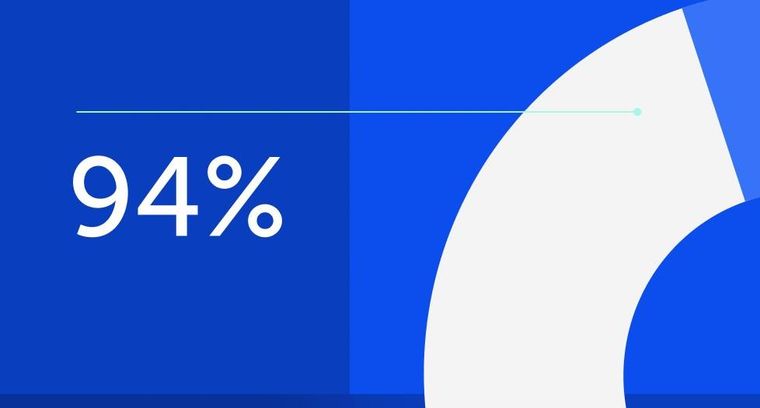
94% of researchers rate our articles as excellent or good
Learn more about the work of our research integrity team to safeguard the quality of each article we publish.
Find out more
ORIGINAL RESEARCH article
Front. Microbiol., 01 April 2025
Sec. Microbial Physiology and Metabolism
Volume 16 - 2025 | https://doi.org/10.3389/fmicb.2025.1567054
Introduction: Strain ALG19, a predominant culturable bacterium isolated from the larval gut of the emerald ash borer (Agrilus planipennis) infesting velvet ash (Fraxinus velutina), was investigated to determine its taxonomic identity and evaluate its cellulose-degrading potential.
Methods: The taxonomic classification of ALG19 was determined through whole-genome sequencing, average nucleotide identity (ANI) analysis, and phylogenetic reconstruction based on single-copy orthologous genes. Functional annotation of carbohydrate-active genes was performed using the COG, KEGG, and CAZy databases. Cellulolytic activity was assessed using a multi-faceted approach. First, carboxymethyl cellulose hydrolysis assays were conducted to evaluate cellulolytic capability. Additionally, filter paper degradation and the utilization of velvet ash phloem cellulose were examined. For these experiments, the strain was cultured in an inorganic salt medium supplemented with the respective cellulose substrates for 60 days.
Results: Genomic analyses confirmed that ALG19 belongs to Delftia tsuruhatensis. The strain harbors 283 COG-annotated genes associated with carbohydrate transport and metabolism, 355 KEGG genes involved in carbohydrate metabolism pathways, and 105 CAZy-annotated carbohydrate-active enzymes. Phenotypic assays revealed a carboxymethyl cellulose hydrolysis zone ratio of 1.74. After a 60-day incubation period, ALG19 completely decomposed filter paper strips into flocs, resulting in a 38.06% reduction in dry weight compared to control samples, which basically retained their original shape. Furthermore, the strain degraded velvet ash phloem cellulose, leaving a residual content of 69.91%. This was 15.60% lower than the control, which exhibited a residual content of 82.83%.
Discussion: These findings demonstrate that D. tsuruhatensis ALG19 is capable of degrading cellulose present in the host plant of the emerald ash borer, its associated insect. This study identifies a potential target microorganism for future pest management strategies, which could mitigate the damage caused by the emerald ash borer by impairing its digestive capacity.
The emerald ash borer (Agrilus planipennis), a highly destructive pest, has significantly impacted the economy and ecology of regions where ash trees (Fraxinus spp.) are found. Originating in Northeast Asia, its distribution spans China, Japan, and Korea, among other Asian countries (Wei et al., 2004). Through transportation, it has spread to many other countries, including the United States, Russia, and Ukraine. It is now recognized as the most damaging and economically costly invasive forest pest in North America and Europe (Nisbet et al., 2015; Bauer et al., 2015). Furthermore, susceptible ash trees imported from North America, such as velvet ash (F. velutina), green ash (F. pennsylvanica), and white ash (F. americana), have suffered severe damage from the emerald ash borer (Sun et al., 2024). For instance, in Beijing, China, the damaged area of ash forests due to the emerald ash borer reached 210 hectares in 2017 (Bureau Beijing Gardening and Greening, 2017). The emerald ash borer larvae primarily feed on the phloem of ash trees, which contains cellulose, a difficult-to-decompose component of cell walls (Cappaert et al., 2005). Therefore, to feed on ash trees, the emerald ash borer must digest the cellulose present in the trees.
Gut bacteria form a complex micro-ecosystem within insects and have a close symbiotic relationship with them (Pan et al., 2020). Studies have shown that the composition of gut bacteria is influenced by the insect’s diet (Li C. et al., 2022; Li J. et al., 2022). For instance, in the European corn borer (Ostrinia nubilalis), a diet of corn has been shown to shape the gut bacterial community, enabling the insect to utilize specific bacteria for the digestion of corn lignocellulose (Li C. et al., 2022; Li J. et al., 2022). These bacteria play a crucial role in nutrient digestion, producing various digestive enzymes that help the host insect degrade indigestible food components, such as cellulose (Jing et al., 2020). By studying the cellulose degradation function of gut bacteria in herbivorous insects, like the emerald ash borer, we can gain a deeper understanding of their adaptability to host plants. Furthermore, research on gut bacteria with cellulose degradation capabilities has broader implications beyond insect biology. These bacteria can also be used directly or indirectly for the degradation of biomass resources like straw (Jeong et al., 2024), and have the potential to reduce pollution from industrial wastewater in aquatic ecosystems by degrading lignin produced in the paper-making industry (Saha et al., 2022). Furthermore, there is potential for developing low-toxicity insecticides that target cellulose-degrading bacteria, thereby weakening the digestive ability of insects.
