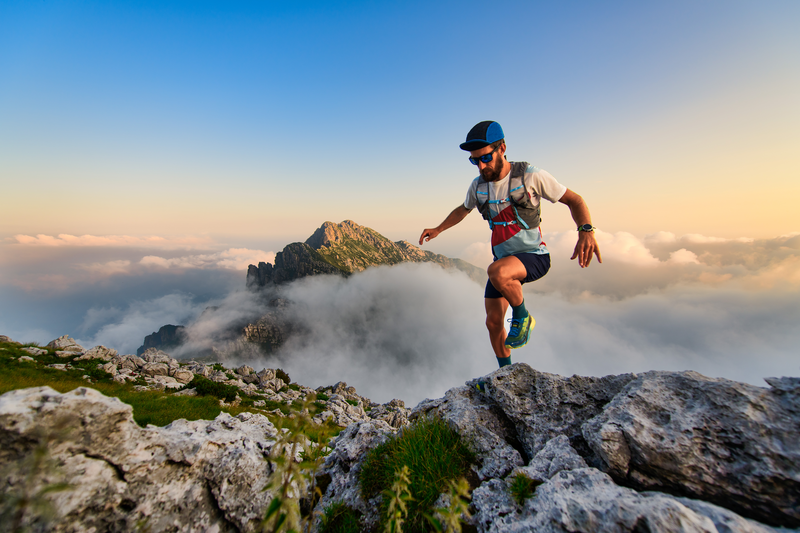
94% of researchers rate our articles as excellent or good
Learn more about the work of our research integrity team to safeguard the quality of each article we publish.
Find out more
REVIEW article
Front. Microbiol.
Sec. Microorganisms in Vertebrate Digestive Systems
Volume 16 - 2025 | doi: 10.3389/fmicb.2025.1556827
This article is part of the Research Topic Fecal Microbiota Transplants: challenges in translating microbiome research to clinical applications View all 18 articles
The final, formatted version of the article will be published soon.
You have multiple emails registered with Frontiers:
Please enter your email address:
If you already have an account, please login
You don't have a Frontiers account ? You can register here
Fecal microbiota transplantation (FMT) represents a therapeutic approach that directly regulates the gut microbiota of recipients, normalizes its composition and reaping therapeutic rewards.Currently, in addition to its general application in treating Clostridium difficile (C. difficile) infection (CDI), FMT treatment has also been extended to the fields of other gastrointestinal diseases, infections, gut-liver or gut-brain axis disorders, metabolic diseases and cancer, etc. Prior to FMT, rigorous donor screening is essential to reduce the occurrence of adverse events. In addition, it is imperative to evaluate whether the recipient can safely and effectively undergo FMT treatment. However, the efficacy of FMT is influenced by the complex interactions between the gut microbiota of donor and recipient, the degree of donor microbiota engraftment is not necessarily positively related with the success rate of FMT. Furthermore, an increasing number of novel factors affecting FMT outcomes are being identified in recent clinical trials and animal experiments, broadening our understanding of FMT treatment. This article provides a comprehensive review of the application scenarios of FMT, the factors influencing the safety and efficacy of FMT from the aspects of both the donors and the recipients, and summarizes how these emerging novel regulatory factors can be combined to predict the clinical outcomes of patients undergoing FMT.
Keywords: Gut Microbiota, fecal microbiota transplantation, donor-recipient interplay, Prediction model, Safety and efficiency
Received: 07 Jan 2025; Accepted: 07 Mar 2025.
Copyright: © 2025 Liu, Li, Chen, Yao, Zhou, Wang, Meng, Ji, Yu and Chen. This is an open-access article distributed under the terms of the Creative Commons Attribution License (CC BY). The use, distribution or reproduction in other forums is permitted, provided the original author(s) or licensor are credited and that the original publication in this journal is cited, in accordance with accepted academic practice. No use, distribution or reproduction is permitted which does not comply with these terms.
* Correspondence:
Zihan Yu, Tianjin Medical University General Hospital, Tianjin, China
Xin Chen, Tianjin Medical University General Hospital, Tianjin, China
Disclaimer: All claims expressed in this article are solely those of the authors and do not necessarily represent those of their affiliated organizations, or those of the publisher, the editors and the reviewers. Any product that may be evaluated in this article or claim that may be made by its manufacturer is not guaranteed or endorsed by the publisher.
Research integrity at Frontiers
Learn more about the work of our research integrity team to safeguard the quality of each article we publish.