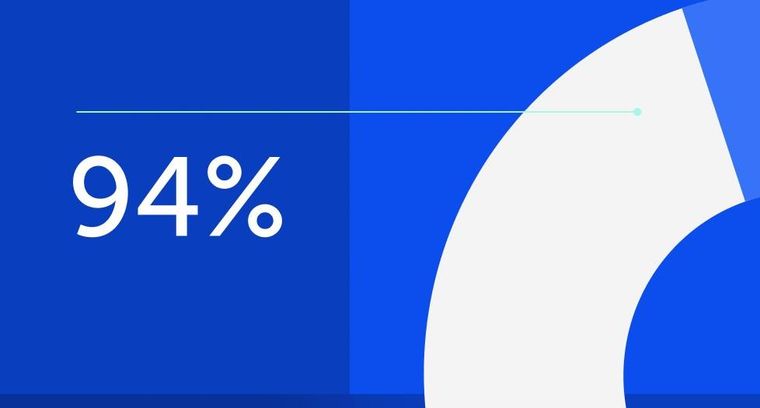
94% of researchers rate our articles as excellent or good
Learn more about the work of our research integrity team to safeguard the quality of each article we publish.
Find out more
REVIEW article
Front. Microbiol., 26 March 2025
Sec. Antimicrobials, Resistance and Chemotherapy
Volume 16 - 2025 | https://doi.org/10.3389/fmicb.2025.1568899
This article is part of the Research TopicEmerging Antimicrobials: Sources, Mechanisms of Action, Spectrum of Activity, Combination Antimicrobial Therapy, and Resistance MechanismsView all 22 articles
The increasing prevalence of antimicrobial resistance (AMR) presents a significant global health challenge, underscoring the urgent need for novel antimicrobial agents. Actinomycetes, particularly Streptomyces species, are well known for synthesizing bioactive compounds with antibacterial, antifungal, and antiviral properties. This review explores the diversity and antimicrobial potential of actinomycetes from Saudi Arabia’s unique ecosystems, including terrestrial (soil, rhizosphere), aquatic (marine, freshwater), extreme (deserts, caves, hot springs, mountains, and mangroves), and other unique environments. The adaptation of these microorganisms to harsh environmental conditions has driven the evolution of unique strains with enhanced biosynthetic capacities. Several studies have demonstrated their antimicrobial efficacy against multidrug-resistant pathogens, including methicillin-resistant Staphylococcus aureus (MRSA), extended-spectrum beta-lactamase (ESBL)-producing Enterobacteriaceae, Pseudomonas aeruginosa, and Candida albicans. However, challenges in actinomycete research persist, including difficulties in culturing rare strains, limited genomic characterization, and high production costs. Recent advancements, such as genome mining, metagenomics, AI-driven bioinformatics, and CRISPR-based gene activation, offer promising avenues for unlocking novel antimicrobial compounds. Additionally, synthetic biology, advanced fermentation technologies, and nanotechnology-based drug delivery systems are enhancing the industrial scalability of actinomycete-derived antibiotics. Beyond antimicrobials, actinomycete-derived compounds show potential applications in oncology, immunotherapy, and agriculture. Alternative therapeutic strategies, including quorum sensing inhibitors, phage therapy, and combination therapies, are being explored to combat AMR. Cutting-edge analytical techniques, such as mass spectrometry, liquid chromatography, and nuclear magnetic resonance spectroscopy (NMR), are essential for structural elucidation and mechanism characterization of new bioactive compounds. To harness Saudi Arabia’s microbial biodiversity effectively, interdisciplinary collaborations between microbiologists, biotechnologists, and pharmaceutical industries are crucial. Sustainable bioprospecting and advanced bioprocessing strategies will facilitate the translation of actinomycete-derived bioactive compounds into clinically viable therapeutics. Expanding research efforts into underexplored Saudi ecosystems can lead to groundbreaking discoveries in antibiotic development and beyond.
Antimicrobial resistance (AMR) has emerged as a critical global health challenge, significantly impacting infection management and clinical outcomes. The increasing prevalence of resistant microbial strains has led to heightened morbidity and mortality rates, particularly among vulnerable populations, including children, the elderly, and immunocompromised individuals. The World Health Organization (WHO) estimates that by 2050, AMR could lead to 10 million deaths annually, emphasizing the urgent need for novel antimicrobial agents (Alanis, 2005; Mancuso et al., 2021; Naghavi et al., 2024).
Multidrug-resistant (MDR) pathogens, including methicillin-resistant Staphylococcus aureus (MRSA) and vancomycin-resistant Staphylococcus aureus (VRSA), have emerged as critical concerns, alongside vancomycin-resistant Enterococcus spp. (VRE) and penicillin-resistant Streptococcus pneumoniae (PRSP), which further complicate treatment options. Extended-spectrum beta-lactamase (ESBL)- producing Escherichia coli (E. coli) and Klebsiella pneumoniae (K. pneumoniae) have been increasingly reported, alongside carbapenem-resistant Enterobacteriaceae (CRE), which exhibit resistance to last-line β-lactam antibiotics. Additionally, non-fermenting Gram-negative bacteria, including multidrug-resistant Pseudomonas aeruginosa (P. aeruginosa) and Acinetobacter baumannii (A. baumannii), have emerged as significant nosocomial pathogens with limited therapeutic options. This trend has been extensively documented in recent literature (Zowawi, 2016; Alotaibi, 2019; Thabit et al., 2023; Kiran et al., 2024). Moreover, the rise of multidrug-resistant Mycobacterium tuberculosis (MDR-TB) further exacerbates the global burden of antimicrobial resistance (Yezli and Memish, 2012).
Given the alarming rise in MDR pathogens and the diminishing efficacy of existing antibiotics, the search for novel antimicrobial compounds has become imperative. However, the decline in pharmaceutical investment in antibiotic discovery poses a major challenge to addressing this crisis. The extended time required for toxicological and clinical investigations, high costs, and lower profitability compared to other drug categories have significantly slowed the development of new antibiotics (Alenazi et al., 2023). As a result, researchers have increasingly turned to natural microbial biodiversity as a promising source of novel antimicrobial agents with potent bioactivity and minimal toxicity.
Among these microbial taxa, actinomycetes—particularly the genus Streptomyces—have historically been prolific producers of bioactive secondary metabolites, accounting for approximately 75% of clinically relevant antibiotics. While extensive research has focused on soil-derived actinomycetes, species isolated from extreme and underexplored environments have demonstrated exceptional potential for the biosynthesis of novel antimicrobial compounds (Aly et al., 2019; Al-Shaibani et al., 2021; Jagannathan et al., 2021; Alaidaroos, 2022). However, these niche ecosystems remain largely untapped, warranting further investigation.
In this context, exploring geographically unique and environmentally challenging habitats offers an opportunity to discover novel actinomycetes with distinct antimicrobial properties. Saudi Arabia’s diverse ecosystems—including deserts, coastal regions, caves, and mountainous terrains—constitute an underexplored reservoir of actinomycetes with significant antimicrobial potential (Al-Ghamdi et al., 2021; Almuhayawi et al., 2021; Albasri et al., 2024). The extreme environmental conditions of these habitats impose selective pressures that drive the evolution of unique biosynthetic gene clusters (BGCs), which may serve as promising sources for antibiotic discovery and biotechnological applications (Adegboye and Babalola, 2012). Despite their potential, actinomycetes from these distinct ecological niches remain largely uncharacterized.
This review aims to systematically assess the antimicrobial activities of actinomycetes from Saudi Arabia’s environments, elucidate novel bioactive compounds, and explore their applications in addressing antibiotic resistance. By bridging this knowledge gap, we seek to contribute to the identification of new therapeutic agents and advance our understanding of microbial diversity in challenging ecological niches.
This systematic review was conducted following the Preferred Reporting Items for Systematic Reviews and Meta-Analyses (PRISMA) guidelines to ensure transparency and rigor. A comprehensive literature search was performed across multiple scientific databases, including PubMed, Scopus, Web of Science, ScienceDirect, and Google Scholar, to identify relevant studies on actinomycetes in Saudi Arabia. Additionally, reference lists of selected articles were manually screened for additional sources. Relevant scientific books were also consulted to provide historical and foundational insights.
The search terms used included:
• “Actinomycetes in Saudi Arabia”
• “Antimicrobial properties of actinomycetes”
• “Antimicrobial resistance”
• “Extremophilic actinomycetes”
• “Bioactive compounds from actinomycetes”
The literature search covered studies published up to 2024 to ensure the inclusion of the most recent and relevant findings.
The inclusion and exclusion criteria were established to ensure the selection of high-quality and relevant studies.
Inclusion Criteria:
1. Peer-reviewed publications focusing on actinomycetes from Saudi Arabia.
2. Research on the antimicrobial activity and bioactive compound production of these isolates.
3. Experimental studies involving isolation, characterization, and bioactivity screening of actinomycetes.
4. Studies of antimicrobial resistance in Saudi Arabia to provide context for the significance of new antimicrobial agents.
5. Comparative studies that include actinomycetes from multiple environments (e.g., terrestrial, aquatic, extreme habitats).
6. Articles published in English.
Exclusion Criteria:
1. Non-peer-reviewed sources, including conference abstracts and opinion articles.
2. Studies lacking clear and comprehensive data presentation.
3. Studies irrelevant to the core focus of this review.
4. Articles with limited accessibility or those not available through major scientific databases.
The study selection process followed the PRISMA flowchart approach:
1. Identification: All retrieved records from the database searches were compiled, and duplicates were removed.
2. Screening: Titles and abstracts were reviewed to exclude irrelevant studies.
3. Eligibility Assessment: Full-text articles were assessed against the inclusion and exclusion criteria.
4. Inclusion: Eligible studies were included in the final qualitative and quantitative synthesis.
A standardized data extraction sheet was used to collect information from the selected studies. Extracted data included:
• Study details (author, year of publication)
• Sampling locations
• Identified actinomycete strains
• Antimicrobial activity (spectrum and potency)
• Bioactive compounds detected
• Methods used for antimicrobial activity assessment
A comparative analysis was conducted to identify trends in actinomycete diversity, antimicrobial properties, and their distribution across various environments in Saudi Arabia. The findings were synthesized to highlight the ecological and pharmacological significance of these microorganisms.
To ensure the reliability and validity of the included studies, a risk of bias assessment was performed using appropriate tools based on study design. Studies were evaluated for methodological quality, completeness of data reporting, and potential conflicts of interest. The results of the risk of bias assessment were documented to account for variations in study quality.
By adopting the PRISMA framework, this systematic review aims to provide a comprehensive and transparent synthesis of the available literature on actinomycetes in Saudi Arabia.
Actinomycetes are a diverse group of Gram-positive bacteria classified under the phylum Actinomycetota within the domain Bacteria.1 These microorganisms are characterized by a high guanine-cytosine (G + C) content in their genomic DNA, which contributes to their genetic stability and adaptability. The term “actinomycetes” was derived from the Greek words “aktis” (a ray) and “mykes” (fungus), reflecting their filamentous morphology (Chaudhary et al., 2013; Siro and Pipite, 2024). Historically, actinomycete were considered transitional form between bacteria and fungi due to their mycelial growth pattern. However, they are definitively classified as prokaryotes based on their cellular organization, possessing nuclei without a membrane-bound structure and a peptidoglycan-based cell wall. Their structural and physiological diversity is extensive, encompassing filamentous and non-filamentous forms, motile and non-motile variants, as well as aerobic and anaerobic species. Furthermore, they exhibit variability in their reproductive strategies, forming either spores or non-spore-bearing structures. A distinguishing characteristic of actinomycetes is their ability to develop both aerial and substrate mycelia, along with structures such as sporangia and conidial chains, which enhance their survival and adaptation in extreme environments. Their colonies typically exhibit a powdery texture and adhere firmly to the agar surface, a feature that aids in their identification and differentiation from other bacterial taxa (Goodfellow et al., 2012). Additionally, actinomycetes demonstrate intrinsic resistance to various antibacterial agents, a trait that has contributed to their significance in biotechnology and antibiotic production.
Actinomycetes are recognized for their ability to produce bioactive secondary metabolites that exhibit a diverse array of biological effects, including antibacterial, antifungal, antiviral, antiparasitic, anticancer, and immunosuppressant effects, as well as enzymes and other bioactive compounds (Bérdy, 2005; Kekuda et al., 2010; Chaudhary et al., 2013; Jagannathan et al., 2021). These compounds have wide-ranging applications in environmental, agricultural, industrial, pharmaceutical, and clinical fields (Figure 1). Their biosynthetic potential is largely attributed to BGCs encoding polyketide synthases (PKSs), nonribosomal peptide synthetases (NRPSs), and other enzymes involved in the production of bioactive compounds (Adegboye and Babalola, 2012). Recent advancements in comparative genomics have significantly enhanced the discovery and optimization of antibiotic biosynthetic pathways, providing new insights into their metabolic potential. Moreover, metagenomics and synthetic biology approaches have emerged as powerful tools for uncovering novel secondary metabolites and improving their yield through genetic modifications (Mitousis et al., 2020; van Bergeijk et al., 2020; Jagannathan et al., 2021).
Among the approximately 22,000 known microbial secondary metabolites, actinomycetes account for 70–75% of these bioactive compounds, with fungi contributing around 20%, Bacillus species 7%, and other bacterial taxa collectively representing only 1–3%. In terms of antibiotic production, actinomycetes are responsible for approximately 50–55% of all clinically established antibiotics. Within this group, the genus Streptomyces contributes nearly 75% of these compounds, while the remaining 25% are produced by non-Streptomyces species, commonly referred to as “rare actinomycetes” (Bawazir et al., 2019; Dimri et al., 2020; Ngamcharungchit et al., 2023).
Rare actinomycetes include genera distinct from Streptomyces or those infrequently isolated under standard laboratory conditions. These genera include Actinomadura, Actinoplanes, Amycolatopsis, Actinokineospora, Acrocarpospora, Actinosynnema, Actinopolyspora, Actinoalloteichus, Catenuloplanes, Cryptosporangium, Dermabacter, Dactylosporangium, Frankia, Kytococcus, Kibdelosporangium, Kineosporia, Kutzneria, Microbacterium, Micromonospora, Microbiospora, Microtetraspora, Micropolyspora, Nocardia, Nocardiopsis, Nonomuraea, Planomonospora, Planobispora, Pseudonocardia, Rhodococcus, Saccharomonospora, Saccharopolyspora, Saccharothrix, Streptosporangium, Streptoverticillium, Spirilliplanes, Salinispora, Thermoactinomyces, Thermomonospora, Thermopolyspora, Thermobifida, Verrucosispora, and Virgosporangium (Lazzarini et al., 2000; Khanna et al., 2011; Tiwari and Gupta, 2012; Tiwari and Gupta, 2013; Hug et al., 2018). These taxa are increasingly recognized as valuable sources of novel bioactive compounds, particularly considering the growing challenge of antimicrobial resistance.
Actinomycetes have historically been the primary source of clinically important antimicrobial agents, including β-lactams (e.g., cephamycin), polyketides, glycopeptides (e.g., vancomycin), ansamycins (e.g., rifamycin), macrolides (e.g., erythromycin), tetracycline, aminoglycosides, chloramphenicol, quinolones, sulfonamides, polyenes, polyethers, nucleosides, lipopeptides (e.g., daptomycin), peptides, and numerous other bioactive molecules (Baltz, 2007; Mahajan and Balachandran, 2012; Alharbi, 2016; Barka et al., 2016; De Simeis and Serra, 2021). Among actinomycetes, Streptomyces species have been particularly prolific in producing antimycobacterial agents, with many compounds exhibiting potent activity against Mycobacterium species. Notable examples include caprazamycin B, sansanmycins, urdamycinone E, urdamycinone G, dehydroxyaquayamycin, streptcytosine A, chrysomycin A, and dinactin (Alharbi, 2016; Al-Dhabi et al., 2020a). These metabolites underscore the potential of Streptomyces and other actinomycetes as invaluable reservoirs for the discovery of novel antimicrobial agents.
