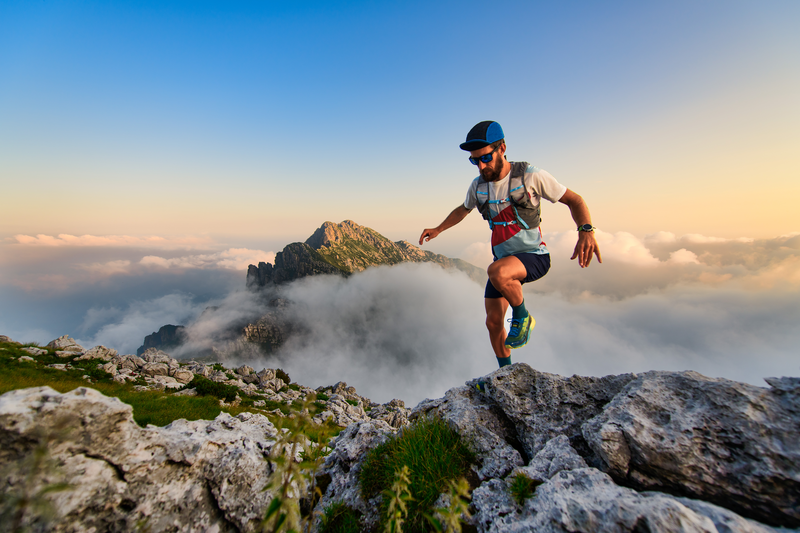
94% of researchers rate our articles as excellent or good
Learn more about the work of our research integrity team to safeguard the quality of each article we publish.
Find out more
ORIGINAL RESEARCH article
Front. Microbiol.
Sec. Food Microbiology
Volume 16 - 2025 | doi: 10.3389/fmicb.2025.1570312
This article is part of the Research Topic Beneficial Microbes for Sustainable Postharvest Management of Fresh Produce View all 6 articles
The final, formatted version of the article will be published soon.
You have multiple emails registered with Frontiers:
Please enter your email address:
If you already have an account, please login
You don't have a Frontiers account ? You can register here
Strawberries are renowned for their exceptional flavor and nutritional properties but have a short shelf life due to rapid ripening and a high vulnerability to postharvest microbial decay. Postbiotic formulations (PBFs) derived from lactic acid bacteria (LAB) can be developed into effective preservation products, extending postharvest shelf life while maintaining fruit quality. Methods. This study aimed to assess the effects of postbiotic-based formulations (PBFs) consisting of two key components: 1) a precipitated peptide-protein extract (PP) from Weissella cibaria UTNGt21O, serving as the antimicrobial agent, and 2) an exopolysaccharide (EPS) from W. confusa UTNCys2-2, functioning as the biopolymer carrier. These formulations were tested against a multidrug-resistant Serratia liquefaciens P4StpC1 strain, isolated from ready-to-eat strawberries, and their potential mode of action was analyzed in vitro. Time-kill assays and electron microscopy were used to evaluate their impact on the target cells. Furthermore, the performance of PBFs was compared to a commercial disinfectant (C1) in terms of their effects on strawberry microbiota and fruit quality, employing bacteriological techniques and 16S amplicon metagenomic analysis. Results. The selected PBFs showed bacteriolytic effect on Serratia in vitro. The target cell viability was significantly reduced upon one hour co-cultivation by inducing several morphological and ultrastructural modifications. Dipping strawberries at the ripe stage four in PBFs indicated no increase in total cell counts, thus the microorganisms colonization was retained during storage with refrigeration. The 16S metagenome analysis showed that the treatment impacted the fruit microbiota, significantly increasing Lactobacillus abundance (p < 0.001) by day eight compared to the disinfectant control. This suggests the formulation supports beneficial microbes, enhancing antimicrobial effects. Additionally, the postbiotic coating improved shelf-life, preserved fruit quality, and delayed deterioration in strawberries. The strawberries quality attributes were not affected by the treatment. Principal Component Analysis (PCA) revealed clear sample separation based on maturity stage, independent of the treatment. Conclusion. The results highlight the potential of crosslinking of a peptide-protein fraction with EPS to prevent the colonization of undesirable microorganisms on postharvest strawberries while enhancing their safety and quality.
Keywords: Postbiotics, antimicrobials, strawberries, Serratia liquefaciens, 16S amplicon
Received: 03 Feb 2025; Accepted: 10 Mar 2025.
Copyright: © 2025 Tenea, Reyes and Flores. This is an open-access article distributed under the terms of the Creative Commons Attribution License (CC BY). The use, distribution or reproduction in other forums is permitted, provided the original author(s) or licensor are credited and that the original publication in this journal is cited, in accordance with accepted academic practice. No use, distribution or reproduction is permitted which does not comply with these terms.
* Correspondence:
Gabriela N. Tenea, Universidad Técnica del Norte, Ibarra, Ecuador
Disclaimer: All claims expressed in this article are solely those of the authors and do not necessarily represent those of their affiliated organizations, or those of the publisher, the editors and the reviewers. Any product that may be evaluated in this article or claim that may be made by its manufacturer is not guaranteed or endorsed by the publisher.
Research integrity at Frontiers
Learn more about the work of our research integrity team to safeguard the quality of each article we publish.