome are on their way for recurrent infections. Those with clinical trial IDs, are intravaginally administered, and have published results are summarized in Table 1.
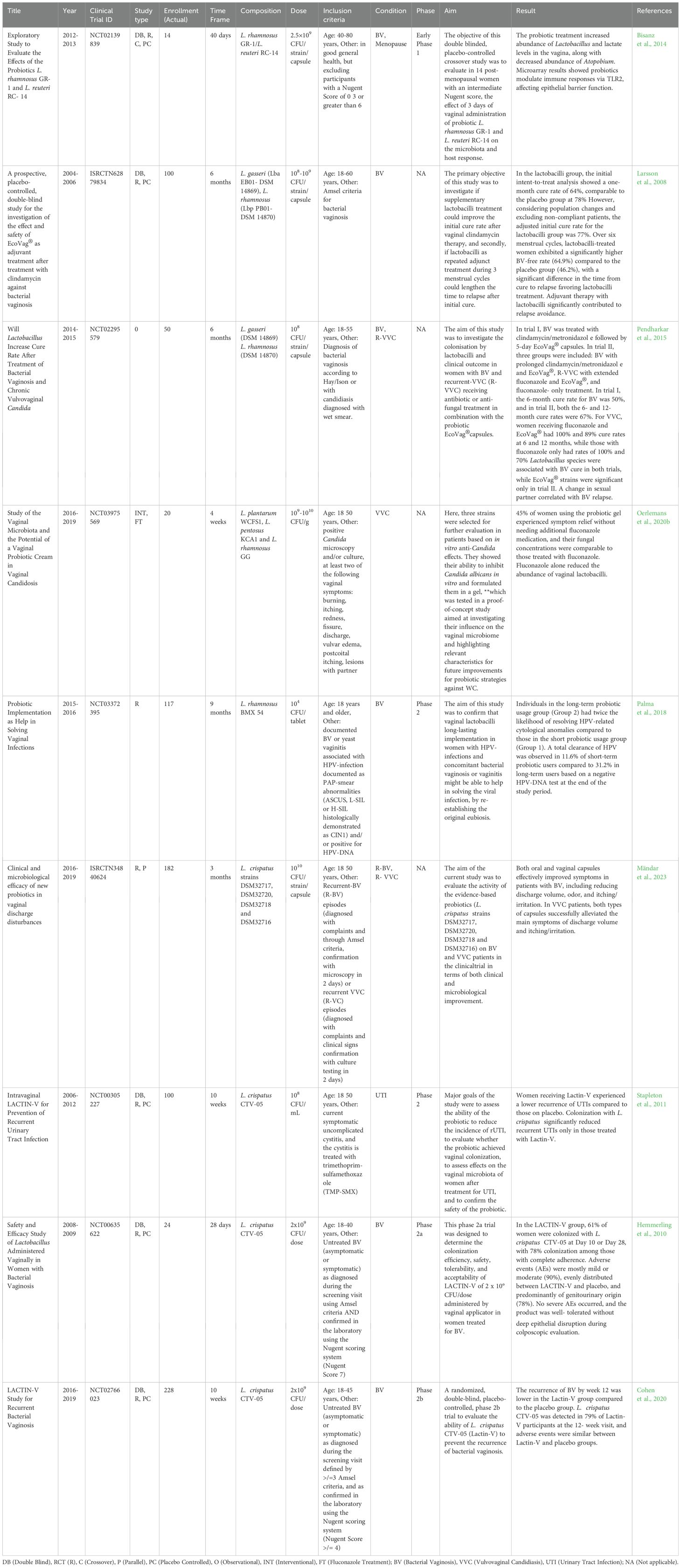
Table 1. List of published clinical trials of intravaginally-administered adjuvant probiotic therapy and LBP development.
Early phase 1 trials have shown that vaginal administration of L. rhamnosus GR1 and L. reuteri RC-14 can increase vaginal Lactobacillus and modulate the immune response via TLR2 (Bisanz et al., 2014). EcoVag® with L. gasseri DSM 14869 and L. rhamnosus DSM 14870 showed possibility for adjunct therapy during three menstrual cycles, demonstrating a higher BV-free rate (64.90%) in the lactobacilli group compared to placebo group (46.2%) (Larsson et al., 2008). EcoVag® also shows strong potential in recurrent VVC alongside fluconazole treatment, but with only a 6- or 12-month cure rate of 50 and 67%, respectively, alongside clindamycin/metronidazole treatment for BV, respectively (Pendharkar et al., 2015). L. plantarum WCFS1, L. pentosus KCA1 and L. rhamnosus GG administered as a probiotic gel against VVC also achieved 45% symptom relief in their interventional therapy (Oerlemans et al., 2020b). Lastly in a phase 2 trial, L. rhamnosus BMX54 showed long-term use benefits for HPV clearance, in the context of vaginal dysbiosis (Palma et al., 2018).
As far, it is still debatable if the effect may be dependent on Lactobacillus species or their natural ecological niche, as similar reference strain standards have been utilized. However, given that most studies are interventional with BV recurrence, larger clinical cohorts are still lacking. Further, long-term effects are only found in a few of these studies, and few have been done without the use of antibiotics; thus, the effect on varying vaginal microbiomes, retention, and mechanisms of action of these probiotic strains developed as potential LBP candidates may still need elucidation as the LBP drug functionality is still lacking in most studies.
Nevertheless, it has generally been accepted that native strains are more competitive and well-adapted to their ecological niche, such as in the use of glycogen in the vaginal microenvironment as a carbon source. Most lactobacilli are not capable of utilizing glycogen directly, but native vaginal strains have a generally efficient acquisition of glycogen-derived resources released by α-amylase production that break down glycogen in the vaginal tract (Nunn and Forney, 2016). Notably, LBPs containing native vaginal microbiome strains also stand out for their potential benefits in biofilm disruption and immunomodulation (Łaniewski and Herbst-Kralovetz, 2021). Exciting new prospectives for using natural, non-antibiotic pre-treatment alternatives, potentially in combination with LBPs, lie ahead (Latham-Cork et al., 2021).
Extensive research highlights L. crispatus as advantageous, providing protection against various pathogens and correlating with positive health and pregnancy outcomes (Ghartey et al., 2014; Argentini et al., 2022; Baud et al., 2023). Furthermore, compared to other dominant lactobacilli strains, pangenomic gene analyses have shown greater enrichment and evolutionary acquisition of key beneficial and protective functional genes (Bhattacharya et al., 2023). Moreover, although bacteriocin and organic acid biosynthesis are still strain-specific, L. crispatus seem to more ubiquitously carry key functionalities as compared to the heterogeneity found in either L. iners, L. gasseri or L. jensenii (Bhattacharya et al., 2023).
L. crispatus strains DSM32717, DSM32720, DSM32718 and DSM32716 which come from a collection of reproductive tract microorganisms, have been phenotyped and observed to be effective in reducing BV and VVC symptoms using both oral and vaginal capsules (Mändar et al., 2023). However, as far, Lactin-V based on the single strain L. crispatus CTV-05, has been the only one that has reached clinical phase 3, recently showing relative success in their clinical phase 2 trials for recurrent urinary tract infections in non-pregnant women (Stapleton et al., 2011), and in their phase 2a, and phase 2b trials for recurrent BV applications (Hemmerling et al., 2010; Cohen et al., 2020). In the studies, a 78% colonization rate in women treated for BV, and a lower BV recurrence after 12 weeks with Lactin-V has been demonstrated (Hemmerling et al., 2010; Cohen et al., 2020). Furthermore, Lactin-V has recently shown to be generally safe and well tolerated in pregnant women (Bayar et al., 2023), and is currently being investigated for use in reducing pre-term birth in women at high risk and enhancing in vitro fertilization success rates.
Vaginal microbiome transplantation
Aside from Lactin-V, exploratory studies using VMT have been performed for intractable and recurrent bacterial vaginosis in five women, with four achieving full long-term remission without adverse outcomes, despite a repeat VMT for three (Lev-Sagie et al., 2019). This provides promising results and opens more research opportunities based on VMT. In line with this, Freya Biosciences has started exciting work in LBPs based on VMT to improve reproductive outcomes. Two proof-of-concept intervention studies with their FB101 product have been completed, with published results in a patient presenting with severe vaginal dysbiosis and a history of recurrent pregnancy loss (Wrønding et al., 2023). In this antibiotic-free VMT approach, confirming donor strain engraftment followed by a successful pregnancy and delivery of the patient’s vaginal microbiome from 90% G. vaginalis to 81.2% L. crispatus and 9% L. jensenii with just one VMT treatment. It remains, however, that post-VMT treatment, the patient received 75mg daily of acetylsalicylic-acid and low-molecular-weight heparin to resolve antiphospholipid syndrome that can have contributed to the successful pregnancy (Wrønding et al., 2023). The study demonstrates how VMT can be further utilized in other gynecological conditions resulting from abnormal vaginal microbiota and improving reproductive health outcomes. Large-scale randomized, placebo-controlled clinical studies are ongoing, but no results have been published.
The future applications of vaginal LBPs in influencing the cervical and endometrial microbiota have become a major focus in maternal and fetal health. Further understanding of the cervicovaginal microbiota can assist in guiding the treatment of vaginal dysbiosis relevant to endometrial function to improve reproductive health outcomes (Odendaal et al., 2024).
Regulatory pathways and challenges in the development of vaginally administered LBPs
The microbiome field has dramatically matured over the past decade, but the development and regulatory approval of life biopharmaceutical products pose several challenges, given the complexity of these live products. However, advancements like the approval of LBPs for preventing CDI recurrence (Mullard, 2022) marked a pivotal milestone that remains to be expanded to other indications.