Actinomycetes are ubiquitous microorganisms present in several ecosystems, including terrestrial, aquatic, and extremophilic environments (Figure 2). They live as free-living saprophytic bacteria. Some isolates can live inside the tissues of plants, insects, or aquatic animals. They adapt well to a range of conditions, including usual and harsh habitats characterized by elevated or reduced temperatures, intense radiation, acidic or alkaline pH levels, high salinity, and settings with restricted moisture or nutrients. These organisms have important applications in biotechnology and medicine due to their ability to produce a variety of bioactive compounds with antimicrobial activity (Zenova et al., 2011; Meenakshi et al., 2024).
Actinomycetes are primarily free-living microorganisms located in diverse habitats. They are also well known as soil saprophytes and are responsible for the distinctive earthy scent of freshly plowed soil due to the synthesis of geosmin (Sharma et al., 2014). Among soil actinomycetes, Streptomyces is the most dominant genus. However, the diversity and bioactivity of actinomycetes are significantly influenced by environmental factors such as geographical location, soil temperature, type, pH, organic matter content, agricultural activities, aeration, nutrient availability, moisture content, and surrounding vegetation (Arifuzzaman et al., 2010). They are especially prevalent in slightly alkaline organic rich soils, where they synthesize diverse secondary metabolites essential for medical and agricultural applications. Their ecological roles extend beyond nutrient cycling to include nitrogen fixation (e.g., Frankia sp.), solubilization and immobilization of nutrients, siderophore production, and biocontrol activity against soil-borne pathogens (Dilip et al., 2013).
Numerous studies across different regions have underscored the significant antimicrobial potential of soil-derived actinomycetes. Studies from India (Pandey et al., 2011; Patel et al., 2014) have identified diverse soil-derived actinomycetes strains from agricultural soils, riversides and grassland areas, often linked to high organic content and moderate climate conditions producing antimicrobial compounds against multidrug-resistant pathogens such as Staphylococcus aureus (S. aureus), E. coli, and P. aeruginosa, and antifungal properties. Similar findings have been reported from Egypt (Elbendary et al., 2018; Sebak et al., 2021), identified actinomycetes that exhibit inhibitory effects against a wide array of pathogens, including S. aureus, MRSA, Bacillus cereus (B. cereus), E. coli, K. pneumoniae, P. aeruginosa, Salmonella typhi (S. typhi), Candida albicans (C. albicans), Aspergillus niger (A. niger), and Aspergillus flavus (A. flavus). Recent work by Atallah et al. (2023a) identified Streptomyces rochei (S. rochei), which exhibited significant antimicrobial activities against Bacillus subtilis (B. subtilis), Salmonella enteritidis (S. enteritidis), and P. aeruginosa. Awad et al. (2024) reported similar findings, with actinomycetes exhibiting antibacterial effects against S. aureus, MRSA, and P. aeruginosa, as well as antifungal activity, particularly against Aspergillus fumigatus (A. fumigatus) and Fusarium solani (F. solani).
Unlike temperate and tropical regions, Saudi Arabia soils exhibit environmental conditions, including high temperatures, aridity, and variable salinity, favoring the survival of stress-tolerant actinomycetes with specialized secondary metabolites (Alharbi et al., 2012; Al-Kadeeb et al., 2012; Mohamed et al., 2013b). Similar ecological trends have been observed in actinomycetes from the Atacama Desert, where extremophilic strains have demonstrated unique biosynthetic capabilities, producing compounds distinct from those of mesophilic strains (Okoro et al., 2009; Rateb et al., 2011).
Numerous investigations in Saudi Arabia have proven the significant antimicrobial potential of soil-derived actinomycetes (Table 1). The discovery of Streptomyces flavogriseus from agricultural soils in Riyadh and Qassim (Al-Kahtani, 2016) and S. rochei from Al-Kharj (Muharram et al., 2013) showed broad-spectrum antimicrobial activity against Gram-positive bacteria such as S. aureus and B. subtilis, as well as Gram-negative bacteria like E. coli and antifungal activity against C. albicans and A. niger. More recently, Ibnouf et al. (2022) documented additional antimicrobial-producing actinomycetes from Al-Kharj, isolating bioactive compounds with diverse medical uses, including antibacterial, antifungal, anti-inflammatory, antioxidant, and anti-cancer characteristics. Some of these compounds also serve as essential ingredients in cosmetics. Additionally, Ababutain et al. (2012a, 2012b) identified a Streptomyces strain from Dammam synthesizing lincomycin-like antibiotics, effective against both Gram-positive and Gram-negative bacteria, as well as insects. Abid et al. (2016) recovered Streptomyces strains from Jazan with activity against bacteria like B. subtilis, S. aureus, P. aeruginosa, E. coli, and Shigella sonnei (S. sonnei) with inhibition zones ranging from 3.25 to 26.875 mm in diameter. In terms of antifungal activity, these strains were effective against Fusarium moniliforme, Helminthosporium oryzae, A. flavus, Aspergillus japonicus, and Fusarium verticillioides with an inhibition zone ranging from 13.3 to 40 mm. Similar findings were reported by Sheik et al. (2017) in Ad-Dawadmi and Jastaniah et al. (2019b) in Jeddah, where Streptomyces spp. showed antibacterial efficacy against E. coli, K. pneumoniae, P. aeruginosa, S. aureus, MRSA, and Enterococcus faecalis (E. faecalis). These investigations underscore the pharmaceutical potential of Saudi soil-derived actinomycetes, especially Streptomyces, as valuable sources of innovative antimicrobial compounds. The diversity of these isolates and their extensive efficacy against antibiotic-resistant bacteria and harmful fungi highlight their significance in combating the global issue of antimicrobial resistance.
The rhizosphere—the soil microzone surrounding plant roots—is a crucial ecological niche where actinomycetes play a significant role in nutrient cycling, organic matter decomposition, and suppression of soil-borne pathogens. Through complex interactions with plant roots, rhizosphere actinomycetes enhance soil fertility, plant health, and resistance against pathogenic microbes (Adegboye and Babalola, 2012). This symbiotic interaction is essential for fostering sustainable agriculture by diminishing the necessity for chemical-based fertilizers and pesticides. In addition to their agricultural benefits, many rhizosphere-derived actinomycetes, especially from genera such as Streptomyces, Micromonospora, Microbispora, Nocardiopsis, Rhodococcus, and Nocardia, synthesize bioactive compounds with antibacterial, antifungal, antiviral, and anticancer properties (Matsumoto and Takahashi, 2017; Singh and Dubey, 2018; Alzahrani et al., 2022).
Extensive global studies have demonstrated the role of rhizosphere actinomycetes in plant growth promotion and phytopathogen control (Gohain et al., 2015; Refaat et al., 2017; Elsayed et al., 2020). Streptomyces sp. CAH29 from the rhizosphere has yielded bioactive molecules such as tetrangomycin, which exhibits antibacterial and antifungal activity against S. aureus, Streptococcus pyogenes (S. pyogenes), MRSA, and C. albicans, with inhibition zones of 14, 10, 12, and 8 mm, respectively (Özakin et al., 2016). Similarly, picolinamycin, isolated from an Indian Streptomyces strain, demonstrated significant antibacterial activity against S. aureus with minimum inhibitory concentration (MIC) of 0.04–5.12 μg/mL, and moderate anti-mycobacterial effects at higher concentrations (MIC: 10.24 μg/mL) (Maiti et al., 2020).
In contrast, research in Saudi Arabia has primarily focused on the diversity and characterization of rhizosphere actinomycetes, with limited studies investigating their role as biocontrol agents. However, emerging evidence suggests that these microorganisms could serve as promising candidates for agricultural applications. Ahmed et al. (2021) obtained actinomycetes strains from the rhizosphere of Satureja hortensis in Jouf area of Saudi Arabia. The phenolic content of these strains exhibited significant antioxidant, antibacterial, and antiprotozoal action against Trypanosoma cruzi (T. cruzi). Similarly, Streptomyces spororaveus RDS28, recovered from rhizosphere soils in Riyadh, exhibited broad-spectrum antifungal activity (Al-Askar et al., 2011). Further, Streptomyces griseorubens E44G demonstrated potent antagonism against Fusarium oxysporum (F. oxysporum) (Al-Askar et al., 2014). More recently, Streptomyces abyssalis SHR13, isolated from rhizosphere soils in Jeddah and Yanbu Al-Nakheel, exhibited inhibitory activity against F. oxysporum, A. niger, K. pneumoniae, and P. aeruginosa (Khallaf et al., 2023).
Additional studies in Saudi Arabia have expanded the scope of rhizosphere actinomycete research. Streptomyces ramulosus, identified from the catnip rhizosphere in Al-Madinah Al-Munawwarah, demonstrated antibacterial action against both Gram-negative and Gram-positive pathogens (Abo-Shadi et al., 2010). Furthermore, Streptomyces flavogriseus (KF235416), isolated from Acacia tortilis rhizosphere soil, exhibited potent antimicrobial activity against a range of pathogens, MRSA, VRE, A. baumannii, S. pyogenes, E. coli, Salmonella typhimurium (S. typhimurium), and Shigella spp., in addition to fungi such as Aspergillus spp. and C. albicans (Al-Garni et al., 2014). These findings suggest that Saudi Arabia harbors untapped biocontrol agents for agricultural use. Table 2 shows the diversity and antimicrobial activity of actinomycetes from rhizosphere soil in Saudi Arabia.
Table 2. Diversity and antimicrobial activity of rhizosphere soil -derived actinomycetes in Saudi Arabia.
While global studies have led to significant antibiotic discoveries, and actinomycete-based biofertilizers and biopesticides have been developed and commercialized, Saudi research remains in a developmental phase, with great potential for future biotechnological applications. Addressing underexplored environments- rhizosphere of native Saudi plants, such as Acacia and Zygophyllum species-could unlock novel actinomycetes with specialized bioactive metabolites, contributing to global efforts in drug discovery and sustainable agriculture.
Actinomycetes from aquatic habitats have historically received less attention compared to their terrestrial counterparts. However, these microorganisms, known for their prolific production of bioactive compounds, have been extensively isolated from both freshwater and marine environments (Aly et al., 2019; Al-Shaibani et al., 2021) (see Table 3).
Marine ecosystems, encompassing over two-thirds of the surface of the earth, are distinguished by significant variations in the temperature, salinity, nutrient availability, oxygen levels, and pressure. These factors contribute to the extensive microbial diversity of marine habitats and influence the genetic adaptation of marine actinomycetes, leading to the biosynthesis of distinct secondary metabolites that differ from those of their terrestrial counterparts (Subramani and Sipkema, 2019; Mothana et al., 2022; Chakraborty et al., 2023). The successful isolation of rare marine actinomycetes requires a comprehensive understanding and precise control of key environmental parameters, including pH, culture temperature, oxygen levels, and nutritional requirements. To optimize the cultivation of these microorganisms, growth media should closely mimic the osmotic conditions of seawater. Notably, sodium ions (Na+) represent a critical component in culture media, as they are essential for the growth and physiological activity of marine actinomycetes (Subramani and Sipkema, 2019).
While earlier studies questioned whether marine actinomycetes were merely terrestrial contaminants, subsequent research has confirmed the existence of indigenous marine actinomycetes that require seawater for optimal growth (Fenical and Jensen, 2006). Marine actinomycetes belong to a diverse array of genera, including Actinoplanes, Actinomycetospora, Agrococcus, Aeromicrobium, Arsenicicoccus, Arthrobacter, Brevibacterium, Citricoccus, Dietzia, Geodermatophilus, Janibacter, Kocuria, Microbacterium, Microlunatus, Micromonospora, Nocardia, Nocardioides, Nocardiopsis, Rhodococcus, Salinispora, Sciscionella, Serinicoccus, Saccharopolyspora, Salinibacterium, Streptomyces, and Thermoactinomyces (Subramani and Aalbersberg, 2013; Alharbi, 2016; Bawazir et al., 2019; Subramani and Sipkema, 2019). Notably, marine-derived actinomycetes produce a wide range of novel bioactive compounds, including pacificanones, salinipyrones, rifamycins, arenimycin, cyanosporaside A, saliniketals A and B, marinomycin A–D, abyssomicin-C, and others, which exhibit antimicrobial, antifungal, and anticancer properties, underscoring their pharmaceutical potential (Subramani and Aalbersberg, 2012; Subramani and Sipkema, 2019).
Marine sediments are recognized as the primary reservoir of actinomycetes; however, they have also been isolated from diverse marine environments, including seawater, sand, rocks, and marine flora and fauna such as sponges (Abdelmohsen et al., 2014), corals (Mahmoud and Kalendar, 2016), seagrass (Siro and Pipite, 2024), fish (Jagannathan et al., 2021), mollusks (Dilip et al., 2013), shrimps (Ali et al., 2020), and algae (El-Gendy et al., 2008).
Several studies have highlighted the significant antimicrobial efficacy of marine actinomycetes against MDR bacterial and fungal pathogens, demonstrating their potential as a valuable source of novel antimicrobial agents (Valli et al., 2012; Hegazy et al., 2023). For instance, actinomycetes isolated from the Bay of Bengal, predominantly Streptomyces species, displayed potent antimicrobial activity against S. aureus, S. typhi, B. cereus, E. coli, K. pneumoniae, and phytopathogenic fungi (Kalyani et al., 2012; Mohanraj and Sekar, 2013). Similarly, Streptomyces griseorubens and S. rochei, recovered from the black sand shores of Egypt, exhibited strong antimicrobial activity against MDR pathogens such as B. subtilis, S. enteritidis, and P. aeruginosa (Atallah et al., 2023b). Additionally, Ribeiro et al. (2020) isolated 52 actinomycetes from marine sediments along the Portuguese coast, primarily from the Micromonospora genus. Six Streptomyces strains exhibited antifungal activity against C. albicans, with MIC values ranging from 3.9 to 125 μg/mL.
Saudi Arabia’s marine environments, including the Red Sea and the Arabian Gulf, along with various inland freshwater bodies (wadis, oases, and artificial lakes), present extreme conditions that influence microbial diversity and metabolic adaptations. The Red Sea and Arabian Gulf, in particular, harbor halotolerant actinomycetes that have adapted to high salinity and fluctuating temperatures. For example, Actinopolyspora saudiensis sp. nov., a halophilic actinomycete isolated from hypersaline soil in Jeddah, exhibits exceptional salt tolerance, thriving at NaCl concentrations of up to 30%, underscoring its potential in biotechnological uses in saline conditions (Kiki and Al Turk, 2013). Several studies have demonstrated the antimicrobial potential of marine actinomycetes from these environments (Nadeem et al., 2015; Abdelfattah et al., 2016; El Samak et al., 2018; Hamed et al., 2019). Marine-derived Salinispora and Micromonospora strains have been identified as prolific producers of bioactive metabolites with potent antifungal and antibacterial properties, presenting promising leads for antibiotic development (Mahmoud and Kalendar, 2016; Alqahtani et al., 2022; Mothana et al., 2022).
Additionally, actinomycetes isolated from the Red Sea have exhibited remarkable antimicrobial activity. Aly et al. (2011) recovered 90 actinomycete strains from soil, marine sediments, seawater, wastewater, marine shrimp, and well water in Jeddah. Among these, 10 isolates displayed strong inhibitory effects against C. albicans, with Streptomyces sp. BM21 producing a protein that disrupted fungal cell wall regeneration. Similarly, Mohamed et al. (2013a) identified 18 Streptomyces-like isolates from Red Sea sediments, demonstrating substantial antimicrobial activity. Further investigations by Al-Garni et al. (2014) resulted in the isolation of 81 actinomycete strains from Jeddah and Al-Madinah Al-Munawwarah, many of which exhibited broad-spectrum antimicrobial properties. Notably, Streptomyces sp. Al-Dhabi-100 from Jazan demonstrated potent antibacterial activity against E. faecalis, B. subtilis, and S. aureus, along with anti-tubercular and antioxidant properties (Al-Dhabi et al., 2020a). Marine sponge-associated actinomycetes from the Red Sea have also proven to be a valuable source of bioactive compounds. Abdelmohsen et al. (2014) isolated 48 actinomycetes strains, with extracts from 9 strains showing significant antibacterial, antifungal, antiparasitic, and antiviral activities. Notably, two strains, identified as Salinispora spp., exhibited broad-spectrum inhibition against multiple pathogenic microorganisms. Similarly, Abdelmohsen et al. (2010) also explored the marine environment, isolating 90 actinomycetes strains from sponges collected near Ras Mohamed, Egypt, and Rovinj, Croatia. Additionally, Shamikh et al. (2020) focused on actinomycetes associated with marine sponges collected from Hurghada, Egypt. This study identified novel species from the genera Micromonospora, Nocardia, and Gordonia, which showed activity against S. aureus, E. faecalis, C. albicans, and Trypanosoma brucei.
