- Key Laboratory of Animal Disease-Resistance Nutrition, Key Laboratory of Sichuan Province, Ministry of Agriculture and Rural Affairs, Animal Nutrition Institute, Sichuan Agricultural University, Chengdu, China
Introduction: Pediococcus acidilactici CNCM I-4622 (PA) is a homofermentative Gram-positive coccus that produces lactic acid as a major metabolic by-product. However, the potential of PA to improve intestinal function and, as a result, improve production performance and quality in aged laying hens remains unclear. This study aimed to investigate the effects of PA on egg production, egg quality, intestinal health, and cecal microbiota in aged laying hens.
Methods: A total of 180 Lohmann pink laying hens, aged 80 weeks, were randomly assigned to five groups and fed either a basal diet (PA0) or basal diets supplemented with PA at concentrations of 50, 100, 150, and 200 mg/kg (PA50, PA100, PA150, and PA200).
Results: Compared to the PA0 group, PA did not have a significant effect on the production performance of laying hens (p > 0.05). However, the content of diamine oxidase and the expression level of IL-8 mRNA in the PA50, P100, P150, and P200 groups were significantly reduced (p < 0.05). Additionally, the ileal villus height was significantly increased (p < 0.05). The cecal chowder pH and ileal crypt depth were also significantly lower (p < 0.05), while lipase activity in the ileal mucosa of the PA50 group was significantly increased compared to the PA0 group (p < 0.05). Furthermore, the expression of INF-γ and TNF-α mRNA in the jejunal mucosa was significantly down-regulated (p < 0.05), whereas the expression of Claudin mRNA was significantly up-regulated (p < 0.05). Notably, the relative abundance of Bacteroidota, Fusobacteriota, and Fusobacterium in the PA50 group was significantly higher than that in the PA0 group (p < 0.05).
Discussion: Additionally, cecal metabolomic analysis indicated that following the addition of PA, the pathways enriched with differential metabolites were primarily related to arginine and proline metabolism. Therefore, PA has the potential to improve intestinal morphology and flora, mitigate intestinal inflammatory factors, and strengthen intestinal barrier function. These benefits are attributed to the modulation of arginine and proline metabolic pathways, with optimal effects observed at an addition of 50 mg/kg.
Introduction
Due to improved production capabilities among domestic fowl, the average lifespan of commercial laying hens has increased from 72 to 80 weeks (Liu et al., 2022). However, between 80 and 100 weeks of age, hens experience a decline in both egg production and quality. As a result, they are gradually removed from the flock and referred to as ‘spent hens’ and are classified as aged laying hens (Fan and Wu, 2022). The primary challenge faced by these aged laying hens is the decline in intestinal function (Gan et al., 2020; Abdollahi et al., 2021; Feng et al., 2021).
In recent years, there has been a significant surge of interest in extending the production period of hens. Intestinal health is closely associated with the overall well-being, defense mechanisms, and nutritional status of the host as they age (An et al., 2018). Maintaining gastrointestinal tract health is critically important in contemporary poultry farming practices aimed at enhancing production, especially in laying hens (Fu et al., 2022). The gastrointestinal is the primary pathway for nutrient absorption and the initiation of immune responses. However, this pathway is vulnerable to disruption from a variety of stressors, including environmental fluctuations, pathogens, and changes in feed. Furthermore, the gastrointestinal tract is essential for immune response, microbial defense, and hormonal regulation (Fu et al., 2022). Animal guts are host to trillions of microbes that perform vital functions, including the harvesting, storage, and expenditure of energy derived from dietary sources for the host, effectively acting as an additional organ (Becattini et al., 2016). Disruption of the balance among these microorganisms can adversely affect the health and productivity of hens. Consequently, significant efforts are being made to enhance the production performance of aged laying hens through nutritional interventions. These interventions include supplementing exogenous enzymes, prebiotics, probiotics, and minerals (Kim et al., 2012; Abdelqader et al., 2013b; Ghasemian and Jahanian, 2015). In recent years, interest in probiotics has surged, supported by increasing evidence of their efficacy as a promising approach to enhancing digestive health (Lee et al., 2018; Ji et al., 2019; Cai et al., 2020). Among these probiotics, PA has garnered considerable attention due to its potent physiological activity and resilience. PA represents a category of lactic acid bacteria notable for certain key traits, including its resistance to acidic environments, lack of porphyrin synthesis, and a strictly fermentative (homofermentative) metabolism that is facultatively anaerobic, primarily producing lactic acid as the main metabolic byproduct (Li et al., 2021). Furthermore, PA is recognized as a consistent producer of bacteriocins (Parada et al., 2007). Bacteriocins can colonize and persist in the human gut while having a beneficial effect on the host, including modulation of the composition of the gut microbiota, improvement of the host immune response, enhancement of the gut barrier function, and the modulation of immune system activities (Qiao et al., 2021). Collectively, these factors contribute to improved gut health, which subsequently enhances the productivity and quality of eggs produced by laying hens (Quarantelli et al., 2008; Mikulski et al., 2012; Mikulski et al., 2020; Shanmugam et al., 2024). Previous studies have indicated that PA can significantly improve both the production performance and egg quality of laying hens (Mikulski et al., 2012; Mikulski et al., 2020). However, the potential effects of PA on aged laying hens remain unclear. Therefore, the objective of this study is to elucidate the specific impacts of PA on the gut health of aged laying hens, ultimately aiming to improve laying performance and egg quality.
Materials and methods
All experimental procedures were carried out in compliance with the guidelines established by the Animal Welfare Committee. Furthermore, the experimental protocol received approval from the Institutional Animal Care and Use Committee (IACUC) of Sichuan Agricultural University (No. 20181105).
Probiotic preparation
Pediococcus acidilactici (CNCM I-4622 at 1 × 108 CFU/g, Bactocell®; PA) was produced by Lallemand SAS (Blagnac, France).
Experimental design
A total of 180 Lohmann pink laying hens at 80 weeks of age were housed individually in wire-layer cages. The hens were randomly allocated to five treatments with six replicates (six hens per replicate). PA was added in the basal diet (PA0) at concentrations of 50, 100, 150, and 200 mg/kg, respectively. A corn-soybean-meal basal diet in mash form was formulated to meet the nutrient requirements of hens as recommended by the National Research Council (1994) and the feeding standards for laying hens according to the Chinese Feeding Standard of Chicken (NY/T 33-2004) (Table 1). The study duration was 12 weeks (from 80 to 91 weeks of age) with free access to feed and water and a lighting schedule of 16 L:8 D.
Sample collection
At the end of week twelve, a total of 18 eggs from each group (3 eggs per replicate) were collected for egg quality assessment. The egg yolks were then carefully separated, with three yolks from each replicate mixed together and stored at −20°C.
At the end of the trial, 30 hens (one hen per replicate) were randomly selected. Blood samples were obtained via venipuncture from the wing vein using a sterile syringe, followed by centrifugation at 3,500 g for 10 min to isolate the serum, which was subsequently stored at −20°C until further analysis. Following this procedure, all selected hens were euthanized using carbon dioxide asphyxiation. The anterior segment of the jejunum and ileum was excised, and its contents were rinsed. The mucosal surface was scraped with a glass slide and placed into a cryopreservation tube, which was immediately frozen in liquid nitrogen and stored at −80°C. Approximately 2 cm sections from the middle of the ileum were excised and immersed in a 4% (v/v) formalin solution for histomorphometric measurement. The cecal chyme was initially stored in liquid nitrogen before being transferred to an ultra-low temperature refrigerator (−80°C) until analysis.
Production performance
The number of dead hens, feed weight, egg count, egg weight, and the quantity of unqualified eggs (including dirty, malformed, broken, soft, and sand-shelled eggs) were recorded daily. Subsequently, the hen-day egg production rate and the hen-housed egg production rate, along with the average daily feed intake (ADFI), feed conversion ratio (FCR), average egg weight, and the qualified egg rate, were calculated on a weekly basis. The following formulas were employed:
Average daily feed intake (g/bird) = total feed consumption (g)/cumulative number of laying hens on the day of feeding; hen-housed egg production rate (%) = total number of eggs/cumulative number of laying hens at the beginning day of the trial × 100; hen-day egg production rate (%) = total number of eggs/cumulative number of laying hens per week × 100; feed conversion ratio = total feed consumption/total egg weight per week; average egg weight (g) = total egg weight/total number of eggs; egg mass (g/hen) = total cumulative egg weight/number of hens at the beginning day of the trial.
Egg quality
The strength and thickness (blunt end, tip, and equator) of the eggshell were quantified using an eggshell strength tester and an eggshell thickness tester (Robotmation Co., Ltd., Tokyo, Japan). The color of the eggshell was assessed with a colorimeter (3NH-NR20XE, China). The internal quality of the egg was analyzed using an egg multi-tester (EMT-5200, Robotmation Co., Ltd., Tokyo, Japan), which provided measurements of the Haugh unit, albumen height, and yolk color. Additionally, the egg yolk was separated from the albumen using an egg separator, and the weight of the yolk was recorded. The egg yolk or eggshell index was calculated as 100 × [egg yolk or eggshell weight (g)/egg weight (g)]. The egg shape index was determined as the ratio of the longitudinal diameter to the transverse diameter.
Fatty acids of egg yolk
The freeze-dried egg yolk sample was weighed and transferred to a homogenization tube. The tube was then filled with 0.8 mL of chloroform and 0.7 mL of a methanol-water solution. The sample was homogenized for 1 min, after which the supernatant was collected. An additional 2 mL of potassium hydroxide methanol solution was added to the supernatant to initiate the saponification reaction. After cooling the sample in an ice bath, 2 mL of boron trifluoride methanol was added, and the mixture was placed in a water bath at −80°C. Heating for 2 min completed the methyl esterification process. Once the mixture cooled to room temperature, 1 mL of n-hexane (0.05 g/L BHT n-hexane solution) and 2 mL of saturated NaCl solution were added, followed by the collection of the supernatant. The fatty acids in the egg yolk were analyzed using gas chromatography (GC-2010 Plus gas chromatograph coupled to a 2010 Plus single quadrupole mass spectrometer; Shimadzu Corp., Kyoto, Japan).
Serum biochemical parameters
Alanine aminotransferase (ALT), aspartate aminotransferase (AST), urea nitrogen (UREA), total protein (TP), albumin (ALB), total cholesterol (TC), triglycerides (TG), high-density lipoprotein (HDL-C), and low-density lipoprotein (LDL-C) were measured using an automated biochemical analyzer (Hitachi 3100, China). All biochemical kits were from Nanjing Jiancheng Bioengineering Institute (Nanjing, China).
ELISA assay
The concentrations of serum diamine oxidase (DAO), D-lactic acid (D-LA), and lipopolysaccharide (LPS) were determined using an enzyme-labeled instrument (Thermo Multiskan Ascent, United States) with an enzyme immunoassay.
Approximately 0.2 g of jejunal and ileal mucosa was collected, to which physiological saline was added at a ratio of 1:4 to create a 20% tissue homogenate. This mixture was then centrifuged at 3,000 r/min for 10 min, and the supernatant was collected. The activities of trypsin and chymotrypsin, as well as the concentration of secretory immunoglobulin A (SIgA), were quantified using an enzyme-linked immunosorbent assay kit obtained from Jiangsu Enzyme Immuno-Bio Co., Ltd. The preparation and handling of the reagents were performed in strict accordance with the provided instructions. The measurement of lipase and amylase was conducted using kits supplied by the Nanjing Jiancheng Bioengineering Institute (Nanjing, China).