Current research has revealed that many insect gut bacteria have the ability to degrade cellulose. Using traditional methods, two Bacillus strains with cellulose-degrading capabilities were isolated from fall armyworm larvae (Spodoptera frugiperda) (Zhang et al., 2023). Similarly, 45 strains with cellulose-degrading capabilities were isolated from the intestines of white-striped long-horned beetle larvae (Batocera horsfieldi), with two strains from the genera Ochrobactrum and Raoultella being particularly efficient cellulase producers (Su et al., 2024). In red palm weevil larvae (Rhynchophorus ferrugineus), seven species from the genera Klebsiella, Serratia, Enterobacter, and Citrobacter were found to degrade cellulose (Muhammad et al., 2017). Additionally, five bacterial species isolated from termite guts were confirmed to have cellulose-degrading abilities, including Paenibacillus lactis, Lysinibacillus macroides, Stenotrophomonas maltophilia, Lysinibacillus fusiformis, and Bacillus cereus (Ali et al., 2019). To date, 63 bacterial genera with cellulose-degrading functions have been documented (Liu et al., 2024). However, it is believed that many more insect gut bacteria with this capability remain undiscovered and unused. This has driven continued interest in studying gut bacteria with microbial degradation functions, particularly in insects with strong feeding capacities and high destructive potential.
Carboxymethyl cellulose is frequently used to assess the function of cellulose degradation. It is documented that out of 46 studies focused on gut bacteria in insects with cellulose degradation capabilities, 42 studies utilized carboxymethyl cellulose to verify the degradation function of strains. The remaining studies used filter paper cellulose, microcrystalline cellulose, or food digestibility as indicators (Liu et al., 2024). While carboxymethyl cellulose offers simplicity and feasibility in cellulose degradation tests, it also has limitations. Specifically, although both carboxymethyl cellulose (C8H16O8) and plant cellulose [(C6H10O5)n] are polysaccharides, they differ in chemical structure and physical properties (Sawant et al., 2023). Carboxymethyl cellulose is a water-soluble, chemically modified cellulose ether that microorganisms can easily degrade. In contrast, plant cellulose decomposition is influenced by crystallinity and requires specific cellulases (Fu, 2019). Using plant cellulose in research provides a more accurate reflection of gut bacterial ability to digest host plant nutrients in insects. This approach will provide a stronger scientific basis for developing control strategies that target gut bacteria capable of cellulose degradation.
Strain ALG19 is a dominant dominant bacterium among the culturable gut microbiota of emerald ash borer larvae. In this study, taxonomic identification and carbohydrate hydrolysis capability analysis of strain ALG19 were conducted using genome sequencing. To further evaluate its cellulose degradation capabilities, experiments were conducted using carboxymethyl cellulose, filter paper cellulose, and phloem cellulose extracted from velvet ash, the host plant of the emerald ash borer. The ultimate goal of this research was to elucidate the ability of gut bacteria to assist host insects in digesting nutrients, providing a foundation for pest management strategies targeting insect gut bacteria.
The test strain ALG19 was isolated from the guts of the emerald ash borer larvae collected from the infected velvet ash forest in the “Millennium Show Forest” in Xiong’an New Area, China. It was inoculated into the Luria-Bertani liquid medium and cultured with constant temperature shaker at 30°C and 180 r/min until the suspension became turbid. The obtained suspension was used for genomic DNA extraction using the PureLink® Genomic DNA Kit (Invitrogen, CA, United States). Whole-genome sequencing was performed using the Illumina HiSeq novaseq6000 sequencing system (Illumina, CA, United States) and PacBio technology. The sequencing depth of the second-generation data is 158×, and the sequencing depth of the third-generation data is 79×. The genome sequencing work was undertaken by Shanghai Majorbio Bio-Pharm Technology Co., Ltd. (Shanghai, China).
Genomic information of seven strains of Delftia tsuruhatensis were compared with strain ALG19. Those seven strains were isolated from different sources, and their accession in Genbank and source were list in Table 1. Benchmarking Universal Single-Copy Orthologs (BUSCO) was used to evaluate the completeness of bacterial genome (Manni et al., 2021). The final completion map of the sequencing results was drawn by the Prodigal software (Hyatt et al., 2010). The average nucleotide identity (ANI) of the genome was calculated to measure the nucleotide-level genomic similarity among analyzed strains (Kastner et al., 2024). A species tree was generated using OrthoFinder1 with the Species Tree of All Genes (STAG) algorithm (Emms and Kelly, 2019), and visualized by the FigTree software2 (Boore, 2006). Meanwhile, the genome of strain ALG19 was subjected to COG functional annotation, KEGG metabolic pathway annotation and CAZy gene annotation to predict the potential of strain ALG19 to degrade cellulose. All genome analyses were completed on the Majorbio Cloud Platform (Ren et al., 2022).