In 2012, the Food and Drug Administration (FDA) was the first regulatory agency to create the category of LBP and to publish a guideline for industry stakeholders entitled “Early Clinical Trials with Live Biotherapeutic Products: Chemistry, Manufacturing, and Control Information” and recently updated in 2016 (FDA (Food and Drug Administration), 2016). The European Directorate for the Quality of Medicines and Healthcare (EDQM) accepted this new category of medical products on the European market in 2019 entitled “EDQM (European Pharmacopoeia) 3053E General Monograph on Live Biotherapeutic Products” (EDQM, 2019). However, LBPs currently have no separate status in the European regulatory framework. Therefore, developers in the EU need to rely on relevant regulatory concepts for biological medicinal products, but with guidelines from the European Medicines Agency based on information from the International Council for Harmonisation of Technical Requirements for Pharmaceuticals for Human Use (ICH) regarding “ICH guideline E8 on General Considerations for Clinical Studies” in 2021 (European Medicines Agency, 2021) and the Committee for Medicinal Products for Human Use (CHMP) for “CHMP guideline on Human Cell-Based Medicinal Products” for scientific guidelines in addressing development, manufacturing and quality control (European Medicines Agency, 2008) and a revised guideline on “First-in-Human Clinical Trials with Investigational Medicinal Products” (European Medicines Agency, 2017). All these regulations allow for comprehensive risk analysis and mitigation strategies, specifically with their intended use (Rouanet et al., 2020).
LBPs, such as individual strains of bacteria, defined consortia, or VMTs, involve intricate biological processes that are not fully known. Regulatory agencies must assess the complex mechanisms of action on both the human host and host microbiome, potential side effects, and long-term safety. The proposed mechanisms of action are generally to interfere with the growth of a pathogenic or potentially pathogenic microorganism in the vagina or to stimulate other potentially beneficial cellular processes because of transient persistence and/or long-term colonization with the microorganisms contained in the LBP (Wu et al., 2022; Lagenaur et al., 2021). Investigational objectives vary concerning prevention versus treatment and stand-alone therapy versus adjunct therapy to antimicrobial or other therapy. With this regard, newly developed and emerging in vitro and in vivo tools are becoming more available to support LBP risk documentation before early clinical trials. However, there are still challenges regarding quality control and manufacturing of LBP, as ensuring consistent quality and manufacturing processes for biopharmaceuticals is complex. The biological nature of these products potentially has unknown inherent risks and can lead to manufacturing variability. Thus, maintaining product integrity during large-scale production is one of the significant obstacles to overcome.
The early clinical phases are designed to determine tolerability, dosage range, and administration regimen, which depend on the formulation and delivery route. As of current, designing an effective dosage form and delivery system for vaginal administration poses challenges. The dosage form should provide a controlled release and adhere to the vaginal mucosa for optimal absorption. Safety and tolerability studies need to consider the unique environment of the vagina regarding potential irritation, allergic reactions, or other adverse effects. The potential for immune responses to LBP can impact both safety and efficacy, so when appropriate, an “optimal effective dose” and a “safe maximal dose” are necessary to determine the points at which the intended effect is achieved with no or acceptable adverse effects. Thus, developers must assess and manage immunogenicity risks through appropriate study designs and monitoring strategies in the target populations.
Intended study populations vary from healthy individuals who may be at risk for specific diseases, such as infertility or pregnancy complications, to individuals severely afflicted with vaginal infections or dysplasia. Given this, designing appropriate clinical trials for live biopharmaceuticals can be challenging due to unique patient populations, limited historical data, and the need for sensitive endpoints. Likewise, target populations may require repeat treatment or longer-term usage of the LBPs, where biobanking of samples from consenting participants in the different trials and clinical phases may also be necessary (Rouanet et al., 2020). Thus, rigorous, and well-designed clinical trials are crucial for demonstrating safety and efficacy, where confounding factors influential to the data should also be considered. In addition, patient knowledge, acceptance, and adherence to vaginally administered treatments may be influenced by cultural, social, and individual factors and norms. Ensuring user-friendly, comfortable, and discreet administration methods is crucial.
Finally, the rapid advancement of biopharmaceutical technologies may outpace the development of regulatory science. Addressing scientific gaps and uncertainties in evaluating these products is an ongoing challenge for regulatory agencies. Furthermore, LBP developers often seek global approval, requiring coordination with multiple regulatory agencies. Achieving global harmonization in regulatory requirements and standards can be difficult due to varying regional expectations. Addressing these challenges requires collaboration between regulators, industry stakeholders, and researchers. Ongoing dialogue, transparency, and a proactive approach to adapting regulations to scientific advancements are essential for the successful development, approval, and access to LBPs.
Conclusion and future directions
Understanding the mechanisms by which the vaginal microbiota can influence the progression of gynecological and obstetrical disorders will lead to the development of personalized approaches to shape the microbiota composition and function, aiding prevention, and treatment of diseases. High-quality studies, including functional genomics, are needed to define the composition of the vaginal microbiota and its mechanistic involvement to the different conditions. This includes a detailed mapping of the ecology of the vaginal microbiota in health and disease to understand the interactions between microbes, pathogens and potential treatments based on live biopharmaceuticals. Furthermore, the complex interplay between the human host and vaginal microbes requires a deeper understanding for successful development of vaginal microbiome-directed therapies.
Regulatory approval of VMT, isolated bacterial strains, or defined consortia of strains will depend on the documentation and demonstration of quality, safety, and efficacy to allow for an assessment of the benefit-risk ratio for an intended use. More refined clinical trials with larger cohorts, defined uses, standardized trials, and longer-term follow-ups will also be necessary for maturing the field and the pioneer LBPs in development. The registration of LBPs as drugs will require a close dialogue between developers and regulatory agencies as the regulatory requirements are not fully defined yet. With this regard, accurate use of terminologies can significantly improve the process.
Author contributions
VV: Conceptualization, Writing – original draft, Writing – review & editing, Investigation. EL: Writing – original draft, Investigation, Writing – review & editing. IH: Writing – review & editing, Investigation, Writing – original draft. YZ: Writing – review & editing, Writing – original draft. JD: Writing – review & editing, Conceptualization, Investigation, Writing – original draft. IS: Supervision, Project administration, Conceptualization, Investigation, Writing – review & editing, Writing – original draft.
Funding
The author(s) declare financial support was received for the research, authorship, and/or publication of this article. Ferring Pharmaceuticals supports this research by a research collaboration with Karolinska Institutet. The funder was not involved in the study design, collection, analysis, interpretation of data, the writing of this article, or the decision to submit it for publication.
Acknowledgments
The authors would like to thank Laura Poole for the help in the preparation of the figures for this manuscript.
Conflict of interest
The authors declare that the research was conducted in the absence of any commercial or financial relationships that could be construed as a potential conflict of interest.
The author(s) declared that they were an editorial board member of Frontiers, at the time of submission. This had no impact on the peer review process and the final decision.
Publisher’s note
All claims expressed in this article are solely those of the authors and do not necessarily represent those of their affiliated organizations, or those of the publisher, the editors and the reviewers. Any product that may be evaluated in this article, or claim that may be made by its manufacturer, is not guaranteed or endorsed by the publisher.
References
Abdelmaksoud A. A., Koparde V. N., Sheth N. U., Serrano M. G., Glascock A. L., Fettweis J. M., et al. (2016). Comparison of Lactobacillus crispatus isolates from Lactobacillus-dominated vaginal microbiomes with isolates from microbiomes containing bacterial vaginosis-associated bacteria. Microbiol. (Reading) 162, 466–475. doi: 10.1099/mic.0.000238
Agarwal K., Robinson L. S., Aggarwal S., Foster L. R., Hernandez-Leyva A., Lin H., et al. (2020). Glycan cross-feeding supports mutualism between Fusobacterium and the vaginal microbiota. PloS Biol. 18, e3000788. doi: 10.1371/journal.pbio.3000788
Aldunate M., Srbinovski D., Hearps A. C., Latham C. F., Ramsland P. A., Gugasyan R., et al. (2015). Antimicrobial and immune modulatory effects of lactic acid and short chain fatty acids produced by vaginal microbiota associated with eubiosis and bacterial vaginosis. Front. Physiol. 6. doi: 10.3389/fphys.2015.00164
Amsel R., Totten P. A., Spiegel C. A., Chen K. C., Eschenbach D., Holmes K. K. (1983). Nonspecific vaginitis. Diagnostic criteria and microbial and epidemiologic associations. Am. J. Med. 74, 14–22. doi: 10.1016/0002-9343(83)91112-9
Anahtar M. N., Byrne E. H., Doherty K. E., Bowman B. A., Yamamoto H. S., Soumillon M., et al. (2015). Cervicovaginal bacteria are a major modulator of host inflammatory responses in the female genital tract. Immunity 42, 965–976. doi: 10.1016/j.immuni.2015.04.019
Anton L., Ferguson B., Friedman E. S., Gerson K. D., Brown A. G., Elovitz M. A. (2022). Gardnerella vaginalis alters cervicovaginal epithelial cell function through microbe-specific immune responses. Microbiome 10, 119. doi: 10.1186/s40168-022-01317-9
Argentini C., Fontana F., Alessandri G., Lugli G. A., Mancabelli L., Ossiprandi M. C., et al. (2022). Evaluation of modulatory activities of Lactobacillus crispatus strains in the context of the vaginal microbiota. Microbiol. spectr. 10, e0273321. doi: 10.1128/spectrum.02733-21
Armstrong E., Kaul R. (2021). Beyond bacterial vaginosis: vaginal lactobacilli and HIV risk. Microbiome 9, 1. doi: 10.1186/s40168-021-01183-x
Bagga R., Arora P. (2020). Genital Micro-Organisms in Pregnancy. Front. Public Health 8, 225. doi: 10.3389/fpubh.2020.00225
Balkus J. E., Richardson B. A., Rabe L. K., Taha T. E., Mgodi N., Kasaro M. P., et al. (2014). Bacterial vaginosis and the risk of Trichomonas vaginalis acquisition among HIV-1-negative women. Sex Transm. Dis. 41, 123–128. doi: 10.1097/OLQ.0000000000000075
Bapna M., Maurer J., Ruddy S., Karnik K., Turett G., Urban C., et al. (2023). A case of Lactobacillus jensenii associated native valve endocarditis. IDCases 32, e01806. doi: 10.1016/j.idcr.2023.e01806
Baud A., Hillion K. H., Plainvert C., Tessier V., Tazi A., Mandelbrot L., et al. (2023). Microbial diversity in the vaginal microbiota and its link to pregnancy outcomes. Sci. Rep. 13, 1. doi: 10.1038/s41598-023-36126-z
Bayar E., MacIntyre D. A., Sykes L., Mountain K., Parks T. P., Lee P. P., et al. (2023). Safety, tolerability, and acceptability of Lactobacillus crispatus CTV-05 (LACTIN-V) in pregnant women at high-risk of preterm birth. Benef. Microbes 14, 45–55. doi: 10.3920/BM2022.0084
Beigi R. H., Austin M. N., Meyn L. A., Krohn M. A., Hillier S. L. (2004a). Antimicrobial resistance associated with the treatment of bacterial vaginosis. Am. J. Obstet. Gynecol. 191, 1124–1129. doi: 10.1016/j.ajog.2004.05.033
Beigi R. H., Meyn L. A., Moore D. M., Krohn M. A., Hillier S. L. (2004b). Vaginal yeast colonization in nonpregnant women: a longitudinal study. Obstet- Gynecol. Open Access 104, 926–930. doi: 10.1097/01.AOG.0000140687.51048.73
Bellad M. B., Hoffman M. K., Mallapur A. A., Charantimath U. S., Katageri G. M., Ganachari M. S. (2018). Clindamycin to reduce preterm birth in a low resource setting: a randomised placebo-controlled clinical trial. BJOG. 1601–1609. doi: 10.1111/1471-0528.15290
Bhujel R., Mishra S. K., Yadav S. K., Bista K. D., Parajuli K. (2021). Comparative study of Amsel's criteria and Nugent scoring for diagnosis of bacterial vaginosis in a tertiary care hospital, Nepal. BMC Infect. Dis. 21 (1), 825. doi: 10.1186/s12879-021-06562-1
Berard A. R., Brubaker D. K., Birse K., Lamont A., Mackelprang R. D., Noël-Romas L., et al. (2023). Vaginal epithelial dysfunction is mediated by the microbiome, metabolome, and mTOR signaling. Cell Rep. 42, e112474. doi: 10.1016/j.celrep.2023.112474
Bhattacharya A., Das S., Bhattacharjee M. J., Mukherjee A. K., Khan M. R. (2023). Comparative pangenomic analysis of predominant human vaginal lactobacilli strains towards population-specific adaptation: understanding the role in sustaining a balanced and healthy vaginal microenvironment. BMC Genomics 24, 565. doi: 10.1186/s12864-023-09665-y
Bisanz J. E., Seney S., McMillan A., Vongsa R., Koenig D., Wong L., et al. (2014). A systems biology approach investigating the effect of probiotics on the vaginal microbiome and host responses in a double blind, placebo-controlled clinical trial of post-menopausal women. PloS One 9, e104511. doi: 10.1371/journal.pone.0104511
Blomberg L., Backman K., Kirjavainen P. V., Karvonen A. M., Harju M., Keski-Nisula L. (2023). Vulvovaginal yeast infections, gestational diabetes and pregnancy outcome. BMC Pregnancy Childbirth 23, 70. doi: 10.1186/s12884-023-05391-1
Bogavac M., Brkić S., Simin N., Grujić Z., Bozin B. (2010). Do bacterial vaginosis and chlamydial infection affect serum cytokine level? Srp. Arh. Celok. Lek. 138, 444–448. doi: 10.2298/SARH1008444B
Bohrer J. C., Kamemoto L. E., Almeida P. G., Ogasawara K. K. (2012). Acute chorioamnionitis at term caused by the oral pathogen Fusobacterium nucleatum. Hawaii J. Med. Public Health 71, 280–281.