Intestinal morphology of ileum
The hematoxylin-eosin (H&E) staining and sealing were performed by Beijing Dacome Biotechnology Co., Ltd. Villus height (VH) and crypt depth (CD) were measured using a digital trinocular camera microscope (BA400 Digital, Japan). VH and CD were quantified in 10 villi from each slice, and the ratio of villus height to crypt depth (VH/CD) was subsequently calculated.
RNA extraction and RT-qPCR
Total RNA was extracted from the jejunum mucosa using TRIzol reagent (TaKaRa, Dalian, China), and complementary DNA (cDNA) was synthesized with the PrimeScript RT reagent kit (Takara). Real-time PCR was conducted using SYBR Premix Ex Taq (Takara) on an Applied Biosystems 7900HT Real-Time PCR system (Applied Biosystems, Foster City, CA). The primer sequences for all genes are provided in Table 2.
The RNA quality (intact ribosomal RNA 28 s/18 s) was evaluated by agarose gel electrophoresis, and RNA concentrations were subsequently quantified by spectrophotometer (NanoDrop 2000, Thermo Fisher Scientific). Complementary DNA (cDNA) was obtained by the reverse transcription process, and real-time polymerase chain reaction (PCR) was then performed in triplicate on an ABI 7500 Real-time PCR detection system (Applied Biosystems). This amplification program consisted of 95°C/15 min, followed by 40 cycles of 95°C/5 s and 60°C/30 s, and a final melting curve analysis. The β-actin was selected as the reference gene. The normalisation factor, which was subsequently used to normalise the relative amounts of RNA of interest, was obtained by calculating the geometric mean of the values of the selected reference genes.
Analysis of SCFAs in the cecal contents by gas chromatography
The cecal contents must be accurately weighed and subsequently diluted with ultrapure water at a ratio of 1:8. The resulting mixture should be thoroughly shaken and mixed, after which the pH value should be measured using a portable pH meter (Testo 205, Testo AG, Schwarzwald, Germany).
A cecal content sample weighing 0.5 g should be placed into a centrifuge tube, followed by the addition of 1.2 mL of ultrapure water. The sample must then be vortexed for 3–5 min to ensure complete dissolution. After vortexing, the sample should be allowed to stand for 30 min, after which centrifugation at 10,000 g for 15 min should be conducted in the extraction solution. The resulting supernatant (1 mL) is to be combined with 0.2 mL of a 25% (w/v) metaphosphoric acid solution and 23.3 μL of a 210 mmol/L crotonic acid solution. The samples should be mixed thoroughly and incubated at 4°C for 30 min. Following incubation, centrifugation at 8,000 g for 10 min is to be performed. After centrifugation, 0.3 mL of the supernatant should be added to 0.9 mL of chromatographic methanol and mixed well, resulting in a 1:3 dilution. The sample should then be centrifuged at 8,000 rpm for 5 min. Finally, the supernatant should be filtered through a 0.22 μm filter into a 1.5 mL EP tube for subsequent analysis.
The precise weights of the following acids must be determined: 0.91 g of acetic acid, 0.37 g of propionic acid, 0.18 g of butyric acid, and isobutyric acid, as well as 0.20 g of valeric acid and isovaleric acid. These acids must then be dissolved in pure water and diluted to a final volume of 100 mL. The resulting concentrations were measured at 151.54, 50, and 20.03, respectively. Furthermore, the concentrations were calculated to be 20.03, 19.44, and 19.44 mmol/L.
The measurement was conducted using a gas chromatograph (CP-3800, Varian, United States). The chromatographic column employed was an HP-FFAP capillary column (30 m × 0.53 mm). The column was 53 mm in diameter and 1 μm in pore size. The temperature of the syringe or detector was 220°C, the injection volume was 1 μL, and the split ratio was 50:1. The carrier gas is high-purity N2, the column flow is 1 mL/min, the FID detector temperature is 250°C, the gas high-purity H2 flow is 40 mL/min, the gas-assisted zero-stage airflow is 400 mL/min, and the makeup gas is high-purity N2 flow rate 35 mL/min. The chromatographic column is HP-FFAP, and the column oven is programmed to rise in temperature as follows: starting at a temperature of 100°C, rising to 190°C at a rate of 20°C/min, holding for 0.5 min; the running time is 5 min. The calculation formula is as follows: w = X * 4 * 1.223 (mmol/L) * Vwater * Mmolecular weight/m0.
Analysis of intestinal microflora
The magnetic bead method for genomic DNA extraction from cecal contents was employed using a kit from Tiangen Biotech (Beijing) Co., Ltd., Beijing, China. After quantifying the extracted DNA, a polymerase chain reaction (PCR) analysis was conducted on the V4 variable region of the bacterial 16S rRNA using the following primers: forward primer, 5′-CCTAYGGGRBGCASCAG-3′; reverse primer, 5′-GGACTACNNGGGTATCTAAT-3′. The obtained PCR products were separated by electrophoresis in agarose gels (2%, w/v), and the target band’s product should be recovered using a universal DNA purification and recovery kit (Tiangen Biotech (Beijing) Co., Ltd., Beijing, China). The NEB Next® Ultra™ II FS DNA PCR-free Library Prep Kit (New England Biolabs) was employed for library construction. The constructed library was quantified using Qubit and Q-PCR methods. Once qualified, the library underwent PE 250 on-machine testing on the NovaSeq 6000.
Bioinformatic analysis
Delete the offline data based on the barcode sequence and PCR amplification primer sequence. The resulting spliced sequence is referred to as RawTags data. Utilize fastp software (Version 0.23.1) to conduct rigorous filtering on the spliced raw tags to obtain high-quality tags data, known as clean tags. The Uparse algorithm (Uparse v7.0.1001) was employed to cluster all effective tags from all samples. By default, the sequences were clustered into Operational Taxonomic Units (OTUs) with 97% similarity for subsequent species annotation. Finally, alpha diversity of the microbial community was calculated using QIIME (Version 1.9.1), while beta diversity was analyzed using R software (Version 4.0.3) to compare differences among the various treatment groups.
Metabolomics analysis of cecal content
A volume of 300 μL of an 80:20 (v/v) methanol-water solution should be added to a sample of cecal content that has been previously homogenized and centrifuged at 14,000 g for 5 min. The resulting supernatant is then analyzed using liquid chromatography-mass spectrometry (LC-MS). The samples are subsequently transported to Novogene Bioinformatics Technology Co., Ltd. in Beijing, China, for further analysis.
Statistical analysis
All data were analyzed using SAS 9.4 software. Differences between two groups were assessed using an independent sample t-test. For comparisons involving more than two groups, one-way ANOVA was employed, followed by Duncan’s test for multiple comparisons. Results are presented as the mean with the standard error of the mean (SEM). p-values <0.05 were considered significant, and 0.05 ≤ p < 0.10 was considered a tendency.
Results
Production performance
Throughout the entire study period, no significant differences were observed among the treatments with dead hens (1, 0, 2, 1, and 2 hens, respectively; data not shown). As indicated in Table 3, the addition of PA did not significantly affect the hen-day egg production rate, hen-housed egg production rate, ADFI, FCR, and egg mass during weeks 1 to 12 (p > 0.05). Notably, the ADFI in the PA50 group was significantly lower than that of the PA0 group at week 12 (p < 0.05, Figure 1A). Furthermore, the average egg weights in the PA100 and PA200 groups at weeks 3, 8, 11, and 12 were significantly higher than those in the PA0 group (p < 0.05, Figure 1B). The qualified egg rates in the PA50 and PA150 groups were significantly greater than those in the PA100 and PA200 groups (p = 0.022), but with no significant difference from the PA0 group. Additionally, the large egg rate (egg weight ≥70 g) in the PA100 and PA200 groups was significantly higher than that in the PA50 and PA150 groups (data not shown).
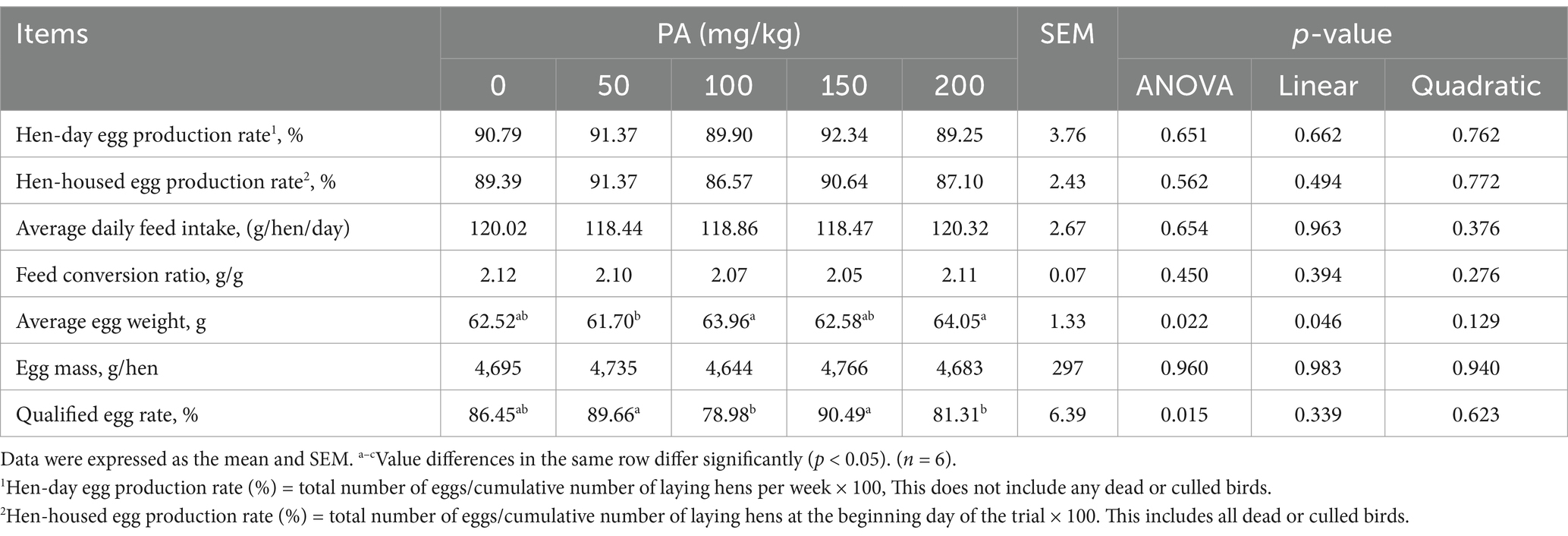
Table 3. Effects of dietary supplementation of different levels of PA on production performance of aged laying hens during 1 to 12 weeks.
