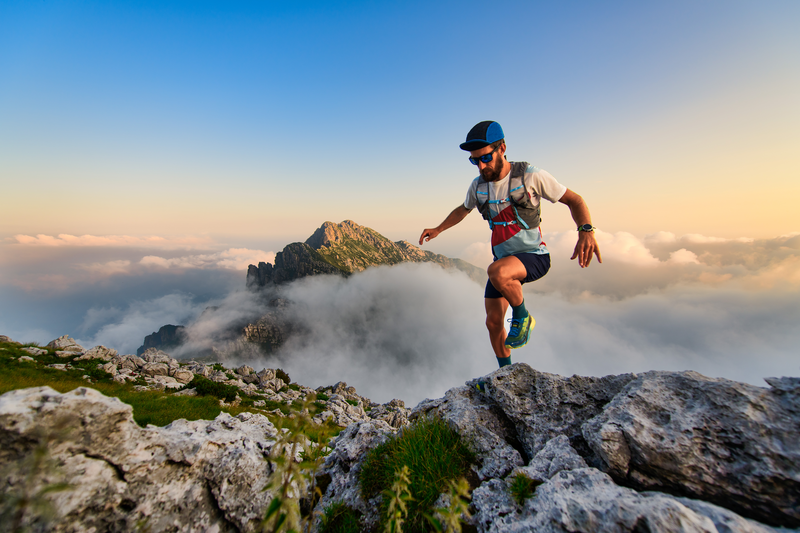
95% of researchers rate our articles as excellent or good
Learn more about the work of our research integrity team to safeguard the quality of each article we publish.
Find out more
ORIGINAL RESEARCH article
Front. Microbiol. , 18 March 2025
Sec. Microorganisms in Vertebrate Digestive Systems
Volume 16 - 2025 | https://doi.org/10.3389/fmicb.2025.1553404
Introduction: This study aimed to compare oral microbiome profiles between obese and lean individuals without clinical periodontitis, and to assess changes in the oral microbiome of obese subjects following bariatric surgery.
Methods: Individuals with a body mass index (BMI) > 30 were enrolled in the obese group, whereas those with a BMI < 23 served as controls. The obese surgery group, which consented to bariatric surgery, was followed up at 1, 3, and 6 months with clinical examinations. Oral examinations were conducted and periodontal disease was classified based on probing results. Saliva, buccal and subgingival microbiome samples were analyzed for community diversity, relative bacterial abundance, and differential abundance between control (n = 24) and obese group (n = 31). To evaluate effect size and statistical power, we used micropower, a simulation-based method for Permutational Multivariate Analysis of Variance-based β-diversity comparisons.
Results: The obese group exhibited distinct alpha diversity (buccal: Chao1 p = 0.0002, Shannon p = 0.0003, supragingival: Shannon p < 0.0001) compared with the control group. Bray-Curtis distance analysis indicated significant disparities in microbiome composition distribution in saliva (p = 0.003), buccal (p = 0.002), and subgingival plaque samples (p = 0.001). Although the obese and normal weight groups exhibited no significant periodontal differences, the obese group showed distinct species associated with periodontal disease, especially in subgingival plaque including Filifactor alocis, Peptostreptococcaceae spp., Prevotella spp., and Treponema maltophilum. Cluster analysis of the obese surgery group indicated the emergence of microbiomes associated with a healthy state that increased over time including Streptococcus salivarious and various Veillonella spp., whereas clusters containing periodontal pathogens including Porphyromonas spp., tended to diminish.
Discussion: The oral microbiome at 6 months post-bariatric surgery indicates a potential shift toward a healthy periodontal state, suggesting that weight loss interventions may positively impact oral microbial communities even in the absence of clinical periodontitis.
In recent decades, the global prevalence of obesity has reached alarming levels and it has emerged as a significant public health concern. Obesity not only poses a substantial burden on an individual’s physical and psychological well-being but is also a pivotal risk factor for the development of various metabolic disorders, including type 2 diabetes mellitus, cardiovascular diseases, and certain cancers (Hruby and Hu, 2015; Arnold et al., 2015; Lauby-Secretan et al., 2016; GBD 2015 Obesity Collaborators et al., 2017).
Mounting evidence suggests that the gut microbiome plays a key role in the development and progression of obesity (Ridaura et al., 2013). The gut microbiota actively participates in energy regulation, nutrient metabolism, and the modulation of inflammation, thereby influencing host physiology and adiposity (Turnbaugh et al., 2006; Shen et al., 2013). The oral microbiome is the second largest microbiome community after the gut (NIH HMP Working Group et al., 2009) and has been well studied in relation to systemic diseases; however, it is comparatively less studied than the gut microbiome in relation to obesity. Recently, the oral microbiome has been shown to be associated with weight gain (Yang et al., 2019), and influence metabolic diseases, including obesity, through various mechanisms related to low-grade inflammation (Goodson et al., 2009; Gasmi Benahmed et al., 2021; Ganesan et al., 2021). Additionally, the oral microbiome, particularly species known as the “red complex,” has been strongly linked to periodontitis (Chen et al., 2018; Manzoor et al., 2024). Several studies have suggested a complex interplay between the oral microbiome, obesity, and periodontal health (reviewed by Reytor-González et al., 2024). Obesity has been associated with alterations in the oral microbiome composition, potentially predisposing individuals to periodontal disease. However, the exact mechanisms linking obesity, oral microbial dysbiosis, and periodontal health remain unclear, particularly in individuals without clinical periodontitis.
Bariatric surgery is an effective and durable method for weight loss in patients with severe obesity (Maciejewski et al., 2016; Schauer et al., 2017). Several studies have documented dynamic shifts in the gut microbiome (Furet et al., 2010; Liou et al., 2013) but few have investigated oral microbial diversity and abundance following bariatric surgery (Džunková et al., 2020; Stefura et al., 2022). There is insufficient research on whether surgical improvement of obesity in patients can lead to changes in the oral microbiome.