Borgogna J. C., Shardell M. D., Grace S. G., Santori E. K., Americus B., Li Z., et al. (2021). Biogenic amines increase the odds of bacterial vaginosis and affect the growth of and lactic acid production by vaginal lactobacillus spp. Appl. Environ. Microbiol. 87, e03068–e03020. doi: 10.1128/AEM.03068-20
Boris S., Suárez J. E., Vázquez F., Barbés C. (1998). Adherence of human vaginal lactobacilli to vaginal epithelial cells and interaction with uropathogens. Infect. Immun. 66, 1985–1989. doi: 10.1128/IAI.66.5.1985-1989.1998
Bradshaw C. S., Morton A. N., Hocking J., Garland S. M., Morris M. B., Moss L. M., et al. (2006). High recurrence rates of bacterial vaginosis over the course of 12 months after oral metronidazole therapy and factors associated with recurrence. J. Infect. Dis. 193, 1478–1486. doi: 10.1086/jid.2006.193.issue-11
Bradshaw C. S., Sobel J. D. (2016). Current treatment of bacterial vaginosis-limitations and need for innovation. J. Infect. Dis. 214, S14–S20. doi: 10.1093/infdis/jiw159
Brigtsen A. K., Jacobsen A. F., Dedi L., Melby K. K., Fugelseth D., Whitelaw A. (2015). Maternal Colonization with Group B Streptococcus Is Associated with an Increased Rate of Infants Transferred to the Neonatal Intensive Care Unit. Neonatology 108 (3), 157–163. doi: 10.1159/000434716
Brotman R. M., Bradford L. L., Conrad M., Gajer P., Ault K., Peralta L., et al. (2012). Association between Trichomonas vaginalis and vaginal bacterial community composition among reproductive-age women. Sex Transm. Dis. 39, 807–812. doi: 10.1097/OLQ.0b013e3182631c79
Carter K. A., Fischer M. D., Petrova M. I., Balkus J. E. (2023). Epidemiologic evidence on the role of Lactobacillus iners in sexually transmitted infections and bacterial vaginosis: A series of systematic reviews and meta-analyses. Sex Transm. Dis. 50, 224–235. doi: 10.1097/OLQ.0000000000001744
Cauci S., Culhane J. F., Di Santolo M., McCollum K. (2008). Among pregnant women with bacterial vaginosis, the hydrolytic enzymes sialidase and prolidase are positively associated with interleukin-1beta. Am. J. Obstet. Gynecol. 198, 132.e1–132.e1327. doi: 10.1016/j.ajog.2007.05.035
CDC (U.S. Centers for Disease Control and Prevention) (2021) Bacterial vaginosis -sti treatment guidelines. sexually transmitted infections treatment guideline. Available at: https://www.cdc.gov/std/treatment-guidelines/bv.htm (Accessed August 23, 2024).
Chee W. J. Y., Chew S. Y., Than L. T. L. (2020). Vaginal microbiota and the potential of Lactobacillus derivatives in maintaining vaginal health. Microb. Cell. Fact. 19, 203. doi: 10.1186/s12934-020-01464-4
Cheng L., Norenhag J., Hu Y. O. O., Brusselaers N., Fransson E., Ährlund-Richter A., et al. (2020). Vaginal microbiota and human papillomavirus infection among young Swedish women. NPJ Biofilms Microbiomes 6, 39. doi: 10.1038/s41522-020-00146-8
Chiu S. F., Huang P. J., Cheng W. H., Huang C. Y., Chu L. J., Lee C. C., et al. (2021). Vaginal Microbiota of the Sexually Transmitted Infections Caused by Chlamydia trachomatis and Trichomonas vaginalis in Women with Vaginitis in Taiwan. Microorganisms 9, 1864. doi: 10.3390/microorganisms9091864
Cohen C. R., Wierzbicki M. R., French A. L., Morris S., Newmann S., Reno H., et al. (2020). Randomized trial of lactin-V to prevent recurrence of bacterial vaginosis. N. Engl. J. Med. 382, 1906–1915. doi: 10.1056/NEJMoa1915254
Coleman J. S., Gaydos C. A. (2018). Molecular diagnosis of bacterial vaginosis: an update. J. Clin. Microbiol. 56, e00342–e00318. doi: 10.1128/JCM.00342-18
Cone R. A. (2014). Vaginal microbiota and sexually transmitted infections that may influence transmission of cell-associated HIV. J. Infect. Dis. 210, S616–S621. doi: 10.1093/infdis/jiu459
Cools P., Jespers V., Hardy L., Crucitti T., Delany-Moretlwe S., Mwaura M., et al. (2016). A multi-country cross-sectional study of vaginal carriage of group B streptococci (GBS) and escherichia coli in resource-poor settings: prevalences and risk factors. PloS One 11, e0148052. doi: 10.1371/journal.pone.0148052
Cordaillat-Simmons M., Rouanet A., Pot B. (2020). Live biotherapeutic products: the importance of a defined regulatory framework. Exp. Mol. Med. 52, 1397–1406. doi: 10.1038/s12276-020-0437-6
Coudray M. S., Madhivanan P. (2020). Bacterial vaginosis-A brief synopsis of the literature. Eur. J. Obstet. Gynecol. Reprod. Biol. 245, 143–148. doi: 10.1016/j.ejogrb.2019.12.035
Damelin L. H., Paximadis M., Mavri-Damelin D., Birkhead M., Lewis D. A., Tiemessen C. T. (2011). Identification of predominant culturable vaginal Lactobacillus species and associated bacteriophages from women with and without vaginal discharge syndrome in South Africa. J. Med. Microbiol. 60, 180–183. doi: 10.1099/jmm.0.024463-0
De Alberti D., Russo R., Terruzzi F., Nobile V., Ouwehand A. C. (2015). Lactobacilli vaginal colonisation after oral consumption of Respecta(®) complex: a randomised controlled pilot study. Arch. Gynecol. Obstet. 292, 861–867. doi: 10.1007/s00404-015-3711-4
Delgado-Diaz D. J., Jesaveluk B., Hayward J. A., Tyssen D., Alisoltani A., Potgieter M., et al. (2022). Lactic acid from vaginal microbiota enhances cervicovaginal epithelial barrier integrity by promoting tight junction protein expression. Microbiome 10, 141. doi: 10.1186/s40168-022-01337-5
Delgado-Diaz D. J., Tyssen D., Hayward J. A., Gugasyan R., Hearps A. C., Tachedjian G. (2020). Distinct immune responses elicited from cervicovaginal epithelial cells by lactic acid and short chain fatty acids associated with optimal and non-optimal vaginal microbiota. Front. Cell Infect. Microbiol. 9. doi: 10.3389/fcimb.2019.00446
Dessì D., Margarita V., Cocco A. R., Marongiu A., Fiori P. L., Rappelli P. (2019). Trichomonas vaginalis and Mycoplasma hominis: new tales of two old friends. Parasitology 146, 1150–1155. doi: 10.1017/S0031182018002135
Disha T., Haque F. (2022). Prevalence and risk factors of vulvovaginal Candidosis during pregnancy: a review. Infect Dis Obstet Gynecol. 6195712. doi: 10.1155/2022/6195712
Doerflinger S. Y., Throop A. L., Herbst-Kralovetz M. M. (2014). Bacteria in the vaginal microbiome alter the innate immune response and barrier properties of the human vaginal epithelia in a species-specific manner. J. Inf. Dis. 209, 1989–1999. doi: 10.1093/infdis/jiu004
Donders G. (2010). Diagnosis and management of bacterial vaginosis and other types of abnormal vaginal bacterial flora: a review. Obstet. Gynecol. Surv. 65, 462–473. doi: 10.1097/OGX.0b013e3181e09621
Donders G. G. G., Bellen G., Grinceviciene S., Ruban K., Vieira-Baptista P. (2017). Aerobic vaginitis: no longer a stranger. Res. Microbiol. 168, 845–858. doi: 10.1016/j.resmic.2017.04.004
Donders G., Bellen G., Rezeberga D. (2011). Aerobic vaginitis in pregnancy. BJOG 118, 1163–1170. doi: 10.1111/j.1471-0528.2011.03020.x
Donders G. G., Ruban K., Bellen G. (2015). Selecting anti-microbial treatment of aerobic vaginitis. Curr. Infect. Dis. Rep. 17, 477. doi: 10.1007/s11908-015-0477-6
Donders G. G., Vereecken A., Bosmans E., Dekeersmaecker A., Salembier G., Spitz B. (2002). Definition of a type of abnormal vaginal flora that is distinct from bacterial vaginosis: aerobic vaginitis. BJOG 109, 34–43. doi: 10.1111/j.1471-0528.2002.00432.x
Donnarumma G., Molinaro A., Cimini D., De Castro C., Valli V., De Gregorio V., et al. (2014). Lactobacillus crispatus L1: high cell density cultivation and exopolysaccharide structure characterization to highlight potentially beneficial effects against vaginal pathogens. BMC Microbiol. 14, 137. doi: 10.1186/1471-2180-14-137
Edelman S. M., Lehti T. A., Kainulainen V., Antikainen J., Kylväjä R., Baumann M., et al. (2012). Identification of a high-molecular-mass Lactobacillus epithelium adhesin (LEA) of Lactobacillus crispatus ST1 that binds to stratified squamous epithelium. Microbiol. (Reading) 158, 1713–1722. doi: 10.1099/mic.0.057216-0
