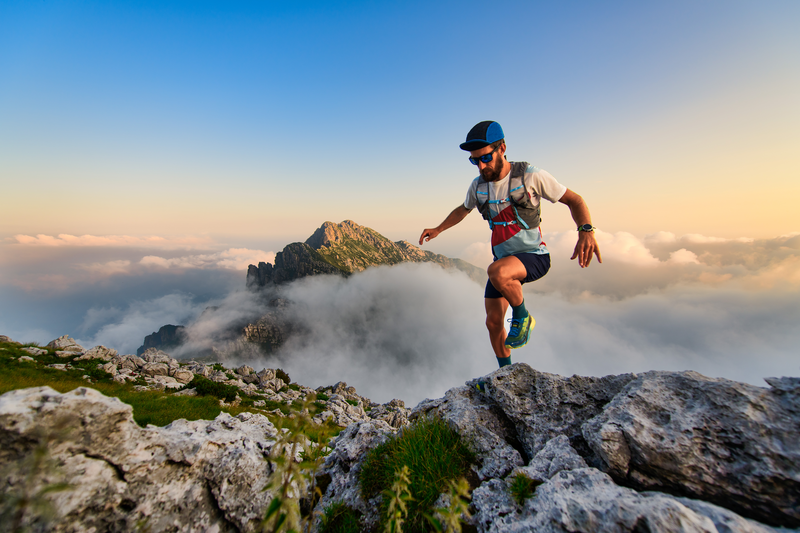
94% of researchers rate our articles as excellent or good
Learn more about the work of our research integrity team to safeguard the quality of each article we publish.
Find out more
ORIGINAL RESEARCH article
Front. Microbiol.
Sec. Microorganisms in Vertebrate Digestive Systems
Volume 16 - 2025 | doi: 10.3389/fmicb.2025.1557778
This article is part of the Research Topic The Interaction Between Food Ingredients and Gut Microbiome on Health and Disease View all 9 articles
The final, formatted version of the article will be published soon.
You have multiple emails registered with Frontiers:
Please enter your email address:
If you already have an account, please login
You don't have a Frontiers account ? You can register here
Ulcerative colitis (UC) is a global disease for which there is little of effective treatment options, thus creating an urgent need for the development of new drug candidates from natural and functional foods. Amomum tsaoko Crevost et Lemarie is a kind of medicinal and food herb that is rich in flavonoids. However, the pharmacological effects of Amomum tsaoko flavonoids (ATF) on UC have not yet been reported. In the present study, the intervention effects and anti-colitis mechanisms of ATF in the DSS-induced UC mice were explored. The results demonstrated that a high dose of ATF (100 mg/kg) led to a significant restoration of body weight, disease activity index score, and colon length, in addition to ameliorating colonic tissue damage in UC mice. ATF reduced the serum levels of LPS, inhibited the activation of the colonic TLR4/NF-κB/NLRP3 signaling pathway, and increased the mRNA expression of tight junction proteins such as ZO-1, Occludin, and Claudin4.Furthermore, ATF was found to reduce the relative abundance of the DSS-induced conditional pathogenic (Escherichia-Shigella, Colidextribacter, and Oscillibacter), increase the potential probiotic taxa (Akkermansia, Bifidobacterium and unclassified_f__Atopobiaceae). Interestingly, these genera were found to be significantly correlated with the UC core phenotypic indicators. These findings indicated that ATF may alleviate UC symptoms by modulating the gut microbiota-LPS/TLR4/NF-κB/NLRP3 axis. The present study has the potential to serve as a valuable reference point for understanding the role of natural flavonoids in the prevention of inflammatory diseases, and to expand the future applications of ATF in the fields of food and medicine.
Keywords: Amomum tsaoko, Flavonoids, ulcerative colitis, Escherichia-Shigella, Akkermansia, Bifidobacterium, Colidextribacter, Oscillibacter
Received: 09 Jan 2025; Accepted: 25 Feb 2025.
Copyright: © 2025 Huang, Zhao, Yang, Duan, Sheng, Tian, Peng and Gao. This is an open-access article distributed under the terms of the Creative Commons Attribution License (CC BY). The use, distribution or reproduction in other forums is permitted, provided the original author(s) or licensor are credited and that the original publication in this journal is cited, in accordance with accepted academic practice. No use, distribution or reproduction is permitted which does not comply with these terms.
* Correspondence:
Xiaoyu Gao, Yunnan Agricultural University, Kunming, 650201, Yunnan, China
Disclaimer: All claims expressed in this article are solely those of the authors and do not necessarily represent those of their affiliated organizations, or those of the publisher, the editors and the reviewers. Any product that may be evaluated in this article or claim that may be made by its manufacturer is not guaranteed or endorsed by the publisher.
Research integrity at Frontiers
Learn more about the work of our research integrity team to safeguard the quality of each article we publish.