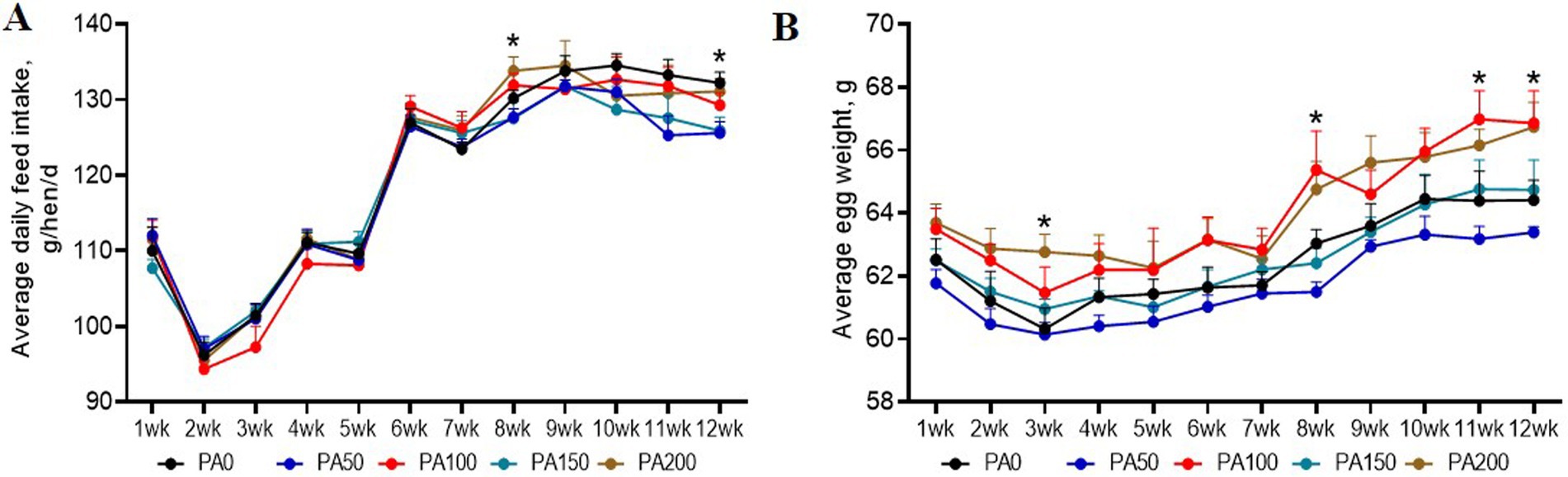
Figure 1. Effects of dietary supplementation of different levels of Pediococcus acidilactici on the production performance of aged laying hens during 1 to 12 weeks. (A) Average daily feed intake. (B) Average egg weight. Values are means and SEM, n = 6. Significant correlations are indicated by asterisks (*p < 0.05).
Egg quality and fatty acid profile of egg yolk
As shown in Table 4, the egg yolk color of the PA150 group was significantly lower than that of the PA0 group, as well as the PA50, PA100, and PA200 groups (p = 0.011).
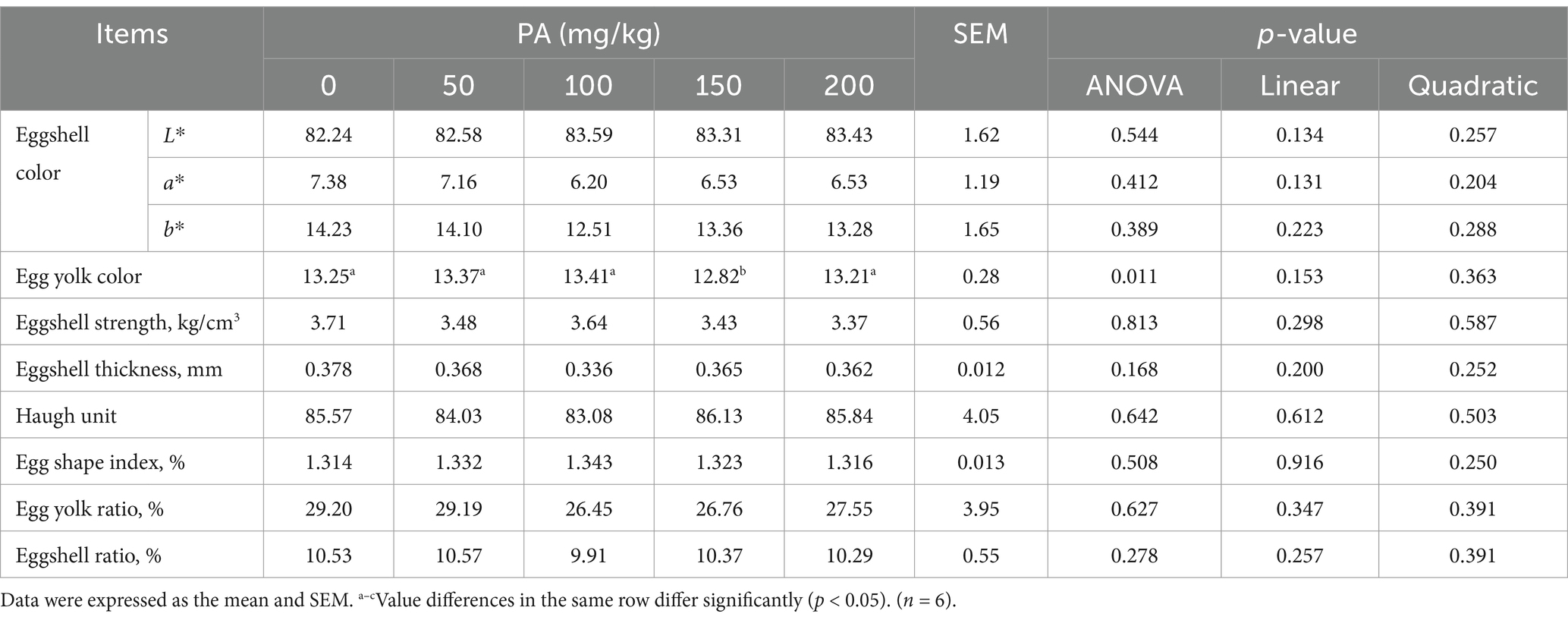
Table 4. Effects of dietary supplementation of different levels of PA on 12-week egg quality of aged laying hens.
Compared to the PA0 group, the content of C14:1 in the egg yolks of the PA150 group was significantly reduced (p = 0.013). Additionally, the content of C17:0 in the PA200 group was significantly decreased (p = 0.002), while the SFA in the PA200 group was significantly increased (p = 0.009, Table 5). The levels of C16:1 and the n−6/n−3 ratio in the egg yolk exhibited a linear relationship with the increasing PA concentration (p < 0.05). Conversely, C17:0, SFA, n−3 PUFA, and the UFA/SFA ratio demonstrated a quadratic relationship with the increasing PA concentration (p < 0.05).
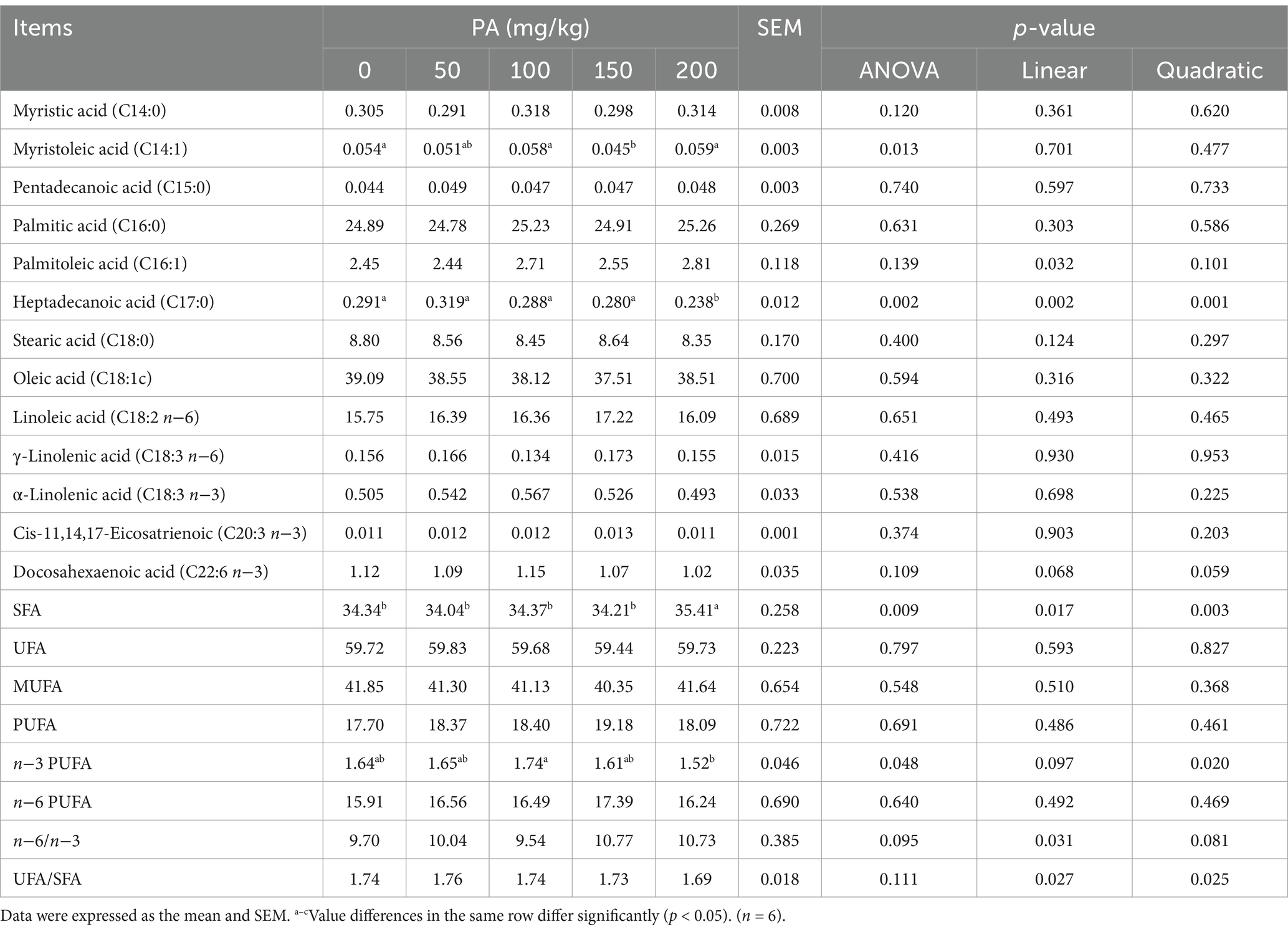
Table 5. Effects of dietary supplementation of different levels of PA on 12-week fatty acids in egg yolks of aged laying hens.
Serum indicators
Compared with the PA0 group, the serum TP, TG, and HDL-C contents of the PA100 group increased significantly (p < 0.05, Table 6). Conversely, the serum DAO levels in the PA50, PA100, PA150, and PA200 groups were significantly lower than those in the PA0 group (p < 0.05, Table 7). Additionally, the content of D-LA is the content of (p = 0.092). Notably, both TG and DAO serum levels increased with rising PA concentrations, demonstrating a quadratic curve change (p < 0.05).
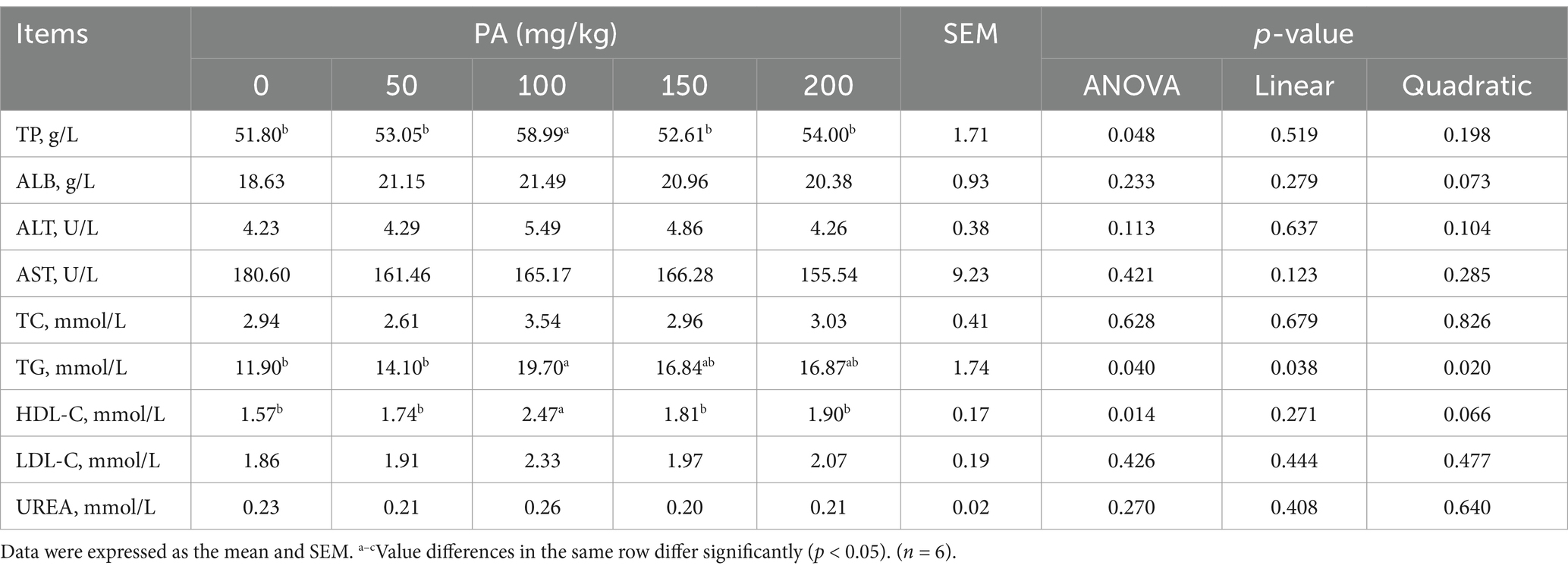
Table 6. Effects of dietary supplementation of different levels of PA on serum biochemical indicators of aged laying hens.