Edwards V. L., Smith S. B., McComb E. J., Tamarelle J., Ma B., Humphrys M. S., et al. (2019). The cervicovaginal microbiota-host interaction modulates Chlamydia trachomatis infection. mBio 10, e01548–e01519. doi: 10.1128/mBio.01548-19
Eschenbach D. A. (2007). Bacterial vaginosis: resistance, recurrence, and/or reinfection? Clin. Inf. Dis. 44, 220–221. doi: 10.1086/509584
Esmaeili S. A., Mahmoudi M., Rezaieyazdi Z., Sahebari M., Tabasi N., Sahebkar A., et al. (2018). Generation of tolerogenic dendritic cells using Lactobacillus rhamnosus and Lactobacillus delbrueckii as tolerogenic probiotics. J. Cell Biochem. 119, 7865–7872. doi: 10.1002/jcb.v119.9
European Medicines Agency (2008). Committee for Medicinal Product for Human use (CHMP) Guideline on Human Cell-Based Medicinal Products–EMEA/CHMP/410869/2006. Available online at: https://www.ema.europa.eu/en/documents/scientific-guideline/guideline-human-cell-based-medicinal-products_en.pdf (Accessed August 26, 2024).
European Medicines Agency (2017). Committee for medicinal products for human use (CHMP) guideline on strategies to identify and mitigate risks for first-in-human and early clinical trials with investigational medicinal products–EMEA/CHMP/SWP/28367/07 rev. 1. Available online at: https://www.ema.europa.eu/en/documents/scientific-guideline/guideline-strategies-identify-and-mitigate-risks-first-human-and-early-clinical-trials-investigational-medicinal-products-revision-1_en.pdf (Accessed August 26, 2024).
European Medicines Agency (2021). ICH guideline E8 (R1) on general considerations for clinical studies. Available online at: https://www.ema.europa.eu/en/documents/regulatory-procedural-guideline/ich-guideline-e8-r1-general-considerations-clinical-studies_en.pdf (Accessed August 26, 2024).
European Pharmacopoeia Commission (EDQM) (2019). General monograph on live biotherapeutic products for human use, (3053). Ph. Eur. 9, 6522–6523.
Fan A., Yue Y., Geng N., Zhang H., Wang Y., Xue F. (2013). Aerobic vaginitis and mixed infections: comparison of clinical and laboratory findings. Arch. Gynecol. Obstet. 287, 329–335. doi: 10.1007/s00404-012-2571-4
Farr A., Sustr V., Kiss H., Rosicky I., Graf A., Makristathis A., et al. (2020). Oral probiotics to reduce vaginal group B streptococcal colonization in late pregnancy. Sci. Rep. 10, 19745. doi: 10.1038/s41598-020-76896-4
FDA (Food and Drug Administration) (2016). Early clinical trials with live biotherapeutic products: chemistry, manufacturing, and control information. Available online at: https://www.fda.gov/media/82945/download (Accessed January 7, 2024).
Feehily C., Crosby D., Walsh C. J., Lawton E. M., Higgins S., McAuliffe F. M., et al. (2020). Shotgun sequencing of the vaginal microbiome reveals both a species and functional potential signature of preterm birth. NPJ Biofilms Microbiomes 6, 50. doi: 10.1038/s41522-020-00162-8
Fettweis J. M., Serrano M. G., Brooks J. P., Edwards D. J., Girerd P. H., Parikh H. I., et al. (2019). The vaginal microbiome and preterm birth. Nat. Med. 25, 1012–1021. doi: 10.1038/s41591-019-0450-2
France M., Alizadeh M., Brown S., Ma B., Ravel J. (2022). Towards a deeper understanding of the vaginal microbiota. Nat. Microbiol. 7, 367–378. doi: 10.1038/s41564-022-01083-2
France M. T., Ma B., Gajer P., Brown S., Humphrys M. S., Holm J. B., et al. (2020). VALENCIA: a nearest centroid classification method for vaginal microbial communities based on composition. Microbiome 8, 166. doi: 10.1186/s40168-020-00934-6
Fredricks D. N., Fiedler T. L., Marrazzo J. M. (2005). Molecular identification of bacteria associated with bacterial vaginosis. N. Engl. J. Med. 353, 1899–1911. doi: 10.1056/NEJMoa043802
Gao Y., Shang Q., Wei J., Chen T. (2021). The correlation between vaginal microecological dysbiosis-related diseases and preterm birth: A review. Med. Microecol. 8, 100043. doi: 10.1016/j.medmic.2021.100043
Ge G., Yang Z., Li D., Zhang N., Chen B., Shi D. (2022). Distinct host immune responses in recurrent vulvovaginal candidiasis and vulvovaginal candidiasis. Front. Immunol. 13, 959740. doi: 10.3389/fimmu.2022.959740
Ghartey J. P., Smith B. C., Chen Z., Buckley N., Lo Y., Ratner A. J., et al. (2014). Lactobacillus crispatus dominant vaginal microbiome is associated with inhibitory activity of female genital tract secretions against Escherichia coli. PloS One 9, e96659. doi: 10.1371/journal.pone.0096659
Gigi R. M. S., Buitrago-Garcia D., Taghavi K., Dunaiski C. M., van de Wijgert J. H. H. M., Peters R. P. H., et al. (2023). Vulvovaginal yeast infections during pregnancy and perinatal outcomes: systematic review and meta-analysis. BMC Women’s Health 23, 116. doi: 10.1186/s12905-023-02258-7
Gomez-Lopez N., Romero R., Arenas-Hernandez M., Schwenkel G., St Louis D., Hassan S. S., et al. (2017). In vivo activation of invariant natural killer T cells induces systemic and local alterations in T-cell subsets prior to preterm birth. Clin. Exp. Immunol. 189, 211–225. doi: 10.1111/cei.12968
Grewal K., Lee Y. S., Smith A., Brosens J. J., Bourne T., Al-Memar M., et al. (2022). Chromosomally normal miscarriage is associated with vaginal dysbiosis and local inflammation. BMC Med. 20, 38. doi: 10.1186/s12916-021-02227-7
Gudnadottir U., Debelius J. W., Du J., Hugerth L. W., Danielsson H., Schuppe-Koistinen I., et al. (2022). The vaginal microbiome and the risk of preterm birth: a systematic review and network meta-analysis. Sci. Rep. 12, 7926. doi: 10.1038/s41598-022-12007-9
Haahr T., Clausen T. D., Thorsen J., Rasmussen M. A., Mortensen M. S., Lehtimäki J., et al. (2022). Vaginal dysbiosis in pregnancy associates with risk of emergency caesarean section: a prospective cohort study. Clin. Microbiol. Infect. 28, 588–595. doi: 10.1016/j.cmi.2021.08.028
Haahr T., Jensen J. S., Thomsen L., Duus L., Rygaard K., Humaidan P. (2016). Abnormal vaginal microbiota may be associated with poor reproductive outcomes: a prospective study in IVF patients. Hum. Reprod. 31, 795–803. doi: 10.1093/humrep/dew026
Han Y. W., Fardini Y., Chen C., Iacampo K. G., Peraino V. A., Shamonki J. M., et al. (2010). Term stillbirth caused by oral Fusobacterium nucleatum. Obstet. Gynecol. 115, 442–445. doi: 10.1097/AOG.0b013e3181cb9955
Han Y. W., Redline R. W., Li M., Yin L., Hill G. B., McCormick T. S. (2004). Fusobacterium nucleatum induces premature and term stillbirths in pregnant mice: implication of oral bacteria in preterm birth. Infect. Immun. 72, 2272–2279. doi: 10.1128/IAI.72.4.2272-2279.2004
Hanson L., Vandevusse L., Duster M., Warrack S., Safdar N. (2014). Feasibility of oral prenatal probiotics against maternal group B Streptococcus vaginal and rectal colonization. J. Obstet. Gynecol. Neonatal. Nurs. 43, 294–304. doi: 10.1111/1552-6909.12308
Hardy L., Cerca N., Jespers V., Vaneechoutte M., Crucitti T. (2017). Bacterial biofilms in the vagina. Res. Microbiol. 168, 865–874. doi: 10.1016/j.resmic.2017.02.001
Hearps A. C., Tyssen D., Srbinovski D., Bayigga L., Diaz D. J. D., Aldunate M., et al. (2017). Vaginal lactic acid elicits an anti-inflammatory response from human cervicovaginal epithelial cells and inhibits production of pro-inflammatory mediators associated with HIV acquisition. Mucosal Immunol. 10, 1480–1490. doi: 10.1038/mi.2017.27
Heczko P. B., Tomusiak A., Adamski P., Jakimiuk A. J., Stefański G., Mikołajczyk-Cichońska A., et al. (2015). Supplementation of standard antibiotic therapy with oral probiotics for bacterial vaginosis and aerobic vaginitis: a randomised, double-blind, placebo-controlled trial. BMC women’s Health 15, 115. doi: 10.1186/s12905-015-0246-6
Hemmerling A., Harrison W., Schroeder A., Park J., Korn A., Shiboski S., et al. (2010). Phase 2a study assessing colonization efficiency, safety, and acceptability of Lactobacillus crispatus CTV-05 in women with bacterial vaginosis. Sex Transm. Dis. 37, 745–750. doi: 10.1097/OLQ.0b013e3181e50026
