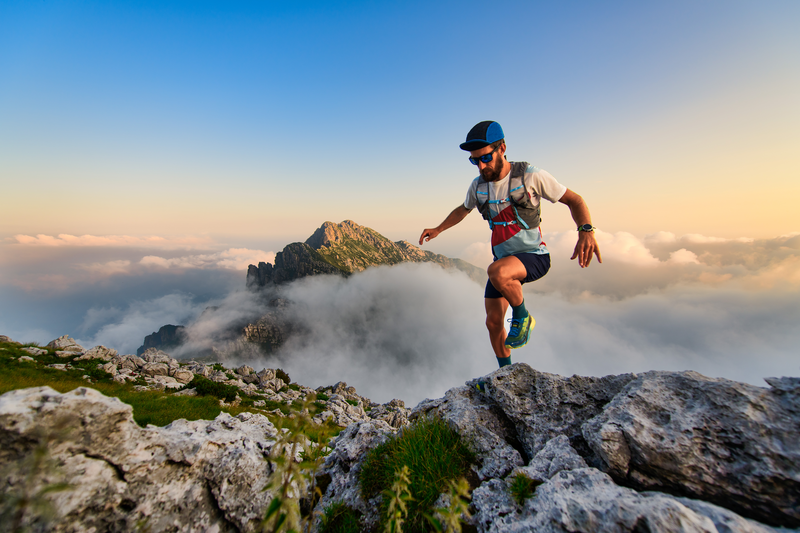
94% of researchers rate our articles as excellent or good
Learn more about the work of our research integrity team to safeguard the quality of each article we publish.
Find out more
ORIGINAL RESEARCH article
Front. Microbiol.
Sec. Systems Microbiology
Volume 16 - 2025 | doi: 10.3389/fmicb.2025.1544934
This article is part of the Research Topic Methods for Imaging and Omics Data Science: Advances, Applications, and Spatiotemporal Innovations View all 9 articles
The final, formatted version of the article will be published soon.
You have multiple emails registered with Frontiers:
Please enter your email address:
If you already have an account, please login
You don't have a Frontiers account ? You can register here
Next-generation sequencing (NGS) has played a pivotal role in the advancement of taxonomics, allowing for the accurate identification, differentiation, and reclassification of several bacteria species. Bacillus velezensis is a Gram-positive, facultatively aerobic, spore-forming bacterium known 2 for its antimicrobial and antifungal properties. Strains of this species are highly relevant in agriculture, biotechnology, the food industry, and biomedicine. In this study, we characterized the genomes of nine Bacillus strains isolated from soil in the state of Bahia (Brazil) using NGS with Illumina platform. Identification was performed by Average Nucleotide Identity (ANI) and digital DNA-DNA hybridization (dDDH) analyses, which revealed a match between the genomic information of the isolates and B. velezensis NRRL B-41580, with a variation of 89.3% to 91.8% by dDDH in TYGS and 95% to 98.04% by ANI in GTDBtk. Two strains, BAC144 and BAC1273, exhibited high similarity to B. amyloliquefaciens subsp. plantarum FZB42. However, the latter strain was subsequently reclassified as B. velezensis. The division pattern observed during identification was confirmed in the phylogenomic analysis, where BAC144 and BAC1273 clustered with Bacillus amyloliquefaciens subsp. plantarum, while the other strains clustered with B. velezensis NRRL B-41580, forming a clade with high genetic similarity, with a bootstrap value of 100%. Furthermore, a synteny analysis demonstrated greater conservation among the strains from this study compared to the reference strain, with the formation of distinct collinear groups. The pangenome analysis revealed an open pangenome, highlighting the genetic diversity within the species. Based on this analysis, a functional annotation was performed to compare exclusive gene repertoires across groups, uncovering distinct adaptations and functional profiles. The identification of bacterial strains belonging to this species is of great importance due to their high applicability. The strains identified in this study underscore the need for more robust taxonomic technologies to accurately classify prokaryotes, which are subject to constant evolutionary changes, requiring the reclassification of several species within the genus Bacillus, many of which are heterotypic synonyms of B. velezensis like Bacillus oryzicola, B. amyloliquefaciens subsp. plantarum and Bacillus methylotrophicus.
Keywords: identification1, characterization2, sequencing3, Bacillus velezensis4, genomic5
Received: 13 Dec 2024; Accepted: 24 Feb 2025.
Copyright: © 2025 Sousa, Campos, Viana, Gomes, Rodrigues, Aburjaile, Fonseca, Batista, Costa, GUEDON, Brenig, Soares and Azevedo. This is an open-access article distributed under the terms of the Creative Commons Attribution License (CC BY). The use, distribution or reproduction in other forums is permitted, provided the original author(s) or licensor are credited and that the original publication in this journal is cited, in accordance with accepted academic practice. No use, distribution or reproduction is permitted which does not comply with these terms.
* Correspondence:
Eduarda Sousa, Laboratory of Cellular and Molecular Genetics, Institute of Biological Sciences, Federal University of Minas Gerais, Belo Horizonte, Minas Gerais, Brazil
Gabriela Campos, Laboratory of Cellular and Molecular Genetics, Institute of Biological Sciences, Federal University of Minas Gerais, Belo Horizonte, Minas Gerais, Brazil
Vasco Ariston De Carvalho Azevedo, Laboratory of Cellular and Molecular Genetics, Institute of Biological Sciences, Federal University of Minas Gerais, Belo Horizonte, Minas Gerais, Brazil
Disclaimer: All claims expressed in this article are solely those of the authors and do not necessarily represent those of their affiliated organizations, or those of the publisher, the editors and the reviewers. Any product that may be evaluated in this article or claim that may be made by its manufacturer is not guaranteed or endorsed by the publisher.
Research integrity at Frontiers
Learn more about the work of our research integrity team to safeguard the quality of each article we publish.