This study aimed to investigate significant differences in the oral microbiota between individuals of normal weight and those with obesity without clinically significant differences in periodontitis. Furthermore, we examined changes in the oral microbiota following surgical intervention.
From September 2021 to March 2023, patients with a body mass index (BMI) > 30 were recruited as the test group (obese group), and those with a BMI < 23 were recruited as the control group. Patients in the obese group who consented to undergo bariatric surgery were referred to as the obese surgery (OS) group and were followed-up at 1, 3, and 6 months to collect clinical examination results, blood test results, and saliva and buccal swab samples (Figure 1). All participants provided written informed consent, and the study protocol was approved by the Institutional Review Board of Seoul National University Bundang Hospital (IRB No.: B-2001-586-303).
Subgingival plaque, saliva, and buccal swabs were collected from all the participants at the Department of Periodontology, Section of Dentistry, Seoul National University Bundang Hospital, Korea. Buccal swab samples were obtained from the mucosa of both cheeks, using a sterile swab kit (Kim et al., 2023). The cotton swab portion of the kit was immersed in a conical tube containing sterile distilled water for more than 20 s, and the tube was then sealed. Subgingival plaque samples were collected from the two deepest periodontal pocket sites of each participant’s teeth using a curette. The collected plaque was transferred onto the periopaper and sealed in a sterile conical tube for storage. Saliva was collected for 20 min without stimulation. Participants were requested to fast for 2 h and refrain from oral hygiene (brushing or flossing teeth) for 2 h before sampling. Samples from all subjects were collected and stored at −80°C for subsequent processing.
Oral examinations were performed by a professional periodontist. Participants were screened for other oral diseases such as dental caries and mucosal lesions. Those with active oral diseases other than periodontitis were excluded from the study. Panoramic radiographs were obtained and the number of missing teeth was counted. To classify periodontitis (PD), probing results were recorded at six sites for each tooth (Matuliene et al., 2008). Periodontal disease was classified according to the criteria of the American Academy of Periodontology/Centers for Disease Control and Prevention (Eke et al., 2012) and further categorized into the non-PD and PD groups based on the presence of at least one site with a probing pocket depth of ≥5 mm (McCracken et al., 2017). Patients were excluded if they had used steroids, immunosuppressants, rheumatic medications, or antibiotics in the past month.
All patients in the OS group underwent laparoscopic sleeve gastrectomy. Sleeve gastrectomy was performed by inserting four trocars into the abdominal cavity, followed by dissection of the greater curvature and fundus of the stomach using a laparoscopic bipolar device. Subsequently, the stomach was resected using a laparoscopic surgical stapler, starting 4 cm from the pylorus, and a 36Fr bougie was used for gastric calibration.
Total DNA was extracted from the buccal and subgingival plaque using a Gram-positive DNA purification kit (Lucigen, Biosearch Technology, Novato, CA). For each saliva sample, 200 μL was centrifuged, and the resulting pellet was used for DNA extraction with a DNA extraction kit. Each sequenced sample was prepared according to the Illumina 16S Metagenomic Sequencing Library protocols to amplify the V1 and V2 region (27F-338R) (Na et al., 2023). DNA quality was measured using PicoGreen and NanoDrop ND-1000 spectrophotometer (Thermo Fisher Scientific, United States) and stored at −80°C until use. The purified amplicons were combined in equimolar amounts and subjected to paired-end sequencing using NovaSeq (Illumina, San Diego, CA, United States).
Demographic characteristics were analyzed using t-tests, chi-square tests, and Fisher’s exact tests. The Wilcoxon rank-sum test was used to compare the clinical parameters of patients undergoing bariatric surgery. Continuous variables are expressed as medians with interquartile ranges, and categorical variables are presented as counts or percentages. Statistical significance was set at p < 0.05. Statistical analyses were performed using R ver. 4.1.2 (R Foundation for Statistical Computing, Vienna, Austria).
Basic microbiome analyses were performed using QIIME2 (version 2020.6) (Hall and Beiko, 2018) and the associated plugins. Choa1 and Shannon’s indices were used to measure alpha diversity. Principal coordinate analysis of the Bray–Curtis distance was performed to determine the community structure using the vegan package v2.3-0 in R software v3.2.1. The Kruskal–Wallis test and non-parametric permutation multivariate analysis of variance were used to assess the statistical significance of alpha and beta diversities, respectively. The species of each Operational Taxonomic Unit (OTU) was determined by a pre-trained Naive Bayes classifier, using the Human Oral Microbiome Database 16S rRNA Extended RefSeq sequences database (version 15.1) (Wade, 2013). Differential abundance of specific bacterial species in each group was assessed using the linear discriminant analysis effect size (LEfSe) (Segata et al., 2011), ANOVA-like Differential Expression (ALDEx2) (Fernandes et al., 2014), and Analysis of Compositions of Microbiomes (ANCOM) (Mandal et al., 2015) tools with their default settings. A linear discriminant analysis (LDA) score of 2.5 was used as the cut-off. Species identified as significant by at least two of these methods were considered reliable. To characterize the microbial variation pattern following bariatric treatment, the expression mode clustering was analyzed and visualized using the time course sequencing (“TCseq”) package (version 1.27.0).
We recruited 55 participants, of which 24 were in the control group (BMI <23) and 31 were in the obese group (BMI ≥30). The demographic characteristics of the participants are shown in Table 1. There were significant differences in BMI (p < 0.001), weight (p < 0.001), and smoking history (p = 0.017) between the two groups. Diabetes and hypertension were significantly more prevalent in the obese group than in the control group. There were no significant differences in the degree of periodontitis or tooth loss between the two groups. However, there was a significant difference in the mean probing pocket depth, which was within the normal range in both groups.