Hinderfeld A. S., Phukan N., Bär A. K., Roberton A. M., Simoes-Barbosa A. (2019). Cooperative interactions between Trichomonas vaginalis and associated bacteria enhance paracellular permeability of the cervicovaginal epithelium by dysregulating tight junctions. Infect. Immun. 87, e00141–e00119. doi: 10.1128/IAI.00141-19
Holm J. B., France M. T., Gajer P., Ma B., Brotman R. M., Shardell M., et al. (2023). Integrating compositional and functional content to describe vaginal microbiomes in health and disease. Microbiome 11, 259. doi: 10.1186/s40168-023-01692-x
Hummelen R., Changalucha J., Butamanya N. L., Cook A., Habbema J. D., Reid G. (2010). Lactobacillus rhamnosus GR-1 and L. reuteri RC-14 to prevent or cure bacterial vaginosis among women with HIV. Int. J. Gynaecol. Obstet. 111, 245–248. doi: 10.1016/j.ijgo.2010.07.008
Husain S., Allotey J., Drymoussi Z., Wilks M., Fernandez-Felix B. M., Whiley A., et al. (2020). Effects of oral probiotic supplements on vaginal microbiota during pregnancy: a randomised, double-blind, placebo-controlled trial with microbiome analysis. BJOG 127, 275–284. doi: 10.1111/1471-0528.15675
Inversetti A., Zambella E., Guarano A., Dell’Avanzo M., Di Simone N. (2023). Endometrial microbiota and immune tolerance in pregnancy. Int. J. Mol. Sci. 24, 2995. doi: 10.3390/ijms24032995
Jacobsson B., Pernevi P., Chidekel L., Jörgen Platz-Christensen J. (2002). Bacterial vaginosis in early pregnancy may predispose for preterm birth and postpartum endometritis. Acta Obstet. Gynecol. Scand. 81, 1006–1010. doi: 10.1034/j.1600-0412.2002.811103.x
Jamieson D. J., Duerr A., Klein R. S., Paramsothy P., Brown W., Cu-Uvin S., et al. (2001). Longitudinal analysis of bacterial vaginosis: findings from the HIV epidemiology research study. Obstet. Gynecol. 98, 656–663. doi: 10.1097/00006250-200110000-00023
Jang S. J., Lee K., Kwon B., You H. J., Ko G. (2019). Vaginal lactobacilli inhibit growth and hyphae formation of Candida albicans. Sci. Rep. 9, 8121. doi: 10.1038/s41598-019-44579-4
Jarrett O. D., Srinivasan S., Richardson B. A., Fiedler T., Wallis J. M., Kinuthia J., et al. (2019). Specific vaginal bacteria are associated with an increased risk of Trichomonas vaginalis acquisition in women. J. Infect. Dis. 220, 1503–1510. doi: 10.1093/infdis/jiz354
Joesoef M. R., Schmid G. P., Hillier S. L. (1999). Bacterial vaginosis: review of treatment options and potential clinical indications for therapy. Clin. Infect. Dis. 28, S57–S65. doi: 10.1086/cid.1999.28.issue-s1
Kaambo E., Africa C., Chambuso R., Passmore J. S. (2018). Vaginal microbiomes associated with aerobic vaginitis and bacterial vaginosis. Front. Public Health 6. doi: 10.3389/fpubh.2018.00078
Kekki M., Kurki T., Pelkonen J., Kurkinen-Räty M., Cacciatore B., Paavonen J. (2001). Vaginal clindamycin in preventing preterm birth and peripartal infections in asymptomatic women with bacterial vaginosis: a randomized, controlled trial. Obstet. Gynecol. 97, 643–648. doi: 10.1016/s0029-7844(01)01321-7
Kim S. Y., Russell L. B., Park J., Verani J. R., Madhi S. A., Cutland C. L., et al. (2014). Cost-effectiveness of a potential group B streptococcal vaccine program for pregnant women in South Africa. Vaccine 32, 1954–1963. doi: 10.1016/j.vaccine.2014.01.062
Koumans E. H., Sternberg M., Bruce C., McQuillan G., Kendrick J., Sutton M., et al. (2007). The prevalence of bacterial vaginosis in the United States 2001-2004; associations with symptoms, sexual behaviors, and reproductive health. Sex Transm. Dis. 34, 864–869. doi: 10.1097/OLQ.0b013e318074e565
Krauss-Silva L., Almada-Horta A., Alves M. B., Camacho K. G., Moreira M. E., Braga A. (2014). Basic vaginal pH, bacterial vaginosis and aerobic vaginitis: prevalence in early pregnancy and risk of spontaneous preterm delivery, a prospective study in a low socioeconomic and multiethnic South American population. BMC Pregnancy Childbirth 14, 107. doi: 10.1186/1471-2393-14-107
Kremleva E. A., Sgibnev A. V. (2016). Proinflammatory cytokines as regulators of vaginal microbiota. Bull. Exp. Biol. Med. 162, 75–78. doi: 10.1007/s10517-016-3549-1
Kroon S. J., Ravel J., Huston W. M. (2018). Cervicovaginal microbiota, women’s health, and reproductive outcomes. Fertil. Steril. 110, 327–336. doi: 10.1016/j.fertnstert.2018.06.036
Kullar R., Goldstein E. J. C., Johnson S., McFarland L. V. (2023). Lactobacillus bacteremia and probiotics: A review. Microorganisms 11, 896. doi: 10.3390/microorganisms11040896
Kwatra G., Adrian P. V., Shiri T., Buchmann E. J., Cutland C. L., Madhi S. A. (2014). Serotype-specific acquisition and loss of group B Streptococcus recto-vaginal colonization in late pregnancy. PloS One 9, e98778. doi: 10.1371/journal.pone.0098778
Lagenaur L. A., Hemmerling A., Chiu C., Miller S., Lee P. P., Cohen C. R., et al. (2021). Connecting the dots: translating the vaginal microbiome into a drug. J. Infect. Dis. 223, S296–S306. doi: 10.1093/infdis/jiaa676
Lamont R. F., Duncan S. L., Mandal D., Bassett P. (2003). Intravaginal clindamycin to reduce preterm birth in women with abnormal genital tract flora. Obstet. Gynecol. 101, 516–522. doi: 10.1016/s0029-7844(02)03054-5
Łaniewski P., Herbst-Kralovetz M. M. (2021). Bacterial vaginosis and health-associated bacteria modulate the immunometabolic landscape in 3D model of human cervix. NPJ Biofilms Microbiomes 7, 88. doi: 10.1038/s41522-021-00259-8
Larsson P. G., Fåhraeus L., Carlsson B., Jakobsson T., Forsum U., Premature study group of the Southeast Health Care Region of Sweden (2006). Late miscarriage and preterm birth after treatment with clindamycin: a randomised consent design study according to Zelen. BJOG 113, 629–637. doi: 10.1111/j.1471-0528.2006.00946.x
Larsson P. G., Stray-Pedersen B., Ryttig K. R., Larsen S. (2008). Human lactobacilli as supplementation of clindamycin to patients with bacterial vaginosis reduce the recurrence rate; a 6-month, double-blind, randomized, placebo-controlled study. BMC Women’s Health 8, 3. doi: 10.1186/1472-6874-8-3
Latham-Cork H. C., Walker K. F., Thornton J. G., Gunnarsson O. S., Säfholm A., Cardell M., et al. (2021). A novel non-antimicrobial treatment of bacterial vaginosis: An open label two-private centre study. Eur. J. Obstet. Gynecol. Reprod. Biol. 256, 419–424. doi: 10.1016/j.ejogrb.2020.11.059
Laue C., Papazova E., Liesegang A., Pannenbeckers A., Arendarski P., Linnerth B., et al. (2018). Effect of a yoghurt drink containing Lactobacillus strains on bacterial vaginosis in women - a double-blind, randomised, controlled clinical pilot trial. Benef. Microbes 9, 35–50. doi: 10.3920/BM2017.0018
Lebeer S., Ahannach S., Gehrmann T., Wittouck S., Eilers T., Oerlemans E., et al. (2023). A citizen-science-enabled catalogue of the vaginal microbiome and associated factors. Nat. Microbiol. 8, 2183–2195. doi: 10.1038/s41564-023-01500-0
Lebeer S., Vanderleyden J., De Keersmaecker S. C. (2010). Host interactions of probiotic bacterial surface molecules: comparison with commensals and pathogens. Nat. Rev. Microbiol. 8, 171–184. doi: 10.1038/nrmicro2297
Lehtoranta L., Ala-Jaakkola R., Laitila A., Maukonen J. (2022). Healthy vaginal microbiota and influence of probiotics across the female life span. Front. Microbiol. 13. doi: 10.3389/fmicb.2022.819958
Lev-Sagie A., Goldman-Wohl D., Cohen Y., Dori-Bachash M., Leshem A., Mor U., et al. (2019). Vaginal microbiome transplantation in women with intractable bacterial vaginosis. Nat. Med. 25, 1500–1504. doi: 10.1038/s41591-019-0600-6
Li J., Lee D. S., Madrenas J. (2013). Evolving bacterial envelopes and plasticity of TLR2-dependent responses: basic research and translational opportunities. Front. Immunol. 4. doi: 10.3389/fimmu.2013.00347
Liu M. B., Xu S. R., He Y., Deng G. H., Sheng H. F., Huang X. M., et al. (2013). Diverse vaginal microbiomes in reproductive-age women with vulvovaginal candidiasis. PloS One 8, e79812. doi: 10.1371/journal.pone.0079812
López-Moreno A., Aguilera M. (2021). Vaginal probiotics for reproductive health and related dysbiosis: systematic review and meta-analysis. J. Clin. Med. 10, 1461. doi: 10.3390/jcm10071461
