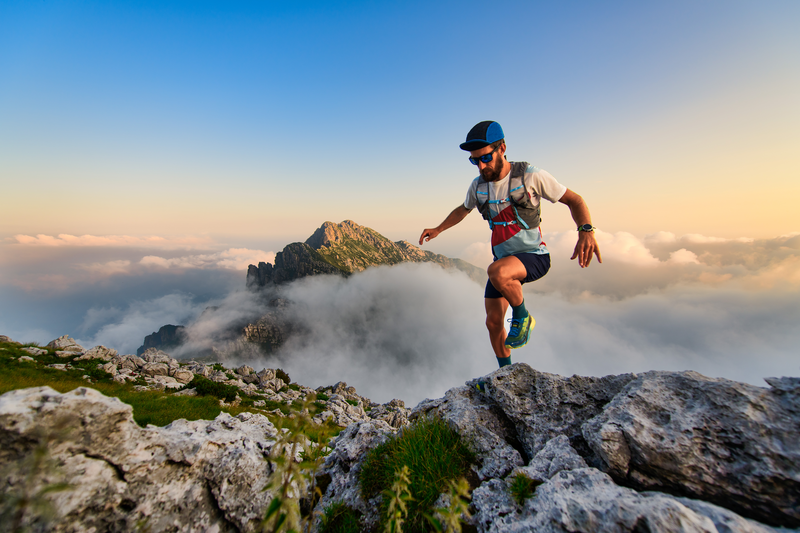
94% of researchers rate our articles as excellent or good
Learn more about the work of our research integrity team to safeguard the quality of each article we publish.
Find out more
ORIGINAL RESEARCH article
Front. Microbiol.
Sec. Microbial Symbioses
Volume 16 - 2025 | doi: 10.3389/fmicb.2025.1531847
This article is part of the Research Topic Community Series in the Wildlife Gut Microbiome and Its Implication for Conservation Biology, Volume III View all 10 articles
The final, formatted version of the article will be published soon.
You have multiple emails registered with Frontiers:
Please enter your email address:
If you already have an account, please login
You don't have a Frontiers account ? You can register here
Bacteria often play important roles in the host adaptation of phytophagous insects. Beetles of the genus Curculio (Coleoptera: Curculionidae) include pest species that bore into the seeds of trees in the family Fagaceae and damage the cotyledons. At present, there are few studies of the taxonomic diversity and functional effects of symbiotic bacteria involved in changes in host ranges and host adaptation of Curculio. Here, we used 16S rRNA gene Illumina and metagenomic sequencing to compare the composition and functions of the bacterial communities of three species of host plants and several Curculio species combinations: Curculio bimaculatus feeding on Castanopsis sclerophylla, C. bimaculatus feeding on Castanopsis tibetana, and Curculio david feeding on Ca. tibetana. The host plants influenced the diversity of symbiotic bacteria, while the Curculio species influenced the community structure of the symbiotic bacteria. Functional predictions showed that symbiotic bacteria contributed to the metabolism of the hosts. However, consistent with the variation in bacteria, the major metabolism-related bacterial genera varied among the treatment groups. Comparisons of metabolic enzymes based on KEGG (Kyoto Encyclopedia of Genes and Genomes) annotation revealed differences in the enzymes involved in insect development and detoxification of plant secondary compounds among the three groups, and the patterns were influenced by the dominance of the Curculio species on the host plants. This study provides valuable insights into the possible role of symbiotic bacteria in Curculio as host insects.
Keywords: symbiotic bacteria, Curculio, Host Adaptation, Host-microbe association, Bacteria composition
Received: 21 Nov 2024; Accepted: 24 Feb 2025.
Copyright: © 2025 LIU, YING, LI, ZHANG and SHU. This is an open-access article distributed under the terms of the Creative Commons Attribution License (CC BY). The use, distribution or reproduction in other forums is permitted, provided the original author(s) or licensor are credited and that the original publication in this journal is cited, in accordance with accepted academic practice. No use, distribution or reproduction is permitted which does not comply with these terms.
* Correspondence:
WEI ZHANG, Research Institute of Subtropical Forestry, Chinese Academy of Forestry, Hangzhou, China
JINPING SHU, Research Institute of Subtropical Forestry, Chinese Academy of Forestry, Hangzhou, China
Disclaimer: All claims expressed in this article are solely those of the authors and do not necessarily represent those of their affiliated organizations, or those of the publisher, the editors and the reviewers. Any product that may be evaluated in this article or claim that may be made by its manufacturer is not guaranteed or endorsed by the publisher.
Research integrity at Frontiers
Learn more about the work of our research integrity team to safeguard the quality of each article we publish.