The alpha diversity of the microbiota was estimated using the Chao1 and Shannon indices. Buccal swab samples from the obese group had significantly higher Chao1 and Shannon indices. The Shannon index of the plaque samples was also significantly higher in the obese group (Figure 2A). For principal coordinate analysis, the Bray–Curtis distance was used to analyze the distribution of the microbiota. The microbiome compositions of saliva, buccal swabs, and subgingival samples were significantly different between the control and obese groups (Figures 2B–D).
Figure 2. Bacterial community comparison between the obese and control groups. (A) Alpha diversities (Chao1 and Shannon indices) of subgingival plaque, saliva, and buccal samples. Beta diversities of (B) saliva, (C) buccal swab, and (D) plaque samples. ** p < 0.01.
When the relative abundances were analyzed, the five most abundant phyla were Bacillota, Bacteroidota, Actinomycetota, Pseudomonadota, and Fusobacteriota, representing more than 95% of the total taxa in the saliva and buccal swabs. In subgingival plaque samples, these five phyla accounted for most taxa in both groups. Interestingly, in the subgingival plaque samples, there was an increase in the abundance of Fusobacteriota (control vs. obese: 10.39% vs. 14.27%), Candidatus Saccharimonadota (4.45% vs. 6.83%), and Spirochaetota (0.29% vs. 0.80%), with a higher prevalence observed in the obese group (Figure 3A).
Figure 3. Relative abundance of bacterial species in saliva, buccal, and subgingival plaque samples of the obese and control groups. (A) Phylum level, (B) genus level. Cr, control group; Ob, obese group.
The six most abundant genera in the saliva and buccal swab samples were Streptococcus, Prevotella, Hemophilus, Rothia, Porphyromonas, and Neisseria accounting for more than 51% of the total taxa in both groups (Figure 3B). In the subgingival plaque samples, Streptococcus, Capnocytophaga, Leptotrichia, Prevotella, Fusobacterium, Neisseria, and Saccharibacteria (TM7) were the most abundant genera, representing more than 43% of the total taxa.
The average abundance of Neisseria (6.66% vs. 4.43%) was higher in saliva samples from the control group. In the buccal swab samples, the abundance of Porphyromonas (4.63% vs. 6.32%), Capnocytophaga (2.91% vs. 3.73%), and Saccharibacteria (TM7) (0.93% vs. 1.19%) was higher in the obese group. In subgingival plaque samples, the abundance of Prevotella (5.54% vs. 7.27%), Fusobacterium (5.40% vs. 6.39%), Tannerella (1.74% vs. 2.79%), Lepiotrichia (4.99% vs. 7.86%), Saccharibacteria (TM7) (3.27% vs. 4.51%), Porphyromonas (2.37% vs. 3.16%), and Treponema (2.69% vs. 3.82%) was higher in the obese group. The distribution of major phyla and genera in the control and obese groups, separately for saliva, buccal swabs, and subgingival samples, is presented in the Supplementary Figures 1, 2.
LEfSe, ALDEx2, and ANCOM were used to evaluate the differences in bacterial species abundance between the obese and control groups. The number of significant species determined by LEfSe in the saliva, buccal swab, and subgingival plaque samples was 38, 65, and 113, respectively (Figures 4A–C). In the control group, Actinomyces sp. HMT-169, Fusobacterium periodonticum, Haemophilus sp. HMT-908, Neisseria bacilliformis, Streptococcus infantis, Streptococcus sp. HMT-066, and Veillonella rogosae were significant in at least two sampling sites. In the obese group, Prevotella micans, Prevotella oralis and Solobacterium moorei were significant in at least two sampling sites. Additionally, analysis of subgingival plaque samples identified several species that were significantly different between the control and obese groups, including Aggregatibacter spp., Leptotrichia spp., Peptostreptococcaceae spp., Prevotella spp., and Treponema spp. (Figure 4C). Interestingly, T. socranskii, T. denticola, and Filifactor alocis, well-known periodontopathogens, were among the most significant species found in the subgingival plaque samples of the obese group.
Figure 4. Comparisons of the taxa in control and obese group samples showing significant differences depending on sampling sites. (A) saliva, (B) buccal, (C) subgingival plaque. Linear discriminant analysis (LDA) and effect size analysis (LEfSe) was performed for the analysis. LDA score > 2 was plotted.
Overall, 13 patients in the obese group underwent bariatric surgery. Upon clinical evaluations of these 13 individuals, remarkable and statistically significant decreases were observed in the BMI and hemoglobin A1c, aspartate aminotransferase, and alanine aminotransferase levels between baseline and 6-month values. While total cholesterol levels did not show a significant difference, triglyceride and high-density lipoprotein cholesterol levels significantly decreased and increased, respectively (Table 2).
Table 2. Clinical characteristics after bariatric surgery at baseline and 1, 3, and 6-month follow-up.
To evaluate the changes in the microbiome following surgical treatment, saliva and buccal samples were collected for up to 6 months. To simplify the comparison, microbiomes were compared between samples obtained at the initial visit and after 6 months. In saliva samples, Porphyromonas spp., Rothia mucilaginosa, Gemella sanguinis, and Streptococcus sp. HMT-066 were among the most significant species at the initial visit. At 6-month follow-up, Provotella histicolla, Streptococcus salivarious, Megasphaera micronuciformis, and Veillonella atypica were among the most significant taxa found in the saliva (Figure 5A). In buccal swab samples, Streptococcus sp_HMT_066, Streptococcus australis, and Capnocytophaga sp. HMT-332 were among the most significant species at the initial visit. At 6-month follow-up, Prevotella histicola, Leptotrichia sp. HMT-417, Streptococcus salivarius, and Veillonalla atypica were the among the significant species (Figure 5B).