Ma X., Wu M., Wang C., Li H., Fan A., Wang Y., et al. (2022). The pathogenesis of prevalent aerobic bacteria in aerobic vaginitis and adverse pregnancy outcomes: a narrative review. Reprod. Health 19 (1), 21. doi: 10.1186/s12978-021-01292-8
MaChado A., Jefferson K. K., Cerca N. (2013). Interactions between Lactobacillus crispatus and bacterial vaginosis (BV)-associated bacterial species in initial attachment and biofilm formation. Int. J. Mol. Sci. 14, 12004–12012. doi: 10.3390/ijms140612004
Macklaim J. M., Fernandes A. D., Di Bella J. M., Hammond J. A., Reid G., Gloor G. B. (2013). Comparative meta-RNA-seq of the vaginal microbiota and differential expression by Lactobacillus iners in health and dysbiosis. Microbiome 1, 12. doi: 10.1186/2049-2618-1-12
Maldonado-Barragán A., Caballero-Guerrero B., Martín V., Ruiz-Barba J. L., Rodríguez J. M. (2016). Purification and genetic characterization of gassericin E, a novel co-culture inducible bacteriocin from Lactobacillus gasseri EV1461 isolated from the vagina of a healthy woman. BMC Microbiol. 16, 37. doi: 10.1186/s12866-016-0663-1
Mancabelli L., Mancino W., Lugli G. A., Milani C., Viappiani A., Anzalone R., et al. (2021). Comparative genome analyses of Lactobacillus crispatus isolated from different ecological niches reveal an environmental adaptation of this species to the human vaginal environment. Appl. Environ. Microbiol. 87, e02899–e02820. doi: 10.1128/AEM.02899-20
Mändar R., Sõerunurk G., Štšepetova J., Smidt I., Rööp T., Kõljalg S., et al. (2023). Impact of Lactobacillus crispatus-containing oral and vaginal probiotics on vaginal health: a randomised double-blind placebo controlled clinical trial. Benef. Microbes 14, 143–152. doi: 10.3920/BM2022.0091
Marciniak A., Karapanagiotidis G. T., Sarsam M., Sharma R. (2014). Postpartum Lactobacillus jensenii endocarditis in patient with bicuspid aortic valve. J. Thorac. Cardiovasc. Surg. 148, e219–e221. doi: 10.1016/j.jtcvs.2014.05.096
Marconi C., Santos-Greatti M. M., Parada C. M., Pontes A., Pontes A. G., Giraldo P. C., et al. (2014). Cervicovaginal levels of proinflammatory cytokines are increased during chlamydial infection in bacterial vaginosis but not in lactobacilli-dominated flora. J. Low. Genit. Tract Dis. 18, 261–265. doi: 10.1097/LGT.0000000000000003
Margarita V., Fiori P. L., Rappelli P. (2020). Impact of Symbiosis Between Trichomonas vaginalis and Mycoplasma hominis on Vaginal Dysbiosis: A Mini Review. Front. Cell Infect. Microbiol. 10. doi: 10.3389/fcimb.2020.00179
Martens M. G., Maximos B., Degenhardt T., Person K., Curelop S., Ghannoum M., et al. (2022). Phase 3 study evaluating the safety and efficacy of oteseconazole in the treatment of recurrent vulvovaginal candidiasis and acute vulvovaginal candidiasis infections. Am. J. Obstet. Gynecol 227, 880.e1–880.e11. doi: 10.1016/j.ajog.2022.07.023
McClure E. M., Goldenberg R. L. (2019). Use of antibiotics to reduce preterm birth. Lancet Glob. Health 7, e18–e19. doi: 10.1016/S2214-109X(18)30543-6
McGregor J. A., French J. I., Jones W., Milligan K., McKinney P. J., Patterson E., et al. (1994). Bacterial vaginosis is associated with prematurity and vaginal fluid mucinase and sialidase: results of a controlled trial of topical clindamycin cream. Am. J. Obstet. Gynecol. 170, 1048–1060. doi: 10.1016/S0002-9378(94)70098-2
McClelland R. S., Richardson B. A., Hassan W. M., Graham S. M., Kiarie J., et al. (2009 2009). Prospective study of vaginal bacterial flora and other risk factors for vulvovaginal candidiasis. J. Infect. Dis. 199 (12), 1883–1890. doi: 10.1086/599213
Mei L., Wang T., Chen Y., Wei D., Zhang Y., Cui T., et al. (2022). Dysbiosis of vaginal microbiota associated with persistent high-risk human papilloma virus infection. J. Transl. Med. 20, 12. doi: 10.1186/s12967-021-03201-w
Mendes-Soares H., Suzuki H., Hickey R. J., Forney L. J. (2014). Comparative functional genomics of Lactobacillus spp. reveals possible mechanisms for specialization of vaginal lactobacilli to their environment. J. Bacteriol. 196, 1458–1470. doi: 10.1128/JB.01439-13
Merhej V., Royer-Carenzi M., Pontarotti P., Raoult D. (2009). Massive comparative genomic analysis reveals convergent evolution of specialized bacteria. Biol. Direct 4, 13. doi: 10.1186/1745-6150-4-13
Mezzasalma V., Manfrini E., Ferri E., Boccarusso M., Di Gennaro P., Schiano I., et al. (2017). Orally administered multispecies probiotic formulations to prevent uro-genital infections: a randomized placebo-controlled pilot study. Arch. Gynecol. Obstet. 295, 163–172. doi: 10.1007/s00404-016-4235-2
Mirmonsef P., Gilbert D., Zariffard M. R., Hamaker B. R., Kaur A., Landay A. L., et al. (2011). The effects of commensal bacteria on innate immune responses in the female genital tract. Am. J. Reprod. Immunol. 65, 190–195. doi: 10.1111/j.1600-0897.2010.00943.x
Mitchell C. M., Balkus J., Agnew K. J., Cohn S., Luque A., Lawler R., et al. (2008). Bacterial vaginosis, not HIV, is primarily responsible for increased vaginal concentrations of proinflammatory cytokines. AIDS Res. Hum. Retroviruses 24, 667–671. doi: 10.1089/aid.2007.0268
Mitra A., MacIntyre D. A., Marchesi J. R., Lee Y. S., Bennett P. R., Kyrgiou M. (2016). The vaginal microbiota, human papillomavirus infection and cervical intraepithelial neoplasia: what do we know and where are we going next? Microbiome 4, 58. doi: 10.1186/s40168-016-0203-0
Mohan A., Rubin J., Chauhan P., Ramirez J. L., Giese G. (2020). Renal and perinephric abscesses involving Lactobacillus jensenii and Prevotella bivia in a young woman following ureteral stent procedure. J. Community Hosp. Intern. Med. Perspect. 10, 162–165. doi: 10.1080/20009666.2020.1742494
Monin L., Whettlock E. M., Male V. (2020). Immune responses in the human female reproductive tract. Immunology 160, 106–115. doi: 10.1111/imm.v160.2
Mullard A. (2022). FDA advisory committee votes for approval of first microbiome-based drug, despite data problems. Nat. Rev. Drug Discovery 21, 786–787. doi: 10.1038/d41573-022-00173-4
Muzny C. A., Blanchard E., Taylor C. M., Aaron K. J., Talluri R., Griswold M. E., et al. (2018). Identification of key bacteria involved in the induction of incident bacterial vaginosis: A prospective study. J. Infect. Dis. 218, 966–978. doi: 10.1093/infdis/jiy243
Muzny C. A., Łaniewski P., Schwebke J. R., Herbst-Kralovetz M. M. (2020). Host-vaginal microbiota interactions in the pathogenesis of bacterial vaginosis. Curr. Opin. Infect. Dis. 33, 59–65. doi: 10.1097/QCO.0000000000000620
Muzny C. A., Taylor C. M., Swords W. E., Tamhane A., Chattopadhyay D., Cerca N., et al. (2019). An updated conceptual model on the pathogenesis of bacterial vaginosis. J. Infect. Dis. 220, 1399–1405. doi: 10.1093/infdis/jiz342
Nasu K., Narahara H. (2010). Pattern recognition via the toll-like receptor system in the human female genital tract. Mediators Inflamm. 2010, 976024. doi: 10.1155/2010/976024
Nelson T. M., Borgogna J. L., Brotman R. M., Ravel J., Walk S. T., Yeoman C. J. (2015). Vaginal biogenic amines: biomarkers of bacterial vaginosis or precursors to vaginal dysbiosis? Front. Physiol. 6. doi: 10.3389/fphys.2015.00253
Netea M. G., Joosten L. A., van der Meer J. W., Kullberg B. J., van de Veerdonk F. L. (2015). Immune defence against Candida fungal infections. Nat. Rev. Immunol. 15, 630–642. doi: 10.1038/nri3897
Nourbakhsh F., Nasrollahzadeh M. S., Tajani A. S., Soheili V., Hadizadeh F. (2022). Bacterial biofilms and their resistance mechanisms: a brief look at treatment with natural agents. Folia Microbiol. (Praha) 67, 535–554. doi: 10.1007/s12223-022-00955-8
Nugent R. P., Krohn M. A., Hillier S. L. (1991). Reliability of diagnosing bacterial vaginosis is improved by a standardized method of gram stain interpretation. J. Clin. Microbiol. 29, 297–301. doi: 10.1128/jcm.29.2.297-301.1991
Nunn K. L., Forney L. J. (2016). Unraveling the dynamics of the human vaginal microbiome. Yale J. Biol. Med. 89, 331–337.