Table 7. Effects of dietary supplementation of different levels of PA on serum intestinal permeability indicators of aged laying hens.
Intestinal morphology and digestive enzyme activity
The ileal VH in the PA50, PA100, PA150, and PA200 groups was significantly higher than that in the PA0 group (p = 0.001). The CD of the PA100 and PA150 groups was significantly lower than that in the PA0 group (p = 0.005). Ileal VH exhibited a linear increase with rising PA concentration (p < 0.001), while CD demonstrated a quadratic change in response to increasing PA concentration (p = 0.001, Table 8). As illustrated in Figure 2B, the SIgA content in the jejunal and ileal mucosa of the PA50, PA100, PA150, and PA200 groups was lower than that in the PA0 group (p > 0.05). Compared with the PA0 group, lipase activity in the jejunum of the PA200 group was significantly increased (p = 0.036), and lipase activity in the ileum of the PA150 group was significantly increased (p = 0.012). Conversely, chymotrypsin activity in the ileum of the PA50 group was significantly decreased (p = 0.004). Both chymotrypsin activity in the ileal mucosa and lipase activity exhibited a quadratic change with increasing PA concentration (p < 0.05, Table 9).

Table 8. Effects of dietary supplementation of different levels of PA on ileum morphology of aged laying hens.
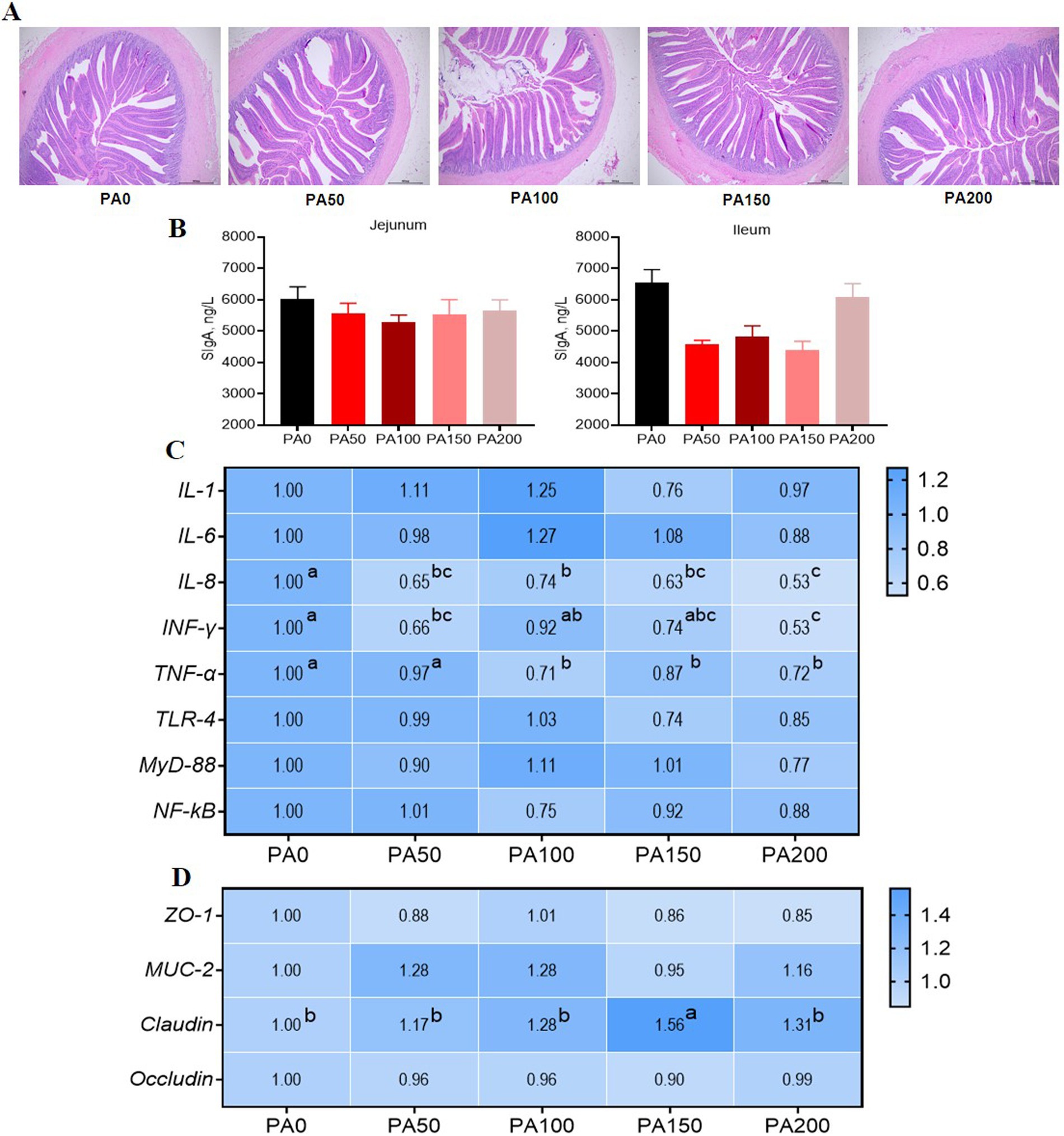
Figure 2. Effects of dietary supplementation of different levels of Pediococcus acidilactici on intestinal morphology and gene expression of aged laying hens. (A) Ileum H&E staining. (B) Intestinal mucosa secretory immunoglobulin A. (C) Jejunal mucosal inflammatory factor gene expression. (D) Jejunal mucosal barrier function gene expression. The larger the value, the bluer the color. Values are means and SEM, n = 6. Different letters indicate significant differences at p < 0.05. Significant correlations are indicated by asterisks (*p < 0.05).
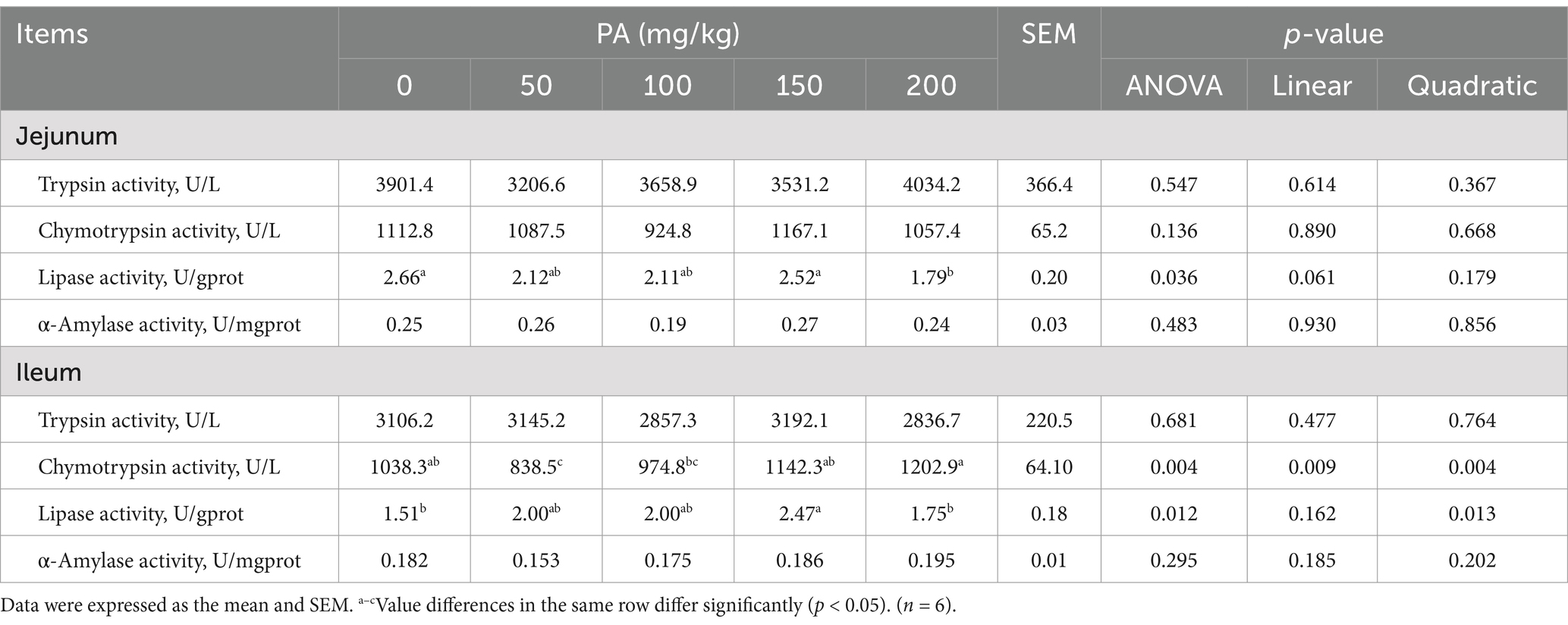
Table 9. Effects of dietary supplementation of different levels of PA on the activity of intestinal digestive enzymes in aged laying hens.
Intestinal inflammatory factors and barrier function related mRNA expression
As illustrated in Figure 2C, the expression of IL-8 mRNA in the jejunal mucosa was significantly reduced in the PA50, PA100, PA150, and PA200 groups when compared to the PA0 group (p < 0.05). Additionally, the expression of INF-γ mRNA was significantly diminished in the PA50 and PA200 groups (p < 0.05). Furthermore, the expression of TNF-α mRNA was significantly lower in the PA100, PA150, and PA200 groups (p < 0.05). Notably, the expression of Claudin mRNA in the PA150 group was significantly higher than that observed in the PA0 group (p < 0.05, Figure 2D).
Analysis of SCFAs in the cecal contents by gas chromatography
The pH value of cecal contents in the PA150 and PA200 groups was significantly lower than that observed in the PA0 group, as well as in the PA50 and PA100 groups (p < 0.05, Figure 3A). Additionally, when compared to the PA0 group, the levels of isobutyric acid and isovaleric acid were significantly elevated in the PA100 group (p < 0.05, Figure 3B).