Figure 5. Comparisons of the taxa in samples obtained at baseline and 6-month follow-up from the surgical treatment group, showing significant differences depending on sampling sites. (A) saliva, (B) buccal. Linear discriminant analysis (LDA) and effect size analysis (LEfSe) was performed for the analysis. LDA score > 2 was plotted. Time course sequencing (TCseq) analysis in saliva (C) and swab (D) following surgical treatment.
To further characterize the variation pattern of microbes following surgical treatment, TCseq analysis was performed, and eight clusters were determined as the ideal grouping strategy. Microbes from saliva cluster 1 exhibited an increasing trend. These include Bifidobacterium dentium, K. oralis, K. dentrificans, L. mirabilis, N. bacilliformis, and Streptococcus spp. The microbes in saliva clusters 7 and 8 exhibited a decreasing trend. These included Actinomyces naeslundii, A. odontolyticus, Capnocytophaga leadbetteri, C. gingivalis, C. granulosum, C. sputigena, Eikenella corrodens, and Porphyromonas pasteri (Figure 5C). In cluster 1 from buccal swab samples, K. oralis, K. dentrificans, and various Streptococcus spp. showed an increasing pattern. In clusters 2 and 7 from buccal swab samples, the abundance of F. alocis, Capnocytophaga, Fusobacterium nucleautum subsp. Vincentii, and P. gingivalis showed a decreasing pattern (Figure 5D).
In this study, we compared the oral microbiomes in the saliva, buccal swabs, and subgingival plaque of obese and control groups and analyzed changes in the oral microbiome following bariatric surgery. The obese group was more enriched with microbes known to be periodontopathogens, including Filifactor alocis, Treponema spp., T. socranskii, and T. denticola, at least at two sampling sites, than the control group. Additionally, cluster analysis of the OS group identified clusters of healthy state–related microbiomes that increased over time, and clusters of periopathogen-related microbiomes that tended to decrease.
Studies on the association between the oral microbiome and obesity have shown that the composition of the oral microbiome differs between healthy individuals and those with obesity at different sampling sites (Mathur and Barlow, 2015; Koliada et al., 2017; Tam et al., 2018). Compared with saliva or buccal swab samples, we found that there were a greater number of genera in the subgingival plaque samples that showed differences between the obese and control groups (Figure 3), suggesting that the subgingival plaque microbiome may be more useful in explaining obesity than that of other sites. Additionally, compared to the control group, the obese group showed a higher proportion of Porphyromonas, Capnocytophaga, and Saccharibacteria (TM7) in buccal swab samples, whereas Prevotella, Fusobacterium, Tannerella, Lepiotrichia, Saccharibacteria (TM7), Porphyromonas, and Treponema were more abundant in subgingival plaque samples. Porphyromonas, Fusobacterium, Capnocytophaga, Prevotella, Tannerella, and Treponema are genera commonly associated with periodontopathogens (Socransky et al., 1998; Darby and Curtis, 2001; Mineoka et al., 2008).
The number of species varied significantly, with the highest number found in subgingival plaques, followed by buccal swabs and saliva (Figure 4), suggesting that the environment in the subgingival space differed between the obese and control groups. This implies that the subgingival space may be more affected by systemic conditions than other sampling sites, which may lead to more differences. In the saliva of the obese group, Prevotella micans, Prevotella oralis and Solobacterium moorei were significant in at least two samples. In addition, T. socranskii, T. denticola, and Filifactor alocis were among the most significant species found in the subgingival plaque samples of the obese group, Prevotella oralis, and Prevotella sp. HMT-315 (Nadkarni et al., 2012) are conventional periodontal pathogens. S. moorei and F. alocis are involved in the onset and progression of periodontitis (Hiranmayi et al., 2017). The abundance of Saccharibacteria (TM7) HMT-351 is associated with periodontitis; however, the mechanism is not clear (Baker et al., 2024). In contrast, in the control group, Actinomyces sp. HMT-169, Fusobacterium periodonticum, and Haemophilus sp. HMT-908 were significant, which are commonly associated with periodontal health or gingivitis (Zaura et al., 2009; Abusleme et al., 2021).
Despite the absence of significant differences in clinical periodontitis, the obese and control groups exhibited differences in oral microbiome composition, especially in the subgingival plaque samples. These findings suggest a strong association between obesity and periodontitis. Three of the eight longitudinal studies included in this systematic review reported a direct association between obesity and the development of periodontitis (Keller et al., 2015). Our study provides evidence of an oral microbiome base that support the associations found in these studies. The species that were significantly increased in the obese group (Figure 4) were present in relatively low proportions in the overall microbiome; however, they were associated with the onset of periodontal disease. Therefore, although periodontal disease is currently mild in these individuals, its severity is likely to increase in the future. This is also related to the finding that periodontal disease and the healthy state do not differ significantly in the type of microbiome. The difference between the healthy state, gingivitis, and periodontitis lies in the frequency or composition ratio of the microbiome (Chen et al., 2018; Abusleme et al., 2021).
The possibility of a close relationship between obesity and the oral microbiome was explored by comparing the pre-and post-bariatric surgery microbiomes, which revealed that post-bariatric surgery saliva and buccal samples were dominated by healthy and gingivitis-associated microbiomes (Figures 5A,B). There was a gradual change in the microbiome at 3- and 6-months post-surgery, which was more clearly observed through cluster analysis. Cluster analysis of the microbiome, categorized according to decreasing or increasing patterns, was performed using saliva and buccal swabs (Figures 5C,D). In the swab samples, clusters that decreased after surgery included species associated with periodontitis such as Filifactor alocis, Fusobacterium nucleatum subsp. vincentii, Porphyromonas gingivalis, and Prevotella intermedia. Clusters that increased after surgery included Kingella, Streptococcus, Rothia, and Veillonella spp., which are commensal microbes found in a healthy state. Veillonella spp. can serve as bridges or commensal species in the absence of periodontitis (Zhou et al., 2021).
