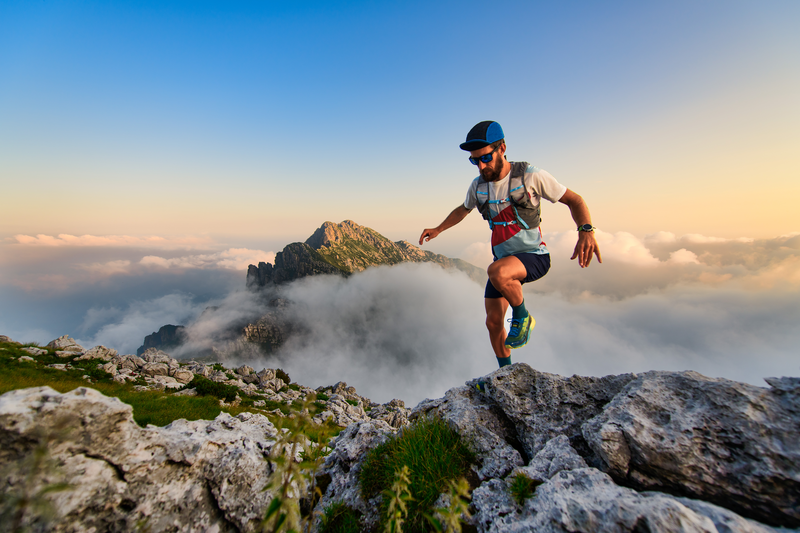
94% of researchers rate our articles as excellent or good
Learn more about the work of our research integrity team to safeguard the quality of each article we publish.
Find out more
ORIGINAL RESEARCH article
Front. Microbiol.
Sec. Microbe and Virus Interactions with Plants
Volume 16 - 2025 | doi: 10.3389/fmicb.2025.1573724
The final, formatted version of the article will be published soon.
You have multiple emails registered with Frontiers:
Please enter your email address:
If you already have an account, please login
You don't have a Frontiers account ? You can register here
A The pomegranate (Punica granatum) is a significant economic tree species. In recent years, the root collar rot has severely affected pomegranates in the dry-hot valley regions of Yunnan Province, China. The rhizosphere microbiome plays a crucial role in plant growth, development, and disease resistance. This study utilized Illumina MiSeq sequencing to analyze the fungal communities in the roots and rhizosphere soils of healthy and diseased pomegranates, focusing on the impact of root collar rot disease on the diversity and structural composition of these communities. The results indicated that in the unique fungal communities of healthy plant roots, the relative abundance of ectomycorrhizal and arbuscular mycorrhizal functional (AMF) groups was 53.77%, including genera such as Glomus and Septoglomus. After infection with root collar rot disease, the rhizosphere fungal communities became more monotonous, with increased differentiation within sample groups. Fungal groups associated with plant diseases and soil nutrient structures underwent significant changes. The disease altered the composition and functional group proportions of rhizosphere fungal communities, a process linked to soil nutrient structures. And the balance between plant-pathogen-related and saprotrophic functional groups in the rhizosphere was disrupted. Through Koch's postulates verification, the pathogen was identified as Lauriomyces bellulus. This is the first report of collar rot of pomegranate caused by L. bellulus in China. Studying the differences in rhizosphere fungal community structures and quantities in response to new diseases aids in the rapid prediction of pathogens, effectively saving diagnostic time, and provides theoretical support for disease prediction, diagnosis, and control. Pomegranate (Punica granatum L.) has been widely cultivated for its nutritional and medicinal value, strong environmental adaptability, ease of management, and high economic benefits. In recent years, root collar rot has become increasingly prevalent in the dry-hot valley regions of Yunnan Province, China, causing significant economic losses for local fruit growers. This soilborne disease occurs when the base of the plant stem and the root collar are infected by pathogens. Internationally, Phytophthora species have been identified as common pathogens (Basım et al., 2013;Kurbetli et al., 2020;Ghaderi et al., 2021).
Keywords: pomegranate, Root collar rot, fungal in the pomegranate rhizosphere, the diversity and structural composition, the soil physicochemical properties
Received: 09 Feb 2025; Accepted: 06 Mar 2025.
Copyright: © 2025 Wu, Chen, Chen, Yang, Aiting and Wu. This is an open-access article distributed under the terms of the Creative Commons Attribution License (CC BY). The use, distribution or reproduction in other forums is permitted, provided the original author(s) or licensor are credited and that the original publication in this journal is cited, in accordance with accepted academic practice. No use, distribution or reproduction is permitted which does not comply with these terms.
* Correspondence:
Jianrong Wu, Key Laboratory of Forest Disaster Warning and Control of Yunnan Province, College of Forestry, Southwest Forestry University, Kunming, China
Disclaimer: All claims expressed in this article are solely those of the authors and do not necessarily represent those of their affiliated organizations, or those of the publisher, the editors and the reviewers. Any product that may be evaluated in this article or claim that may be made by its manufacturer is not guaranteed or endorsed by the publisher.
Research integrity at Frontiers
Learn more about the work of our research integrity team to safeguard the quality of each article we publish.