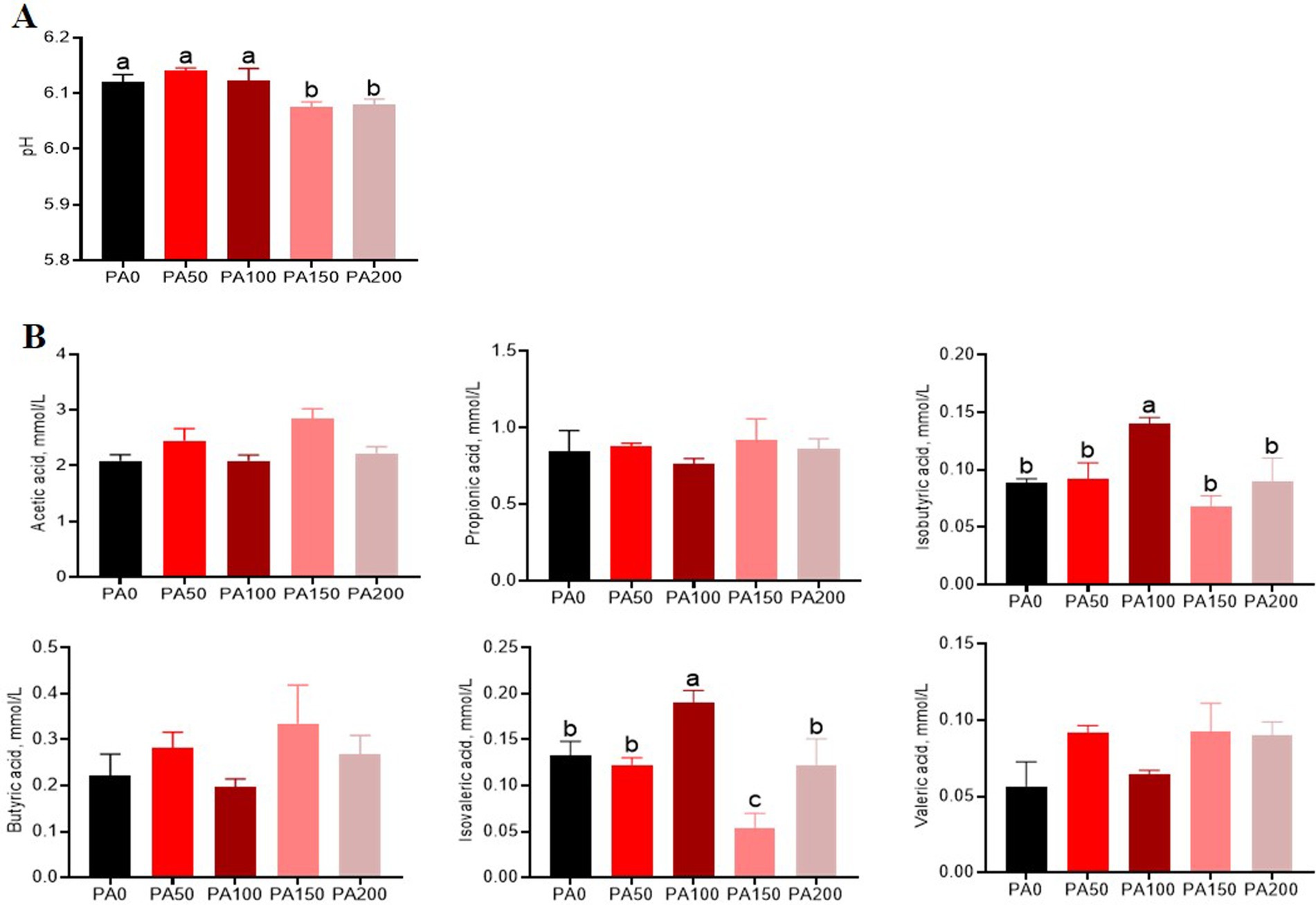
Figure 3. Effects of different concentrations of Pediococcus acidilactici on pH and volatile fatty acids in the cecal contents of aged laying hens. (A) pH value. (B) Short-chain fatty acids. Values are means and SEM, n = 6. Different letters indicate significant differences at p < 0.05.
Microbial analysis of cecal contents
The Chao1, observed species, and Ace indices of the PA100 group were significantly lower than those of the PA0 group and the PA50, PA150, and PA200 groups (p < 0.05, Figure 4A). Among a total of 1077 OTUs, 58.96% (635 core OTUs) were shared among the five groups (Figure 4B). In contrast, the relative abundance of Bacteroidota in the PA150 and PA200 groups was significantly higher than that in the PA0 group (p < 0.05, Figures 4C,E). Additionally, the relative abundance of Proteobacteria in the PA100 group was significantly elevated compared to the PA0 group as well as the PA50, PA150, and PA200 groups (p < 0.05). The relative abundance of Fusobacteriota was significantly lower in the PA100 and PA150 groups than in the PA0 group (p < 0.05, Figures 4D,F). Furthermore, the relative abundance of Euryarchaeota was significantly higher in the PA200 group compared to the PA0, PA50, PA100, and PA150 groups (p < 0.05). The relative abundance of Lactobacillus in the PA100 group was significantly greater than that in the PA0, PA50, and PA150 groups (p < 0.05). Interestingly, the relative abundances of Lactobacillus (p < 0.05), Ligilactobacillus (p > 0.05), and Limosilactobacillus (p > 0.05) were observed in the PA group. To further investigate the differences in intestinal flora structure among the various treatment groups, linear discriminant analysis effect size (LefSe) analysis was performed on the cecal contents of the hens, with results displayed in Figure 4G. The PA50 group exhibited the most significant differences in microbial composition, particularly with f_Tannerellaceae being significantly enriched. In the PA100 group, the only microorganism that showed significant differences was f_Succinivibrionaceae.
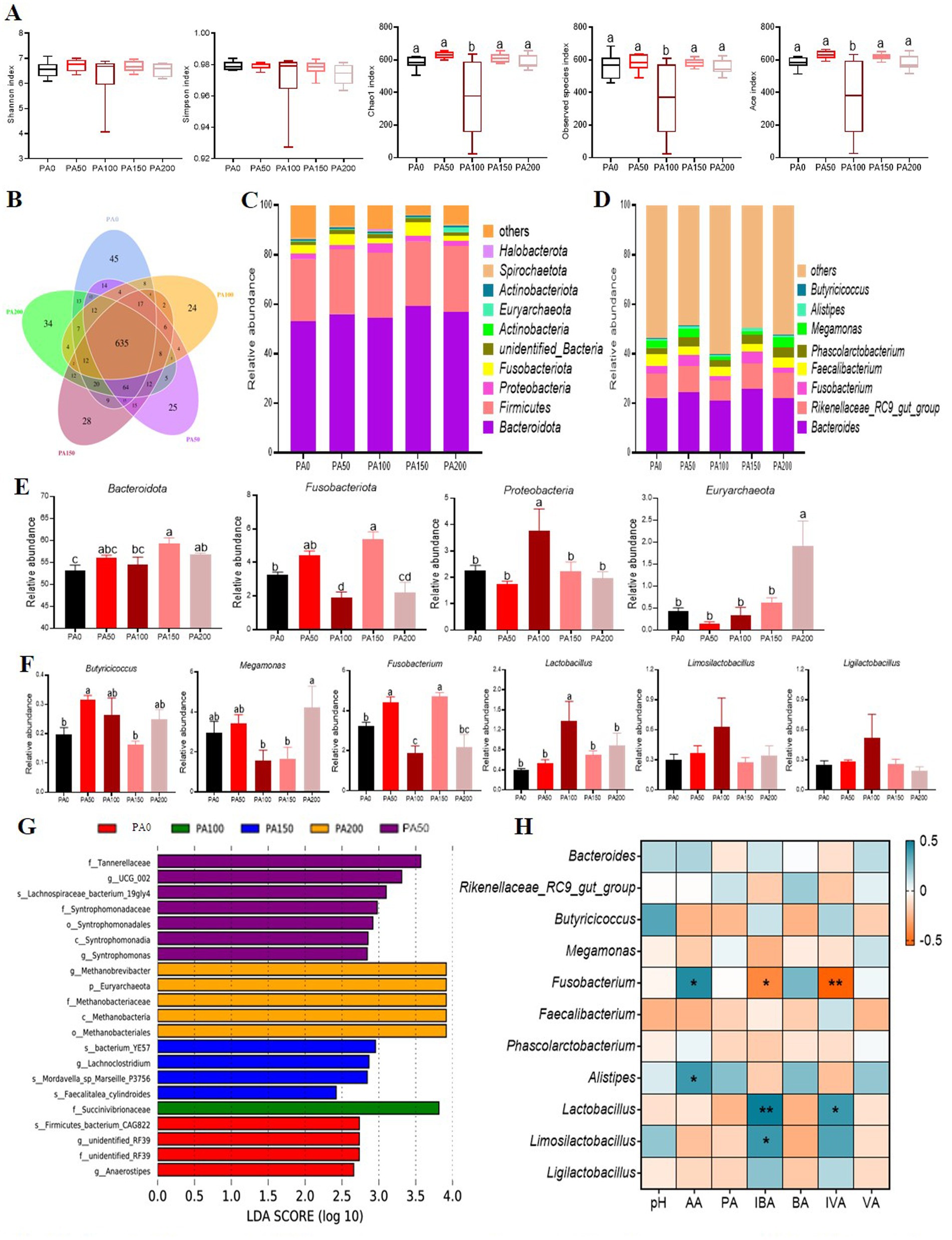
Figure 4. Effects of different concentrations of Pediococcus acidilactici on the microbial structure of the cecal contents of aged laying hens. (A) Alpha diversity index. (B) Venn diagram of operational taxonomic units (OTUS). (C,D) The cecal bacterial community compositions at phylum and genus levels. (E) Resultant composition of microorganisms in cecal contents (phylum level). (F) Resultant composition of microorganisms in cecal contents (genus level). (G) Microbial LefSe analysis of the cecum. (H) Pearson correlation coefficient analysis of intestinal microorganisms with short-chain fatty acids. Blue and yellow cells represent positive and negative correlations, respectively. AA, acetic acid; PA, propionic acid; IBA, isobutyric acid; BA, butyric acid; IVA, isovaleric acid; VA, isovaleric acid. Values are means + SEM, n = 6. Different letters indicate significant differences at p < 0.05. Significant correlations are indicated by asterisks (*p < 0.05 and **p < 0.01).
The Pearson correlation coefficient analysis of intestinal microorganisms and short-chain fatty acids reveals that acetic acid is positively correlated with Fusobacterium and Alistipes (p < 0.05, Figure 4H). Isobutyric acid shows a positive correlation with Lactobacillus (p < 0.01) and Limosilactobacillus (p < 0.05), while exhibiting a negative correlation with Fusobacterium (p < 0.05). Additionally, isovaleric acid is positively correlated with Lactobacillus (p < 0.05) and negatively correlated with Fusobacterium (p < 0.01).
Metabolomics analysis of cecal contents
The results of the partial least squares discrimination analysis indicate that each group of models is well-established. The metabolite volcano plot comparing the PA and control groups revealed a total of 91 metabolites with significant differences. Among these, 39 metabolites were found to be upregulated, while 52 were downregulated (Figure 5A). All metabolites identified in the cecal contents were matched to the KEGG database to obtain information regarding the pathways in which these metabolites participate. The arginine and proline metabolic pathways emerged as the most enriched pathways for the differential metabolites (Figure 5B). Within the arginine and proline metabolism pathways, we observed that PA significantly increased the levels of spermine and D-proline (p < 0.05). Additionally, it significantly elevated the contents of 3-methylhistidine and 5-methylcytosine (p < 0.05), while significantly decreasing the level of calcitriol (p < 0.05, Figure 5C). In the correlation analysis between metabolomics and 16S, among these differential metabolites, spermine exhibited a significant positive correlation with Alistipes (p < 0.05). Conversely, Faecalibacterium and Fusobacterium demonstrated a significant negative correlation with 5-methylcytosine (p < 0.05, Figure 5D).
