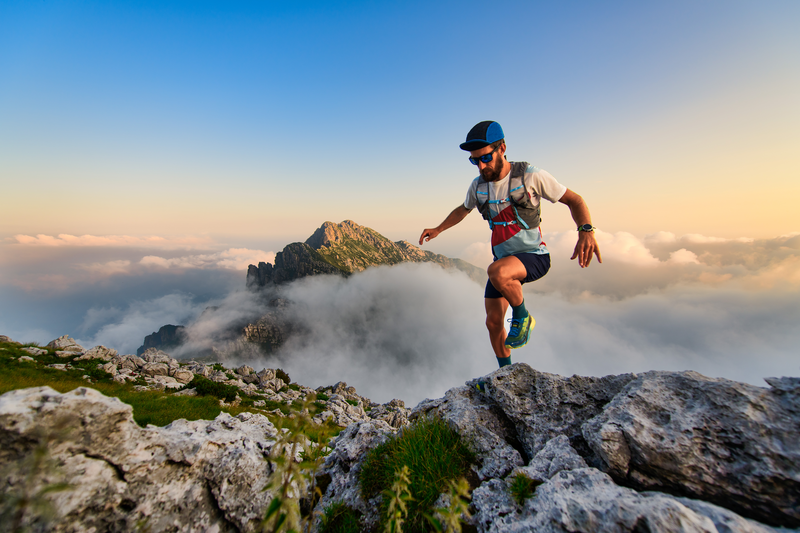
94% of researchers rate our articles as excellent or good
Learn more about the work of our research integrity team to safeguard the quality of each article we publish.
Find out more
EDITORIAL article
Front. Microbiol.
Sec. Food Microbiology
Volume 16 - 2025 | doi: 10.3389/fmicb.2025.1577852
This article is part of the Research Topic Omics Technologies and Bioinformatic Tools in Probiotic Research View all 7 articles
The final, formatted version of the article will be published soon.
You have multiple emails registered with Frontiers:
Please enter your email address:
If you already have an account, please login
You don't have a Frontiers account ? You can register here
Probiotics are defined as live microorganisms that can promote intestinal and extraintestinal health benefits when consumed in sufficient quantity (Hill et al., 2014). Several bifidobacteria, lactobacilli and enterococci have been classified as probiotics due to their safety profile and health-promoting properties. These microorganisms are commonly found in various habitats, including dairy and non-dairy fermented products, the mammalian gastrointestinal microbiota and the environment. For a new strain to be classified as a probiotic, a number of criteria should be fulfilled: resistance to gastrointestinal transit, lack of virulence and transmissible antibiotic resistance genes, and health-promoting activities (e.g. antimicrobial, immunomodulatory, antioxidant). Regulatory authorities have established conventional microbiological assays to assess these phenotypes (FAO/WHO, 2001). In addition, high-throughput multi-omics approaches are now being applied to complement existing methodologies and provide deeper molecular and cellular insights into probiotic-host interactions (Kiousi et al., 2021).In the era of (meta)genomics, the availability of whole genome sequences (WGS) of probiotic strains has increased exponentially. The integration of the genomic element in probiotic studies supports the prediction of the safety and functional profile of a new strain. In addition, WGS is the "gold standard" for the taxonomic classification of new isolates into species due to its higher discriminatory power. Indeed, the increased availability of WGS facilitated the reclassification of the diverse emended Lactobacillus spp. into 25 genera based on shared ecological and metabolic properties (Zheng et al., 2020). Currently, EFSA requires WGS of microorganisms to be used in the food chain to monitor genes of concern (e.g. virulence factors, antibiotic resistance genes) (EFSA, 2024). In this context, genomic analyses supplemented with in vitro assays were performed by Wei et al. to evaluate the safety and functional traits of Limosilactobacillus reuteri A51, a strain previously isolated from yak yoghurt. It was found that the strain codes for genes related to stress responses, survival and attachment in the gastrointestinal tract, and for biosynthetic clusters for antimicrobial compounds and exopolysaccharides. The strain also exhibited increased tolerance to simulated gastrointestinal conditions, as well as antioxidant and antimicrobial activity. Wang et al.performed similar analysis for Lactiplantibacillus plantarum HOM2217, a strain isolated from human milk, and examined its potential as an alternative treatment for obesity. It was shown that the strain possesses cholesterol removal activity in vitro. In addition, it could regulate lipid metabolism and inflammation, therefore contributing to the prevention of obesity in rats fed a high-fat diet (HFD). Similarly, Enterococcus rotai CMTB-CA6, a strain isolated from the medicinal plant Cantella asiatica by Kim et al., limited the growth of skin pathogens, induced commensal growth and increased dermal fibroblast viability in vitro. No transferable antimicrobial resistance genes or virulence factors were identified in the WGS of the strain, suggesting its safe use in skin care products.Apart from probiotics derived from aerobic or microaerophilic environments, strictly anaerobic commensal microorganisms, most notably Feacalibacterium prausnitzii and Akkermansia muciniphila have been used as next-generation probiotics (O'Toole et al., 2017).These microorganisms are adapted to grow in the GI tract of the host and thus present an evolutionary advantage in the niche. Indeed, Vergalito et al. showed that A. muciniphila ATCC BAA-835 exhibited increased viability in simulated GI tract conditions and higher adhesion capacity compared to Lacticaseibacillus rhamnosus GG, one of the most well-studied probiotic strains. Genes for mucus-degrading enzymes and for two mucus-binding proteins involved in the adhesion capacity of A. muciniphila were also detected in the genome of the strain.Comparative genomic analysis has supported the identification of conserved antiphage responses in the genome of bacteria. One of these adaptive systems is clustered regularly interspaced short