The Arabian Gulf has similarly yielded actinomycetes with promising biomedical applications. Streptomyces sp. Al-Dhabi-89, isolated from Dammam, demonstrated potent antibacterial activity against MDR pathogens such as E. coli, K. pneumoniae, P. aeruginosa, A. baumannii, Proteus mirabilis (P. mirabilis), multidrug-resistant S. aureus and Enterococcus faecium (E. faecium), with MIC values ranging from 7.81 to 62.5 μg/mL (Al-Dhabi et al., 2018). Likewise, Streptomyces sp. Al-Dhabi-90 exhibited antibacterial activity against vancomycin-resistant Enterococcus faecium and ESBL-producing E. coli and P. aeruginosa (Al-Dhabi et al., 2019b). Moreover, Streptomyces sp. Al-Dhabi-97, isolated from Saudi Arabian seashores, displayed broad-spectrum antimicrobial activity, with MIC values ranging from 62.5 to 500 μg/mL (Al-Dhabi et al., 2020b).
These findings highlight the vast biotechnological potential of marine actinomycetes from Saudi Arabia’s aquatic ecosystems, reinforcing their role as promising sources for novel antimicrobial agents and pharmaceutical applications.
While marine ecosystems, with their high salinity and nutrient limitations, provide an environment for extremophilic actinomycetes, Saudi Arabia’s freshwater ecosystems—including seasonal wadis, oases, artificial lakes, dams, and underground aquifers— remain largely unexplored despite their potential to harbor diverse microbial communities. The seasonal fluctuations in water availability and salinity within these freshwater environments may contribute to microbial diversity, including actinomycetes with significant biotechnological potential.
Although marine actinomycetes have been extensively studied, freshwater environments have also been recognized as reservoirs of bioactive actinomycetes. Several studies have reported the isolation of actinomycetes from freshwater sources with potent antimicrobial activities. For instance, Streptomyces viridiviolaceus, isolated from Lake Bardawil in Egypt, exhibited broad-spectrum antibacterial activity (Rabeh et al., 2007). Similarly, genera such as Saccharopolyspora and Actinosynnema, isolated from freshwater sources in South Africa, demonstrated antimicrobial potential against both Gram-positive and Gram-negative bacteria (Sibanda et al., 2010). Further evidence from the Nile River in Egypt (Aly and El-Sabbagh, 2004), Lake Fetzara in Algeria (Benhadj et al., 2019), Lonar Lake in India (Kharat et al., 2009), and Lake Tana in Ethiopia (Gebreyohannes et al., 2013) has underscored the presence of actinomycetes producing bioactive compounds with antibacterial, antifungal, and anticancer properties.
Given the ecological dynamics of Saudi Arabia’s freshwater ecosystems, comprehensive multi-seasonal sampling and genomic studies are necessary to elucidate actinomycete diversity under varying hydrological conditions. Such investigations would facilitate the identification of novel bioactive compounds, advancing our understanding of their potential applications in medicine and biotechnology.
Actinomycetes are known to occur in typical conditions, as well as in extreme and underexplored environments, including deserts (Li et al., 2019), caves (Farda et al., 2022), hot springs (AlSediy et al., 2022), eruptions of volcanoes (Ruwandeepika et al., 2022), mangroves (Law et al., 2019), mountainous areas (Albasri et al., 2024), thermal industrial wastes (Alaidaroos, 2022), Arctic and Antarctic regions (Augustine et al., 2012; Encheva-Malinova et al., 2014). Their ability to survive in such conditions is attributed to their physiological and metabolic flexibility, including the formation of resistant spores (arthrospores) and the production of secondary metabolites that facilitate survival (Shivlata and Satyanarayana, 2015). Notably, unexplored extreme habitats often yield novel and rare actinomycete isolates with antimicrobial properties (Aly et al., 2019). However, variations in environmental factors, isolation techniques, and screening methodologies significantly influence the diversity of isolated actinomycetes and the antimicrobial activity they exhibit.
Table 4 summarizes the diversity and antimicrobial activity of actinomycetes from extremophilic environments in Saudi Arabia.
Table 4. Diversity and antimicrobial activity of actinomycetes from extremophilic environments in Saudi Arabia.
Desert ecosystems, characterized by extreme temperatures, low humidity, and scarce nutrient availability, represent an untapped source of novel microbial species and bioactive compounds. Actinomycetes in desert environments have demonstrated significant antimicrobial potential, particularly in producing antibiotics against multidrug-resistant pathogens. The methodology used for their isolation can significantly impact the recovery of bioactive strains. Pre-treatment techniques, such as heat treatment, dry-air exposure, and chemical enrichment using calcium carbonate, can selectively enrich for specific actinomycete populations while inhibiting the growth of competing microbes. Heat treatment, for example, eliminates non-spore-forming bacteria, thereby enhancing the isolation of thermophilic actinomycetes (Subramani and Aalbersberg, 2013). The choice of culture media also plays a crucial role in the metabolic expression of actinomycetes, as nutrient-rich media may favor fast-growing species, potentially overlooking slow-growing or rare actinomycetes (Nithya et al., 2015; Atta et al., 2010). Starch casein agar is widely used for isolating Streptomyces species due to its ability to support sporulation, whereas minimal media can promote the production of stress-induced metabolites not typically expressed in nutrient-rich conditions. Temperature fluctuations in deserts also induce physiological adaptations that enhance secondary metabolite production, often leading to bioactive compounds with unique structures (Almuhayawi et al., 2021).
Studies from various desert regions have underscored the diversity and antimicrobial potential of actinomycetes. In Egypt, Hozzein et al. (2011) identified diverse actinomycetes with notable antimicrobial activity. Ziyat et al. (2019) found that approximately 50% of actinomycetes isolated from Kazakhstan’s deserts exhibited antibacterial efficacy. In Pakistan, Fatima et al. (2019) isolated 110 actinomycete strains, with several demonstrating strong activity against MRSA. Similarly, actinomycetes isolated from Iran’s arid soils showed significant antimicrobial activity (Majidzadeh et al., 2021). Saleem et al. (2023) reported that actinomycetes from China’s Kubuqi Desert exhibited antimicrobial activity against multiple bacterial and fungal pathogens such as S. aureus, Micrococcus luteus (M. luteus), B. subtilis, E. coli, Salmonella enterica (S. enterica), and Saccharomyces cerevisiae (S. cerevisiae).
Despite these findings, research on actinomycetes from Saudi Arabia’s desert habitats—covering around 95% of the country—remains limited. The Rub’ al Khali (Empty Quarter) and An Nafud Desert, among the hottest and driest locations on earth, present unique conditions for microbial adaptation. Actinomycetes isolated from these regions demonstrate remarkable thermotolerance and antimicrobial potential that could address antibiotic resistance and meet the demand for new antimicrobial agents (Alotaibi et al., 2020; Almuhayawi et al., 2021; Alzahrani, 2021). For instance, a thermophilic strain, Thermomonospora spp. T-SA-125, obtained from desert soil in Saudi Arabia, produced a water-soluble antibiotic potent against Gram-positive as well as Gram-negative bacteria (Dewedar et al., 1979). Streptomyces jeddahensis sp. nov., isolated near Jeddah, withstands temperatures up to 50°C (Röttig et al., 2017). Alghamdi et al. (2021) identified actinomycetes from Jeddah and Baljurashi soil samples, with some closely related to Streptomyces species showing antibacterial activity, specifically against S. aureus, Staphylococcus epidermidis (S. epidermidis), and B. subtilis. Atta et al. (2010) identified Streptomyces torulosus KH-4 from the Al-Khurmah desert and as notably effective against both Gram-positive and Gram-negative bacteria, as well as fungi. In a similar manner, Shinwari et al. (2013) identified Streptomyces species, isolated from soils of the Saudi desert. Most of these isolates exhibited significant antimicrobial activity against pathogens such as S. aureus, B. subtilis, E. coli, P. aeruginosa, Salmonella suis, S. sonnei, and C. albicans. In another study, Nithya et al. (2018) revealed that a crude extract from Streptomyces sp. DA3-7 obtained from a desert in Riyadh had antibacterial and cytotoxic properties.
Recent Saudi research continues to uncover promising desert-derived actinomycetes. Alkhelaiwi et al. (2021) isolated 30 actinomycetes strains (F1-30) from soil samples obtained from the Al Thumamah area of Saudi Arabia. Ten strains exhibited antimicrobial activity against at least one pathogen. Almuhayawi et al. (2021) isolated 21 actinomycetes from the Al-Jouf desert in Saudi Arabia. The isolates, mostly Streptomyces species, generated a substantial quantity of bioactive compounds exhibiting notable antioxidant, antibacterial, and antiprotozoal activities against T. cruzi. Desert plant associations impact actinomycetes communities. Root-associated actinomycetes exhibit increased antimicrobial activity due to plant-microbe interactions. Hausawi et al. (2021) discovered Streptomyces species from desert soil surrounding various plants in Makkah, Saudi Arabia. These isolates exhibited activity against pathogens including Streptococcus pneumoniae (S. pneumoniae), MRSA, S. aureus, S. pyogenes, A. niger, and C. albicans, indicating their potential as novel antibiotic sources for medicinal use. Additionally, Al-Khamash et al. (2023) found that several plant-associated actinomycetes strains, collected from the Al Thumama desert in the Riyadh region of Saudi Arabia, showed antimicrobial activity against K. pneumoniae, B. subtilis, and C. albicans.
Desert-derived actinomycetes represent an emerging frontier in microbial biotechnology. While Saudi Arabia’s deserts hold immense potential for novel antibiotic discovery, variations in isolation techniques and environmental factors significantly impact microbial diversity and bioactivity. Addressing these variables through standardized methodologies and advanced genomic approaches to reveal biosynthetic gene clusters responsible for antibiotic production will pave the way for more consistent and impactful discoveries.
Cave environments hold great potential for discovering new antimicrobial compounds due to the intense competition among microorganisms for limited nutrients. This competition drives the production of antimicrobial substances as a survival mechanism. For example, Streptomyces isolated from volcanic caves has been shown to produce high levels of secondary metabolites. Unique cave conditions—such as reduced light, high humidity, low nutrient availability, high concentration of minerals (carbonate, sulphates, phosphates, and potassium), acidity, and lack of oxygen— enhance microbial production of these compounds (Jones, 2001; Cheeptham, 2012; Farda et al., 2022). Recent years have witnessed an increasing interest in the examination of microbial diversity in caves owing to their harsh environments (Groth et al., 2002; Duangmal et al., 2012). Actinomycetes, prevalent in these ecosystems, are particularly attractive sources for novel drug discoveries, demonstrating potential for pharmaceutical applications (Rangseekaew and Pathom-Aree, 2019; Farda et al., 2022). Yücel and Yamaç (2010) reported that Streptomyces sp. 1,492, isolated from Turkish karstic caves, exhibited antimicrobial activity against multidrug-resistant bacteria such as MRSA and A. baumannii. Similarly, Streptomyces badius (S142) and Actinoplanes friuliensis (S761) isolated from soil, rock-soil, and bat manure of Shuanghe Cave in China were found to inhibit various pathogenic microorganisms (Long et al., 2019). A comprehensive study in Hampoeil Cave, Iran, identified 76 actinobacterial strains, including genera such as Streptomyces, Micromonospora, Micrococcus, Kocuria, and Corynebacterium. Of these, 25.3% exhibited antimicrobial activity (Hamedi et al., 2019). Similarly, Iquebal et al. (2021) isolated 235 bacterial strains from Pukzing Cave, India, with actinomycetes representing the predominant bacterial group. Approximately 20.4% of these isolates displayed broad-spectrum antimicrobial activity against pathogens like S. aureus, P. aeruginosa, E. coli, M. luteus, B. subtilis, and C. albicans.
Saudi Arabia is known for its rich geological landscape, including some of the rarest wild caves with remarkable geological and geomorphic features. However, the microbial content of these caves has been largely understudied. Few geological or microbial investigations have been conducted in the caves of Saudi Arabia, leaving a significant knowledge gap. Caves such as Jabal Al Qarah, Harrat Khaybar lava caves Dahl Rumahah, Kahf Al Shuwaymis, and the Umm Jirsan Lava-Tube are among the oldest known caves explored for their ecological and microbial diversity (Pint, 2009; Jastaniah et al., 2019a). These caves offer an uncharted environment for the discovery of extremophilic bacteria, particularly actinomycetes, which exhibit remarkable adaptations to oligotrophic conditions, low temperatures, and high humidity levels. Notably, actinomycetes isolated from Saudi caves, such as those in Jabal Al-Qarah, have demonstrated the ability to thrive in nutrient-deficient environments while exhibiting resilience to extreme physicochemical conditions. Caves rich in minerals such as sulfur, limestone, and gypsum serve as specialized habitats for mineral-tolerant actinomycetes, many of which possess bioactive properties. These microorganisms are of particular interest due to their potential to produce antimicrobial compounds and other secondary metabolites with biotechnological applications (Jastaniah et al., 2019a; Aly et al., 2020; Al-Ghamdi et al., 2021).
Initial microbial investigations have begun to characterize Saudi cave microbiomes. Amasha (2018) reported diverse microbial taxa, including Proteobacteria, Actinobacteria, Firmicutes, and Bacteroidetes, from Mossy, Hotel, and Reda Caves in the Summan region near Riyadh. Earlier work in Ghar Al Hibashi Cave, southeast of Makkah, revealed a diverse microbial assemblage, underscoring the potential of Saudi caves as reservoirs for novel bioactive compounds (Amasha, 2012). More recent studies have identified promising actinomycetes isolates from Saudi caves. Aly et al. (2020) and Bahamdain (2020) isolated Streptomyces griseorubens CL2 and Streptomyces CL24 from a cave in the Saman region, demonstrating potent activity against MRSA and E. faecalis. Al-Ghamdi et al. (2021) investigated the Umm Jirsan lava tube in Harrat Khaybar, isolating actinomycetes with strong antimicrobial properties against MRSA, S. aureus, E. faecalis, Serratia marcescens (S. marcescens), Salmonella sp., E. coli, P. aeruginosa and K. pneumoniae. Extreme cave environments, characterized by nutrient limitations and high humidity, support specialized microbial communities that produce antimicrobials with significant potential for agricultural, industrial, and medical applications. While global research has identified numerous novel strains, Saudi Arabian caves remain an underexplored resource. Expanding research in these ecosystems through standardized methodologies, genomic approaches, and interdisciplinary collaborations could unlock unique microbial taxa for biotechnological advancements.
Hot springs, characterized by elevated temperatures and unique physicochemical conditions, provide an ideal habitat for hyperthermophilic microorganisms. These microbes, thriving at temperatures between 45°C and 80°C, possess specialized membrane lipids rich in saturated, straight-chain fatty acids that ensure stability and fluidity under extreme conditions (Cherifa et al., 2023). Their metabolic adaptations make them valuable for biotechnological applications.