A single colony of the ALG19 strain was inoculated into the Luria-Bertani liquid medium and then cultured at 30°C and 180 r/min for 24 h to obtain the bacterial suspension, which was subsequently used for the determination of carboxymethyl cellulose degradation capacity as previously reported method (Li et al., 2021). Take 18 mL of the carboxymethyl cellulose Congo red medium and pour it into a Petri dish with diameter of 9 cm. After the medium has solidified, use a puncher with diameter of 8 mm to create two symmetrical holes. Subsequently, add 70 μL of sterile water (used as the control) and the suspension of strain ALG19, respectively. The Petri dish was sealed with parafilm and then incubated at 30°C for 3 days. Three biological replicates were performed to eliminate errors. The ratio of the diameter of the degradation circle (D) to the diameter of the punched hole (d) was calculated to evaluate the degradation capacity of the ALG19 strain on carboxymethyl cellulose.
An inorganic salt liquid medium was prepared according to the following formulation: 1 g of (NH4)2SO4, 3 g of KH2PO4, 7 g of K2HPO4, 0.1 g of MgSO4·7H2O, and 1,000 mL of H2O. A single colony of strain ALG19 was inoculated into 25 mL of inorganic salt liquid medium, and then cultured at 30°C and 180 r/min for 12 h. The resulting bacterial suspension was used to assess the ability of strain ALG19 to degrade both filter paper cellulose and phloem cellulose extracted from velvet ash, the host plant of the emerald ash borer. Subsequently, a conical flask with 100 mL of inorganic salt medium was prepared and mixed with 2 mL of bacterial suspension and 3 sterile filter paper strips (1 cm × 4 cm, 0.04 g each). The mixture was incubated at 30°C and 180 r/min for 60 days. A control was prepared using bacterial suspension sterilized at 121°C under high pressure steam for 30 min. The experiment was repeated three times. The filter paper’s disintegration was observed, the residue was filtered, dried at 50°C, weighed, and the degradation rate was calculated to study the degradation capacity of the ALG19 strain on filter paper cellulose.
Cellulose extracted from the phloem of velvet ash was also used to test the degradation capacity of strain ALG19. The phloem was collected from healthy plants using a peeling knife, washed with distilled water to remove impurities, and dried at 60°C until it reached a constant weight. The dried phloem was pulverized into powder and passed through a 100-mesh sieve for cellulose extraction. To extract the cellulose, an optimized HNO3-NaOH method was employed (Wen et al., 2017). The procedure involved mixing the phloem powder with a 3% HNO3 solution at a liquid-to-solid ratio of 35 mL/g and heating it at 90.7°C for 2.9 h. The mixture was then filtered and washed until it reached a neutral pH. An equal volume of 3% NaOH solution was added to the acid-treated phloem, and the mixture was heated at 90.7°C for another 1.5 h. Once cooled to about 75°C, H2O2 was introduced to reach a concentration of 5%, and the heating continued for an additional 0.5 h. The extracted cellulose was then filtered, washed with distilled water until neutral pH, dried at 60°C, and sterilized at 121°C for 30 min.
For the degradation assay, 0.5 g of the sterilized ash cellulose was mixed with 25 mL of inorganic salt medium and 2 mL of the bacterial suspension of strain ALG19. Subsequently, the resulting mixture was incubated at 30°C and 180 r/min for 60 days. After incubation, the mixture was centrifuged at 8,000 r/min for 10 min to obtain the precipitate. The precipitate was then dried at 70°C until it reached a constant weight. The cellulose content in the precipitate was measured using a cellulose content kit (Grace Bio-Tech, Suzhou, China), and the degradation rate of the ash cellulose was calculated to evaluate the degradation capacity of strain ALG19. A control was prepared using an equal volume of bacterial suspension inactivated at 121°C for 30 min. The experiment was repeat three times to reduce error.
Variance homogeneity and normal distribution were assessed to determine the suitability of parametric or non-parametric tests. Based on the results, the relationship between the hydrolysis circle of strain ALG19 and the diameter of the punched hole was conducted using Kruskal–Wallis tests. Additionally, the dry weight of the centrifugal precipitate from filter paper strips and the cellulose content in the extracted phloem cellulose from velvet ash were analyzed using Student’s t-tests. All statistical analyses were performed using IBM SPSS Statistics 21, and significance was set at p < 0.05.
The genome of strain ALG19 is 6,796,678 base pairs long. It contains 6,146 coding genes, which total 6,092,280 base pairs in length, with an average gene length of 991 bp. The genome has a GC content of 67.43%. Figure 1A illustrates the genomic features of ALG19 using a Circos plot. To determine its phylogenetic position, the 16S rRNA sequence of ALG19 was used to build a phylogenetic tree (Figure 1B), preliminarily identifying it as D. tsuruhatensis. To further confirm its classification, seven D. tsuruhatensis strains isolated from diverse environments—including mice, humans, seawater, soil, hospital sink, the mosquito Anopheles stephensi, and wastewater—were chosen for comparative genomic studies. These strains had similar genome sizes, a comparable number of protein-coding genes, and GC contents ranging from 66.5 to 68%. Additionally, their BUSCO assembly quality scores were all above 98%, making them suitable for the analysis. The analysis revealed that except BB1455 from wastewater, which had an ANI value of only 90.14%, other strains had ANI values above 98.50% when compared to ALG19. A phylogenetic tree based on single-copy orthologous genes (Figure 1C) further confirmed that ALG19 grouped with the other six D. tsuruhatensis strains, excluding BB1455. This evidence strongly supports the classification of strain ALG19 as part of the D. tsuruhatensis species.
