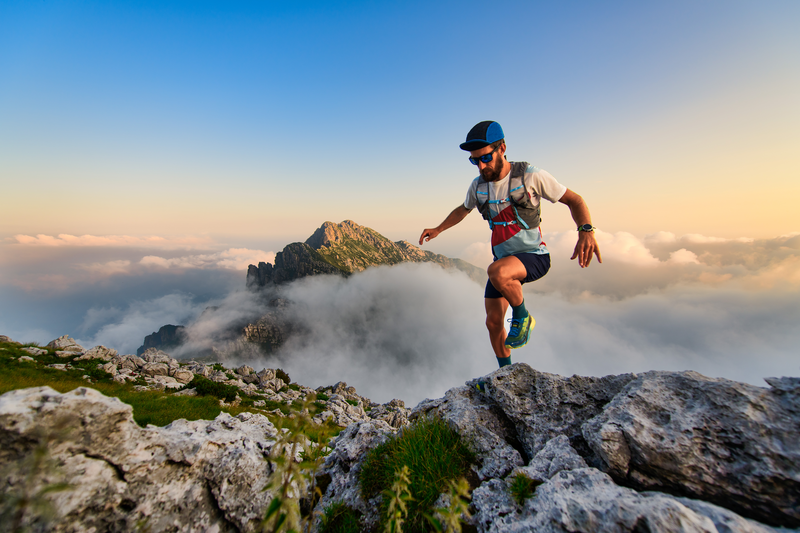
94% of researchers rate our articles as excellent or good
Learn more about the work of our research integrity team to safeguard the quality of each article we publish.
Find out more
ORIGINAL RESEARCH article
Front. Microbiol.
Sec. Microbe and Virus Interactions with Plants
Volume 16 - 2025 | doi: 10.3389/fmicb.2025.1570230
The final, formatted version of the article will be published soon.
You have multiple emails registered with Frontiers:
Please enter your email address:
If you already have an account, please login
You don't have a Frontiers account ? You can register here
Endophytic and rhizosphere microorganisms play crucial roles in influencing the quality and secondary metabolite accumulation of traditional Chinese medicinal. In this study, high-throughput sequencing technology was utilized to analyze the community structure and diversity of endophytic and rhizosphere microorganisms in Ardisia crenata Sims from seven distinct origins. The correlations analysis between soil physicochemical properties and active compounds was conducted to elucidate the role of these soil microorganisms in A. crenata Sims. A total of 8 514 557 high-quality reads were generated from 140 plant and soil sample in A. crenata Sims based on high-throughput sequencing. The fungal species composition within the endophytic and rhizosphere soil samples of A. crenata Sims is rich and varied, exhibiting notable disparities across different geographical regions of the plant. The alpha diversity and beta diversity indicated significant differences in microbial diversity and community structure between soil and plants. As for endophytic fungi, the dominant phyla in both plants and soil were Ascomycota and Basidiomycota, with different dominant genera between the two compartments. LEfSe analysis at the genus level identified 80 and 124 fungal indicator taxa associated with plants and soil, respectively, including Aspergillus, Acremonium, Fusarium, among others. Co-occurrence network analysis demonstrated intimate interactions among soil fungal microorganisms. Examination of soil physicochemical factors and the primary active constituent (bergenin) across different regions of A. crenata Sims indicated that the highest bergenin concentration is found in the Guangxi region, whereas the Guizhou region boasts relatively abundant soil
Keywords: Ardisia crenata Sims, rhizosphere soil, Endophytic, microorganisms, active compounds
Received: 03 Feb 2025; Accepted: 04 Mar 2025.
Copyright: © 2025 Liu, Luo, Yang, Liu, Zhou and Zhou. This is an open-access article distributed under the terms of the Creative Commons Attribution License (CC BY). The use, distribution or reproduction in other forums is permitted, provided the original author(s) or licensor are credited and that the original publication in this journal is cited, in accordance with accepted academic practice. No use, distribution or reproduction is permitted which does not comply with these terms.
* Correspondence:
Chang Liu, Guizhou University of Traditional Chinese Medicine, Guiyang, China
Disclaimer: All claims expressed in this article are solely those of the authors and do not necessarily represent those of their affiliated organizations, or those of the publisher, the editors and the reviewers. Any product that may be evaluated in this article or claim that may be made by its manufacturer is not guaranteed or endorsed by the publisher.
Research integrity at Frontiers
Learn more about the work of our research integrity team to safeguard the quality of each article we publish.