In saliva samples, a decrease after surgery was observed in clusters 7 and 8, whereas an increase was observed in cluster 1. Among the species that show a tendency to decrease, Porphyromonas, Stomatobaculum spp., and Porphyromonas pasteri are species associated with periodontitis, while Prevotella histicola, which shows a significant increase in saliva cluster 1, is associated with healthy subjects (Moritani et al., 2015). Although the trending species in the saliva and buccal swab samples were not the same, we observed a decrease in the microbiome species associated with periodontitis after bariatric surgery, along with an improvement in obesity-related clinical examination values, suggesting that there may be a link between obesity and periodontal disease through the oral microbiome.
There are notable differences between the outcomes of bariatric surgery and medications such as metformin and statins in terms of oral microbiome alteration and clinical enhancement. Metformin, commonly used for diabetes management, has been shown to decrease the relative proportion of disease-related oral microbiota (Sun et al., 2020). Similarly, statins used for hyperlipidemia are associated with minor changes in oral microbial composition (Kamińska et al., 2019; DeClercq et al., 2021). However, these medications exhibit limited effects on reshaping the oral microbiome toward a healthier state. With the emergence of novel weight loss medications, such as GLP-1 receptor agonists, the paradigm of obesity treatment is rapidly evolving. These pharmacological agents provide non-surgical options for significant weight loss, potentially challenging the traditional dominance of bariatric surgery. This shift underscores the importance of investigating how different methods of weight loss—surgical or pharmacological—affect the oral microbiota. Understanding how quantitative changes in body weight led to qualitative shifts in microbial communities presents an intriguing avenue for future research. While our findings suggest that bariatric surgery induces more substantial shifts in the oral microbiome compared to medication-based approaches, further studies are needed to confirm these effects. The observed alterations in microbial composition post-surgery, coupled with clinical improvements, highlight the potential of surgical interventions to influence both metabolic and oral health outcomes.
Our study had several limitations. First, the number of subjects in the bariatric surgery group was not large enough, and additional subject loss during the follow-up period limited our ability to identify more than a trend toward changes in the microbiome after surgery. While the Control and Obese groups had an adequate number of subjects for microbiome analysis, the bariatric surgery group had a relatively small sample size, which tended to be insufficient for achieving reliable statistical power (Kelly et al., 2015). In general, the identification of species from an insufficient number of analyzed samples may have limitations, as many oral microbiomes can be present in both symbiotic and dysbiotic situations in a healthy state, gingivitis, or periodontitis (Colombo and Tanner, 2019). However, our observations suggest that as obesity improves, there appears to be a trend toward changes in the oral microbiome that may potentially reduce the abundance of species associated with dysbiosis and periodontal disease. Second, we were unable to obtain subgingival plaque samples after surgery because the OS group did not visit a dentist; this requires further investigation. Third, another limitation of this study is the lack of dietary data and caries data for the obese patient group. Pre-surgery high intake of carbohydrates and fats, as well as post-surgery dietary changes, can influence not only obesity but also the composition of the oral microbiome (Millen et al., 2022). Recent research by Millen et al. has shown that fermentable carbohydrates (e.g., sucrose) promote the growth of cariogenic oral bacteria such as Streptococcus mutans. However, with the exception of Leptotrichia spp., this study did not find significant effects on the periodontal disease-associated microbiomes that showed changes in our research. Future research should be designed to incorporate dietary changes and analyze their association with microbiome alterations. This would provide a more comprehensive understanding of the relationship between diet, obesity, and oral microbiome composition. In addition, the smoking rate in the obese group showed a significant difference, which could potentially influence the study results. In our study, Capnocytophaga in buccal swab samples and Leptotrichia in subgingival plaque samples were genera that were more prevalent in the obese group. These genera have also been reported in other studies as being dominant in smokers (Wu et al., 2016). While it cannot be conclusively stated that smoking had the greatest impact on the oral microbiome characteristics of the obese group, it is clear that smoking is a factor that can influence the oral microbiome. Future studies should consider this when designing their research.
Notwithstanding, this is the first study to identify changes in periodontitis-associated bacteria in saliva and buccal swabs after bariatric surgery, and notably, these bacteria showed a significant decrease over time.
In summary, a comparison of the oral microbiota of obese and normal-weight groups without significant differences in clinical periodontal disease showed differences in the oral microbiome especially in the subgingival plaque samples, with a notable presence of species related to periodontal disease. Oral microbiome cluster analysis 6 months after bariatric surgery indicated the potential for changes in the oral microbiome toward a healthy state. Consequently, individuals with obesity need careful oral health management through regular check-ups to prevent periodontitis.
The datasets presented in this study can be found in online repositories. The names of the repository/repositories and accession number(s) can be found at: https://www.ncbi.nlm.nih.gov/, PRJEB74337.
The studies involving humans were approved by Institutional Review Board of Seoul National University Bundang Hospital. The studies were conducted in accordance with the local legislation and institutional requirements. The participants provided their written informed consent to participate in this study.
K-SK: Conceptualization, Data curation, Formal analysis, Methodology, Validation, Writing – original draft, Writing – review & editing. HN: Conceptualization, Formal analysis, Methodology, Software, Validation, Visualization, Writing – original draft, Writing – review & editing. TO: Data curation, Writing – original draft, Writing – review & editing. HH: Data curation, Writing – review & editing. JK: Data curation, Writing – review & editing. J-SH: Data curation, Writing – review & editing. H-JL: Conceptualization, Data curation, Funding acquisition, Writing – review & editing. YP: Conceptualization, Data curation, Methodology, Writing – review & editing. JC: Conceptualization, Data curation, Formal analysis, Funding acquisition, Writing – review & editing.