Figure 1. Genomic analysis of strain ALG19. (A) Circos plot illustrating the genomic features of strain ALG19. (B) Phylogenetic tree showing the genetic relationship between strain ALG19 and other related strains, as inferred from 16S rRNA sequence data. (C) Phylogenetic analysis based on single-copy orthologous genes, including strain ALG19 and seven D. tsuruhatensis strains.
A total of 5,084 genes were categorized into 25 functional clusters in the COG database. Notably, no genes were assigned to the nuclear structure cluster (cluster Y). Among the annotated genes, 283 were associated with the carbohydrate transport and metabolism cluster (cluster G). In terms of gene count, cluster G ranked ninth, with eight other clusters having a higher number of annotated genes, ranging from 308 to 613 genes (Figure 2A). In the KEGG database, 4,630 genes were annotated. Of these, 3,443 genes related to metabolism, comprising 74.36% of the annotated genes and dominating the biological processes category. Within the metabolism-related genes, 355 were associated with the carbohydrate metabolism pathway. In terms of gene abundance, 476 genes were associated with carbohydrate metabolism, while 1,349 genes were linked to the global and overview maps pathway (Figure 2B).
Figure 2. Gene annotation results for strain ALG19. (A) Annotation of COG function clusters. (B) Annotation of KEGG pathways. (C) Annotation within the CAZy database. (B) The clusters include: CP for cellular processes, EIP for environmental info processing, GIP for genetic info processing, HD for human diseases, Metab for metabolism, and OS for organismal systems. (C) Displays the count of genes annotated within gene families including glycoside hydrolase (GH), glycosyltransferase (GT), carbohydrate esterase (CE), carbohydrate-binding module (CBM), polysaccharide lyase (PL), and auxiliary activity (AA).
Annotation within the CAZy database revealed 105 carbohydrate-active enzyme genes in D. tsuruhatensis ALG19, including 22 glycoside hydrolase (GH) genes, 28 glycosyltransferase (GT) genes, 29 carbohydrate esterase (CE) genes, 2 carbohydrate-binding module (CBM) genes, 2 polysaccharide lyase (PL) genes, and 22 auxiliary activity (AA) genes. Furthermore, the GH genes were classified into 10 families, with the GH109 family having five genes, the GH24 family having five genes, and the GH23 family having four genes. The GT genes were distributed across 10 families, with the GT41 family being the most prevalent with seven genes. The CE genes were categorized into eight families, with the CE1 family having the largest number at 12 genes. The two CBM genes were from the CBM13 and CBM50 families, respectively, and both PL genes belonged to the PL9 family. The AA genes were divided into five families, with the AA3 family having the highest count of 10 genes (Figure 2C). The collective annotation results from the COG, KEGG, and CAZy databases strongly support the capability of D. tsuruhatensis ALG19 to degrade cellulose.
The Congo red assay showed that strain ALG19 produced a conspicuous hydrolysis circle, but no such prominent circle was observed in the control group. The diameter ratio of the hydrolysis circle of strain ALG19 to the diameter of the punched hole was 1.74, which was markedly higher than that in the control group (df = 1, χ2 = 4.355, p = 0.037). This finding suggests that D. tsuruhatensis strain ALG19 possesses the capacity to degrade carboxymethyl cellulose (Figures 3A,D).
Figure 3. Cellulose degradation efficiency of strain ALG19 on three cellulose variants. (A,E) Demonstrate its ability to degrade carboxymethyl cellulose. (C,D) Its capacity to degrade filter paper cellulose, with (B) serving as a control for the filter paper. The final panel (F) illustrates ALG19’s ability to degrade the cellulose found in the phloem of the host plant, velvet ash. The data are expressed as mean values, with the error bars in the figures indicating the standard error.
The filter paper cellulose degradation experiment indicated that following a 60-day incubation in the inorganic salt medium, the filter paper strips in the control group presented rough edges, disintegration at corners, and central splits, while still largely maintained their initial shape. In contrast, inoculation with live strain ALG19 result in significant pronounced disintegration, with the filter paper strips completely broken down into flocculent particles. The dry weight of the centrifugal recovery product in the treatment group was 0.074 g, significantly lower than the 0.103 g in the control group (df = 4, t = 7.294, p = 0.002). The disintegration rate attained 38.06%, which was 2.69 times that of the control group. Consequently, it can be inferred that D. tsuruhatensis ALG19 exhibits a capacity to degrade filter paper cellulose (Figures 3B,C,E).
Subsequent tests of degradative capability revealed that after 60 days of co-cultivation in an inorganic salt medium, the cellulose content in the extracted phloem cellulose from velvet ash was reduced to 69.91% when inoculated with the live strain ALG19. This was significantly lower than the 82.83% observed in the control group (Figure 3F, df = 16, t = 3.028, p = 0.008). The calculated degradation rate was 15.60%. These results indicate that the gut bacterium D. tsuruhatensis ALG19, isolated from emerald ash borer larvae, has the ability to degrade the cellulose in the phloem of the host plant, velvet ash.