Microbial communities in hot springs encompass diverse thermophiles, including sporulating and non-sporulating bacilli, actinomycetes, and cyanobacteria (Jiang and Xu, 1993). Among them, actinomycetes—especially Streptomyces spp.—exhibit remarkable potential for synthesizing antimicrobial compounds and industrially relevant enzymes (Bahamdain et al., 2020; Cherifa et al., 2023). These compounds have wide-ranging uses, spanning from pharmaceutical development to bioremediation. However, the isolation and retention of thermophilic actinomycetes in pure cultures, despite their potential, continue to pose significant challenges. This has constrained research opportunities and impeded the identification of novel substances from these organisms (Alrumman et al., 2019; Ruwandeepika et al., 2022; Albasri et al., 2024).
Despite increasing interest in the thermophilic secondary metabolism, Saudi Arabia’s hot springs remain relatively unexplored. With approximately ten geothermal springs in regions such as Jizan, Al-Lith and Aseer, these sites present varied thermal and chemical conditions that support extremophiles (Khiyami et al., 2012; El-Gayar et al., 2017; Alrumman et al., 2018; Yasir et al., 2019). Several studies have reported actinomycetes from these environments capable of producing heat-stable enzymes and antimicrobial compounds, making them promising candidates for pharmaceutical and industrial applications (Al-Dhabi et al., 2019a; AlSediy et al., 2022).
Significant findings include the isolation of Streptomyces Al-Dhabi-1 from the Tharban hot spring, which exhibits potent antibacterial activity against Streptococcus agalactiae (S. agalactiae) and K. pneumoniae, along with antifungal properties against Cryptococcus neoformans (C. neoformans), C. albicans, Trichophyton mentagrophytes (T. mentagrophytes), and A. niger (Al-Dhabi et al., 2016). Another strain, Streptomyces sp. Al-Dhabi − 2, demonstrated broad-spectrum antimicrobial activity against B. cereus, E. faecalis, S. typhimurium, and P. aeruginosa (Al-Dhabi et al., 2019a). Further studies by Bahamdain et al. (2020) identified Streptomyces strains from soil sediments, hot spring water, and inner channel walls of the Oyun Al-Haar Al-Lith hot spring, which produce key enzymes such as keratinase, gelatinase, chitinase, lipase, amylase, and protease, with applications in pharmaceuticals, biofuel production, and waste degradation.
Recent investigations into microbial diversity in soils from hot springs in Jizan, Al-Lith, and Tabuk have revealed a diverse range of dominant microbial phyla, including Acidobacteriota, Actinobacteriota, Desulfobacterota, Firmicutes, Halanaerobiaeota, Nitrospirota, and Thermotogota. Notably, Actinobacteriota were most prevalent in Al-Lith, while Deinococcota dominated Jizan and Chloroflexi in Tabuk. While traditional culture-based methods have identified key thermophilic actinomycetes, they may underestimate the overall microbial diversity. Advanced molecular techniques, such as metagenomics and 16S rRNA sequencing, are increasingly being utilized to characterize microbial populations in extreme environments, providing a more comprehensive understanding of their diversity and ecological roles (AlSediy et al., 2022).
These findings suggest that Saudi Arabia’s hot springs harbor diverse thermophilic actinomycetes with significant antimicrobial and industrial potential. However, further genomic, biochemical, and ecological studies are required to fully harness their capabilities. Integrating modern omics technologies, including metabolomics, transcriptomics, and genomics, will facilitate the discovery of novel bioactive compounds, elucidate gene functions, metabolic pathways, and biosynthetic potential. Such advancements will enhance the discovery of new antimicrobial agents and industrially valuable enzymes, reinforcing the importance of these unique environments in biotechnology.
Mountainous regions, characterized by diverse heights, temperature fluctuations, and various soil compositions, offer a distinctive environment for microbial communities, especially actinomycetes. Adverse environmental conditions, like cold temperatures, elevated UV radiation, and restricted nutrition availability, serve as selective pressures, driving the evolution of actinomycetes strains with enhanced survival mechanisms. These adaptations often include the production of bioactive compounds with potent antimicrobial properties, which help the organisms compete with other microorganisms in their habitat. As a result, actinomycetes isolated from mountain soils have shown considerable potential in the development of new antibiotics, especially at a time when antimicrobial resistance is a growing global concern (Bull, 2011; Shivlata and Satyanarayana, 2015).
Studies across various mountainous regions have revealed the vast antimicrobial potential of actinomycetes. Gurung et al. (2009) isolated 79 actinomycetes from the Kalapatthar region of Mount Everest, with many demonstrating broad-spectrum antibacterial activity, including against MDR pathogens such as MRSA. Similarly, Zhu et al. (2014) examined over 800 actinomycetes from the Qinling and Himalayan mountains in China, identifying 96 isolates—primarily from Streptomyces—capable of inhibiting ESKAPE pathogens (E. faecium, S. aureus, K. pneumoniae, A. baumannii, P. aeruginosa, and Enterobacter cloacae), which are known for their resistance to conventional treatments. In Yemen’s Hadhramout governorate, Bawazir et al. (2017) identified Nocardia otitidiscaviarum, a potent isolate with broad-spectrum antibacterial activity. These findings collectively underscore the untapped potential of mountain-derived actinomycetes in novel antibiotic discovery.
The mountainous regions of Saudi Arabia, including the Aseer and Hijaz ranges, offer unique cooler, and more humid microclimates that contribute to the country’s environmental diversity. These conditions make the region promising for the exploration of bioactive microorganisms. Recent studies have highlighted the antimicrobial potential of actinomycetes from these regions. Alqahtani et al. (2022) studied actinomycetes isolated from the riverbed of Rijal Alma in Aseer, revealing strong antibacterial activity, particularly against E. coli and B. subtilis. Expanding on this, Albasri et al. (2024) focused on thermophilic actinomycetes from Uhud Mountain in Madinah, isolating strains closely related to Streptomyces macrosporus and Streptomyces rameus. These isolates exhibited significant antibacterial activity against B. cereus and S. aureus and notable antifungal activity against A. niger, Alternaria alternata (A. alternata), and Penicillium species. The discovery of such promising isolates in Saudi Arabia underscores the region’s potential as a reservoir of novel actinomycetes with pharmaceutical significance.
Mangroves are unique coastal ecosystems located in the intertidal zones of tropical and subtropical regions, particularly in Southeast Asia. These environments are characterized by extreme conditions such as high salinity, tidal fluctuations, and temperature variations, yet they support diverse microbial communities, including actinomycetes. The ability of actinomycetes to thrive in such harsh conditions suggests that they possess unique metabolic pathways, some of which may contribute to the production of bioactive compounds with potential applications in medicine and industry (Law et al., 2019).
Actinomycetes, particularly Streptomyces species, are prominent soil inhabitants within mangrove ecosystems and have gained considerable attention due to their capacity to produce a wide array of bioactive compounds, including antibiotics, antifungals, anticancer agents, and antioxidants. Studies conducted across diverse mangrove habitats have demonstrated significant variations in actinomycetes diversity and bioactivity, often influenced by geographical and environmental factors (Naikpatil and Rathod, 2011; Rajkumar et al., 2012; Ara et al., 2013). Many studies across East, South, and Southeast Asia have highlighted the diversity of mangrove actinomycetes as a rich source of novel bioactive compounds, many of which demonstrate strong antagonistic activity against a wide spectrum of microorganisms, including multidrug-resistant pathogens (Satheeja and Jebakumar, 2011; Sathiyaseelan and Stella, 2011; Govindarajan et al., 2021).
India possesses one of the most extensive mangrove ecosystems in South Asia, and numerous researchers have investigated the diversity of actinomycetes inside these areas. For example, Mitra et al. (2008) studied the distribution of actinomycetes in the Sundarbans mangroves and identified that the actinomycetes obtained from soil samples were classified into nine genera, displaying significant antimicrobial potential. Subsequent research in the Sundarbans by Arifuzzaman et al. (2010) and Sengupta et al. (2015) reinforced these findings, confirming the antimicrobial efficacy of various isolates. Other Indian mangroves, such as those in the Bay of Bengal and Pichavaram, Tamil Nadu, have also been explored for their actinobacterial potential. Ramesh and Mathivanan (2009) found that isolates from the Bay of Bengal exhibited strong antimicrobial activity against human pathogens, including P. aeruginosa, E. coli, B. subtilis, S. epidermidis, and C. albicans. Similarly, Usha et al. (2010) identified Streptomyces parvulus KUAP106 from Pichavaram mangroves, demonstrating potent antibacterial effects against B. subtilis, S. aureus, P. aeruginosa, and E. coli. Additional studies by Mangamuri et al. (2012) and Mangamuri et al. (2014) evaluated actinomycetes from the Nizampatnam and Coringa mangroves, which exhibited antimicrobial effects against E. coli, S. aureus, and C. albicans.
Mangroves in Southeast Asia exhibit comparable diversity of actinomycetes. In Malaysia, Lee et al. (2014) isolated multiple strains with significant antibacterial properties against a range of pathogens, including B. subtilis, B. cereus, E. faecalis, S. epidermidis, MRSA, S. typhi, Acinetobacter calcoaceticus, and Aeromonas hydrophila. Studies in Indonesia (Retnowati et al., 2017) reported actinobacteria from genera such as Streptomyces, Amycolatopsis, Nocardiopsis, and Saccharomonospora, highlighting their biotechnological potential. In East Asia, Hong et al. (2009) documented over 2,000 actinomycete isolates from Chinese mangroves, predominantly Streptomyces, with notable antimicrobial activity against C. albicans and S. aureus. Wei et al. (2010) further identified antibiotic-producing strains from Streptomyces, Micromonospora, and Nocardia, suggesting their relevance in infection treatment.
Research on Saudi Arabian mangroves, particularly those in Jazan and Jubail, is gaining momentum, revealing their potential as sources of novel actinomycetes (Reyad, 2013; Qureshi et al., 2021). Isolates from these environments have demonstrated promising antimicrobial activity against multidrug-resistant pathogens such as MRSA and have exhibited capabilities in producing industrially significant hydrolytic enzymes like amylase, cellulase, and lipase. These findings emphasize the untapped potential of arid-region mangroves in pharmaceutical (e.g., antibiotics and anticancer) and industrial biotechnology (e.g., biofuel production, bioremediation. and food industries). Furthermore, sustainability should remain a central focus to ensure long-term benefits without compromising mangrove conservation.
Actinomycetes are widely distributed across various ecosystems, extending beyond soil and marine environments to unique niches. These include the digestive tracts of arthropods, airborne spores, endophytic relationships with plants, and organic waste environments such as composts.
Actinomycetes are found in the digestive tracts of various arthropods, where they play crucial roles in host defense and microbiome stability (Mitousis et al., 2020; Olano and Rodríguez, 2024). For instance, African Tetraponera penzigi ants harbor Streptomyces formicae, which produces potent antibiotics known as formicamycins. These compounds exhibit significant inhibitory effects against multidrug-resistant pathogens such as MRSA and VRE (Qin et al., 2017). Additionally, rare actinomycetes, such as Nocardiosis sp., have been identified in the gut of bees and characterized by the expression of antibiotic biosynthetic genes (Selim et al., 2021). In Saudi Arabia, Streptomyces species were isolated from the gut of Apis mellifera yemintica honeybees, with extracts demonstrating significant antibacterial, antifungal, and anticancer properties (Al-Enazi et al., 2022). Similarly, earthworm castings serve as reservoirs of actinomycetes with potential antimicrobial activity and industrial enzyme production. Dominant genera in these castings include Streptomyces and Streptosporangium. Notably, Streptomyces isolates from earthworm castings exhibit antagonistic activity against wood-degrading fungi, suggesting applications in veterinary and human medicine (Selim et al., 2021).
Actinomycetes spores are pervasive in the atmosphere, where they contribute to respiratory allergies and have been identified as producers of bioactive compounds. Airborne Nocardia spores, for example have been associated with antimicrobial production (Lacey and Crook, 1988; Selim et al., 2021). However, further studies are needed to assess their impact on respiratory health and their potential industrial applications in bioprospecting novel antibiotics.
There are many reports demonstrating the distribution of endophytic actinomycetes in diverse plants, such as crop plants, medicinal plants, halophytes, and some woody tree species, mainly in the roots, stems or leaves of these plants. They are considered useful microorganisms due to their roles as a defense barrier against phytopathogens as well as their application in medical and industrial fields (Aly et al., 2019; Selim et al., 2021; Alzahrani et al., 2022). For example, Streptomyces parvulus Av-R5, associated with the root of Aloe vera exhibited strong antibacterial activity against multidrug-resistant S. aureus, K. pneumoniae, and A. niger (Chandrakar and Gupta, 2019). These findings highlight the potential of endophytic actinomycetes in the production of novel bioactive compounds, which can be further explored for applications in agriculture and medicine.
Composts and decayed fodder represent another critical habitat for actinomycetes due to their conducive damp, aerobic, and alkaline environments. In these environments, thermophilic actinomycetes, such as Saccharomonospora, Saccharopolyspora, Streptomyces, Thermoactinomyces, Thermobifida, and Thermomonospora, play an essential role in organic matter degradation. These organisms frequently form surface blooms, colloquially known as “fire tooth,” as temperatures rise during composting processes (Dilip et al., 2013; Bawazir and Shantaram, 2018; Ngamcharungchit et al., 2023). Research in Saudi Arabia has underscored the adaptability of actinomycetes to extreme environmental conditions. Isolates obtained from poultry farm waste, soil, water, fodder, and feathers have demonstrated promising biotechnological applications, including organic waste degradation and contributions to environmental remediation (Aly and Tork, 2018; Khalel et al., 2020).
Despite the remarkable antimicrobial potential of actinomycetes from diverse Saudi Arabian habitats, several challenges hinder their full exploitation for pharmaceutical and biotechnological applications. These challenges span across various stages, from strain isolation to industrial-scale production and regulatory approval.
• Limited Cultivability of Rare Actinomycetes: Many actinomycetes, particularly those from extreme environments, remain uncultivable under standard laboratory conditions. Conventional methods often fail to replicate the biochemical and ecological conditions required for their growth. Addressing this challenge necessitates customized media, advanced isolation methodologies (e.g., pretreatment), and in situ cultivation techniques such as isolation chip (iChip) technology, which facilitates the growth of previously unculturable microorganisms by preserving their natural environmental conditions. Additionally, co-cultivation strategies that simulate microbial interactions can enhance the growth of certain actinomycetes by mimicking their ecological niches (Hug et al., 2018).
• Complexity of Biosynthetic Gene Clusters (BGCs): The production of secondary metabolites is regulated by intricate BGCs, many of which remain silent under conventional culture conditions. Genome mining and activation strategies, such as co-cultivation and chemical elicitors, are needed to enhance metabolite production (van Bergeijk et al., 2020).
• High Costs of Large-Scale Production: The transition from laboratory-scale isolation to industrial-scale antibiotic production faces economic barriers, including costly fermentation processes and low yields of bioactive compounds (De Simeis and Serra, 2021).
• Regulatory and Safety Challenges: The approval of new antimicrobial agents requires extensive preclinical and clinical evaluations, which are time-consuming and costly (De Simeis and Serra, 2021; Jagannathan et al., 2021).
• Environmental and Sustainability Concerns: Overharvesting of natural habitats for actinomycete isolation may impact local ecosystems. Sustainable bioprospecting practices must be implemented to balance microbial conservation and discovery efforts (Jagannathan et al., 2021).
• Limited Integration of Advanced Technologies: A significant limitation in actinomycete research is the inadequate integration of modern technologies, including comprehensive genomic, metagenomic, and metabolomic characterization. The application of genome mining, synthetic biology, omics technologies (genomics, transcriptomics, proteomics, and metabolomics), AI-driven bioinformatics, and CRISPR-based gene editing remains underutilized, constraining the identification of novel BGCs responsible for antibiotic production (Ngamcharungchit et al., 2023).
• Challenges in Scalable Cultivation Techniques: While metagenomic-based screening approaches provide an alternative to direct cultivation by enabling the identification and characterization of novel microbial strains through environmental DNA analysis, their scalability remains a challenge. Further technological refinements are required to integrate these techniques into high-throughput screening pipelines (Ngamcharungchit et al., 2023).
