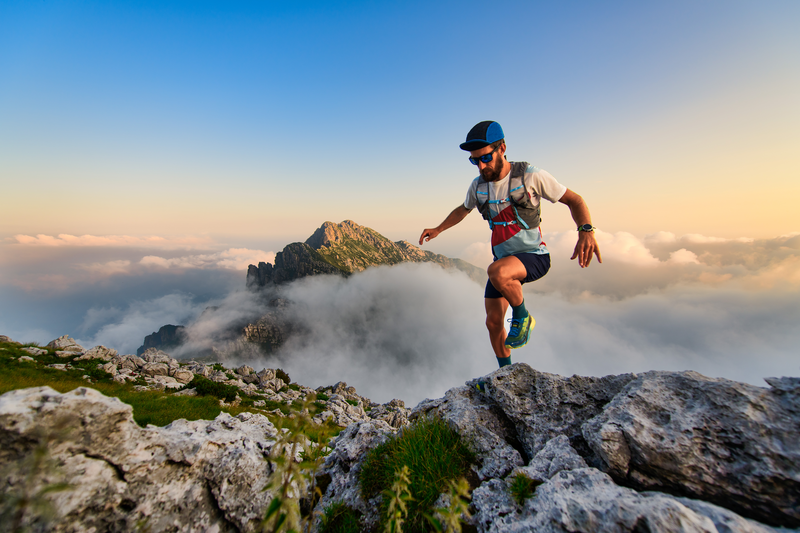
94% of researchers rate our articles as excellent or good
Learn more about the work of our research integrity team to safeguard the quality of each article we publish.
Find out more
ORIGINAL RESEARCH article
Front. Microbiol.
Sec. Microbiotechnology
Volume 16 - 2025 | doi: 10.3389/fmicb.2025.1565907
The final, formatted version of the article will be published soon.
You have multiple emails registered with Frontiers:
Please enter your email address:
If you already have an account, please login
You don't have a Frontiers account ? You can register here
Selenium is a vital trace mineral that regulates essential physiological functions, and the development of sustainable methods for synthesizing selenium nanoparticles (SeNPs) is an active area of research. This study reported the mycosynthesis of SeNPs using the aqueous extract of the edible mushroom Pleurotus ostreatus. The synthesized SeNPs were characterized using various analytical techniques, including UV-visible spectroscopy, dynamic light scattering, transmission electron microscopy (TEM), and Fourier-transform infrared spectroscopy (FTIR). The results revealed that the SeNPs exhibited spherical morphology with a diameter range of 72-148 nm, moderate stability with a zeta potential of -10.5 mV, and a polydispersity index of 0.27. The myco-synthesized SeNPs demonstrated potent antioxidant activity with a DPPH radical scavenging IC50 value of 662.1 ± 1.05 µg/mL, comparable to the standard antioxidant Trolox (IC50 = 24.42 ± 0.87 µM). Furthermore, SeNPs exhibited considerable antimicrobial efficacy against Staphylococcus aureus (17±0.02 mm inhibition zone), followed by Escherichia coli (16±1.04 mm) and Candida albicans (12±0.3 mm). Additionally, SeNPs displayed moderate antiviral activity against a low-pathogenic coronavirus (229E) strain, with a selectivity index (SI) of 5. In agriculture, the application of SeNPs at 10 µM significantly increased primary metabolite production in wheat (Triticum aestivum), with total soluble sugars reaching 54.32 mg/g and soluble proteins increasing to 139.66 mg/g, promoting both shoot and root growth. The comprehensive characterization and evaluation of SeNPs provide valuable insights into their multifunctionality, paving the way for further exploration in medicine, agriculture, and environmental applications.
Keywords: Selenium nanoparticles, Mycosynthesis, Pleurotus ostreatus, antioxidant, antimicrobial, antiviral, Triticum vulgarisaestivum, molecular docking
Received: 23 Jan 2025; Accepted: 26 Feb 2025.
Copyright: © 2025 Sonbol, Zakaria, Mohamed, Abdelmonem and El-Sayed. This is an open-access article distributed under the terms of the Creative Commons Attribution License (CC BY). The use, distribution or reproduction in other forums is permitted, provided the original author(s) or licensor are credited and that the original publication in this journal is cited, in accordance with accepted academic practice. No use, distribution or reproduction is permitted which does not comply with these terms.
* Correspondence:
Heba El-Sayed, Helwan University, Helwan, Egypt
Disclaimer: All claims expressed in this article are solely those of the authors and do not necessarily represent those of their affiliated organizations, or those of the publisher, the editors and the reviewers. Any product that may be evaluated in this article or claim that may be made by its manufacturer is not guaranteed or endorsed by the publisher.
Research integrity at Frontiers
Learn more about the work of our research integrity team to safeguard the quality of each article we publish.