Investigations into the gut microbiota of the emerald ash borer have revealed that the bacterial constituents predominantly consist of genera within the class Gammaproteobacteria in adult emerald ash borers, such as Pantoea, Escherichia, Shigella, Pseudomonas, and Chryseobacterium. Notably, Pantoea has been identified as the dominant bacterial genus in the gut of the emerald ash borer (Mogouong et al., 2020). Furthermore, studies focusing on the emerald ash borer larvae have revealed that five bacterial phyla and 39 bacterial families have been detected, including Pseudomonadaceae, Erwiniaceae, and Enterobacteriaceae (Vecherskii et al., 2022). Pseudomonas was the dominant genus during the larval phase (Vasanthakumar et al., 2008). This study focus on the culturable bacterial strain ALG19, which is present in the gut of the emerald ash borer larvae. It exhibits the highest isolation rate in our previous culturable gut bacteria isolation experiments, prompting further investigation. The strain was identified as D. tsuruhatensis based on genome sequencing.
The genus Delftia was established as a novel bacterial genus in 1999 by Australian scholar Wen and colleagues (Wen et al., 1999). It was established through the analysis of 16S rRNA gene sequences, resulting in the segregation of Comamonas acidovorans from the genus Comamonas. From a phylogenetic perspective, the genus Delftia is taxonomically classified within the family Comamonadaceae, order Burkholderiales, β-subclass of the Proteobacteria. It is worth noting that D. tsuruhatensis is not a newly discovered bacterial species in insect guts. In fact, it has been previously isolated from the gut of Anopheles mosquitoes (Pierre et al., 2023). Furthermore, existing research has demonstrated that D. tsuruhatensis XSP-1, isolated from the sludge of a pesticide factory, exhibits the capacity to degrade pesticides such as methyl parathion, chlorpyrifos, and fenitrothion (Han et al., 2005). Additionally, D. tsuruhatensis AD4, sourced from activated sludge, has been shown to possess a high proficiency in degrading the toxic substance aniline (Yuan et al., 2023). These findings suggest that D. tsuruhatensis may assist insects in adapting to the stress induced by toxic organic compounds, including pesticides. It is also conceivable that this bacterium could be implicated in the adaptation of the emerald ash borer to the defensive substances produced by host plants.
Nutrient digestion is a crucial function of insect gut bacteria (Holtof et al., 2019). However, our literature review revealed no studies on the cellulose degradation capacity of D. tsuruhatensis. Nevertheless, the emerald ash borer’s ability to digest indigestible substances such as cellulose is essential for its feeding on host plants. This prompted us to investigate the cellulose degradation capacity of D. tsuruhatensis ALG19. Building upon previous research, the current study has not only confirmed the ability of strain ALG19 to degrade carboxymethyl cellulose and filter paper cellulose but has also demonstrated its capacity to degrade the phloem cellulose of velvet ash, a preferred host plant of the emerald ash borer. The degradation of velvet ash phloem cellulose suggests that D. tsuruhatensis ALG19 may enhance the emerald ash borer’s digestive capacity and potentially facilitate its adaptation to the host plant. This discovery provides a fundamental basis for a pest management strategy that aims to impair or eliminate the insects’ digestive capabilities by targeting gut bacteria with cellulose degradation capacity (Siddiqui and Khan, 2023). Furthermore, D. tsuruhatensis ALG19 may have direct or indirect applications in the degradation of lignin produced by the paper-making industry, thereby mitigating pollution of aquatic ecosystems from industrial wastewater (Li et al., 2023), or in the degradation of biomass resources such as straw (Chen et al., 2024).
One of the key features of this study is the verification of the cellulose degradation capacity using phloem cellulose extracted from the host plant of the emerald ash borer. This approach differs from previous studies that predominantly relied on carboxymethyl cellulose and filter paper cellulose as substrates (Liu et al., 2024). It is worth noting that different types of celluloses exhibit variations in both chemical structure and physical properties (Sawant et al., 2023). By using the phloem cellulose of the host plant as the exclusive carbon source for validating cellulose degradation, this study provides more reliable evidence regarding the nutrient digestion capacity of insect gut bacteria. However, despite our efforts to screen and optimize the reported cellulose extraction method (results not presented), the purity of the extracted phloem cellulose of velvet ash only reached 84.78%, which is still far below our ideal target of >90% purity. Additionally, in the degradation assays of filter paper cellulose and velvet ash cellulose conducted in this study, the degradation rates were recorded as 38.06 and 15.60% respectively, following a 60 d incubation period. This relatively low degradation rate can be attributed to the choice of medium. To avoid the confounding effects of a nutrient-rich environment, an oligotrophic inorganic salt medium was chosen, with filter paper cellulose or velvet ash cellulose serving as the sole carbon source. This significantly limited the growth of D. tsuruhatensis ALG19 and the degradation rates. The 60-day incubation period, while necessary to observe measurable degradation under oligotrophic conditions, may have further constrained the degradation efficiency due to the prolonged nutrient limitation and potential accumulation of metabolic by-products that could inhibit bacterial activity. Therefore, future studies could explore shorter incubation periods with optimized inorganic salt media that better support the growth of strain ALG19, to more accurately assess its cellulose degradation potential.