palindromic repeats (CRISPR) -and CRISPR-associated (Cas) loci that encodes for a primitive acquired immunity system that patrols the cytoplasm and degrades phage-derived genomic sequences (Makarova et al., 2020). In the context of the food industry, phages can decrease cell growth and cause cell lysis, therefore significantly affect the fermentation capacity of microbial strains, and the texture and aroma of the end-product (Ranveer et al., 2024). In lactobacilli CRISPR-Cas arrays are distributed in a strain-specific manner. Indeed, Rostampour et al. found that Cas proteins are present in 22% of the available genomes of Lp. plantarum strains, with subtype II-A being the most common among strains, followed by type I-E. Further analysis showed that subtype II-A could play a more active role in the defensive capacity of the strains due to its larger repeat-spacer arrays. Of note, these spacers also seem to confer resistance to plasmid-mediated gene transfer, therefore presenting broader activity. It should be noted that CRISPR-Cas engineering is a leading method for efficient manipulation of genomic sequences. Therefore, understanding the prevalence of these systems in lactobacilli can support the expansion of the lactobacilli bioengineering toolkit (Parvin and Sadras, 2024). Furthermore, comparative genomics can be utilized for the identification of conserved genes involved in antibiotic resistance. Aborode et al. applied this method to identify antibiotic resistance genes in Escherichia coli genomes. The structure of the gene products was predicted in silico and docking experiments were performed to evaluate the capacity of phytochemicals to be effective inhibitors. Utilizing this approach, the authors confirmed that hesperidin, a flavanone glycoside derived from citrus fruit, presented favorable pharmacokinetics, good stability and plausible binding positions against resistant E. coli strains carrying the MacB, gidB and katG genes.In conclusion, this Research Topic provides valuable knowledge and information on the mechanisms of action and health-promoting activities of novel potential probiotic strains isolated from different sources, such as dairy products and environmental samples. Clearly, the probiotic field is moving towards mechanistic studies to establish causative relationships between probiotics administration and host health. In this context, new databases, search engines, and bioinformatics pipelines have been developed to systematically collect and present the bulk of data presented in these studies, to support their meaningful interpretation and visualization, and to facilitate comparative analysis and the selection of the most promising strains for further research. For example, Probio-ichnos is a manually curated, literaturederived database that collects and presents data for microorganisms with in vitro probiotic properties (Tsifintaris et al., 2024), MASI catalogues the interactions between microbiota and probiotics with active substances (Zeng et al., 2021), and ODRAP explores prebiotic activity (Guseva et al., 2020). Moreover, the machine learning platform iProbiotics supports the rapid identification of probiotic properties from WGS data (Sun et al., 2022), whereas ProbioMinServer is an integrated platform for evaluating the safety and functionality of potential probiotic strains (Liu et al., 2023). These databases, platforms, bioinformatic tools, and methodologies presented in this Research Topic can be used in combination to provide a holistic view on the interplay between probiotics, food and intestinal microbiota, leading to tailor-made, strain-, host-, and disease-specific probiotic supplements.
Keywords: Probiotics, multi-omics, Metagenomics, Transcriptomics, databases, Bioinformatic tools, whole genome sequence, CRISPR-Cas
Received: 16 Feb 2025; Accepted: 19 Feb 2025.
Copyright: © 2025 Galanis, Papadimitriou and Moloney. This is an open-access article distributed under the terms of the Creative Commons Attribution License (CC BY). The use, distribution or reproduction in other forums is permitted, provided the original author(s) or licensor are credited and that the original publication in this journal is cited, in accordance with accepted academic practice. No use, distribution or reproduction is permitted which does not comply with these terms.
* Correspondence:
Alex Galanis, Department of Molecular Biology and Genetics, Democritus University of Thrace, Alexandroupolis, Greece
Disclaimer: All claims expressed in this article are solely those of the authors and do not necessarily represent those of their affiliated organizations, or those of the publisher, the editors and the reviewers. Any product that may be evaluated in this article or claim that may be made by its manufacturer is not guaranteed or endorsed by the publisher.
Research integrity at Frontiers
Learn more about the work of our research integrity team to safeguard the quality of each article we publish.