• Advancements in Metagenomics and Genome Mining: Novel actinomycetes and their bioactive metabolites can be identified using metagenomic approaches, whole-genome sequencing, and bioinformatics tools, bypassing the need for traditional cultivation (Ngamcharungchit et al., 2023).
• AI and Machine Learning in Drug Discovery: AI-driven predictive models can streamline the discovery of novel antimicrobial compounds by analyzing large datasets of biosynthetic gene clusters and metabolic pathways (Ngamcharungchit et al., 2023)
• CRISPR and Synthetic Biology Approaches: Genetic engineering and synthetic biology can be utilized to enhance the biosynthetic potential of actinomycetes, leading to increased production of antibiotics and novel compounds (Mitousis et al., 2020).
• Bioprocess Optimization for Industrial Applications: The development of advanced fermentation techniques, such as continuous fermentation and metabolic engineering, can improve yield and reduce production costs (De Simeis and Serra, 2021).
• Integration of Omics Technologies: The “One Strain–Many Compounds” (OSMAC) approach, AI-driven drug discovery, bioinformatics, and CRISPR-based activation of cryptic BGCs offer promising avenues for unlocking novel bioactive compounds. Omics technologies provide comprehensive insights into gene expression, regulatory networks, and metabolite biosynthesis, thereby streamlining drug discovery and optimizing strain engineering (Ngamcharungchit et al., 2023).
• Bioprocessing and Drug Delivery Innovations: Bioprocessing advancements, such as scalable fermentation and nanotechnology-based drug delivery systems, can enhance the stability, efficacy, and bioavailability of actinomycete-derived compounds (Ngamcharungchit et al., 2023).
• Expanding Bioprospecting to Novel Ecological Niches: The exploration of extreme environments, including deserts, caves, marine ecosystems, and endophytic niches, is broadening the scope of actinomycete biodiversity and uncovering new antimicrobial metabolites. Expanding cultivation techniques and bioprospecting in these unique ecosystems will facilitate the discovery of actinomycetes with unique biosynthetic potential (Ngamcharungchit et al., 2023)
• Non-Traditional Therapeutic Strategies Against Antibiotic Resistance: In response to the rising threat of antimicrobial resistance, alternative treatment approaches are gaining attention. These include quorum sensing inhibitors, phage therapy, and anti-virulence drugs derived from actinomycetes to reduce resistance development (Jagannathan et al., 2021).
• Application Beyond Antimicrobials: Actinomycete-derived bioactive compounds are increasingly being explored for their potential applications beyond antibiotics, including oncology, immunotherapy, and agriculture. These compounds exhibit anticancer, anti-inflammatory, and immunomodulatory properties, opening new avenues for therapeutic development (Ngamcharungchit et al., 2023).
• Advanced Analytical Techniques for Compound Characterization: The identification and structural elucidation of novel bioactive compounds require cutting-edge analytical technologies, including mass spectrometry (MS), liquid chromatography (LC), gas chromatography (GC), and nuclear magnetic resonance (NMR) spectroscopy. These methods are essential for determining the molecular structures, biosynthetic pathways, and mechanisms of action of new antimicrobial compounds, thereby accelerating drug discovery and development (Hug et al., 2018; Jagannathan et al., 2021).
• Interdisciplinary Collaborations: Strengthening partnerships between microbiologists, biotechnologists, and pharmaceutical industries is crucial to accelerating the development of actinomycete-derived antibiotics (Hug et al., 2018).
This systematic review highlights the diverse actinomycete populations across Saudi Arabia’s terrestrial, aquatic, and extremophilic environments, emphasizing their untapped potential as sources of novel bioactive compounds. Actinomycetes from these unique ecosystems demonstrate significant antimicrobial efficacy against multidrug-resistant pathogens, reinforcing their importance in combating the global antimicrobial resistance crisis. However, challenges such as strain cultivability, biosynthetic gene activation, and industrial scalability must be addressed to fully harness their potential. Integrating advanced genomic, bioinformatics, and synthetic biology tools offers promising solutions to overcome these barriers. Future research should prioritize interdisciplinary collaborations, sustainable bioprospecting, and bioprocess optimization to facilitate the translation of actinomycete-derived bioactive compounds into clinically viable therapeutics. The unique microbial biodiversity of Saudi Arabia represents an invaluable resource for the discovery of next-generation antibiotics and other pharmaceutical innovations.
NH: Conceptualization, Data curation, Formal analysis, Funding acquisition, Investigation, Methodology, Project administration, Resources, Software, Supervision, Validation, Visualization, Writing – original draft, Writing – review & editing.
The author(s) declare that no financial support was received for the research and/or publication of this article.
The author declares that the research was conducted in the absence of any commercial or financial relationships that could be construed as a potential conflict of interest.
The authors declare that no Gen AI was used in the creation of this manuscript.
All claims expressed in this article are solely those of the authors and do not necessarily represent those of their affiliated organizations, or those of the publisher, the editors and the reviewers. Any product that may be evaluated in this article, or claim that may be made by its manufacturer, is not guaranteed or endorsed by the publisher.
Ababutain, I. M., Abdul Aziz, Z. K., and Al-Meshhen, N. A. (2012a). Lincomycin antibiotic biosynthesis produced by Streptomyces Sp. isolated from Saudi Arabia soil: I-taxonomical, antimicrobial and insecticidal studies on the producing organism. Canad. J. Pure Appl. Sci. 6, 1739–1748.
Ababutain, I. M., Abdul Aziz, Z. K., and Al-Meshhen, N. A. (2012b). Lincomycin antibiotic biosynthesis produced by Streptomyces Sp. isolated from Saudi Arabia soil: II-extraction, separation and purification of Lincomycin. Canad. J. Pure Appl. Sci. 6, 1905–1911.
Abdelfattah, M. S., Elmallah, M. I. Y., Hawas, U. W., Abou El-Kassema, L. T., and Eid, M. A. G. (2016). Isolation and characterization of marine-derived actinomycetes with cytotoxic activity from the Red Sea coast. Asian Pac. J. Trop. Biomed. 6, 651–657. doi: 10.1016/j.apjtb.2016.06.004
Abdelmohsen, U. R., Pimentel-Elardo, S. M., Hanora, A., Radwan, M., Abou-El-Ela, S. H., Ahmed, S., et al. (2010). Isolation, phylogenetic analysis and anti-infective activity screening of marine sponge-associated actinomycetes. Mar. Drugs 8, 399–412. doi: 10.3390/md8030399
Abdelmohsen, U. R., Yang, C., Horn, H., Hajjar, D., Ravasi, T., and Hentschel, U. (2014). Actinomycetes from Red Sea sponges: sources for chemical and phylogenetic diversity. Mar. Drugs 12, 2771–2789. doi: 10.3390/md12052771
Abid, I., Mujamammi, R., and Alkahtani, M. D. (2016). Antimicrobial activity and molecular identification of Streptomyces strains isolated from Saudi Arabia. J. Environ. Biol. 37, 1225–1230. doi: 10.22438/jeb/37/6/prn.97
Abo-Shadi, M. A., Sidkey, N. M., and Al-Mutrafy, A. M. (2010). Antimicrobial agent producing microbes from some Soils' rhizosphere in Al-Madinah Al-Munawwarah, KSA. J. Am. Sci. 6, 915–925.
Adegboye, M. F., and Babalola, O. O. (2012). Taxonomy and ecology of antibiotic producing actinomycetes. Afr. J. Agric. Res. 7, 2255–2261. doi: 10.5897/AJARX11.071
Ahmed, M. M., Hagagy, N., and AbdElgawad, H. (2021). Establishment of actinobacteria–Satureja hortensis interactions under future climate CO2-enhanced crop productivity in drought environments of Saudi Arabia. Environ. Sci. Pollut. Res. 28, 62853–62867. doi: 10.1007/s11356-021-14777-7
Alaidaroos, B. A. (2022). Rare Actinomycetes from undiscovered sources as a source of novel antimicrobial agents to control multidrug-resistant Bacteria. Int. J. Pharm. Res. Allied Sci. 11, 158–167. doi: 10.51847/dPfaJ9FIEp
Alanis, A. J. (2005). Resistance to antibiotics: are we in the post-antibiotic era? Arch. Med. Res. 36, 697–705. doi: 10.1016/j.arcmed.2005.06.009
Al-Askar, A. A., Abdulkhair, W. M., Rashad, Y. M., Hafez, E. E., Ghoneem, K. M., and Baka, Z. A. (2014). Streptomyces griseorubens E44G: a potent antagonist isolated from soil in Saudi Arabia. J. Pure Appl. Microbiol. 8, 221–230.
Al-Askar, A. A., Abdul Khair, W. M., and Rashad, Y. M. (2011). In vitro antifungal activity of Streptomyces spororaveus RDS 28 against some phytopathogenic fungi. Afr. J. Agric. Res. 6, 2835–2842.
Albasri, H., Almohammadi, M., and Mawad, A. (2024). Biological activity and enzymatic productivity of two thermophilic Actinomycetes isolated from the Uhud Mountain. Appl. Ecol. Environ. Res. 22, 3975–3992. doi: 10.15666/aeer/2205_39753992
Al-Dhabi, N. A., Esmail, G. A., Duraipandiyan, V., and Arasu, M. V. (2019a). Chemical profiling of Streptomyces sp. Al-Dhabi-2 recovered from an extreme environment in Saudi Arabia as a novel drug source for medical and industrial applications. Saudi J. Biol. Sci. 26, 758–766. doi: 10.1016/j.sjbs.2019.03.009
Al-Dhabi, N. A., Esmail, G. A., Duraipandiyan, V., Valan Arasu, M., and Salem-Bekhit, M. M. (2016). Isolation, identification and screening of antimicrobial thermophilic Streptomyces sp. Al-Dhabi-1 isolated from Tharban hot spring, Saudi Arabia. Extremophiles 20, 79–90. doi: 10.1007/s00792-015-0799-1
Al-Dhabi, N. A., Esmail, G. A., Ghilan, A. K. M., Arasu, M. V., and Duraipandiyan, V. (2020a). Metabolite profiling of Streptomyces sp. Al-Dhabi-100 isolated from the marine environment in Saudi Arabia with anti-bacterial, anti-tubercular and anti-oxidant potentials. J. King Saud Univ. Sci. 32, 1628–1633. doi: 10.1016/j.jksus.2019.12.021
Al-Dhabi, N. A., Esmail, G. A., Ghilan, A. K. M., Arasu, M. V., Duraipandiyan, V., and Ponmurugan, K. (2020b). Chemical constituents of Streptomyces sp. strain Al-Dhabi-97 isolated from the marine region of Saudi Arabia with antibacterial and anticancer properties. J. Infect. Public Health 13, 235–243. doi: 10.1016/j.jiph.2019.09.004
Al-Dhabi, N. A., Ghilan, A. K. M., Arasu, M. V., and Duraipandiyan, V. (2018). Green biosynthesis of silver nanoparticles produced from marine Streptomyces sp. Al-Dhabi-89 and their potential applications against wound infection and drug-resistant clinical pathogens. J. Photochem. Photobiol. B Biol. 189, 176–184. doi: 10.1016/j.jphotobiol.2018.09.012
Al-Dhabi, N. A., Ghilan, A. K. M., Esmail, G. A., Arasu, M. V., Duraipandiyan, V., and Ponmurugan, K. (2019b). Bioactivity assessment of the Saudi Arabian marine Streptomyces sp. Al-Dhabi-90, metabolic profiling and its in vitro inhibitory property against multidrug resistant and extended-spectrum beta-lactamase clinical bacterial pathogens. J. Infect. Public Health 12, 549–556. doi: 10.1016/j.jiph.2019.01.065
Al-Enazi, N. M., Abdel-Raouf, N., Alharbi, R. M., and Sholkamy, E. N. (2022). Metabolic profiling of Streptomyces sp. strain ESS_AMH1 isolated from Apis mellifera yemintica’s gut microbiome, and its anticancer activity against breast cancer (mcf7) and hepatocarcinoma (hepg2) cell lines, as well as antimicrobial activity. Appl. Sci. 12:12257. doi: 10.3390/app122312257
Alenazi, A. M., Anwar, Y., Abo-Aba, S. E. M., and Bataweel, N. M. (2023). A review on Actinomycetes distribution, isolation, and their medical applications. Novel Res. Microbiol. J. 7, 1918–1931. doi: 10.21608/nrmj.2023.294180
Al-Garni, S. M., Sabir, J. S. M., El-Hanafy, A. A. E. M., Kabli, S. A., Al-Twiley, D. A., and Ahmed, M. M. (2014). Isolation and identification of antimicrobial Actinomycetes strains from Saudi environment. J. Food Agric. Environ., 12, 1072–1079. Available online at: https://www.researchgate.net/publication/262263583 (Accessed March 20, 2025).
Alghamdi, A. A., Abdelmoneim, T. S., Kadasa, N., and Almulaiky, Y. Q. (2021). Evaluating desert Actinomycetes for enzyme and antibacterial production. J. Pure Appl. Microbiol. 15, 976–982. doi: 10.22207/JPAM.15.2.56
Al-Ghamdi, S. A., Jastaniah, S. D., and Amasha, R. H. (2021). Isolation and screening of actinomycetes from umm Jirsan cave, Saudi Arabia for their antibacterial activity. Biosci. Biotech. Res. Comm. 14, 10–21786. doi: 10.21786/bbrc/14.1/50
Alharbi, N. S. (2016). Novel bioactive molecules from marine actinomycetes. Biosci. Biotech. Res. Asia 13, 1905–1927. doi: 10.13005/bbra/2346
Alharbi, S. A., Chinnathambi Arunachalam, C. A., Murugan, A. M., and Wainwright, M. (2012). Antibacterial activity of actinomycetes isolated from terrestrial soil of Saudi Arabia. J. Food Agric. Environ. 10, 1093–1097.
Ali, M. H., Aljadaani, S., Khan, J., Sindi, I., Aboras, M., and Aly, M. M. (2020). Isolation and molecular identification of two Chitinase producing Bacteria from marine shrimp Shell wastes. Pakistan J. Biol. Sci. 23, 139–149. doi: 10.3923/pjbs.2020.139.149
Al-Kadeeb, S. A., Al-Rokban, A. H., and Awad, F. (2012). Characterization and identification of actinomycetes isolated from contaminated soil in Riyadh. Int. J. Sci. Eng. Res. 3, 1–8.