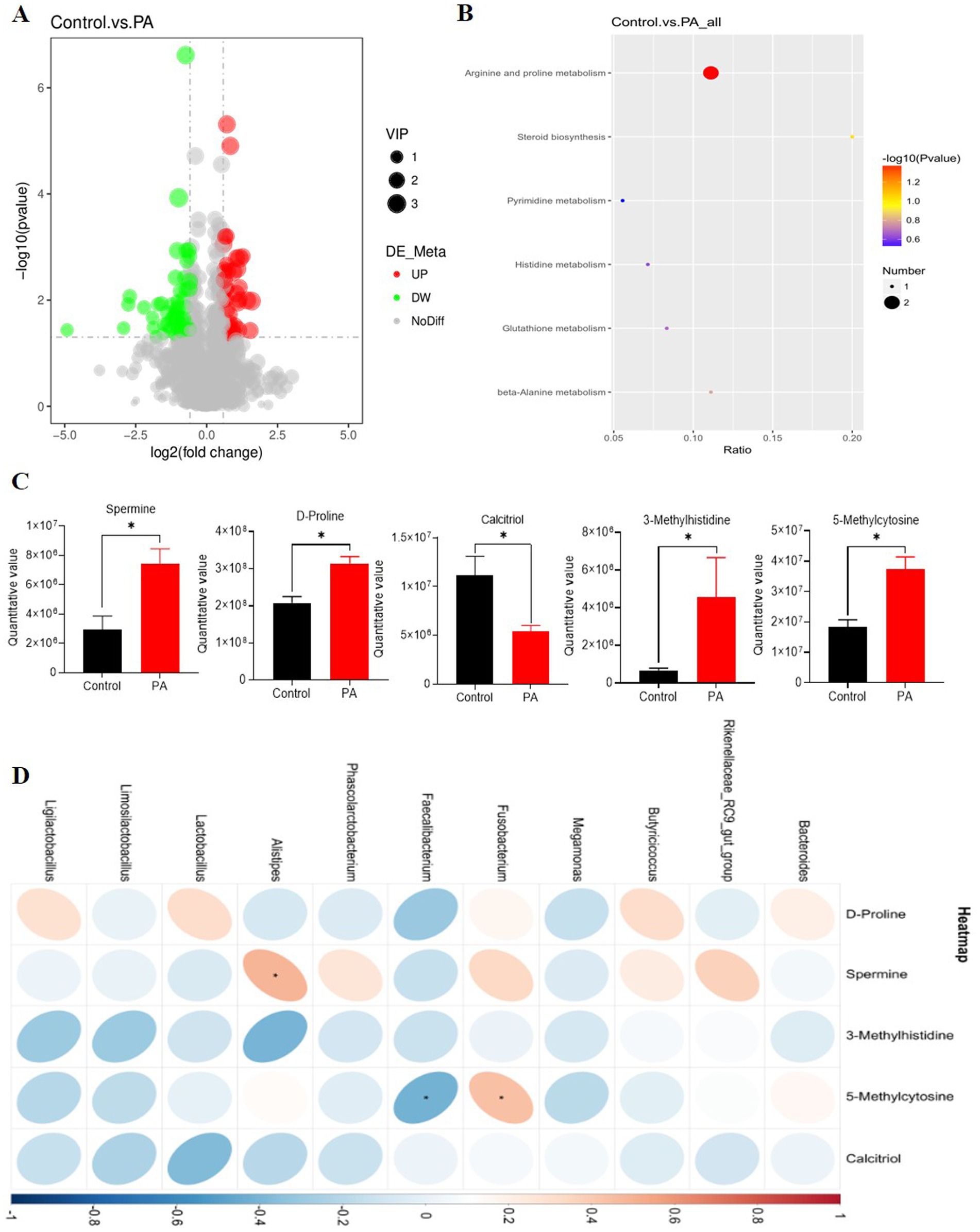
Figure 5. Effects of Pediococcus acidilactici supplementation on metabolomic profiles of cecal digesta of aged laying hens. (A) Differential metabolite volcanogram. The significantly up-regulated metabolites are represented by red dots, while down-regulated metabolites are represented by green dots. (B) Cecal chyme differential metabolites enriched by the Kyoto Encyclopedia of Genes and Genomes (KEGG). The color of the spot indicates the p-value. The redder, the more significant the enrichment. The size of the spot represented the number of different metabolites enriched. (C) Differential metabolites. *The value difference between the control group and PA group is significant (p < 0.05). (D) Correlation between differential metabolites and bacterial genera in the cecal digesta of laying hens. Red and blue cells represent positive and negative correlations, respectively. Significant correlations are indicated by asterisks (*p < 0.05). Control: no PA group; PA: combination of PA50, PA100, PA150, and PA200 groups.
Discussion
The dietary probiotics have been shown to improve both egg production and egg quality by reducing pathogenic bacterial loads and preventing damage to the gut structure (Zhang et al., 2012; Forte et al., 2016; Upadhaya et al., 2016). The results of this study suggest that incorporating PA (CNCM I-4622) into the diet of laying hens can improve egg production rates and FCR, particularly at a dosage of 150 mg/kg. Additionally, our study found that while the inclusion of 100 mg/kg and 200 mg/kg of PA increased average egg weight, it also led to a decrease in the egg production rate. A recent study corroborated that the addition of 100 mg/kg of PA significantly improved the egg production rate of laying hens (Mikulski et al., 2020). In prior research, it was observed that a diet supplemented with 100 mg/kg of PA resulted in notable increases in egg mass and average egg weight, alongside a reduction in the feed conversion ratio (Mikulski et al., 2012). Previous reports have indicated that probiotics can improve nutrient absorption by enhancing the intestinal environment of animals, thereby contributing to increased egg weight (Nahashon et al., 1994). The observed increase in egg weight was primarily attributed to a higher proportion of eggshell and greater eggshell thickness, which subsequently reduced the rate of damaged eggs (Mikulski et al., 2012; Mikulski et al., 2020). Furthermore, Mikulski et al. (2020) noted that improvements in egg weight and shell quality may be linked to enhanced calcium absorption and retention associated with probiotic supplementation.
In our study, 150 mg/kg PA significantly decreased the color of egg yolk. Previous research has indicated that feed composition and conversion rates can influence egg yolk color, with carotenoid content in eggs also playing a crucial role (Zhao et al., 2019). Consequently, the impact of probiotics on egg yolk color necessitates further investigation. Our findings demonstrate that PA alters the levels of myristoleic acid, heptadecanoic acid, SFA, and n−3 PUFA in egg yolk. An earlier study revealed that the addition of the PA strain MA18/5 M (consistent with CNCM I-4622 composition) at 100 mg/kg led to a significant decrease in palmitoleic acid content in egg yolk, accompanied by notable increases in margaric, margaroleic, and linoleic acids, as well as a substantial rise in overall PUFA and n−6 PUFA content (Mikulski et al., 2012). Saleh et al. (2016) reported that the incorporation of lactic acid bacteria in feed significantly reduced palmitoleic acid levels in egg yolks. Conversely, this approach markedly elevated the concentrations of oleic acid, linoleic acid, palmitoleic acid, gamma-linolenic acid, arachidonic acid, and nervonic acid, resulting in an overall increase in polyunsaturated fatty acids and a decrease in saturated fatty acids. Research suggests that the mechanism by which probiotics elevate unsaturated fatty acids in the intestine may be linked to amino acids and the activity of lactic acid bacteria (Saleh et al., 2013). Similar studies by Haddadin et al. (1996) and Ramasamy et al. (2008) indicated that Lactobacillus had a minimal effect on the fatty acid composition of egg yolks, which may be attributed to the specific type of probiotic used and the dosage administered (Abdelqader et al., 2013a; Mikulski et al., 2020).
Serum biochemical indicators serve as reflections of alterations in tissue cell permeability and metabolic function within the body, making them sensitive markers of animal health status (Jia et al., 2019). This study found that the addition of 100 mg/kg significantly elevated serum TP, TG, and HDL-C levels. These findings align with previous research. Specifically, studies have demonstrated that probiotics enhance serum/plasma immunoglobulin levels and induce changes in immune cell populations and their phagocytic capabilities (Peralta-Sánchez et al., 2019). HDL-C serves as an indicator of excess cholesterol being excreted into the intestines (Wang W. W. et al., 2020). Consequently, this study indicates that incorporating 100 mg/kg of PA into the diet not only increased serum TG levels but also facilitated the excretion of surplus cholesterol into the intestine, thereby regulating blood lipid metabolism. Additionally, endotoxins, commonly referred to as lipopolysaccharides, are components of the cell wall of Gram-negative bacteria (Wang et al., 2021). D-LA, a byproduct of bacterial metabolism, has been proposed as a potentially valuable marker for assessing the extent of intestinal damage and gut barrier dysfunction (Murray et al., 1993). DAO, an enzyme present in elevated concentrations in the intestinal mucosa of humans and other mammals, serves as a marker for mucosal maturation and integrity (Lei et al., 2013). Our findings indicate that the addition of PA significantly reduced serum concentrations of DAO, consistent with the results reported by Lei et al. (2013). This suggests that intestinal permeability is largely dependent on the integrity of the intestinal barrier. Intracellular DAO, which acts as a biomarker for intestinal permeability, can be released into the circulatory system in substantial quantities. When there is a breach in the integrity of the intestinal barrier, the levels of DAO in the blood increase (Wu et al., 2022). Damage to the intestinal epithelial barrier is evidenced by the presence of abnormally tight junctions and increased epithelial permeability, leading to the influx of greater quantities of DAO, D-LA, and LPS into the peripheral circulation (Deng et al., 2023).
The morphology of the small intestine and the activity of digestive enzymes are critical indicators for assessing the digestive and absorptive functions in poultry. A reduction in VH and CD may lead to impaired nutrient absorption, increased secretion within the gastrointestinal tract, and reduced performance (Xu et al., 2003). In contrast, an increase in VH and the V/C is positively correlated with enhanced epithelial cell turnover. Previous studies have shown that probiotic supplementation can stimulate the proliferation of intestinal epithelial cells in chickens (Mappley et al., 2011). Yang et al. (2020) demonstrated that the dietary inclusion of Clostridium butyricum can significantly improve the VH and V/C ratios in the duodenum, jejunum, and ileum of laying hens. The results of our study indicated that the addition of PA significantly increased the height of ileal VH in laying hens while reducing the CD. Furthermore, PA was found to significantly increase the V/C. The observed improvement in the intestinal mucosal structure in the PA treatment group may lead to improved nutrient absorption and increased disease resistance, a finding supported by measurements of digestive enzyme activity. Previous research has demonstrated that probiotics can improve dietary digestibility by producing hydrolytic enzymes that facilitate nutrient absorption, including phytase, lipase, amylase, and protease (Wang and Gu, 2010; Flint et al., 2012). The existing literature on the effects of probiotics on intestinal digestive enzymes is extensive. For instance, a study found that the administration of Clostridium butyricum to the diet of broilers infected with Escherichia coli K88 resulted in enhanced activities of amylase, protease, and lipase (Zhang et al., 2016). This finding was also corroborated by Jiang et al. (2009). Our study’s results indicate that the addition of 150 mg/kg and 200 mg/kg PA significantly increased lipase activity and chymotrypsin activity in the ileal mucosa, respectively. Ogawa et al. (2015) demonstrated that Lactobacillus aerogenes SBT2055 significantly reduced lipase activity, increased the size of lipid emulsion droplets, and inhibited lipid absorption. This finding contrasts with the results of Gong et al. (2018), who showed that the addition of probiotics to basal diets can markedly enhance amylase and lipase activities. In their studies, Rodjan et al. (2018) and Wang et al. (2021) found that the inclusion of Bacillus subtilis did not significantly affect the lipase activity in broiler chickens. This discrepancy may be attributed to the varying efficacy of different probiotic strains in enzyme production and/or the stimulation of endogenous enzyme production in broilers (Wang and Gu, 2010). Additionally, differences in pH levels within the gastrointestinal tract may also contribute to these outcomes. It is well established that the pH of the gastrointestinal tract significantly influences nutrient absorption and the gut microbiome in birds (Liu et al., 2021). The activity of various digestive enzymes is contingent upon maintaining a specific pH range, which is essential for the enzymes to perform their digestive and absorptive functions (Wang et al., 2021).