Furthermore, this study focuses solely on the cellulose degradation function of D. tsuruhatensis ALG19, thus providing an incomplete picture of its ecological role. As previously mentioned, the capacity of other D. tsuruhatensis strains to degrade toxic substances may assist the emerald ash borer in adapting to the defensive compounds of the host plant. However, further research is needed to verify these inferences and identify the specific defensive substances of the host plant that D. tsuruhatensis can degrade. Previous research has also shown that D. tsuruhatensis NF83-1 exhibits pronounced inhibitory effects against pathogenic fungi such as Rhizoctonia solani, Alternaria brassicae, and Sclerotinia sclerotiorum, as well as pathogenic bacteria including Xanthomonas oryzae pv. oryzicola, Erwinia amylovora, and Ralstonia solanacearum (Chen et al., 2024). This suggests that D. tsuruhatensis has potential as a biological agent for plant diseases. Therefore, the ecological role and functional attributes of D. tsuruhatensis ALG19 in nature warrant further in-depth research and exploration.
Given the multifaceted capabilities of D. tsuruhatensis, its potential applications extend beyond plant disease control to pest management. In the field of pest management, future research could focus on identifying the key enzymes in the ALG19 strain responsible for degrading ash cellulose and developing specific cellulase inhibitors targeting ALG19’s enzymatic machinery. Such efforts would provide a foundation for designing precision inhibitors to block symbiotic cellulose digestion in the ash borer gut ecosystem, thereby disrupting larval nutrient acquisition. This approach mirrors the developmental trajectory of Ledprona, the first sprayable RNA interference (RNAi) biopesticide. Before Ledprona’s introduction, RNAi lacked practical pest control applications, but its success demonstrated how foundational research on nucleic acid delivery mechanisms enabled market-ready solutions. Although still in the research phase, this approach aligns with emerging trends in microbiome-mediated pest management, highlighting its potential for future applications.
The bacterial strain ALG19, isolated from the gut of emerald ash borer larvae, was identified as D. tsuruhatensis. Genomic annotations in the COG, KEGG, and CAZy databases confirm the capability of D. tsuruhatensis ALG19 to degrade cellulose. Further assays confirmed that strain ALG19 possesses the ability to degrade carboxymethyl cellulose, filter paper cellulose, and phloem cellulose from velvet ash. Future studies should develop specific cellulase inhibitors targeting ALG19’s enzymatic machinery, which could disrupt larval nutrient acquisition by blocking symbiotic cellulose digestion in the gut ecosystem.
The raw sequence data were deposited in the National Center for Biotechnology Information (NCBI) Sequence-Read Archive (SRA) database under accession number PRJNA1205903.
Y-HW: Visualization, Writing – original draft. QL: Investigation, Writing – original draft. TV: Writing – review & editing. H-LW: Investigation, Writing – review & editing. J-YF: Writing – original draft. X-YS: Investigation, Writing – review & editing. Y-JW: Writing – original draft. J-YY: Writing – original draft. J-YZ: Conceptualization, Funding acquisition, Software, Supervision, Visualization, Writing – original draft, Writing – review & editing. H-PL: Funding acquisition, Supervision, Writing – review & editing.
The author(s) declare that financial support was received for the research and/or publication of this article. This study was supported by the National Science Foundation: Dimensions Collaborative Research Project (No. 32061123002), Research Special Funds for Introduced Talents at Hebei Agricultural University (No. YJ2021027), and Research Project on Basic Scientific Research Fund for Provincial Universities in Hebei Province (No. KY2022051) in China.
The authors declare that the research was conducted in the absence of any commercial or financial relationships that could be construed as a potential conflict of interest.
The authors declare that no Gen AI was used in the creation of this manuscript.
All claims expressed in this article are solely those of the authors and do not necessarily represent those of their affiliated organizations, or those of the publisher, the editors and the reviewers. Any product that may be evaluated in this article, or claim that may be made by its manufacturer, is not guaranteed or endorsed by the publisher.
Ali, H. R., Hemeda, N. F., and Abdelaliem, Y. F. (2019). Symbiotic cellulolytic bacteria from the gut of the subterranean termite Psammotermes hypostoma Desneux and their role in cellulose digestion. AMB Express 9, 1–9. doi: 10.1186/s13568-019-0830-5
Bauer, L. S., Duan, J. J., Gould, J. R., and Van Driesche, R. (2015). Progress in the classical biological control of Agrilus planipennis Fairmaire (Coleoptera: Buprestidae) in North America. Can. Entomol. 147, 300–317. doi: 10.4039/tce.2015.18
Boore, J. L. (2006). The use of genome-level characters for phylogenetic reconstruction. Trends Ecol. Evol. 21, 439–446. doi: 10.1016/j.tree.2006.05.009
Bureau Beijing Gardening and Greening (2017). Implementation plan for Agrilus planipennis management in Beijing. Available online at: https://yllhj.beijing.gov.cn/zwgk/2024nzcwj/2024nqtwj/202406/t20240624_3726886.shtml (Accessed March 27, 2017).
