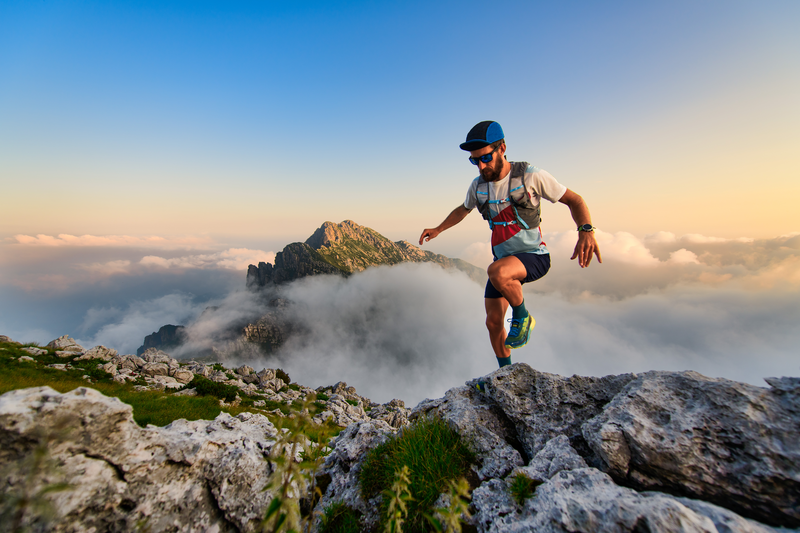
94% of researchers rate our articles as excellent or good
Learn more about the work of our research integrity team to safeguard the quality of each article we publish.
Find out more
ORIGINAL RESEARCH article
Front. Microbiol.
Sec. Microbial Symbioses
Volume 16 - 2025 | doi: 10.3389/fmicb.2025.1548808
The final, formatted version of the article will be published soon.
You have multiple emails registered with Frontiers:
Please enter your email address:
If you already have an account, please login
You don't have a Frontiers account ? You can register here
Enterobacter cancerogenus (E. cancerogenus) is a facultative anaerobic, gram-negative bacterium that can be utilized for the biological control of pests. However, the molecular mechanisms underlying the pathogenicity of E. cancerogenus in insect hosts remain largely unexplored. In this study, the Bombyx mori model was employed to investigate the pathogenicity of Enterobacter cancerogenus strain ECL7, a bacterium pathogenic to silkworms, through whole-genome sequencing, 16S rDNA sequencing, and transcriptome analysis. The results revealed that ECL7 harbors virulence genes associated with biofilm formation, adhesion, type III secretion system (T3SS), type VI secretion system (T6SS), and other factors, which collectively lead to damage to the peritrophic matrix (PM) and intestinal epithelial cells of the silkworm midgut following infection, and reduced silkworm larval survival rates and inhibited their growth and development. Additionally, ECL7 infection altered the composition and abundance of intestinal microorganisms, with Enterobacteriaceae and Enterobacteriales becoming dominant species. ECL7 also stimulated the expression of genes related to the Toll and IMD immune signaling pathways, resulting in the upregulation of antimicrobial peptide–related differentially expressed genes (DEGs). Furthermore, transcriptomic analysis revealed an upregulation of DEGs associated with oxidative stress in response to ECL7 infection. This study provides valuable insights into the molecular mechanisms underlying the interaction between E. cancerogenus and silkworms. The findings contribute to the prevention and control of infections caused by this bacterium in sericulture production and offer novel ideas for the potential application of E. cancerogenus in pest biological control.
Keywords: Enterobacter cancerogenus, Bombyx mori, intestinal infection, Bacterial disease, Interaction, Gut Microbiota, RNA-Seq
Received: 20 Dec 2024; Accepted: 07 Mar 2025.
Copyright: © 2025 Luo, Lai, Wu, Ren, Zhao, Liu and Long. This is an open-access article distributed under the terms of the Creative Commons Attribution License (CC BY). The use, distribution or reproduction in other forums is permitted, provided the original author(s) or licensor are credited and that the original publication in this journal is cited, in accordance with accepted academic practice. No use, distribution or reproduction is permitted which does not comply with these terms.
* Correspondence:
Hongmei Liu, Engineering Research Center of Medical Biotechnology, School of Biology and Engineering, Guizhou Medical University, Anshun, China
Yaohang Long, Engineering Research Center of Medical Biotechnology, School of Biology and Engineering, Guizhou Medical University, Anshun, China
Disclaimer: All claims expressed in this article are solely those of the authors and do not necessarily represent those of their affiliated organizations, or those of the publisher, the editors and the reviewers. Any product that may be evaluated in this article or claim that may be made by its manufacturer is not guaranteed or endorsed by the publisher.
Research integrity at Frontiers
Learn more about the work of our research integrity team to safeguard the quality of each article we publish.