Probiotics have been shown to protect the intestinal epithelium by preventing the entry of pathogens into the gut (Vicente et al., 2008), inducing the expression of intestinal protective factors (Lutgendorff et al., 2009), strengthening tight junctions (Mennigen et al., 2009), and reinforcing the cytoskeleton of epithelial cells (Sherman et al., 2005). SIgA is the primary antibody found in intestinal secretions and is the most prevalent immunoglobulin in the mucosa. Furthermore, SIgA plays a crucial role in the formation of SIgA-coated bacterial complexes, which are essential for maintaining intestinal immune homeostasis (Macpherson et al., 2008). In our study, the addition of PA reduced the concentration of SIgA in the jejunum and ileum, thereby maintaining intestinal immune balance. Additionally, we found that PA significantly decreased the expression of pro-inflammatory factors IL-8, INF-γ, and TNF-α in the jejunal mucosa. Furthermore, 150 mg/kg also significantly elevated the expression of Claudin. Several studies have demonstrated that the consumption of probiotics can suppress the expression of pro-inflammatory cytokines while enhancing the expression of anti-inflammatory cytokines (Hu et al., 2021; Wang et al., 2021). The production of inflammatory cytokines can bolster the immune response, providing protection against pathogen invasion. However, an excessive inflammatory response may lead to tissue damage. Collectively, these findings suggest that probiotics may stimulate the immune response to protect against pathogen intrusion and thus sustain intestinal equilibrium by maintaining a delicate balance between pro-inflammatory and anti-inflammatory reactions.
SCFAs are a class of saturated fatty acids characterized by having fewer than six carbon atoms. In addition to serving as the primary energy source for colonocytes, substantial evidence suggests that SCFAs play a pivotal role in maintaining health and modulating immune and inflammatory responses (van der Hee and Wells, 2021). A study conducted by Xu et al. (2021) demonstrated that the dietary inclusion of probiotics can elevate the levels of various SCFAs in cecal contents. Consistent with this research, our study indicated that the addition of PA resulted in a significant reduction in cecal chyme pH; this observation may be attributed to the increase in SCFA concentrations and alterations in gut microbial structure. The incorporation of PA into the diet of laying hens has been shown to enhance the concentrations of acetic acid, propionic acid, butyric acid, valeric acid, and isobutyric acid in the cecal chyme. Furthermore, research indicates that the observed increase in immunoglobulins and alterations in cytokine levels may be associated with enhanced SCFA production (Parada Venegas et al., 2019; Luu et al., 2020).
The significance of the commensal gut microbiota for the normal functioning of the gut’s immune system is paramount (Neish, 2009; Adeoye et al., 2016). Numerous studies have demonstrated that probiotics can positively influence the composition of the intestinal microbiota (Kristensen et al., 2016). Specifically, probiotic supplementation has been shown to significantly alter the overall structure of the fecal bacterial community, particularly in terms of β-diversity (Kristensen et al., 2016). It is well established that the intestinal microbiota has several physiological effects on the host, including the metabolization of dietary nutrients, the production of SCFAs from indigestible carbohydrates, the synthesis of amino acids and vitamins, and the regulation of metabolism (Kamada et al., 2012). The addition of PA resulted in the Simpson index, Chao1 index, observed species index, and ACE index in cecal chyme. At the phylum level, varying concentrations of PA altered the relative abundances of Bacteroidota, Fusobacteriota, Proteobacteria, and Euryarchaeota in the cecal digesta of laying hens. Similarly, at the genus level, different concentrations of PA affected the relative abundances of Butyricicoccus, Megamonas, Fusobacterium, and Lactobacillus. Notably, PA increased the relative abundances of Fusobacteriota and Euryarchaeota at the phylum level while significantly enhancing the relative abundances of Fusobacterium, Phascolarctobacterium, and Lactobacillus at the genus level. Research indicates that Fusobacteriota and Euryarchaeota metabolize carbohydrates into SCFAs, which confer several benefits to the host, including the provision of energy to gastrointestinal cells (Wang et al., 2022; Zhu et al., 2022a). Furthermore, Bacteroidetes play a crucial role in regulating lipid and bile acid metabolism, as well as maintaining energy balance in the host (Zhu et al., 2022c). Furthermore, research has demonstrated that incorporating probiotics into the diet can significantly enhance the concentration of lactic acid bacteria (Mountzouris et al., 2010) and improve the activity of the cecal microflora (Mountzouris et al., 2007). This indicates that probiotics selectively promote specific taxa (Schaub et al., 2022). In our study, an increase in PA concentration was accompanied by a corresponding rise in the relative abundance of Lactobacillus in cecal digesta, which may also contribute to the significant decrease in pH observed. These results align with findings from Lei et al. (2015) and Yang et al. (2012). Additionally, several studies have shown that lactic acid bacteria can inhibit the colonization of Campylobacter in the digestive tract of chickens (Kobierecka et al., 2017). Consequently, PA has the potential to significantly reduce the colonization of harmful bacteria within the intestinal tract, increase the concentration of beneficial bacteria, and optimize the intestinal microenvironment of laying hens, thereby enhancing production performance.
Recent investigations have demonstrated a close relationship between the host’s gut microbiota and metabolic pathways (Wang D. et al., 2020; Zhang J. et al., 2021; Zhang X. et al., 2021). Metabolomics, a novel analytical approach, enables the detection of changes in small endogenous metabolites that may be influenced by external factors or internal disruptions. This technique can be utilized to diagnose and predict the underlying mechanisms of these alterations (Vang et al., 2022; Von-Hafe et al., 2022). Analysis of the metabolomic data reveals that the differential metabolites following the addition of PA are primarily enriched in the arginine and proline metabolism pathways. These pathways have been proposed to be closely linked to inflammation (Wijnands et al., 2015). The study indicated that several metabolites, including spermine, D-proline, 3-methylhistidine, and 5-methylcytosine, were significantly upregulated upon the addition of PA. Notably, amino acid metabolism is a critical regulator of inflammation and atherosclerosis, with arginine and proline metabolism identified as the key pathways relevant to SII and hs-CRP (Zhu et al., 2022b). Furthermore, arginine is ultimately converted into putrescine, proline, and glutamine through the action of specific enzymes. Putrescine can be transformed into spermidine and spermine, while glutamine can enter the TCA cycle and be oxidized to provide energy, generating CO2 (Zhang et al., 2022). Therefore, it is hypothesized that PA may alleviate intestinal inflammation in chickens by modulating the metabolic pathways of arginine and proline.
Conclusion
In conclusion, this study demonstrates that the inclusion of PA in the diet of the aged laying hen improves egg production by improving intestinal morphology, digestive enzyme activity, and intestinal permeability, as well as increasing SCFAs and the intestinal microbial structure in laying hens. These improvements may be linked to the metabolic pathways of arginine and proline. Ultimately, these findings contribute to a deeper understanding of the mechanisms through which dietary PA regulates both production performance and intestinal health in laying hens.
Data availability statement
The datasets generated for this study can be found in The National Center for Biotechnology Information (NCBI), (Accession No. PRJNA1211985).
Ethics statement
The animal study was approved by Institutional Animal Care and Use Committee of Sichuan Agricultural University. The study was conducted in accordance with the local legislation and institutional requirements.
Author contributions
AD: Writing – original draft, Conceptualization, Data curation, Methodology, Software, Writing – review & editing. XD: Conceptualization, Supervision, Writing – review & editing. JW: Conceptualization, Supervision, Writing – review & editing. QZ: Conceptualization, Supervision, Writing – review & editing. SB: Conceptualization, Supervision, Writing – review & editing. YL: Conceptualization, Supervision, Writing – review & editing. YX: Conceptualization, Supervision, Writing – review & editing. SL: Conceptualization, Supervision, Writing – review & editing. YM: Conceptualization, Supervision, Writing – review & editing. HP: Writing – review & editing. KZ: Conceptualization, Data curation, Funding acquisition, Investigation, Methodology, Project administration, Resources, Supervision, Writing – review & editing.
Funding
The author(s) declare that financial support was received for the research, authorship, and/or publication of this article. This research was supported by a grant from the Laying Hen Industry Technology Innovation System Construction Project, which is guided by the Central Government for Local Science and Technology Development (2022ZYDF), as well as the Natural Science Foundation of Sichuan Province (2022NSFSC0061).
Acknowledgments
We express our gratitude to all members involved in sample collection and data analysis. The authors would also like to thank Dr. Shuai Wang for his invaluable assistance with the animal experiments.
Conflict of interest
The authors declare that the research was conducted in the absence of any commercial or financial relationships that could be construed as a potential conflict of interest.
Generative AI statement
The authors declare that no Gen AI was used in the creation of this manuscript.
Publisher’s note
All claims expressed in this article are solely those of the authors and do not necessarily represent those of their affiliated organizations, or those of the publisher, the editors and the reviewers. Any product that may be evaluated in this article, or claim that may be made by its manufacturer, is not guaranteed or endorsed by the publisher.