Al-Kahtani, M. D. (2016). Isolation and molecular identification of Streptomyces spp. with antibacterial activity from KSA. Egypt. Acad. J. Biol. Sci. C Physiol. Mol. Biol. 8, 65–73. doi: 10.21608/eajbsc.2016.13682
Al-Khamash, N. M., Hozzein, W. N., and Alharbi, S. A. (2023). Metabolites profiling and antimicrobial activity of Actinobacteria associated with plants collected from Thumama in Riyadh region Saudi Arabia. Sch. Acad. J. Biosci. 11, 163–170. doi: 10.36347/sajb.2023.v11i05.001
Alkhelaiwi, F. M., Ara, I., and Moubayed, N. (2021). Active antibiotics production by Actinomycetes indigenous to Saudi Arabia soils: life sciences-microbiology for health care. Int. J. Life Sci. Pharma Res. 11, 20–29. doi: 10.22376/ijpbs/lpr.2021.11.6.L20-29
Almuhayawi, M. S., Mohamed, M. S., Abdel-Mawgoud, M., Selim, S., Al Jaouni, S. K., and AbdElgawad, H. (2021). Bioactive potential of several actinobacteria isolated from microbiologically barely explored desert habitat, Saudi Arabia. Biology 10:235. doi: 10.3390/biology10030235
Alotaibi, F. (2019). Carbapenem-resistant Enterobacteriaceae: an update narrative review from Saudi Arabia. J. Infect. Public Health 12, 465–471. doi: 10.1016/j.jiph.2019.03.024
Alotaibi, M. O., Sonbol, H. S., Alwakeel, S. S., Suliman, R. S., Fodah, R. A., Jaffal, A. S. A., et al. (2020). Microbial diversity of some sabkha and desert sites in Saudi Arabia. Saudi J. Biol. Sci. 27, 2778–2789. doi: 10.1016/j.sjbs.2020.06.038
Alqahtani, S. S., Moni, S. S., Sultan, M. H., Bakkari, M. A., Madkhali, O. A., Alshahrani, S., et al. (2022). Potential bioactive secondary metabolites of Actinomycetes sp. isolated from rocky soils of the heritage village Rijal Alma, Saudi Arabia. Arab. J. Chem. 15:103793. doi: 10.1016/j.arabjc.2022.103793
Alrumman, S. A., Mostafa, Y. S., Al-Qahtani, S. T., Sahlabji, T., and Taha, T. H. (2019). Antimicrobial activity and GC-MS analysis of bioactive constituents of thermophilic bacteria isolated from Saudi hot springs. Arab. J. Sci. Eng. 44, 75–85. doi: 10.1007/s13369-018-3597-0
Alrumman, S., Mostafa, Y. S. M., Al-Qahtani, S., and Taha, T. H. T. (2018). Hydrolytic enzyme production by thermophilic bacteria isolated from Saudi hot springs. Open Life Sci. 13, 470–480. doi: 10.1515/biol-2018-0056
AlSediy, K. H., Ashy, R. A., Al-Fassi, F. A., and AlJudaibi, A. A. (2022). Microbial diversity and abundance in the hot springs on the west coast of Saudi Arabia as a potential source of novel industrial products. Microb. Biosyst. 7, 8–17. doi: 10.21608/mb.2022.134787.1055
Al-Shaibani, M. M., Radin Mohamed, R. M. S., Sidik, N. M., Enshasy, H. A. E., Al-Gheethi, A., Noman, E., et al. (2021). Biodiversity of secondary metabolites compounds isolated from phylum actinobacteria and its therapeutic applications. Molecules 26:4504. doi: 10.3390/molecules26154504
Aly, M. M., Ahmed, B. L., Abu Aba, S., Hassan, R. A., Bataweel, N. M., and Abu-Zeid, M. (2020). Isolation and molecular identification of Streptomyces griseorubens from Al Saman region cave as a producer of antibacterial agent. Int. J. Pharm. Phytopharmacol. Res. 10, 142–148.
Aly, M. M., Al-Aidroos, B. A., and Alfassi, F. A. (2011). Production of non polyenic antifungal agent from Streptomyces sp BM54, isolated from marine shrimps. Crown J. Med. 1, 1–08.
Aly, M. M., Bahamdain, L. A., and Aba, S. A. (2019). Unexplored extreme habitats as sources of novel and rare actinomycetes with enzyme and antimicrobial activities. IOSR 14, 45–54. doi: 10.9790/3008-1406034554
Aly, M. M., and El-Sabbagh, S. M. (2004). Nile-water sediments as a source of actinomycetes exhibiting biomedical activity. In Proceeding 3th International Conference Biological Science.
Aly, M. M., and Tork, S. (2018). High keratinase production and keratin degradation by a mutant strain KR II, derived from Streptomyces radiopugnans KR 12. J. Appl. Biol. Sci. 12, 18–25.
Alzahrani, K. J. (2021). Microbiome studies from Saudi Arabia over the last 10 years: achievements, gaps, and future directions. Microorganisms 9:2021. doi: 10.3390/microorganisms9102021
Alzahrani, K., Jastaniah, S., Amasha, R., and Aly, M. M. (2022). Diversity and colonization of endophytic Actinomycetes in some medicinal plants. J. Contemp. Med. Sci. 8, 153–161. doi: 10.22317/jcms.v8i3.1222
Amasha, R. (2012). Studies on the environmental microbiology and biogeochemistry of desert surface soils. Sheffield, UK: The University of Sheffield.
Amasha, R. H. (2018). Microbial diversity and phylogenetic studies of some microbes obtained from unexplored caves of Saudi Arabia. J. Exp. Biol. Agric. Sci. 6, 342–651. doi: 10.18006/2018.6(2).342.351
Ara, I., Bakir, M. A., Hozzein, W. N., and Kudo, T. (2013). Population, morphological and chemotaxonomical characterization of diverse rare actinomycetes in the mangrove and medicinal plant rhizosphere. Afr. J. Microbiol. Res. 7, 1480–1488. doi: 10.5897/AJMR12.452
Arifuzzaman, M., Khatun, M. R., and Rahman, H. (2010). Isolation and screening of actinomycetes from Sundarbans soil for antibacterial activity. Afr. J. Biotechnol. 9, 4615–4619.
Atallah, B. M., El-Mohsnawy, E., El-Shouny, W. A., and Haroun, S. A. (2023b). Identification and characterization of different potentially antibacterial compounds from a marine Streptomyces sp. Sp1. JAPS 33, 166–173. doi: 10.36899/JAPS.2023.1.0605
Atallah, B. M., Haroun, S. A., and El-Mohsnawy, E. (2023a). Antibacterial activity of two actinomycetes species isolated from black sand in North Egypt. S. Afr. J. Sci. 119, 1–8. doi: 10.17159/sajs.2023/14509
Atta, H. M., Bayoumi, R., El-Sehrawi, M., Aboshady, A., and Al-Humiany, A. (2010). Biotechnological application for producing some antimicrobial agents by actinomycetes isolates from Al-khurmah governorate. Eur. J. Appl. Sci., 2, 98–107. Available online at: https://www.researchgate.net/publication/286861770 (Accessed March 20, 2025).
Augustine, N., Peter, A. W., Kerkar, S., and Thomas, S. (2012). Arctic actinomycetes as potential inhibitors of Vibrio cholerae biofilm. Curr. Microbiol. 64, 338–342. doi: 10.1007/s00284-011-0073-4
Awad, N. M., Rasmey, A. H. M., Elshamy, A. I., and Aboseidah, A. (2024). Antimicrobial activities of some Actinomycetes isolated from cultivated soil, Egypt. Front. Sci. Res. Technol. 1:1112. doi: 10.21608/fsrt.2023.246304.1112
Bahamdain, L. A. (2020). Extreme habitats of Saudi Arabia as sources of novel Actinomycetes with antimicrobial activities. Jeddah: King Abdulaziz University.
Bahamdain, L. A., Abo Aba, S. E., Sabry, A., Amasha, R. H., Noor, S., and Aly, M. M. (2020). Molecular identification and phylogenetic analysis of some rare Actinomycetes obtained from Al-Lith hot spring area of Saudi Arabia. Biosci. Biotech. Res. Comm. 13, 1037–1049. doi: 10.21786/bbrc/13.3/8
Barka, E. A., Vatsa, P., Sanchez, L., Gaveau-Vaillant, N., Jacquard, C., Klenk, H. P., et al. (2016). Taxonomy, physiology, and natural products of Actinobacteria. Microbiol. Mol. Biol. Rev. 80, 1–43. doi: 10.1128/mmbr.00019-15
Bawazir, A. M. A., Al-Haddad, A., Abdullah, A. M., and Shantaram, M. (2017). Actinomycetes from mountains of Hadhramout–Yemen. Int. J. Curr. Microbiol. App. Sci. 8, 3521–3530. doi: 10.20546/ijcmas.2017.608.421
Bawazir, A. M. A., Palaksha, M. S., and Shantaram, M. (2019). An implication of Actinomycetes on human well-being: A review. Int J Pharm Pharm Sci 11, 11–18. doi: 10.22159/ijpps.2019v11i5.31829
Bawazir, A. M. A., and Shantaram, M. (2018). Ecology and distribution of actinomycetes in nature. Int. J. Curr. Res. 10, 71664–71668. doi: 10.24941/ijcr.31442.07.2018
Benhadj, M., Gacemi-Kirane, D., Menasria, T., Guebla, K., and Ahmane, Z. (2019). Screening of rare actinomycetes isolated from natural wetland ecosystem (Fetzara Lake, northeastern Algeria) for hydrolytic enzymes and antimicrobial activities. J. King Saud Univ. Sci. 31, 706–712. doi: 10.1016/j.jksus.2018.03.008
Bull, A. T. (2011). “Actinobacteria of the Extremobiosphere” in Extremophiles handbook. ed. K. Horikoshi (Tokyo: Springer).
Chakraborty, B., Shashiraj, K. N., Kumar, R. S., Bhat, M. P., Basavarajappa, D. S., Almansour, A. I., et al. (2023). Unveiling the pharmacological significance of marine Streptomyces violaceusniger KS20: isolation, characterization, and assessment of its biomedical applications. Meta 13:1022. doi: 10.3390/metabo13091022
Chandrakar, S., and Gupta, A. K. (2019). Actinomycin-producing endophytic Streptomyces parvulus associated with root of aloe vera and optimization of conditions for antibiotic production. Probiot. Antimicrob. Prot. 11, 1055–1069. doi: 10.1007/s12602-018-9451-6
Chaudhary, H. S., Soni, B., Shrivastava, A. R., and Shrivastava, S. (2013). Diversity and versatility of actinomycetes and its role in antibiotic production. J. Appl. Pharm. Sci. 3, S83–S94. doi: 10.7324/JAPS.2013.38.S14
Cheeptham, N. (2012). Advances and challenges in studying cave microbial diversity. Cave Microb. 1, 1–34. doi: 10.1007/978-1-4614-5206-5_1
Cherifa, L., Meissa, M., Uzel, A., and Allaoueddine, B. (2023). Thermophilic Actinobacteria isolated from Tleghma hot spring: a potential source of thermostable α -amylase. Carpathian J. Food Sci. Technol. 15, 168–182. doi: 10.34302/crpjfst/2023.15.4.13
De Simeis, D., and Serra, S. (2021). Actinomycetes: A never-ending source of bioactive compounds—an overview on antibiotics production. Antibiotics 10:483. doi: 10.3390/antibiotics10050483
Dewedar, A., Mourad, F. E., and Sheha, H. (1979). Thermomonospora sp. T-SA-125 and its production of a growth promoting antibiotic. Folia Microbiol. 24, 396–402. doi: 10.1007/BF02927122
Dilip, C. V., Mulaje, S. S., and Mohalkar, R. Y. (2013). A review on actinomycetes and their biotechnological application. Int. J. Pharm. Sci. Res. 4, 1730–1742. doi: 10.13040/IJPSR.0975-8232.4(5).1730-42
Dimri, A. G., Chauhan, A., and Aggarwal, M. L. (2020). Antibiotic potential of actinomycetes from different environments against human pathogens and microorganisms of industrial importance: a review. Sci. Arch. 1, 7–24. doi: 10.47587/SA.2020.1102
Duangmal, K., Mingma, R., Pathom-Aree, W., Niyomvong, N., Inahashi, Y., Matsumoto, A., et al. (2012). Microbispora thailandensis sp. nov., an actinomycete isolated from cave soil. J. Antibiot. 65, 491–494. doi: 10.1038/ja.2012.57
El Samak, M., Solyman, S. M., and Hanora, A. (2018). Antimicrobial activity of bacteria isolated from Red Sea marine invertebrates. Biotechnol. Rep. 19:e00275. doi: 10.1016/j.btre.2018.e00275
Elbendary, A. A., Hessain, A. M., El-Hariri, M. D., Seida, A. A., Moussa, I. M., Mubarak, A. S., et al. (2018). Isolation of antimicrobial producing Actinobacteria from soil samples. Saudi J. Biol. Sci. 25, 44–46. doi: 10.1016/j.sjbs.2017.05.003
El-Gayar, K. E., Al Abboud, M. A., and Essa, A. M. (2017). Characterization of thermophilic Bacteria isolated from two Hot Springs in Jazan, Saudi Arabia. J. Pure Appl. Microbiol. 11, 743–752. doi: 10.22207/JPAM.11.2.13
El-Gendy, M., Hawas, U. W., and Jaspars, M. (2008). Novel bioactive metabolites from a marine derived bacterium Nocardia sp. ALAA 2000. J. Antibiot. 61, 379–386. doi: 10.1038/ja.2008.53
Elsayed, T. R., Galil, D. F., Sedik, M. Z., Hassan, H. M., and Sadik, M. W. (2020). Antimicrobial and anticancer activities of actinomycetes isolated from Egyptian soils. Int. J. Curr. Microbiol. Appl. Sci. 9, 1689–1700. doi: 10.20546/ijcmas.2020.909.209
Encheva-Malinova, M., Stoyanova, M., Avramova, H., Pavlova, Y., Gocheva, B., Ivanova, I., et al. (2014). Antibacterial potential of streptomycete strains from Antarctic soils. Biotechnol. Biotechnol. Equip. 28, 721–727. doi: 10.1080/13102818.2014.947066
Farda, B., Djebaili, R., Vaccarelli, I., Del Gallo, M., and Pellegrini, M. (2022). Actinomycetes from caves: an overview of their diversity, biotechnological properties, and insights for their use in soil environments. Microorganisms 10:453. doi: 10.3390/microorganisms10020453
Fatima, A., Aftab, U., Shaaban, K. A., Thorson, J. S., and Sajid, I. (2019). Spore forming Actinobacterial diversity of Cholistan Desert Pakistan: Polyphasic taxonomy, antimicrobial potential and chemical profiling. BMC Microbiol. 19, 49–17. doi: 10.1186/s12866-019-1414-x
Fenical, W., and Jensen, P. R. (2006). Developing a new resource for drug discovery: marine actinomycete bacteria. Nat. Chem. Biol. 2, 666–673. doi: 10.1038/nchembio841
Gebreyohannes, G., Moges, F., Sahile, S., and Raja, N. (2013). Isolation and characterization of potential antibiotic producing actinomycetes from water and sediments of Lake Tana, Ethiopia. Asian Pac. J. Trop. Biomed. 3, 426–435. doi: 10.1016/S2221-1691(13)60092-1
Gohain, A., Gogoi, A., Debnath, R., Yadav, A., Singh, B. P., Gupta, V. K., et al. (2015). Antimicrobial biosynthetic potential and genetic diversity of endophytic actinomycetes associated with medicinal plants. FEMS Microbiol. Lett. 362:fnv158. doi: 10.1093/femsle/fnv158
Goodfellow, M., Kampfer, P., Busse, H. J., Trujillo, M. E., Suzuki, K., Ludwig, W., et al. (2012). Bergey's manual of systematic bacteriology. New York, NY: Springer.
Govindarajan, G., Mullick, P., Raj, B. A. S., Kumar, P. S., Al-Ansari, M. M., Ilavenil, S., et al. (2021). Susceptibility pattern of methicillin resistance Staphylococcus aureus (MRSA) by flow cytometry analysis and characterization of novel lead drug molecule from Streptomyces species. J. Infect. Public Health 14, 1831–1841. doi: 10.1016/j.jiph.2021.11.001
Groth, I., Schumann, P., Schütze, B., Augsten, K., and Stackebrandt, E. (2002). Knoellia sinensis gen. Nov., sp. nov. and Knoellia subterranea sp. nov., two novel actinobacteria isolated from a cave. Int. J. Syst. Evol. Microbiol. 52, 77–84. doi: 10.1099/00207713-52-1-77
Gurung, T. D., Sherpa, C., Agrawal, V. P., and Lekhak, B. (2009). Isolation and characterization of antibacterial actinomycetes from soil samples of Kalapatthar, Mount Everest region. Nepal J. Sci. Technol. 10, 173–182. doi: 10.3126/njst.v10i0.2957
Hamed, M. M., Abdelfattah, L. S., and Fahmy, N. M. (2019). Antimicrobial activity of marine Actinomycetes and the optimization of culture conditions for the production of antimicrobial agent (s). J. Pure Appl. Microbiol. 13, 2177–2188. doi: 10.22207/JPAM.13.4.30
Hamedi, J., Kafshnouchi, M., and Ranjbaran, M. (2019). A study on actinobacterial diversity of Hampoeil cave and screening of their biological activities. Saudi J. Biol. Sci. 26, 1587–1595. doi: 10.1016/j.sjbs.2018.10.010
Hausawi, A. A., Baeshen, N. A., Al-Hejin, A. M., Al-Matary, M. A., Almaaqar, S. M., and Ahmed, M. M. M. (2021). Screening Different Desert soils in Makkah Province to detect novel antibiotics producing Actinomycetes. Adv. Environ. Biol. 15, 7–13. doi: 10.22587/aeb.2021.15.12.2
Hegazy, G. E., Olama, Z. A., Abou-Elela, G. M., Ramadan, H. S., Ibrahim, W. M., and El Badan, D. E. S. (2023). Biodiversity and biological applications of marine actinomycetes—Abu-Qir Bay, Mediterranean Sea, Egypt. J. Genetic Eng. Biotechnol. 21:150. doi: 10.1186/s43141-023-00612-8
Hong, K., Gao, A. H., Xie, Q. Y., Gao, H., Zhuang, L., Lin, H. P., et al. (2009). Actinomycetes for marine drug discovery isolated from mangrove soils and plants in China. Mar. Drugs 7, 24–44. doi: 10.3390/md7010024
Hozzein, W. N., Rabie, W., and Ali, M. I. A. (2011). Screening the Egyptian desert actinomycetes as candidates for new antimicrobial compounds and identification of a new desert Streptomyces strain. Afr. J. Biotechnol. 10, 2295–2301.
