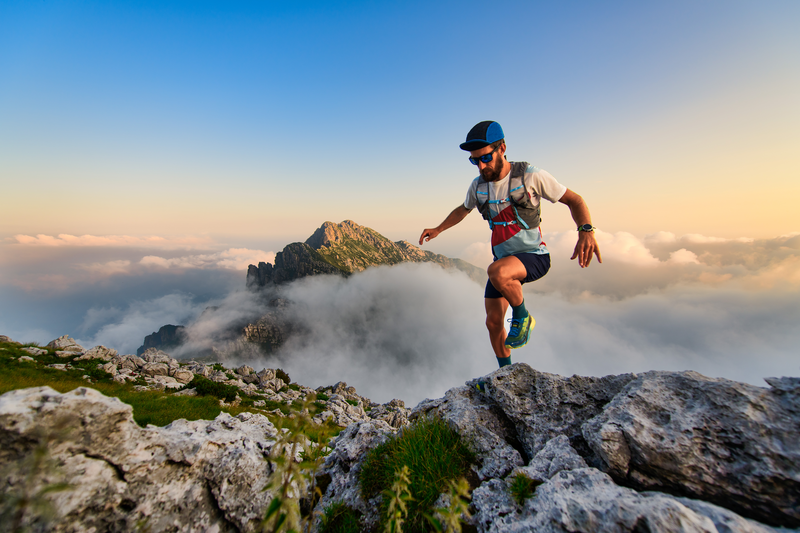
94% of researchers rate our articles as excellent or good
Learn more about the work of our research integrity team to safeguard the quality of each article we publish.
Find out more
ORIGINAL RESEARCH article
Front. Microbiol.
Sec. Microbiotechnology
Volume 16 - 2025 | doi: 10.3389/fmicb.2025.1555989
This article is part of the Research Topic Recent Advances in Agricultural Waste Recycling by Microorganisms and Their Symbiosis View all 9 articles
The final, formatted version of the article will be published soon.
You have multiple emails registered with Frontiers:
Please enter your email address:
If you already have an account, please login
You don't have a Frontiers account ? You can register here
Water scarcity is an escalating environmental concern. The model diatom, Phaeodactylum tricornutum, holds promise as a potential cell factory for the production of high-value natural compounds. However, its dependence on saline water cultivation restricts its use in areas facing water shortages. Although numerous studies have delved into the metabolic mechanisms of plants under water stress, there is a limited understanding when it comes to microalgae. In our study, we employed polyethylene glycol (PEG) to simulate water scarcity conditions, and assessed a range of parameters to elucidate the metabolic responses of P. tricornutum. Water stress induced the generation of reactive oxygen species (ROS), curtailed the photosynthetic growth rate, and amplified lipid content. Our insights shed light on the physiology of P. tricornutum when subjected to water stress, setting the stage for potential applications of microalgae biotechnology in regions grappling with water scarcity.
Keywords: polyethylene glycol, Water stress, Lipid, phaeodactylum tricornutum, Microalgae
Received: 06 Jan 2025; Accepted: 28 Feb 2025.
Copyright: © 2025 Hao, Lai, Shu, Liang, Chen, Huang, Lu and Alimujiang. This is an open-access article distributed under the terms of the Creative Commons Attribution License (CC BY). The use, distribution or reproduction in other forums is permitted, provided the original author(s) or licensor are credited and that the original publication in this journal is cited, in accordance with accepted academic practice. No use, distribution or reproduction is permitted which does not comply with these terms.
* Correspondence:
Yang Lu, Jinan University, Guangzhou, China
Adili Alimujiang, Jinan University, Guangzhou, China
Disclaimer: All claims expressed in this article are solely those of the authors and do not necessarily represent those of their affiliated organizations, or those of the publisher, the editors and the reviewers. Any product that may be evaluated in this article or claim that may be made by its manufacturer is not guaranteed or endorsed by the publisher.
Research integrity at Frontiers
Learn more about the work of our research integrity team to safeguard the quality of each article we publish.