The author(s) declare that financial support was received for the research and/or publication of this article. This work was supported by National Research Foundation of Korea (NRF) grants funded by the Korean government (No. 2023R1A2C2002783), Seoul National University Bundang Hospital (No. 06-2021-0228), and the National Research Foundation of Korea (NRF) funded by the Ministry of Science & ICT (NRF-2017M3A9B6062027).
The authors would like to thank all the subjects who provided the specimens for this study.
The authors declare that the research was conducted in the absence of any commercial or financial relationships that could be construed as a potential conflict of interest.
The authors declare that no Gen AI was used in the creation of this manuscript.
All claims expressed in this article are solely those of the authors and do not necessarily represent those of their affiliated organizations, or those of the publisher, the editors and the reviewers. Any product that may be evaluated in this article, or claim that may be made by its manufacturer, is not guaranteed or endorsed by the publisher.
The Supplementary material for this article can be found online at: https://www.frontiersin.org/articles/10.3389/fmicb.2025.1553404/full#supplementary-material
SUPPLEMENTARY FIGURE 1 | Relative abundance of major phyla.
SUPPLEMENTARY FIGURE 2 | Relative abundance of major genera.
Abusleme, L., Hoare, A., Hong, B. Y., and Diaz, P. I. (2021). Microbial signatures of health, gingivitis, and periodontitis. Periodontol. 86, 57–78. doi: 10.1111/prd.12362
Arnold, M., Pandeya, N., Byrnes, G., Renehan, P. A. G., Stevens, G. A., Ezzati, P. M., et al. (2015). Global burden of cancer attributable to high body-mass index in 2012: a population-based study. Lancet Oncol. 16, 36–46. doi: 10.1016/S1470-2045(14)71123-4
Baker, J. L., Mark Welch, J. L., Kauffman, K. M., McLean, J. S., and He, X. (2024). The oral microbiome: diversity, biogeography and human health. Nat. Rev. Microbiol. 22, 89–104. doi: 10.1038/s41579-023-00963-6
Chen, C., Hemme, C., Beleno, J., Shi, Z. J., Ning, D., Qin, Y., et al. (2018). Oral microbiota of periodontal health and disease and their changes after nonsurgical periodontal therapy. ISME J. 12, 1210–1224. doi: 10.1038/s41396-017-0037-1
Colombo, A. P. V., and Tanner, A. C. R. (2019). The role of bacterial biofilms in dental caries and periodontal and peri-implant diseases: a historical perspective. J. Dent. Res. 98, 373–385. doi: 10.1177/0022034519830686
Darby, I., and Curtis, M. (2001). Microbiology of periodontal disease in children and young adults. Periodontol. 26, 33–53. doi: 10.1034/j.1600-0757.2001.2260103.x
DeClercq, V., Nearing, J. T., and Langille, M. G. I. (2021). Investigation of the impact of commonly used medications on the oral microbiome of individuals living without major chronic conditions. PLoS One 16:e0261032. doi: 10.1371/journal.pone.0261032
Džunková, M., Lipták, R., Vlková, B., Gardlík, R., Čierny, M., Moya, A., et al. (2020). Salivary microbiome composition changes after bariatric surgery. Sci. Rep. 10:20086. doi: 10.1038/s41598-020-76991-6
Eke, P. I., Page, R. C., Wei, L., Thornton-Evans, G., and Genco, R. J. (2012). Update of the case definitions for population-based surveillance of periodontitis. J. Periodontol. 83, 1449–1454. doi: 10.1902/jop.2012.110664
Fernandes, A. D., Reid, J. N., Macklaim, J. M., McMurrough, T. A., Edgell, D. R., and Gloor, G. B. (2014). Unifying the analysis of high-throughput sequencing datasets: characterizing RNA-seq, 16S rRNA gene sequencing and selective growth experiments by compositional data analysis. Microbiome 2, 1–13. doi: 10.1186/2049-2618-2-15
Furet, J. P., Kong, L. C., Tap, J., Poitou, C., Basdevant, A., Bouillot, J. L., et al. (2010). Differential adaptation of human gut microbiota to bariatric surgery-induced weight loss: links with metabolic and low-grade inflammation markers. Diabetes 59, 3049–3057. doi: 10.2337/db10-0253
Ganesan, S. M., Vazana, S., and Stuhr, S. (2021). Waistline to the gumline: relationship between obesity and periodontal disease-biological and management considerations. Periodontol. 87, 299–314. doi: 10.1111/prd.12390
Gasmi Benahmed, A., Gasmi, A., Doşa, A., Chirumbolo, S., Mujawdiya, P. K., Aaseth, J., et al. (2021). Association between the gut and oral microbiome with obesity. Anaerobe 70:102248. doi: 10.1016/j.anaerobe.2020.102248
GBD 2015 Obesity CollaboratorsAfshin, A., Forouzanfar, M. H., Reitsma, M. B., Sur, P., Estep, K., et al. (2017). Health effects of overweight and obesity in 195 countries over 25 years. N. Engl. J. Med. 377, 13–27. doi: 10.1056/NEJMoa1614362
Goodson, J. M., Groppo, D., Halem, S., and Carpino, E. (2009). Is obesity an oral bacterial disease? J. Dent. Res. 88, 519–523. doi: 10.1177/0022034509338353
Hall, M., and Beiko, R. G. (2018). 16S rRNA gene analysis with QIIME2. Methods Mol. Biol. 1849, 113–129. doi: 10.1007/978-1-4939-8728-3_8