Cappaert, D., Mccullough, D. G., Poland, T. M., and Siegert, N. W. (2005). Emerald ash borer in North America: a research and regulatory challenge. Am. Entomol. 51, 152–165. doi: 10.1093/ae/51.3.152
Chen, X., Liang, X., Shi, N., He, L., Ma, Y., Zhu, D., et al. (2024). New wheat straw fermentation feed: recombinant Schizosaccharomyces pombe efficient degradation of lignocellulose and increase feed protein. Prep. Biochem. Biotech. 55, 36–44. doi: 10.1080/10826068.2024.2353637
Emms, D. M., and Kelly, S. (2019). Ortho finder: phylogenetic orthology inference for comparative genomics. Genome Biol. 20:238. doi: 10.1186/s13059-019-1832-y
Fu, S. Y. (2019). Progress in cellulose research. China Pulp Paper 38, 54–64. (in Chinese). doi: 10.11980/j.issn.0254-508X.2019.06.009
Han, J., Sun, L., Dong, X., Cai, Z., Sun, X., Yang, H., et al. (2005). Characterization of a novel plant growth-promoting bacteria strain Delftia tsuruhatensis HR4 both as a diazotroph and a potential biocontrol agent against various plant pathogens. Syst. Appl. Microbiol. 28, 66–76. doi: 10.1016/j.syapm.2004.09.003
Holtof, M., Lenaerts, C., Cullen, D.Vanden, and Broeck, J. (2019). Extracellular nutrient digestion and absorption in the insect gut. Cell Tissue Res. 377, 397–414. doi: 10.1007/s00441-019-03031-9
Hyatt, D., Chen, G., Locascio, P. F., Land, M. L., Larimer, F. W., and Hauser, L. J. (2010). Prodigal: prokaryotic gene recognition and translation initiation site identification. BMC Bioinformatics 11, 1–11. doi: 10.1186/1471-2105-11-119
Jeong, S., Ryu, J., Yang, Q., Zhu, J. Y., and Yoo, C. G. (2024). Recent advances in hydrotropic solvent systems for lignocellulosic biomass utilization. Green Chem. 26, 1806–1830. doi: 10.1039/D3GC03309K
Jing, T., Qi, F., and Wang, Z. (2020). Most dominant roles of insect gut bacteria: digestion, detoxification, or essential nutrient provision? Microbiome 8, 1–20. doi: 10.1186/s40168-020-00823-y
Kastner, P. D., Noell, S. E., and Essig, D. A. (2024). Complete genome sequence of a winter season Vibrio facilitates discovery of a novel subclade of cold-adapted species in the albus clade. Microb. Genomics 10:001178. doi: 10.1099/mgen.0.001178
Li, C., Han, G., Sun, J., Huang, L., Lu, Y., Xia, Y., et al. (2022). The gut microbiota composition of Cnaphalocrocis medinalis and their predicted contribution to larval nutrition. Front. Microbiol. 13:909863. doi: 10.3389/fmicb.2022.909863
Li, Y., Kuramae, E. E., Nasir, F., Wang, E., Zhang, Z., Li, J., et al. (2023). Addition of cellulose degrading bacterial agents promoting keystone fungal-mediated cellulose degradation during aerobic composting: construction the complex co-degradation system. Bioresour. Technol. 381:129132. doi: 10.1016/j.biortech.2023.129132
Li, J., Li, M., and Ni, J. F. (2021). Isolation and cultivation of lignocellulolytic bacteria from termite gut. Micobiome Protocol eBook 101:e2003676. (in Chinese). doi: 10.21769/BioProtoc.2003676
Li, J., Wang, S., Zhao, J., Dong, Z., and Shao, T. (2022). Gut microbiota of Ostrinia nubilalis larvae degrade maize cellulose. Front. Microbiol. 13:816954. doi: 10.3389/fmicb.2022.816954
Liu, Q., Vuong, T. M. D., Wang, X., Wang, H., Zeng, J., and Li, H. (2024). Bibliometric analysis in the field of cellulose-degrading bacteria in insect gut. Microbiol. China 51, 4754–4767. (in Chinese). doi: 10.13344/j.microbiol.china.240231
Manni, M., Berkeley, M. R., Seppey, M., Simão, F. A., and Zdobnov, E. M. (2021). BUSCO update: novel and streamlined workflows along with broader and deeper phylogenetic coverage for scoring of eukaryotic, prokaryotic, and viral genomes. Mol. Biol. Evol. 38, 4647–4654. doi: 10.1093/molbev/msab199
Mogouong, J., Constant, P., Lavallée, R., and Guertin, C. (2020). Gut microbiome of the emerald ash borer, Agrilus planipennis Fairmaire, and its relationship with insect population density. FEMS Microbiol. Ecol. 96:fiaa141. doi: 10.1093/femsec/fiaa141
Muhammad, A., Fang, Y., Hou, Y., and Shi, Z. (2017). The gut entomotype of red palm weevil Rhynchophorus ferrugineus Olivier (Coleoptera: Dryophthoridae) and their effect on host nutrition metabolism. Front. Microbiol. 8:2291. doi: 10.3389/fmicb.2017.02291
Nisbet, D., Kreutzweiser, D., Sibley, P., and Scarr, T. (2015). Ecological risks posed by emerald ash borer to riparian forest habitats: a review and problem formulation with management implications. Forest Ecol. Manag. 358, 165–173. doi: 10.1016/j.foreco.2015.08.030
Pan, X., Wang, X., and Zhang, F. (2020). New insights into cockroach control: using functional diversity of Blattella germanica symbionts. Insects 11:696. doi: 10.3390/insects11100696
Pierre, J. F., Peters, B. M., La Torre, D., Sidebottom, A. M., Tao, Y., Zhu, X., et al. (2023). Peptide YY: a Paneth cell antimicrobial peptide that maintains Candida gut commensalism. Science 381, 502–508. doi: 10.1126/science.abq3178
Ren, Y., Yu, G., Shi, C., Liu, L., Guo, Q., Han, C., et al. (2022). Majorbio cloud: a one-stop, comprehensive bioinformatic platform for multiomics analyses. Imeta 1:e12. doi: 10.1002/imt2.12
Saha, S., Khatun, F., Nahiduzzaman, M., Mahmud, M. P., Rahman, M. M., and Yasmin, S. (2022). A greener way of lactic acid production from cellulose-rich waste materials utilising cellulolytic bacteria population from insect gut. Bioresource Technol. Rep. 18:101021. doi: 10.1016/j.biteb.2022.101021
Sawant, N. B., Tuwar, A., and Salve, M. T. (2023). The brief review on pharmaceutical polymer. Int. J. Humanit. Soc. Sci. Manag. 6, 143–153.
