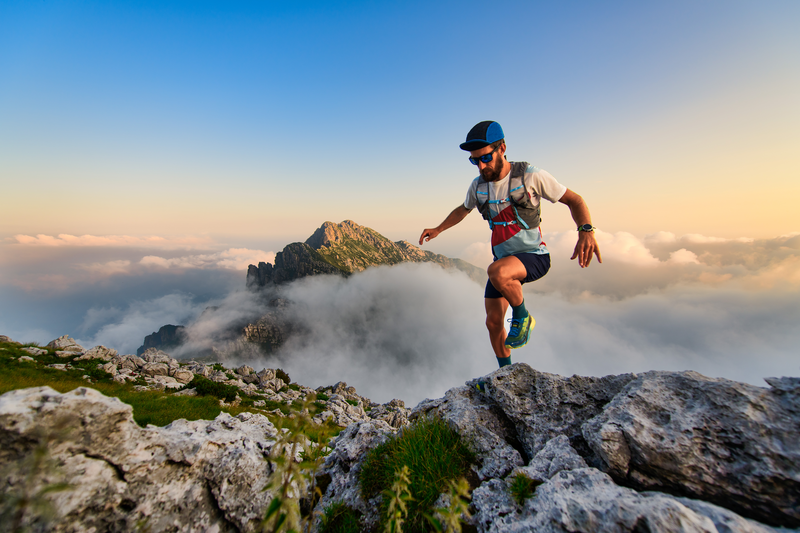
94% of researchers rate our articles as excellent or good
Learn more about the work of our research integrity team to safeguard the quality of each article we publish.
Find out more
ORIGINAL RESEARCH article
Front. Microbiol.
Sec. Aquatic Microbiology
Volume 16 - 2025 | doi: 10.3389/fmicb.2025.1544005
The final, formatted version of the article will be published soon.
You have multiple emails registered with Frontiers:
Please enter your email address:
If you already have an account, please login
You don't have a Frontiers account ? You can register here
Polyunsaturated fatty acids (PUFAs) are vital to the physiological functioning of crustacean zooplankton. However, cyanobacteria blooms frequently lead to PUFA deficiencies, which poses a substantial challenge to population fitness. Therefore, we hypothesize that D. magna adapt to PUFA-deficient conditions by prioritizing PUFA allocation to somatic growth, and then to offspring during reproduction to ensure population persistence. To test this hypothesis, we applied (compound-specific) 13 C labeling to compare the turnover of total carbon and certain groups of fatty acids in Daphnia magna fed with Scenedesmus bijuba for 6 days and then switching to a diet of 13 C labeled Microcystis wesenbergii for 6 days (with food quality deterioration) or to a diet of 13 C-labeled Scenedesmus (without food quality deterioration), respectively.Fatty acid profiles of D. magna mothers and offspring were also analyzed to reveal their PUFA allocation strategies. Life table parameters from D. magna-feeding Scenedesmus switching to Microcystis were compared with D. magna fed with only Scenedesmus or Microcystis to reveal the effect of PUFA allocation on D. magna performance. Our results showed that with food quality deterioration, D. magna exhibited a significantly lower PUFA and carbon turnover and higher offspring:mother ratios in their PUFA contents. Despite this reduced reproduction, the D. magna switching diets showed no significant different intrinsic increasing rate of populations with those fed only Scenedesmus. Meanwhile, the D. magna switching diets performed significantly better than D. magna fed only Microcystis.These results suggest that differential fatty acid allocation of consumers may serve as an adaptive strategy for population maintenance in food quality deterioration and provide ecological implications with cyanobacterial bloom management and Daphnia reproductive plasticity, which needs further explorations.
Keywords: Zooplankton, Microcystis, food quality, Compound-specific stable isotope analysis, polyunsaturated fatty acids
Received: 12 Dec 2024; Accepted: 11 Feb 2025.
Copyright: © 2025 Tang, Wang, Liu, Su, Zhang, Xiaotong, Jin, Su, Wang and Jeppesen. This is an open-access article distributed under the terms of the Creative Commons Attribution License (CC BY). The use, distribution or reproduction in other forums is permitted, provided the original author(s) or licensor are credited and that the original publication in this journal is cited, in accordance with accepted academic practice. No use, distribution or reproduction is permitted which does not comply with these terms.
* Correspondence:
Yali Tang, Jinan University, Guangzhou, China
Disclaimer: All claims expressed in this article are solely those of the authors and do not necessarily represent those of their affiliated organizations, or those of the publisher, the editors and the reviewers. Any product that may be evaluated in this article or claim that may be made by its manufacturer is not guaranteed or endorsed by the publisher.
Research integrity at Frontiers
Learn more about the work of our research integrity team to safeguard the quality of each article we publish.