Nyirjesy P., Brookhart C., Lazenby G., Schwebke J., Sobel J. D. (2022). Vulvovaginal candidiasis: A review of the evidence for the 2021 centers for disease control and prevention of sexually transmitted infections treatment guidelines. Clin. Infect. Dis. 74, S162–S168. doi: 10.1093/cid/ciab1057
O’Hanlon D. E., Moench T. R., Cone R. A. (2011). In vaginal fluid, bacteria associated with bacterial vaginosis can be suppressed with lactic acid but not hydrogen peroxide. BMC Infect. Dis. 11, 200. doi: 10.1186/1471-2334-11-200
Odendaal J., Black N., Bennett P. R., Brosens J., Quenby S., MacIntyre D. A. (2024). The endometrial microbiota and early pregnancy loss. Hum. Reprod. 39, 638–646. doi: 10.1093/humrep/dead274
Oerlemans E. F. M., Bellen G., Claes I., Henkens T., Allonsius C. N., Wittouck S., et al. (2020b). Impact of a lactobacilli-containing gel on vulvovaginal candidosis and the vaginal microbiome. Sci. Rep. 10, 7976. doi: 10.1038/s41598-020-64705-x
Oerlemans E. F. M., Wuyts S., Bellen G., Wittouck S., De Boeck I., Ruban K., et al. (2020a). The dwindling microbiota of aerobic vaginitis, an inflammatory state enriched in pathobionts with limited TLR stimulation. Diagnostics (Basel) 10, 879. doi: 10.3390/diagnostics10110879
Ojala T., Kankainen M., Castro J., Cerca N., Edelman S., Westerlund-Wikström B., et al. (2014). Comparative genomics of Lactobacillus crispatus suggests novel mechanisms for the competitive exclusion of Gardnerella vaginalis. BMC Genomics 15, 1070. doi: 10.1186/1471-2164-15-1070
Olivier F. A. B., Hilsenstein V., Weerasinghe H., Weir A., Hughes S., Crawford S., et al. (2022). The escape of Candida albicans from macrophages is enabled by the fungal toxin candidalysin and two host cell death pathways. Cell. Rep. 40, 111374. doi: 10.1016/j.celrep.2022.111374
Osset J., Bartolomé R. M., García E., Andreu A. (2001). Assessment of the capacity of Lactobacillus to inhibit the growth of uropathogens and block their adhesion to vaginal epithelial cells. J. Infect. Dis. 183, 485–491. doi: 10.1086/jid.2001.183.issue-3
Palma E., Recine N., Domenici L., Giorgini M., Pierangeli A., Panici P. B. (2018). Long-term Lactobacillus rhamnosus BMX 54 application to restore a balanced vaginal ecosystem: a promising solution against HPV-infection. BMC Infect. Dis. 18, 13. doi: 10.1186/s12879-017-2938-z
Pan M., Hidalgo-Cantabrana C., Goh Y. J., Sanozky-Dawes R., Barrangou R. (2020). Comparative Analysis of Lactobacillus gasseri and Lactobacillus crispatus Isolated From Human Urogenital and Gastrointestinal Tracts. Front. Microbiol. 10. doi: 10.3389/fmicb.2019.03146
Pathakumari B., Liang G., Liu W. (2020). Immune defence to invasive fungal infections: A comprehensive review. Biomed. Pharmacother. 130, 110550. doi: 10.1016/j.biopha.2020.110550
Peebles K., Velloza J., Balkus J. E., McClelland R. S., Barnabas R. V. (2019). High global burden and costs of bacterial vaginosis: A systematic review and meta-analysis. Sex Transm. Dis. 46, 304–311. doi: 10.1097/OLQ.0000000000000972
Pendharkar S., Brandsborg E., Hammarström L., Marcotte H., Larsson P. G. (2015). Vaginal colonisation by probiotic lactobacilli and clinical outcome in women conventionally treated for bacterial vaginosis and yeast infection. BMC Infect. Dis. 15, 255. doi: 10.1186/s12879-015-0971-3
Petrova M. I., Lievens E., Malik S., Imholz N., Lebeer S. (2015). Lactobacillus species as biomarkers and agents that can promote various aspects of vaginal health. Front. Physiol. 6. doi: 10.3389/fphys.2015.00081
Petrova M. I., van den Broek M., Balzarini J., Vanderleyden J., Lebeer S. (2013). Vaginal microbiota and its role in HIV transmission and infection. FEMS Microbiol. Rev. 37, 762–792. doi: 10.1111/1574-6976.12029
Piersma S. J. (2011). Immunosuppressive tumor microenvironment in cervical cancer patients. Cancer Microenviron. 4, 361–375. doi: 10.1007/s12307-011-0066-7
Pradhan A., Avelar G. M., Bain J. M., Childers D., Pelletier C., Larcombe D. E., et al. (2019). Non-canonical signalling mediates changes in fungal cell wall PAMPs that drive immune evasion. Nat. Commun. 10, 5315. doi: 10.1038/s41467-019-13298-9
Pradines B., Domenichini S., Lievin-Le Moal V. (2022). Adherent Bacteria and Parasiticidal Secretion Products of Human Cervicovaginal Microbiota-Associated Lactobacillus gasseri Confer Non-Identical Cell Protection against Trichomonas vaginalis-Induced Cell Detachment. Pharm. (Basel) 15, 1350. doi: 10.3390/ph15111350
Rampersaud R., Planet P. J., Randis T. M., Kulkarni R., Aguilar J. L., Lehrer R. I., et al. (2011). Inerolysin, a cholesterol-dependent cytolysin produced by Lactobacillus iners. J. Bacteriol. 193, 1034–1041. doi: 10.1128/JB.00694-10
Ravel J., Gajer P., Abdo Z., Schneider G. M., Koenig S. S., McCulle S. L., et al. (2011). Vaginal microbiome of reproductive-age women. Proc. Natl. Acad. Sci. U.S.A. 108, 4680–4687. doi: 10.1073/pnas.1002611107
Rose W. A. 2nd, McGowin C. L., Spagnuolo R. A., Eaves-Pyles T. D., Popov V. L., Pyles R. B. (2012). Commensal bacteria modulate innate immune responses of vaginal epithelial cell multilayer cultures. PloS One 7, e32728. doi: 10.1371/journal.pone.0032728
Rossi F., Amadoro C., Gasperi M., Colavita G. (2022). Lactobacilli infection case reports in the last three years and safety implications. Nutrients 14, 6. doi: 10.3390/nu14061178
Rouanet A., Bolca S., Bru A., Claes I., Cvejic H., Girgis H., et al. (2020). Live biotherapeutic products, A road map for safety assessment. Front. Med. (Lausanne) 7. doi: 10.3389/fmed.2020.00237
Sabol K. E., Echevarria K. L., Lewis J. S. 2nd (2006). Community-associated methicillin-resistant Staphylococcus aureus: new bug, old drugs. Ann. Pharmacother. 40, 1125–1133. doi: 10.1345/aph.1G404
Salminen M. K., Rautelin H., Tynkkynen S., Poussa T., Saxelin M., Valtonen V., et al. (2004). Lactobacillus bacteremia, clinical significance, and patient outcome, with special focus on probiotic L. rhamnosus GG. Clin. Infect. Dis. 38 (1), 62–69. doi: 10.1086/380455
Salminen M. K., Rautelin H., Tynkkynen S., Poussa T., Saxelin M., Valtonen V., et al. (2006). Lactobacillus bacteremia, species identification, and antimicrobial susceptibility of 85 blood isolates. Clin. Infect. Dis. 42, e35–e44. doi: 10.1086/500214
Satora M., Grunwald A., Zaremba B., Frankowska K., Żak K., Tarkowski R., et al. (2023). Treatment of vulvovaginal candidiasis-an overview of guidelines and the latest treatment methods. J. Clin. Med. 12, 5376. doi: 10.3390/jcm12165376
Schellenberg J. J., Card C. M., Ball T. B., Mungai J. N., Irungu E., Kimani J., et al. (2012). Bacterial vaginosis, HIV serostatus and T-cell subset distribution in a cohort of East African commercial sex workers: retrospective analysis. AIDS 26, 387–393. doi: 10.1097/QAD.0b013e32834ed7f0
Schuster H. J., de Jonghe B. A., Limpens J., Budding A. E., Painter R. C. (2020). Asymptomatic vaginal Candida colonization and adverse pregnancy outcomes including preterm birth: a systematic review and meta-analysis. Am. J. Obstet Gynecol MFM 2, 100163. doi: 10.1016/j.ajogmf.2020.100163
Schwecht I., Nazli A., Gill B., Kaushic C. (2023). Lactic acid enhances vaginal epithelial barrier integrity and ameliorates inflammatory effects of dysbiotic short chain fatty acids and HIV-1. Sci. Rep. 13 (1), 20065. doi: 10.1038/s41598-023-47172-y
Siddiqui T., Kumar K. A., Iqbal A., Doultani P. R., Ashraf T., Eqbal F., et al. (2023). Efficacy and safety of oteseconazole in recurrent vulvovaginal candidiasis (RVVC) - A systematic review and meta-analysis. Heliyon 9, e20495. doi: 10.1016/j.heliyon.2023.e20495
Silao F. G. S., Valeriano V. D., Uddström E., Falconer E., Ljungdahl P. O. (2024). Diverse mechanisms control amino acid-dependent environmental alkalization by Candida albicans. Mol. Microbiol. 121, 696–716. doi: 10.1111/mmi.v121.4
Smaill F. M., Vazquez J. C. (2019). Antibiotics for asymptomatic bacteriuria in pregnancy. Cochrane Database Syst. Rev. 2019, CD000490. doi: 10.1002/14651858.CD000490
Song J., Lang F., Zhao N., Guo Y., Zhang H. (2018). Vaginal lactobacilli induce differentiation of monocytic precursors toward Langerhans-like cells: in vitro evidence. Front. Immunol. 9. doi: 10.3389/fimmu.2018.02437
Sousa L. G. V., Pereira S. A., Cerca N. (2023). Fighting polymicrobial biofilms in bacterial vaginosis. Microb. Biotechnol. 16, 1423–1437. doi: 10.1111/1751-7915.14261
Stapleton A. E. (2016). The vaginal microbiota and urinary tract infection. Microbiol. Spectr. 4 (6). doi: 10.1128/microbiolspec.UTI-0025-2016
Stapleton A. E., Au-Yeung M., Hooton T. M., Fredricks D. N., Roberts P. L., Czaja C. A., et al. (2011). Randomized, placebo-controlled phase 2 trial of a Lactobacillus crispatus probiotic given intravaginally for prevention of recurrent urinary tract infection. Clin. Infect. Dis. 52, 1212–1217. doi: 10.1093/cid/cir183