Siddiqui, R., and Khan, N. A. (2023). Is the gut microbiome of insects a potential source to meet UN sustainable development goals to eliminate plastic pollution? Environ. Microbiol. Rep. 15, 455–458. doi: 10.1111/1758-2229.13166
Su, R., Pan, B., Luo, Y., Zheng, X., Lu, W., and Wang, X. (2024). Characterization of bacterial diversity and screening of cellulose-degrading bacteria in the gut system of Glenea cantor (Fabricius) larvae. Front. Bioeng. Biotech. 12:1340168. doi: 10.3389/fbioe.2024.1340168
Sun, J., Koski, T., Wickham, J. D., Baranchikov, Y. N., and Bushley, K. E. (2024). Emerald ash borer management and research: decades of damage and still expanding. Annu. Rev. Entomol. 69, 239–258. doi: 10.1146/annurev-ento-012323-032231
Vasanthakumar, A., Handelsman, J. O., Schloss, P. D., Bauer, L. S., and Raffa, K. F. (2008). Gut microbiota of an invasive subcortical beetle, Agrilus planipennis Fairmaire, across various life stages. Environ. Entomol. 37, 1344–1353. doi: 10.1603/0046-225X(2008)37[1344:GMOAIS]2.0.CO;2
Vecherskii, M. V., Orlova-Bienkowskaja, M. J., Kuznetsova, T. A., and Bieńkowski, A. O. (2022). Discovery of Rickettsia and Rickettsiella intracellular bacteria in emerald ash borer Agrilus planipennis by metagenomic study of larval gut microbiome in European Russia. Forests 13:974. doi: 10.3390/f13070974
Wei, X., Reardon, D., Wu, Y., and Sun, J. (2004). Emerald ash borer, Agrilus planipennis Fairmaire (Coleoptera: Buprestidae), in China: a review and distribution survey. Acta Entomol. Sin. 47, 679–685. doi: 10.16380/j.kcxb.2004.05.021
Wen, A., Fegan, M., Hayward, C., Chakraborty, S., and Sly, L. I. (1999). Phylogenetic relationships among members of the Comamonadaceae, and description of Delftia acidovorans (den Dooren de Jong 1926 and) gen. Nov., comb. nov. Int. J. Syst. Evol. Micr. 49, 567–576. doi: 10.1099/00207713-49-2-567
Wen, X., Peng, S. Y., Jiang, W. W., Yang, Q., and Luo, Q. (2017). Comparative study on the method of extracting bagasse cellulose. New Chem. Mater. 45, 250–252.
Yuan, C. J., Liu, M., Du, Y., and Chen, Y. (2023). Comparison of the effect of pre-ozonation on the treatment of aniline wastewater by activated sludge process. Int. J. Environ. Sci. Technol. 20, 12167–12178. doi: 10.1007/s13762-023-04770-7
Keywords: Agrilus planipennis , gut bacteria, Delftia tsuruhatensis , cellulose degradation, Fraxinus velutina
Citation: Wang Y-H, Liu Q, Vuong TMD, Wang H-L, Fu J-Y, Su X-Y, Wang Y-J, Yang J-Y, Zeng J-Y and Li H-P (2025) Gut bacterium Delftia tsuruhatensis strain ALG19 isolated from Agrilus planipennis larvae degrades cellulose in Fraxinus velutina. Front. Microbiol. 16:1567054. doi: 10.3389/fmicb.2025.1567054
Received: 26 January 2025; Accepted: 06 March 2025;
Published: 01 April 2025.
Edited by:
Ranjith Kumavath, Pondicherry University, IndiaReviewed by:
Abdul Basit, University of Jhang, PakistanCopyright © 2025 Wang, Liu, Vuong, Wang, Fu, Su, Wang, Yang, Zeng and Li. This is an open-access article distributed under the terms of the Creative Commons Attribution License (CC BY). The use, distribution or reproduction in other forums is permitted, provided the original author(s) and the copyright owner(s) are credited and that the original publication in this journal is cited, in accordance with accepted academic practice. No use, distribution or reproduction is permitted which does not comply with these terms.
*Correspondence: Jian-Yong Zeng, emVuZ3N0dWR5d29ya0AxNjMuY29t; Hui-Ping Li, aHVpcGluZ2xpcGFwZXJAMTYzLmNvbQ==
†ORCID: Zeng Jian-Yong, https://orcid.org/0000-0001-9419-0674
Li Hui-Ping, https://orcid.org/0000-0001-5300-3537
Disclaimer: All claims expressed in this article are solely those of the authors and do not necessarily represent those of their affiliated organizations, or those of the publisher, the editors and the reviewers. Any product that may be evaluated in this article or claim that may be made by its manufacturer is not guaranteed or endorsed by the publisher.
Research integrity at Frontiers
Learn more about the work of our research integrity team to safeguard the quality of each article we publish.