Hug, J. J., Bader, C. D., Remškar, M., Cirnski, K., and Müller, R. (2018). Concepts and methods to access novel antibiotics from actinomycetes. Antibiotics 7:44. doi: 10.3390/antibiotics7020044
Ibnouf, E. O., Aldawsari, M. F., and Waggiallah, H. A. (2022). Isolation and extraction of some compounds that act as antimicrobials from actinomycetes. Saudi J. Biol. Sci. 29:103352. doi: 10.1016/j.sjbs.2022.103352
Iquebal, M. A., Passari, A. K., Jagannadham, J., Ahmad, F., Leo, V. V., Singh, G., et al. (2021). Microbiome of Pukzing cave in India shows high antimicrobial activity against plant and animal pathogens. Genomics 113, 4098–4108. doi: 10.1016/j.ygeno.2021.10.004
Jagannathan, S. V., Manemann, E. M., Rowe, S. E., Callender, M. C., and Soto, W. (2021). Marine actinomycetes, new sources of biotechnological products. Mar. Drugs 19:365. doi: 10.3390/md19070365
Jastaniah, S. D., Amasha, R. H., and Alanbari, A. H. (2019a). Isolation and identification of some antimicrobial producing bacteria from caves in Summan plateau, Saudi Arabia. J. Pharm. Biol. Sci. 14, 26–33.
Jastaniah, S., Dawalibi, S., Amasha, R., and Aly, M. (2019b). Inhibition of some human bacterial pathogens using Streptomyces sp. SD5 obtained from soil sample from Jeddah. J. Exp. Biol. Agric. Sci. 7, 222–232. doi: 10.18006/2019.7(2).222.232
Jiang, C., and Xu, L. (1993). Actinomycete diversity in unusual habitats. Actinomycetes, 4, 47–57. Available online at: http://hdl.handle.net/1807/21366 (Accessed March 20, 2025).
Jones, B. (2001). Microbial activity in caves− A geological perspective. Geomicrobiol. J. 18, 345–357. doi: 10.1080/01490450152467831
Kalyani, A. L. T., Ramya Sravani, K. M., and Annapurna, J. (2012). Isolation and characterization of antibiotic producing actinomycetes from marine soil samples. Int. J. Curr. Pharm. Res. 4, 109–112.
Kekuda, T. P., Shobha, K. S., and Onkarappa, R. (2010). Fascinating diversity and potent biological activities of Actinomycete metabolites. J. Pharm. Res., 3, pp.250–256. Available online at: https://www.researchgate.net/publication/259357914 (Accessed March 20, 2025).
Khalel, A., Alshehri, W., and Aly, M. (2020). Enhancing plant growth by chicken feather compost obtained from feather degradation by Streptomyces enissocaesilis. Biosci. Biotech. Res. C 13, 1847–1853. doi: 10.21786/bbrc/13.4/32
Khallaf, H., Amasha, R., Alaidaroos, B., Jastaniah, S., and Aly, M. (2023). Isolation and molecular characterization of some plant growth promoting actinomycetes from cultivated soil for management of some pathogenic microbes. Agric. Biol. Res. 39, 412–420.
Khanna, M., Solanki, R., and Lal, R. (2011). Selective isolation of rare actinomycetes producing novel antimicrobial compounds. Int. J. Adv. Biotechnol. Res., 2, pp.357–375. Available online at: https://www.researchgate.net/publication/262878272 (Accessed March 20, 2025).
Kharat, K. R., Kharat, A., and Hardikar, B. P. (2009). Antimicrobial and cytotoxic activity of Streptomyces sp. from Lonar Lake. Afr. J. Biotechnol. 8:23.
Khiyami, M. A., Serour, E. A., Shehata, M. M., and Bahklia, A. H. (2012). Thermo-aerobic bacteria from geothermal springs in Saudi Arabia. Afr. J. Biotechnol. 11, 4053–4062. doi: 10.5897/AJB11.3339
Kiki, M. J., and Al Turk, I. M. (2013). Characterization of a novel strain of the genus Actinopolyspora, an extremely halophilic actinomycete isolated from Saudi Arabia. Life Sci. J., 10.pp.603–608. Available online at: https://www.researchgate.net/publication/288308707 (Accessed March 20, 2025).
Kiran, M. A., Alghamdi, S., Ashgar, S., Alhindi, Z., Al-Abdullah, N., and Dablool, A. S. (2024). Systematic review and Meta-analysis on antimicrobial resistance and drug resistance in Saudi Arabia. J. Glob. Antimicrob. Resist. 39, 128–136. doi: 10.1016/j.jgar.2024.07.013
Lacey, J., and Crook, B. (1988). Fungal and actinomycete spores as pollutants of the workplace and occupational allergens. Ann. Occup. Hyg. 32, 515–533. doi: 10.1093/annhyg/32.4.515
Law, J. W. F., Pusparajah, P., Ab Mutalib, N. S., Wong, S. H., Goh, B. H., and Lee, L. H. (2019). A review on mangrove actinobacterial diversity: the roles of Streptomyces and novel species discovery. Progress Microb. Biol. 2:24. doi: 10.36877/pmmb.a0000024
Lazzarini, A., Cavaletti, L., Toppo, G., and Marinelli, F. (2000). Rare genera of actinomycetes as potential producers of new antibiotics. Antonie Van Leeuwenhoek 78, 399–405. doi: 10.1023/A:1010287600557
Lee, L. H., Zainal, N., Azman, A. S., Eng, S. K., Goh, B. H., Yin, W. F., et al. (2014). Diversity and antimicrobial activities of actinobacteria isolated from tropical mangrove sediments in Malaysia. Sci. World J. 2014:698178, 1–14. doi: 10.1155/2014/698178
Li, L. Y., Yang, Z. W., Asem, M. D., Salam, N., Xiao, M., Alkhalifah, D. H. M., et al. (2019). Georgenia alba sp. nov., a novel halotolerant actinobacterium isolated from a desert sand sample. Antonie Van Leeuwenhoek 112, 203–209. doi: 10.1007/s10482-018-1145-2
Long, Y., Jiang, J., Hu, X., Zhou, J., Hu, J., and Zhou, S. (2019). Actinobacterial community in Shuanghe cave using culture-dependent and-independent approaches. World J. Microbiol. Biotechnol. 35, 153–112. doi: 10.1007/s11274-019-2713-y
Mahajan, G. B., and Balachandran, L. (2012). Antibacterial agents from actinomycetes-a review. Front. Biosci. Elite E4, 240–253. doi: 10.2741/373
Mahmoud, H. M., and Kalendar, A. A. (2016). Coral-associated actinobacteria: diversity, abundance, and biotechnological potentials. Front. Microbiol. 7:204. doi: 10.3389/fmicb.2016.00204
Maiti, P. K., Das, S., Sahoo, P., and Mandal, S. (2020). Streptomyces sp SM01 isolated from Indian soil produces a novel antibiotic picolinamycin effective against multi drug resistant bacterial strains. Sci. Rep. 10:10092. doi: 10.1038/s41598-020-66984-w
Majidzadeh, M., Heidarieh, P., Fatahi-Bafghi, M., and Vakili, M. (2021). Antimicrobial activity of Actinobacteria isolated from dry land soil in Yazd, Iran. Mol. Biol. Rep. 48, 1717–1723. doi: 10.1007/s11033-021-06218-y
Mancuso, G., Midiri, A., Gerace, E., and Biondo, C. (2021). Bacterial antibiotic resistance: the most critical pathogens. Pathogens 10:1310. doi: 10.3390/pathogens10101310
Mangamuri, U. K., Muvva, V., Poda, S., and Kamma, S. (2012). Isolation, identification and molecular characterization of rare actinomycetes from mangrove ecosystem of Nizampatnam. Malaysian J. Microbiol. 8, 83–91. doi: 10.21161/mjm.03212
Mangamuri, U. K., Muvva Vijayalakshmi, M. V., and Sudhakar Poda, S. P. (2014). Exploration of actinobacteria from mangrove ecosystems of Nizampatnam and Coringa for antimicrobial compounds and industrial enzymes. Br. Biotechnol. J. 4, 173–184. doi: 10.9734/BBJ/2014/6424
Matsumoto, A., and Takahashi, Y. (2017). Endophytic actinomycetes: promising source of novel bioactive compounds. J. Antibiot. 70, 514–519. doi: 10.1038/ja.2017.20
Meenakshi, S., Hiremath, J., Meenakshi, M. H., and Shivaveerakumar, S. (2024). Actinomycetes: isolation, cultivation and its active biomolecules. J. Pure Appl. Microbiol. 18, 118–143. doi: 10.22207/JPAM.18.1.48
Mitousis, L., Thoma, Y., and Musiol-Kroll, E. M. (2020). An update on molecular tools for genetic engineering of actinomycetes—the source of important antibiotics and other valuable compounds. Antibiotics 9:494. doi: 10.3390/antibiotics9080494
Mitra, A., Santra, S. C., and Mukherjee, J. (2008). Distribution of actinomycetes, their antagonistic behaviour and the physico-chemical characteristics of the world’s largest tidal mangrove forest. Appl. Microbiol. Biotechnol. 80, 685–695. doi: 10.1007/s00253-008-1626-8
Mohamed, S. H., Al-Saeedi, T. A., and Sadik, A. S. (2013a). Halotolerant streptomycetes isolated from soil at Taif region, Kingdom of Saudi Arabia (KSA) I: purification, salt tolerance range, biological and molecular identification. Afr. J. Biotechnol. 12:946. doi: 10.5897/AJB2013.11946
Mohamed, S. H., Ghadeer, B. S., and Sadik, A. S. (2013b). Molecular studies on some soil-Streptomyces strains of western region, Kingdom of Saudi Arabia (KSA). Afr. J. Biotechnol. 12:945. doi: 10.5897/AJB2013.11945
Mohanraj, G., and Sekar, T. (2013). Isolation and screening of actinomycetes from marine sediments for their potential to produce antimicrobials. Int. J. Life Sci. Pharma Res. 2, 115–126.
Mothana, A. A., Al-Shamahy, H. A., Mothana, R. A., Khaled, J. M., Al-Rehaily, A. J., Al-Mahdi, A. Y., et al. (2022). Streptomyces sp. 1S1 isolated from southern coast of the Red Sea as a renewable natural resource of several bioactive compounds. Saudi Pharm. J. 30, 162–171. doi: 10.1016/j.jsps.2021.12.012
Muharram, M. M., Abdelkader, M. S., and Alqasoumi, S. I. (2013). Antimicrobial activity of soil actinomycetes isolated from Alkharj, KSA. Int. Res. J. Microbiol., 4, pp.12–20. Available online at: https://www.researchgate.net/publication/256543472 (Accessed March 20, 2025).
Nadeem, F., Oves, M., Qari, H., and Ismail, I. (2015). Red Sea microbial diversity for antimicrobial and anticancer agents. J. Mol. Biomark Diagn. 7:2. doi: 10.4172/2155-9929.1000267
Naghavi, M., Vollset, S. E., Ikuta, K. S., Swetschinski, L. R., Gray, A. P., Wool, E. E., et al. (2024). Global burden of bacterial antimicrobial resistance 1990–2021: a systematic analysis with forecasts to 2050. Lancet 404, 1199–1226. doi: 10.1016/S0140-6736(24)01867-1
Naikpatil, S. V., and Rathod, J. L. (2011). Selective isolation and antimicrobial activity of rare actinomycetes from mangrove sediment of Karwar. J. Ecobiotechnol., 3, pp.48–53. Available online at: https://updatepublishing.com/journal/index.php/jebt/article/view/192 (Accessed March 20, 2025).
Ngamcharungchit, C., Chaimusik, N., Panbangred, W., Euanorasetr, J., and Intra, B. (2023). Bioactive metabolites from terrestrial and marine actinomycetes. Molecules 28:5915. doi: 10.3390/molecules28155915
Nithya, K., Muthukumar, C., Biswas, B., Alharbi, N. S., Kadaikunnan, S., Khaled, J. M., et al. (2018). Desert actinobacteria as a source of bioactive compounds production with a special emphases on Pyridine-2, 5-diacetamide a new pyridine alkaloid produced by Streptomyces sp. DA3-7. Microbiol. Res. 207, 116–133. doi: 10.1016/j.micres.2017.11.012
Nithya, K., Muthukumar, C., Duraipandiyan, V., Dhanasekaran, D., and Thajuddin, N. (2015). Diversity and antimicrobial potential of culturable actinobacteria from desert soils of Saudi Arabia. J. Pharm. Sci. Res., 7, p.117–122. Available online at: https://www.researchgate.net/publication/306219794 (Accessed March 20, 2025).
Okoro, C. K., Brown, R., Jones, A. L., Andrews, B. A., Asenjo, J. A., Goodfellow, M., et al. (2009). Diversity of culturable actinomycetes in hyper-arid soils of the Atacama Desert, Chile. Antonie Van Leeuwenhoek 95, 121–133. doi: 10.1007/s10482-008-9295-2
Olano, C., and Rodríguez, M. (2024). Actinomycetes associated with arthropods as a source of new bioactive compounds. Curr. Issues Mol. Biol. 46, 3822–3838. doi: 10.3390/cimb46050238
Özakin, S., Davis, R. W., Umile, T. P., Pirinccioglu, N., Kizil, M., Celik, G., et al. (2016). The isolation of tetrangomycin from terrestrial Streptomyces sp. CAH29: evaluation of antioxidant, anticancer, and anti-MRSA activity. Med. Chem. Res. 25, 2872–2881. doi: 10.1007/s00044-016-1708-6
Pandey, A., Ali, I., Butola, K. S., Chatterji, T., and Singh, V. (2011). Isolation and characterization of Actinomycetes from soil and evaluation of antibacterial activities of Actinomycetes against pathogens. Int. J. Appl. Biol. Pharm. Technol. 2, 384–392.
Patel, J. D., Parmar, M., Patel, P., Rohit, P., Taviyad, R., Ansari, P., et al. (2014). Dynamism of antimicrobial activity of actinomycetes—a case study from undisturbed microbial niche. Adv. Microbiol. 4, 324–334. doi: 10.4236/aim.2014.46039
Pint, J.J. (2009). The lava caves of Khaybar, Saudi Arabia. In 15th International Congress of Speleology.