Stika C. S. (2010). Atrophic vaginitis. Dermatol. Ther. 23, 514–522. doi: 10.1111/j.1529-8019.2010.01354.x
Subtil D., Brabant G., Tilloy E., Devos P., Canis F., Fruchart A., et al. (2018). Early clindamycin for bacterial vaginosis in pregnancy (PREMEVA): a multicentre, double-blind, randomised controlled trial. Lancet. 392 (10160), 2171–2179. doi: 10.1016/S0140-6736(18)31617-9
Suárez-García I., Sánchez-García A., Soler L., Malmierca E., Gómez-Cerezo J. (2012). Lactobacillus jensenii bacteremia and endocarditis after dilatation and curettage: case report and literature review. Infection 40, 219–222. doi: 10.1007/s15010-011-0182-9
Tachedjian G., O’Hanlon D. E., Ravel J. (2018). The implausible “in vivo” role of hydrogen peroxide as an antimicrobial factor produced by vaginal microbiota. Microbiome 6, 29. doi: 10.1186/s40168-018-0418-3
Takano T., Kudo H., Eguchi S., Matsumoto A., Oka K., Yamasaki Y., et al. (2023). Inhibitory effects of vaginal lactobacilli on Candida albicans growth, hyphal formation, biofilm development, and epithelial cell adhesion. Front. Cell Infect. Microbiol. 13. doi: 10.3389/fcimb.2023.1113401
Tellapragada C., Eshwara V. K., Bhat P., Kamath A., Aletty S., Mukhopadhyay C. (2017). Screening of vulvovaginal infections during pregnancy in resource constrained settings: Implications on preterm delivery. J. Infect. Public Health 10 (4), 431–437. doi: 10.1016/j.jiph.2016.06.003
Tempera G., Furneri P. M. (2010). Management of aerobic vaginitis. Gynecol. Obstet. Invest. 70, 244–249. doi: 10.1159/000314013
Toprak N. U., Bozan T., Yılmaz S., Buyukbayrak E. E., Tigen E. T. (2022). Polymicrobial bacteremia due to Lactobacillus jensenii and Veillonella montpellierensis in a pregnant patient; case report and review of literature. Anaerobe 75, 102576. doi: 10.1016/j.anaerobe.2022.102576
Tortelli B. A., Lewis W. G., Allsworth J. E., Member-Meneh N., Foster L. R., Reno H. E., et al. (2020). Associations between the vaginal microbiome and Candida colonization in women of reproductive age. Am. J. Obstet. Gynecol. 222, 471.e1–471.e9. doi: 10.1016/j.ajog.2019.10.008
Triggs T., Kumar S., Mitchell M. (2020). Experimental drugs for the inhibition of preterm labor. Expert Opin. Investig. Drug 29, 507–523. doi: 10.1080/13543784.2020.1752661
Turovskiy Y., Ludescher R. D., Aroutcheva A. A., Faro S., Chikindas M. L. (2009). Lactocin 160, a Bacteriocin Produced by Vaginal Lactobacillus rhamnosus, Targets Cytoplasmic Membranes of the Vaginal Pathogen, Gardnerella vaginalis. Probiotics Antimicrob. Proteins 1, 67–74. doi: 10.1007/s12602-008-9003-6
Uwamahoro N., Verma-Gaur J., Shen H. H., Qu Y., Lewis R., Lu J., et al. (2014). The pathogen Candida albicans hijacks pyroptosis for escape from macrophages. mBio 5, e00003–e00e14. doi: 10.1128/mBio.00003-14
Vander Haar E. L., So J., Gyamfi-Bannerman C., Han Y. W. (2018). Fusobacterium nucleatum and adverse pregnancy outcomes: Epidemiological and mechanistic evidence. Anaerobe 50, 55–59. doi: 10.1016/j.anaerobe.2018.01.008
van der Veer C., Hertzberger R. Y., Bruisten S. M., Tytgat H. L. P., Swanenburg J., de Kat Angelino-Bart A., et al. (2019). Comparative genomics of human Lactobacillus crispatus isolates reveals genes for glycosylation and glycogen degradation: implications for in vivo dominance of the vaginal microbiota. Microbiome 7, 49. doi: 10.1186/s40168-019-0667-9
van Teijlingen N. H., van Smoorenburg M. Y., Sarrami-Forooshani R., Zijlstra-Willems E. M., van Hamme J. L., Borgdorff H., et al. (2024). Prevotella timonensis bacteria associated with vaginal dysbiosis enhance HIV-1 susceptibility of vaginal CD4+ T cells. J. Infect. Dis. 230, e43–e47. doi: 10.1093/infdis/jiae166
Velloza J., Heffron R. (2017). The vaginal microbiome and its potential to impact efficacy of HIV pre-exposure prophylaxis for women. Curr. HIV/AIDS Rep. 14, 153–160. doi: 10.1007/s11904-017-0362-z
Verwijs M. C., Agaba S. K., Darby A. C., van de Wijgert J. H. H. M. (2020). Impact of oral metronidazole treatment on the vaginal microbiota and correlates of treatment failure. Am. J. Obstet. Gynecol 222, 157.e1–157.e13. doi: 10.1016/j.ajog.2019.08.008
Vitali D., Wessels J. M., Kaushic C. (2017). Role of sex hormones and the vaginal microbiome in susceptibility and mucosal immunity to HIV-1 in the female genital tract. AIDS Res. Ther. 14, 39. doi: 10.1186/s12981-017-0169-4
Vyshenska D., Lam K. C., Shulzhenko N., Morgun A. (2017). Interplay between viruses and bacterial microbiota in cancer development. Semin. Immunol. 32, 14–24. doi: 10.1016/j.smim.2017.05.003
Wang X., Buhimschi C. S., Temoin S., Bhandari V., Han Y. W., Buhimschi I. A. (2013). Comparative microbial analysis of paired amniotic fluid and cord blood from pregnancies complicated by preterm birth and early-onset neonatal sepsis. PloS One 8, e56131. doi: 10.1371/journal.pone.0056131
Wang Y., Noël-Romas L., Perner M., Knodel S., Molatlhegi R., Hoger S., et al. (2023). Non-lactobacillus-dominant and polymicrobial vaginal microbiomes are more common in younger South African women and predictive of increased risk of human immunodeficiency virus acquisition. Clin. Infect. Dis. 76, 1372–1381. doi: 10.1093/cid/ciac938
Wang S., Wang Q., Yang E., Yan L., Li T., Zhuang H. (2017). Antimicrobial Compounds Produced by Vaginal Lactobacillus crispatus Are Able to Strongly Inhibit Candida albicans Growth, Hyphal Formation and Regulate Virulence-related Gene Expressions. Front. Microbiol. 8. doi: 10.3389/fmicb.2017.00564
Wessels J. M., Felker A. M., Dupont H. A., Kaushic C. (2018). The relationship between sex hormones, the vaginal microbiome and immunity in HIV-1 susceptibility in women. Dis. Model. Mech. 11, dmm035147. doi: 10.1242/dmm.035147
Westman J., Moran G., Mogavero S., Hube B., Grinstein S. (2018). Candida albicans hyphal expansion causes phagosomal membrane damage and luminal alkalinization. mBio 9, e01226–e01218. doi: 10.1128/mBio.01226-18
Wiesenfeld H. C., Hillier S. L., Krohn M. A., Amortegui A. J., Heine R. P., Landers D. V., et al. (2002). Lower genital tract infection and endometritis: insight into subclinical pelvic inflammatory disease. Obstet. Gynecol. 100, 456–463. doi: 10.1097/00006250-200209000-00011
Wrønding T., Vomstein K., Bosma E. F., Mortensen B., Westh H., Heintz J. E., et al. (2023). Antibiotic-free vaginal microbiota transplant with donor engraftment, dysbiosis resolution and live birth after recurrent pregnancy loss: a proof-of-concept case study. EClinicalMedicine 61, 102070. doi: 10.1016/j.eclinm.2023.102070
Wu S., Hugerth L. W., Schuppe-Koistinen I., Du J. (2022). The right bug in the right place: opportunities for bacterial vaginosis treatment. NPJ Biofilms Microbiomes 8, 34. doi: 10.1038/s41522-022-00295-y
Yeoman C. J., Yildirim S., Thomas S. M., Durkin A. S., Torralba M., Sutton G., et al. (2010). Comparative genomics of Gardnerella vaginalis strains reveals substantial differences in metabolic and virulence potential. PloS One 5, e12411. doi: 10.1371/journal.pone.0012411
Zhang C., Liu Y., Gao W., Pan Y., Gao Y., Shen J., et al. (2018). The direct and indirect association of cervical microbiota with the risk of cervical intraepithelial neoplasia. Cancer Med. 7, 2172–2179. doi: 10.1002/cam4.2018.7.issue-5
Zheng N., Guo R., Wang J., Zhou W., Ling Z. (2021). Contribution of Lactobacillus iners to vaginal health and diseases: A systematic review. Front. Cell Infect. Microbiol. 11. doi: 10.3389/fcimb.2021.792787
Zhou Y., Cheng L., Lei Y. L., Ren B., Zhou X. (2021a). The interactions between Candida albicans and mucosal immunity. Front. Microbiol. 12. doi: 10.3389/fmicb.2021.652725
Keywords: dysbiosis, vaginal microbiome, vaginal health, live biopharmaceutical products, lactobacilli
Citation: Valeriano VD, Lahtinen E, Hwang I-C, Zhang Y, Du J and Schuppe-Koistinen I (2024) Vaginal dysbiosis and the potential of vaginal microbiome-directed therapeutics. Front. Microbiomes 3:1363089. doi: 10.3389/frmbi.2024.1363089
Received: 29 December 2023; Accepted: 16 September 2024;
Published: 11 October 2024.
Edited by:
Ken Blount, Rebiotix Inc., United StatesReviewed by:
Tingtao Chen, Nanchang University, Nanchang, ChinaCaroline Mitchell, Massachusetts General Hospital and Harvard Medical School, United States
Copyright © 2024 Valeriano, Lahtinen, Hwang, Zhang, Du and Schuppe-Koistinen. This is an open-access article distributed under the terms of the Creative Commons Attribution License (CC BY). The use, distribution or reproduction in other forums is permitted, provided the original author(s) and the copyright owner(s) are credited and that the original publication in this journal is cited, in accordance with accepted academic practice. No use, distribution or reproduction is permitted which does not comply with these terms.
*Correspondence: Ina Schuppe-Koistinen, ina.schuppe.koistinen@ki.se