Hiranmayi, K. V., Sirisha, K., Ramoji Rao, M. V., and Sudhakar, P. (2017). Novel pathogens in periodontal microbiology. J. Pharm. Bioallied Sci. 9, 155–163. doi: 10.4103/jpbs.JPBS_288_16
Hruby, A., and Hu, F. B. (2015). The epidemiology of obesity: a big picture. PharmacoEconomics 33, 673–689. doi: 10.1007/s40273-014-0243-x
Kamińska, M., Aliko, A., Hellvard, A., Bielecka, E., Binder, V., Marczyk, A., et al. (2019). Effects of statins on multispecies oral biofilm identify simvastatin as a drug candidate targeting Porphyromonas gingivalis. J. Periodontol. 90, 637–646. doi: 10.1002/JPER.18-0179
Keller, A., Rohde, J. F., Raymond, K., and Heitmann, B. L. (2015). Association between periodontal disease and overweight and obesity: a systematic review. J. Periodontol. 86, 766–776. doi: 10.1902/jop.2015.140589
Kelly, B. J., Gross, R., Bittinger, K., Sherrill-Mix, S., Lewis, J. D., Collman, R. G., et al. (2015). Power and sample-size estimation for microbiome studies using pairwise distances and PERMANOVA. Bioinformatics 31, 2461–2468. doi: 10.1093/bioinformatics/btv183
Kim, H., Hong, J. S., Yun, P. Y., Hwang, K. G., Kim, K. S., Lee, H. J., et al. (2023). Exploration of the interplay between spatially distinct microbial habitats through comparative analysis. J. Oral Microbiol. 15:2229693. doi: 10.1080/20002297.2023.2229693
Koliada, A., Syzenko, G., Moseiko, V., Budovska, L., Puchkov, K., Perederiy, V., et al. (2017). Association between body mass index and Firmicutes/Bacteroidetes ratio in an adult Ukrainian population. BMC Microbiol. 17:120. doi: 10.1186/s12866-017-1027-1
Lauby-Secretan, B., Scoccianti, C., Loomis, D., Grosse, Y., Bianchini, F., Straif, K., et al. (2016). Body fatness and cancer--viewpoint of the IARC working group. N. Engl. J. Med. 375, 794–798. doi: 10.1056/NEJMsr1606602
Liou, A. P., Paziuk, M., Luevano, J. M. Jr., Machineni, S., Turnbaugh, P. J., and Kaplan, L. M. (2013). Conserved shifts in the gut microbiota due to gastric bypass reduce host weight and adiposity. Sci. Transl. Med. 5:178ra41. doi: 10.1126/scitranslmed.3005687
Maciejewski, M. L., Arterburn, D. E., Van Scoyoc, L., Smith, V. A., Yancy, W. S. Jr., Weidenbacher, H. J., et al. (2016). Bariatric surgery and long-term durability of weight loss. JAMA Surg. 151, 1046–1055. doi: 10.1001/jamasurg.2016.2317
Mandal, S., Van Treuren, W., White, R. A., Eggesbø, M., Knight, R., and Peddada, S. D. (2015). Analysis of composition of microbiomes: a novel method for studying microbial composition. Microb. Ecol. Health Dis. 26:27663. doi: 10.3402/mehd.v26.27663
Manzoor, M., Leskelä, J., Pietiäinen, M., Martinez-Majander, N., Könönen, E., Niiranen, T., et al. (2024). Shotgun metagenomic analysis of the oral microbiome in gingivitis: a nested case-control study. J. Oral Microbiol. 16:2330867. doi: 10.1080/20002297.2024.2330867
Mathur, R., and Barlow, G. M. (2015). Obesity and the microbiome. Expert Rev. Gastroenterol. Hepatol. 9, 1087–1099. doi: 10.1586/17474124.2015.1051029
Matuliene, G., Pjetursson, B. E., Salvi, G. E., Schmidlin, K., Brägger, U., Zwahlen, M., et al. (2008). Influence of residual pockets on progression of periodontitis and tooth loss: results after 11 years of maintenance. J. Clin. Periodontol. 35, 685–695. doi: 10.1111/j.1600-051X.2008.01245.x
McCracken, G., Asuni, A., Ritchie, M., Vernazza, C., and Heasman, P. (2017). Failing to meet the goals of periodontal recall programs. What next? Periodontol 2000 75, 330–352. doi: 10.1111/prd.12159
Millen, A. E., Dahhan, R., Freudenheim, J. L., Hovey, K. M., Li, L., McSkimming, D. I., et al. (2022). Dietary carbohydrate intake is associated with the subgingival plaque oral microbiome abundance and diversity in a cohort of postmenopausal women. Sci. Rep. 12:2643. doi: 10.1038/s41598-022-06421-2
Mineoka, T., Awano, S., Rikimaru, T., Kurata, H., Yoshida, A., Ansai, T., et al. (2008). Site-specific development of periodontal disease is associated with increased levels of Porphyromonas gingivalis, Treponema denticola, and Tannerella forsythia in subgingival plaque. J. Periodontol. 79, 670–676. doi: 10.1902/jop.2008.070398
Moritani, K., Takeshita, T., Shibata, Y., Ninomiya, T., Kiyohara, Y., and Yamashita, Y. (2015). Acetaldehyde production by major oral microbes. Oral Dis. 21, 748–754. doi: 10.1111/odi.12341
Na, H. S., Song, Y., Yu, Y., and Chung, J. (2023). Comparative analysis of primers used for 16S rRNA gene sequencing in oral microbiome studies. Methods Protoc. 6:71. doi: 10.3390/mps6040071
Nadkarni, M. A., Browne, G. V., Chhour, K. L., Byun, R., Nguyen, K. A., Chapple, C. C., et al. (2012). Pattern of distribution of Prevotella species/phylotypes associated with healthy gingiva and periodontal disease. Eur. J. Clin. Microbiol. Infect. Dis. 31, 2989–2999. doi: 10.1007/s10096-012-1651-5
NIH HMP Working GroupPeterson, J., Garges, S., Giovanni, M., McInnes, P., Wang, L., et al. (2009). The NIH human microbiome project. Genome Res. 19, 2317–2323. doi: 10.1101/gr.096651.109