Qin, Z., Munnoch, J. T., Devine, R., Holmes, N. A., Seipke, R. F., Wilkinson, K. A., et al. (2017). Formicamycins, antibacterial polyketides produced by Streptomyces formicae isolated from African Tetraponera plant-ants. Chem. Sci. 8, 3218–3227. doi: 10.1039/C6SC04265A
Qureshi, K. A., Bholay, A. D., Rai, P. K., Mohammed, H. A., Khan, R. A., Azam, F., et al. (2021). Isolation, characterization, anti-MRSA evaluation, and in-silico multi-target anti-microbial validations of actinomycin X2 and actinomycin D produced by novel Streptomyces smyrnaeus UKAQ_23. Sci. Rep. 11:14539. doi: 10.1038/s41598-021-93285-7
Rabeh, S. A., Azab, E. A., and Aly, M. M. (2007). Studies on bacterioplankton and inhibitory strains of aquatic actinomycetes in Lake Bardawil, Egypt. World J. Microbiol. Biotechnol. 23, 167–176. doi: 10.1007/s11274-006-9206-5
Rajkumar, J., Swarnakumar, N. S., Sivakumar, K., Thangaradjou, T., and Kannan, L. (2012). Actinobacterial diversity of mangrove environment of the Bhitherkanika mangroves, east coast of Orissa, India. Int. J. Sci. Res. Publ. 2, 11–16.
Ramesh, S., and Mathivanan, N. (2009). Screening of marine actinomycetes isolated from the bay of Bengal, India for antimicrobial activity and industrial enzymes. World J. Microbiol. Biotechnol. 25, 2103–2111. doi: 10.1007/s11274-009-0113-4
Rangseekaew, P., and Pathom-Aree, W. (2019). Cave actinobacteria as producers of bioactive metabolites. Front. Microbiol. 10:387. doi: 10.3389/fmicb.2019.00387
Rateb, M. E., Houssen, W. E., Arnold, M., Abdelrahman, M. H., Deng, H., Harrison, W. T., et al. (2011). Chaxamycins A–D, bioactive ansamycins from a hyper-arid desert Streptomyces sp. J. Nat. Prod. 74, 1491–1499. doi: 10.1021/np200320u
Refaat, B. M., Helal, M., and Kobisi, A. A. (2017). Antimicrobial activities of some Actinomycete strains isolated from the Sinai Egypt soils. Egypt. Acad. J. Biol. Sci. G. Microbiol. 9, 21–31. doi: 10.21608/eajbsg.2017.16325
Retnowati, Y., Sembiring, L., Moeljopawiro, S., Djohan, T. S., and Soetarto, E. S. (2017). Diversity of antibiotic-producing actinomycetes in mangrove forest of Torosiaje, Gorontalo, Indonesia. Biodiv. J. Biol. Divers. 18, 1453–1461. doi: 10.13057/biodiv/d18032
Reyad, A. M. (2013). Diverse of enzymatically active actinomycetes associated with mangrove Rhizospher in Jazan coast. Ann. Biol. Res. 4, 100–108.
Ribeiro, I., Girão, M., Alexandrino, D. A., Ribeiro, T., Santos, C., Pereira, F., et al. (2020). Diversity and bioactive potential of actinobacteria isolated from a coastal marine sediment in northern Portugal. Microorganisms 8:1691. doi: 10.3390/microorganisms8111691
Röttig, A., Atasayar, E., Meier-Kolthoff, J. P., Spröer, C., Schumann, P., Schauer, J., et al. (2017). Streptomyces jeddahensis sp. nov., an oleaginous bacterium isolated from desert soil. Int. J. Syst. Evol. Microbiol. 67, 1676–1682. doi: 10.1099/ijsem.0.001839
Ruwandeepika, H. A. D., Fernando, G. C. P., and Jayaweera, T. S. P. (2022). “An overview of biomedical, biotechnological, and industrial applications of actinomycetes,” in Natural products from actinomycetes: Diversity, ecology and drug discovery. eds. R. V. Rai and J. A. Bai (Singapore: Springer), 475–508.
Saleem, M., Hassan, A., Li, F., Lu, Q., Ponomareva, L. V., Parkin, S., et al. (2023). Bioprospecting of desert actinobacteria with special emphases on griseoviridin, mitomycin C and a new bacterial metabolite producing Streptomyces sp. PU-KB10–4. BMC Microbiol. 23:69. doi: 10.1186/s12866-023-02770-8
Satheeja, S. V., and Jebakumar, S. R. (2011). Phylogenetic analysis and antimicrobial activities of Streptomyces isolates from mangrove sediment. J. Basic Microbiol. 51, 71–79. doi: 10.1002/jobm.201000107
Sathiyaseelan, K., and Stella, D. (2011). Isolation, identification and antagonistic activity of marine actinomycetes isolated from the Muthupet mangrove environment. Int. J. Pharm. Biol. Arch. 2, 1464–1468.
Sebak, M., Saafan, A. E., Abdelghani, S., Bakeer, W., Moawad, A. S., and El-Gendy, A. O. (2021). Isolation and optimized production of putative antimicrobial compounds from Egyptian soil isolate Streptomyces sp. MS. 10. BeniSuef Univ. J. Basic Appl. Sci. 10, 1–12. doi: 10.1186/s43088-021-00099-7
Selim, M. S. M., Abdelhamid, S. A., and Mohamed, S. S. (2021). Secondary metabolites and biodiversity of actinomycetes. J. Genetic Eng. Biotechnol. 19:72. doi: 10.1186/s43141-021-00156-9
Sengupta, S., Pramanik, A., Ghosh, A., and Bhattacharyya, M. (2015). Antimicrobial activities of actinomycetes isolated from unexplored regions of Sundarbans mangrove ecosystem. BMC Microbiol. 15, 170–116. doi: 10.1186/s12866-015-0495-4
Shamikh, Y. I., El Shamy, A. A., Gaber, Y., Abdelmohsen, U. R., Madkour, H. A., Horn, H., et al. (2020). Actinomycetes from the Red Sea sponge Coscinoderma mathewsi: isolation, diversity, and potential for bioactive compounds discovery. Microorganisms 8:783. doi: 10.3390/microorganisms8050783
Sharma, M., Dangi, P., and Choudhary, M. (2014). Actinomycetes: source, identification, and their applications. Int. J. Curr. Microbiol. App. Sci., 3, pp.801–832. Available online at: https://www.researchgate.net/publication/330910242 (Accessed March 20, 2025).
Sheik, G. B., Maqbul, M. S., Shankar, G., and Ranjith, M. S. (2017). Isolation and characterization of actinomycetes from soil of ad Dawadmi, Saudi Arabia and screening their antibacterial activities. Int. J. Pharm. Pharm. Sci. 9, 276–279. doi: 10.22159/ijpps.2017v9i10.15402
Shinwari, M. M., Alharbi, S. A., Ara, I., Wainwright, M., and Khiyami, M. A. (2013). Gas chromatography and mass Spectrum analysis of the selective diversity of Actinobacteria isolated from extreme environments in Saudi Arabia. J. Pure Appl. Microbiol. 7, 107–116. doi: 10.13140/RG.2.2.28557.13285
Shivlata, L., and Satyanarayana, T. (2015). Thermophilic and alkaliphilic Actinobacteria: biology and potential applications. Front. Microbiol. 6:1014. doi: 10.3389/fmicb.2015.01014
Sibanda, T., Mabinya, L. V., Mazomba, N., Akinpelu, D. A., Bernard, K., Olaniran, A. O., et al. (2010). Antibiotic producing potentials of three freshwater actinomycetes isolated from the eastern Cape Province of South Africa. Int. J. Mol. Sci. 11, 2612–2623. doi: 10.3390/ijms11072612
Singh, R., and Dubey, A. K. (2018). Diversity and applications of endophytic actinobacteria of plants in special and other ecological niches. Front. Microbiol. 9:1767. doi: 10.3389/fmicb.2018.01767
Siro, G., and Pipite, A. (2024). Mini-review on the antimicrobial potential of actinobacteria associated with seagrasses. Explorat. Drug Sci. 2, 117–125. doi: 10.37349/eds.2024.00038
Subramani, R., and Aalbersberg, W. (2012). Marine actinomycetes: an ongoing source of novel bioactive metabolites. Microbiol. Res. 167, 571–580. doi: 10.1016/j.micres.2012.06.005
Subramani, R., and Aalbersberg, W. (2013). Culturable rare Actinomycetes: diversity, isolation and marine natural product discovery. Appl. Microbiol. Biotechnol. 97, 9291–9321. doi: 10.1007/s00253-013-5229-7
Subramani, R., and Sipkema, D. (2019). Marine rare actinomycetes: a promising source of structurally diverse and unique novel natural products. Mar. Drugs 17:249. doi: 10.3390/md17050249
Thabit, A. K., Alabbasi, A. Y., Alnezary, F. S., and Almasoudi, I. A. (2023). An overview of antimicrobial resistance in Saudi Arabia (2013–2023) and the need for national surveillance. Microorganisms 11:2086. doi: 10.3390/microorganisms11082086
Tiwari, K., and Gupta, R. K. (2012). Rare actinomycetes: a potential storehouse for novel antibiotics. Crit. Rev. Biotechnol. 32, 108–132. doi: 10.3109/07388551.2011.562482
Tiwari, K., and Gupta, R. K. (2013). Diversity and isolation of rare actinomycetes: an overview. Crit. Rev. Microbiol. 39, 256–294. doi: 10.3109/1040841X.2012.709819
Usha, R., Ananthaselvi, P., Venil, C. K., and Palaniswamy, M. (2010). Antimicrobial and antiangiogenesis activity of Streptomyces parvulus KUAP106 from mangrove soil. Eur. J. Biol. Sci. 2, 77–83.
Valli, S., Suvathi, S. S., Aysha, O. S., Nirmala, P., Vinoth, K. P., and Reena, A. (2012). Antimicrobial potential of Actinomycetes species isolated from marine environment. Asian Pac. J. Trop. Biomed. 2, 469–473. doi: 10.1016/S2221-1691(12)60078-1
van Bergeijk, D. A., Terlouw, B. R., Medema, M. H., and van Wezel, G. P. (2020). Ecology and genomics of Actinobacteria: new concepts for natural product discovery. Nat. Rev. Microbiol. 18, 546–558. doi: 10.1038/s41579-020-0379-y
Wei, Y. Z., Zhang, Y. Q., Zhao, L. L., and Li, Q. P. (2010). Isolation, screening and preliminary identification of endophytic actinobacteria from mangroves at Shankou of Guangxi Province. Microbiology 37, 823–828.
Yasir, M., Qureshi, A. K., Khan, I., Bibi, F., Rehan, M., Khan, S. B., et al. (2019). Culturomics-based taxonomic diversity of bacterial communities in the hot springs of Saudi Arabia. Omics 23, 17–27. doi: 10.1089/omi.2018.0176
Yezli, S., and Memish, Z. A. (2012). Tuberculosis in Saudi Arabia: prevalence and antimicrobial resistance. J. Chemother. 24, 1–5. doi: 10.1179/1120009X12Z.0000000001
Yücel, S. E., and Yamaç, M. U. (2010). Selection of Streptomyces isolates from Turkish karstic caves against antibiotic resistant microorganisms. Pak. J. Pharm. Sci. 23, 1–6
Zenova, G. M., Manucharova, N. A., and Zvyagintsev, D. G. (2011). Extremophilic and extremotolerant actinomycetes in different soil types. Eurasian Soil Sci. 44, 417–436. doi: 10.1134/S1064229311040132
Zhu, H., Swierstra, J., Wu, C., Girard, G., Choi, Y. H., Van Wamel, W., et al. (2014). Eliciting antibiotics active against the ESKAPE pathogens in a collection of actinomycetes isolated from mountain soils. Microbiology 160, 1714–1725. doi: 10.1099/mic.0.078295-0
Ziyat, A., Nurgozhina, A., Sergazy, S., and Nurgaziev, M. (2019). Novel actinobacterial diversity in Kazakhstan deserts soils as a source of new drug leads. J. Microbiol. Biotechnol. Food Sci. 8, 1057–1065. doi: 10.15414/jmbfs.2019.8.4.1057-1065
Zowawi, H. M. (2016). Antimicrobial resistance in Saudi Arabia: an urgent call for an immediate action. Saudi Med. J. 37, 935–940. doi: 10.15537/smj.2016.9.16139
AMR - Antimicrobial resistance
WHO - World Health Organization
MDR - Multidrug-resistant
MRSA - Methicillin-resistant Staphylococcus aureus
VRSA - Vancomycin-resistant Staphylococcus aureus
VRE - Vancomycin-resistant Enterococcus spp
PRSP - Penicillin-resistant Streptococcus pneumoniae
ESBL - Extended-spectrum beta-lactamase
E. coli - Escherichia coli
K. pneumoniae - Klebsiella pneumoniae
CRE - Carbapenem-resistant Enterobacteriaceae
P. aeruginosa - Pseudomonas aeruginosa
A. baumannii - Acinetobacter baumannii
MDR-TB - Multidrug-resistant Mycobacterium tuberculosis
BGCs - Biosynthetic gene clusters
PKSs - Polyketide synthases
NRPSs - Non-ribosomal peptide synthetases
S. aureus - Staphylococcus aureus
B. cereus - Bacillus cereus
S. typhi - Salmonella typhi
C. albicans - Candida albicans
A. niger - Aspergillus niger
A. flavus - Aspergillus flavus
S. rochei - Streptomyces rochei
B. subtilis - Bacillus subtilis
S. enteritidis - Salmonella enteritidis
A. fumigatus - Aspergillus fumigatus
F. solani - Fusarium solani
S. sonnei - Shigella sonnei
E. faecalis - Enterococcus faecalis
S. pyogenes - Streptococcus pyogenes
MIC - Minimum inhibitory concentration
T. cruzi - Trypanosoma cruzi
F. oxysporum - Fusarium oxysporum
S. typhimurium - Salmonella typhimurium
P. mirabilis - Proteus mirabilis
E. faecium - Enterococcus faecium
M. luteus - Micrococcus luteus
S. enterica - Salmonella enterica
S. cerevisiae - Saccharomyces cerevisiae
S. epidermidis - Staphylococcus epidermidis
S. pneumoniae - Streptococcus pneumoniae
S. marcescens - Serratia marcescens
S. agalactiae - Streptococcus agalactiae
C. neoformans - Cryptococcus neoformans
T. mentagrophytes - Trichophyton mentagrophytes
A. alternata - Alternaria alternata
omics - genomics, transcriptomics, proteomics, and metabolomics
OSMAC - “One Strain–Many Compounds”
CRISPR - Clustered Regularly Interspaced Short Palindromic Repeats
MS - Mass Spectrometry
LC - Liquid Chromatography
GC - Gas Chromatography
NMR - Nuclear Magnetic Resonance spectroscopy
Keywords: actinomycetes, Streptomyces, antimicrobial activity, antimicrobial resistance, extreme environment, bioactive compound, Saudi Arabia
Citation: Helmi NR (2025) Exploring the diversity and antimicrobial potential of actinomycetes isolated from different environments in Saudi Arabia: a systematic review. Front. Microbiol. 16:1568899. doi: 10.3389/fmicb.2025.1568899
Received: 30 January 2025; Accepted: 13 March 2025;
Published: 26 March 2025.
Edited by:
Piotr Majewski, Medical University of Bialystok, PolandReviewed by:
D. Ipek Kurtböke, University of the Sunshine Coast, AustraliaCopyright © 2025 Helmi. This is an open-access article distributed under the terms of the Creative Commons Attribution License (CC BY). The use, distribution or reproduction in other forums is permitted, provided the original author(s) and the copyright owner(s) are credited and that the original publication in this journal is cited, in accordance with accepted academic practice. No use, distribution or reproduction is permitted which does not comply with these terms.
*Correspondence: Noof Refat Helmi, bmhlbG1pQGthdS5lZHUuc2E=
Disclaimer: All claims expressed in this article are solely those of the authors and do not necessarily represent those of their affiliated organizations, or those of the publisher, the editors and the reviewers. Any product that may be evaluated in this article or claim that may be made by its manufacturer is not guaranteed or endorsed by the publisher.
Research integrity at Frontiers
Learn more about the work of our research integrity team to safeguard the quality of each article we publish.