Reytor-González, C., Parise-Vasco, J. M., González, N., Simancas-Racines, A., Zambrano-Villacres, R., Zambrano, A. K., et al. (2024). Obesity and periodontitis: a comprehensive review of their interconnected pathophysiology and clinical implications. Front. Nutr. 11:1440216. doi: 10.3389/fnut.2024.1440216
Ridaura, V. K., Faith, J. J., Rey, F. E., Cheng, J., Duncan, A. E., Kau, A. L., et al. (2013). Gut microbiota from twins discordant for obesity modulate metabolism in mice. Science 341:1241214. doi: 10.1126/science.1241214
Schauer, P. R., Bhatt, D. L., Kirwan, J. P., Wolski, K., Aminian, A., Brethauer, S. A., et al. (2017). Bariatric surgery versus intensive medical therapy for diabetes −5-year outcomes. N. Engl. J. Med. 376, 641–651. doi: 10.1056/NEJMoa1600869
Segata, N., Izard, J., Waldron, L., Gevers, D., Miropolsky, L., Garrett, W. S., et al. (2011). Metagenomic biomarker discovery and explanation. Genome Biol. 12:R60. doi: 10.1186/gb-2011-12-6-r60
Shen, J., Obin, M. S., and Zhao, L. (2013). The gut microbiota, obesity and insulin resistance. Mol. Asp. Med. 34, 39–58. doi: 10.1016/j.mam.2012.11.001
Socransky, S. S., Haffajee, A. D., Cugini, M. A., Smith, C., and Kent, R. L. (1998). Microbial complexes in subgingival plaque. J. Clin. Periodontol. 25, 134–144. doi: 10.1111/j.1600-051X.1998.tb02419.x
Stefura, T., Zapała, B., Gosiewski, T., Skomarovska, O., Pędziwiatr, M., and Major, P. (2022). Changes in the composition of oral and intestinal microbiota after sleeve gastrectomy and roux-en-Y gastric bypass and their impact on outcomes of bariatric surgery. Obes. Surg. 32, 1439–1450. doi: 10.1007/s11695-022-05954-9
Sun, X., Li, M., Xia, L., Fang, Z., Yu, S., Gao, J., et al. (2020). Alteration of salivary microbiome in periodontitis with or without type-2 diabetes mellitus and metformin treatment. Sci. Rep. 10:15363. doi: 10.1038/s41598-020-72035-1
Tam, J., Hoffmann, T., Fischer, S., Bornstein, S., Gräßler, J., and Noack, B. (2018). Obesity alters composition and diversity of the oral microbiota in patients with type 2 diabetes mellitus independently of glycemic control. PLoS One 13:e0204724. doi: 10.1371/journal.pone.0204724
Turnbaugh, P. J., Ley, R. E., Mahowald, M. A., Magrini, V., Mardis, E. R., and Gordon, J. I. (2006). An obesity-associated gut microbiome with increased capacity for energy harvest. Nature 444, 1027–1031. doi: 10.1038/nature05414
Wade, W. G. (2013). The oral microbiome in health and disease. Pharmacol. Res. 69, 137–143. doi: 10.1016/j.phrs.2012.11.006
Wu, J., Peters, B. A., Dominianni, C., Zhang, Y., Pei, Z., Yang, L., et al. (2016). Cigarette smoking and the oral microbiome in a large study of American adults. ISME J. 10, 2435–2446. doi: 10.1038/ismej.2016.37
Yang, Y., Cai, Q., Zheng, W., Steinwandel, M., Blot, W. J., Shu, X.-O., et al. (2019). Oral microbiome and obesity in a large study of low-income and African-American populations. J. Oral Microbiol. 11:1650597. doi: 10.1080/20002297.2019.1650597
Zaura, E., Keijser, B. J. F., Huse, S. M., and Crielaard, W. (2009). Defining the healthy “core microbiome” of oral microbial communities. BMC Microbiol. 9:259. doi: 10.1186/1471-2180-9-259
Keywords: obesity, bariatric surgery, oral microbiome, periodontal disease, saliva
Citation: Kim K-S, Na HS, Oh TJ, Han H, Kim J, Hong J-S, Lee H-J, Park YS and Chung J (2025) Oral microbiome changes in subjects with obesity following bariatric surgery compared to lean counterparts. Front. Microbiol. 16:1553404. doi: 10.3389/fmicb.2025.1553404
Received: 30 December 2024; Accepted: 27 February 2025;
Published: 18 March 2025.
Edited by:
Franck Carbonero, Washington State University Health Sciences Spokane, United StatesReviewed by:
Megan L. Falsetta, University of Rochester, United StatesCopyright © 2025 Kim, Na, Oh, Han, Kim, Hong, Lee, Park and Chung. This is an open-access article distributed under the terms of the Creative Commons Attribution License (CC BY). The use, distribution or reproduction in other forums is permitted, provided the original author(s) and the copyright owner(s) are credited and that the original publication in this journal is cited, in accordance with accepted academic practice. No use, distribution or reproduction is permitted which does not comply with these terms.
*Correspondence: Hyo-Jung Lee, cGVyaW9sZWVAZ21haWwuY29t; Young Suk Park eW91bmdzdWttZEBnbWFpbC5jb20=; Jin Chung amNodW5nQHB1c2FuLmFjLmty
†These authors have contributed equally to this work and share first authorship
Disclaimer: All claims expressed in this article are solely those of the authors and do not necessarily represent those of their affiliated organizations, or those of the publisher, the editors and the reviewers. Any product that may be evaluated in this article or claim that may be made by its manufacturer is not guaranteed or endorsed by the publisher.
Research integrity at Frontiers
Learn more about the work of our research integrity team to safeguard the quality of each article we publish.