References
Abdelqader, A., Al-Fataftah, A. R., and Daş, G. (2013a). Effects of dietary Bacillus subtilis and inulin supplementation on performance, eggshell quality, intestinal morphology and microflora composition of laying hens in the late phase of production. Anim. Feed Sci. Technol. 179, 103–111. doi: 10.1016/j.anifeedsci.2012.11.003
Abdelqader, A. R., Irshaid, R., and Al-Fataftah, A. R. (2013b). Effects of dietary probiotic inclusion on performance, eggshell quality, cecal microflora composition, and tibia traits of laying hens in the late phase of production. Trop. Anim. Health Prod. 45, 1017–1024. doi: 10.1007/s11250-012-0326-7
Abdollahi, A. A., Karimi, A. A., Sadeghi, M. R., Bedford, M. R., and Ashengroph, M. (2021). The effects of the fiber source and xylanase supplementation on production, egg quality, digestibility, and intestinal morphology in the aged laying hen. Poult. Sci. 100:100936. doi: 10.1016/j.psj.2020.12.033
Adeoye, A. A., Yomla, R., Jaramillo-Torres, A., Rodiles, A., Merrifield, D. L., and Davies, S. J. (2016). Combined effects of exogenous enzymes and probiotic on Nile tilapia (Oreochromis niloticus) growth, intestinal morphology and microbiome. Aquaculture 463, 61–70. doi: 10.1016/j.aquaculture.2016.05.028
An, R., Wilms, E., Masclee, A. A., Smidt, H., Zoetendal, E. G., and Jonkers, D. (2018). Age-dependent changes in GI physiology and microbiota: time to reconsider? Gut 67, 2213–2222. doi: 10.1136/gutjnl-2017-315542
Becattini, S., Taur, Y., and Pamer, E. G. (2016). Antibiotic-induced changes in the intestinal microbiota and disease. Trends Mol. Med. 22, 458–478. doi: 10.1016/j.molmed.2016.04.003
Cai, H., Wen, Z., Li, X., Meng, K., and Yang, P. (2020). Lactobacillus plantarum FRT10 alleviated high-fat diet-induced obesity in mice through regulating the PPARalpha signal pathway and gut microbiota. Appl. Microbiol. Biotechnol. 104, 5959–5972. doi: 10.1007/s00253-020-10620-0
Deng, C., Zhai, Y., Yang, X., Chen, Z., Li, Q., and Hao, R. (2023). Effects of grape seed procyanidins on antioxidant function, barrier function, microbial community, and metabolites of cecum in geese. Poult. Sci. 102:102878. doi: 10.1016/j.psj.2023.102878
Fan, H., and Wu, J. (2022). Conventional use and sustainable valorization of spent egg-laying hens as functional foods and biomaterials: a review. Bioresour. Bioprocess. 9:43. doi: 10.1186/s40643-022-00529-z
Feng, J., Lu, M., Wang, J., Zhang, H., Qiu, K., Qi, G., et al. (2021). Dietary oregano essential oil supplementation improves intestinal functions and alters gut microbiota in late-phase laying hens. J. Anim. Sci. Biotechnol. 12:72. doi: 10.1186/s40104-021-00600-3
Flint, H. J., Scott, K. P., Duncan, S. H., Louis, P., and Forano, E. (2012). Microbial degradation of complex carbohydrates in the gut. Gut Microbes 3, 289–306. doi: 10.4161/gmic.19897
Forte, C., Acuti, G., Manuali, E., Casagrande Proietti, P., Pavone, S., Trabalza-Marinucci, M., et al. (2016). Effects of two different probiotics on microflora, morphology, and morphometry of gut in organic laying hens. Poult. Sci. 95, 2528–2535. doi: 10.3382/ps/pew164
Fu, Z., Yang, H., Xiao, Y., Wang, X., Yang, C., Lu, L., et al. (2022). Ileal microbiota alters the immunity statues to affect body weight in muscovy ducks. Front. Immunol. 13:844102. doi: 10.3389/fimmu.2022.844102
Gan, L., Zhao, Y., Mahmood, T., and Guo, Y. (2020). Effects of dietary vitamins supplementation level on the production performance and intestinal microbiota of aged laying hens. Poult. Sci. 99, 3594–3605. doi: 10.1016/j.psj.2020.04.007
Ghasemian, M., and Jahanian, R. (2015). Dietary mannan-oligosaccharides supplementation could affect performance, immunocompetence, serum lipid metabolites, intestinal bacterial populations, and ileal nutrient digestibility in aged laying hens. Anim. Feed Sci. Technol. 213, 81–89. doi: 10.1016/j.anifeedsci.2015.12.012
Gong, L., Wang, B., Mei, X., Xu, H., Qin, Y., Li, W., et al. (2018). Effects of three probiotic Bacillus on growth performance, digestive enzyme activities, antioxidative capacity, serum immunity, and biochemical parameters in broilers. Anim. Sci. J. 89, 1561–1571. doi: 10.1111/asj.13089
Haddadin, M. S., Abdulrahim, S. M., Hashlamoun, E. A., and Robinson, R. K. (1996). The effect of Lactobacillus acidophilus on the production and chemical composition of hen’s eggs. Poult. Sci. 75, 491–494. doi: 10.3382/ps.0750491
Hu, R., Lin, H., Wang, M., Zhao, Y., Liu, H., Min, Y., et al. (2021). Lactobacillus reuteri-derived extracellular vesicles maintain intestinal immune homeostasis against lipopolysaccharide-induced inflammatory responses in broilers. J. Anim. Sci. Biotechnol. 12:25. doi: 10.1186/s40104-020-00532-4
Jiang, Y. B., Yin, Q. Q., and Yang, Y. R. (2009). Effect of soybean peptides on growth performance, intestinal structure and mucosal immunity of broilers. J. Anim. Physiol. Anim. Nutr. 93, 754–760. doi: 10.1111/j.1439-0396.2008.00864.x
Jia, Q., Zhang, L., Zhang, J., Pei, F., Zhu, S., Sun, Q., et al. (2019). Fecal microbiota of diarrhea-predominant irritable bowel syndrome patients causes hepatic inflammation of germ-free rats and berberine reverses it partially. Biomed. Res. Int. 2019:4530203. doi: 10.1155/2019/4530203
Ji, Y., Park, S., Chung, Y., Kim, B., Park, H., Huang, E., et al. (2019). Amelioration of obesity-related biomarkers by Lactobacillus sakei CJLS03 in a high-fat diet-induced obese murine model. Sci. Rep. 9:6821. doi: 10.1038/s41598-019-43092-y
Kamada, N., Kim, Y. G., Sham, H. P., Vallance, B. A., Puente, J. L., Martens, E. C., et al. (2012). Regulated virulence controls the ability of a pathogen to compete with the gut microbiota. Science 336, 1325–1329. doi: 10.1126/science.1222195
Kim, C. H., Paik, I. K., and Kil, D. Y. (2012). Effects of increasing supplementation of magnesium in diets on productive performance and eggshell quality of aged laying hens. Biol. Trace Elem. Res. 151, 38–42. doi: 10.1007/s12011-012-9537-z
Kobierecka, P. A., Wyszynska, A. K., Aleksandrzak-Piekarczyk, T., Kuczkowski, M., Tuzimek, A., Piotrowska, W., et al. (2017). In vitro characteristics of Lactobacillus spp. strains isolated from the chicken digestive tract and their role in the inhibition of Campylobacter colonization. Microbiology 6:e00512. doi: 10.1002/mbo3.512
Kristensen, N. B., Bryrup, T., Allin, K. H., Nielsen, T., Hansen, T. H., and Pedersen, O. (2016). Alterations in fecal microbiota composition by probiotic supplementation in healthy adults: a systematic review of randomized controlled trials. Genome Med. 8:52. doi: 10.1186/s13073-016-0300-5
Lee, E., Jung, S. R., Lee, S. Y., Lee, N. K., Paik, H. D., and Lim, S. I. (2018). Lactobacillus plantarum strain Ln4 attenuates diet-induced obesity, insulin resistance, and changes in hepatic mRNA levels associated with glucose and lipid metabolism. Nutrients 10:643. doi: 10.3390/nu10050643
Lei, K., Li, Y. L., Yu, D. Y., Rajput, I. R., and Li, W. F. (2013). Influence of dietary inclusion of Bacillus licheniformis on laying performance, egg quality, antioxidant enzyme activities, and intestinal barrier function of laying hens. Poult. Sci. 92, 2389–2395. doi: 10.3382/ps.2012-02686
Lei, X., Piao, X., Ru, Y., Zhang, H., Peron, A., and Zhang, H. (2015). Effect of Bacillus amyloliquefaciens-based direct-fed microbial on performance, nutrient utilization, intestinal morphology and cecal microflora in broiler chickens. Asian-Australas. J. Anim. Sci. 28, 239–246. doi: 10.5713/ajas.14.0330
Liu, X., Xing, K., Ning, R., Carne, S., Wu, X., and Nie, W. (2021). Impact of combined alpha-galactosidase and xylanase enzymes on growth performance, nutrients digestibility, chyme viscosity, and enzymes activity of broilers fed corn-soybean diets. J. Anim. Sci. 99:skab088. doi: 10.1093/jas/skab088
Liu, Z., Cao, Y., Ai, Y., Yin, X., Wang, L., Wang, M., et al. (2022). Microbiome and ileum transcriptome revealed the boosting effects of selenium yeast on egg production in aged laying hens. Anim. Nutr. 10, 124–136. doi: 10.1016/j.aninu.2022.04.001
Li, Z., Song, Q., Wang, M., Ren, J., Liu, S., and Zhao, S. (2021). Comparative genomics analysis of Pediococcus acidilactici species. J. Microbiol. 59, 573–583. doi: 10.1007/s12275-021-0618-6
Lutgendorff, F., Nijmeijer, R. M., Sandstrom, P. A., Trulsson, L. M., Magnusson, K. E., Timmerman, H. M., et al. (2009). Probiotics prevent intestinal barrier dysfunction in acute pancreatitis in rats via induction of ileal mucosal glutathione biosynthesis. PLoS One 4:e4512. doi: 10.1371/journal.pone.0004512
Luu, M., Monning, H., and Visekruna, A. (2020). Exploring the molecular mechanisms underlying the protective effects of microbial SCFAs on intestinal tolerance and food allergy. Front. Immunol. 11:1225. doi: 10.3389/fimmu.2020.01225
Macpherson, A. J., McCoy, K. D., Johansen, F.-E., and Brandtzaeg, P. (2008). The immune geography of IgA induction and function. Mucosal Immunol. 1, 11–22. doi: 10.1038/mi.2007.6
Mappley, L. J., Tchorzewska, M. A., Cooley, W. A., Woodward, M. J., and La Ragione, R. M. (2011). Lactobacilli antagonize the growth, motility, and adherence of Brachyspira pilosicoli: a potential intervention against avian intestinal spirochetosis. Appl. Environ. Microbiol. 77, 5402–5411. doi: 10.1128/AEM.00185-11
Mennigen, R., Nolte, K., Rijcken, E., Utech, M., Loeffler, B., Senninger, N., et al. (2009). Probiotic mixture VSL#3 protects the epithelial barrier by maintaining tight junction protein expression and preventing apoptosis in a murine model of colitis. Am. J. Physiol. Gastrointest. Liver Physiol. 296, G1140–G1149. doi: 10.1152/ajpgi.90534.2008
Mikulski, D., Jankowski, J., Mikulska, M., and Demey, V. (2020). Effects of dietary probiotic (Pediococcus acidilactici) supplementation on productive performance, egg quality, and body composition in laying hens fed diets varying in energy density. Poult. Sci. 99, 2275–2285. doi: 10.1016/j.psj.2019.11.046
Mikulski, D., Jankowski, J., Naczmanski, J., Mikulska, M., and Demey, V. (2012). Effects of dietary probiotic (Pediococcus acidilactici) supplementation on performance, nutrient digestibility, egg traits, egg yolk cholesterol, and fatty acid profile in laying hens. Poult. Sci. 91, 2691–2700. doi: 10.3382/ps.2012-02370
Mountzouris, K. C., Tsirtsikos, P., Kalamara, E., Nitsch, S., Schatzmayr, G., and Fegeros, K. (2007). Evaluation of the efficacy of a probiotic containing Lactobacillus, Bifidobacterium, Enterococcus, and Pediococcus strains in promoting broiler performance and modulating cecal microflora composition and metabolic activities. Poult. Sci. 86, 309–317. doi: 10.1093/ps/86.2.309
Mountzouris, K. C., Tsitrsikos, P., Palamidi, I., Arvaniti, A., Mohnl, M., Schatzmayr, G., et al. (2010). Effects of probiotic inclusion levels in broiler nutrition on growth performance, nutrient digestibility, plasma immunoglobulins, and cecal microflora composition. Poult. Sci. 89, 58–67. doi: 10.3382/ps.2009-00308
Murray, M. J., Barbose, J. J., and Cobb, C. F. (1993). Serum D (-)-lactate levels as a predictor of acute intestinal ischemia in a rat model. J. Surg. Res. 54, 507–509. doi: 10.1006/jsre.1993.1078
Nahashon, S. N., Nakaue, H. S., and Mirosh, L. W. (1994). Production variables and nutrient retention in single comb White Leghorn laying pullets fed diets supplemented with direct-fed microbials. Poult. Sci. 73, 1699–1711. doi: 10.3382/ps.0731699
National Research Council. (1994). Nutrient Requirements of Poultry, 9th Edn. Washington, DC: National Academies Press.
Neish, A. S. (2009). Microbes in gastrointestinal health and disease. Gastroenterology 136, 65–80. doi: 10.1053/j.gastro.2008.10.080
Ogawa, A., Kobayashi, T., Sakai, F., Kadooka, Y., and Kawasaki, Y. (2015). Lactobacillus gasseri SBT2055 suppresses fatty acid release through enlargement of fat emulsion size in vitro and promotes fecal fat excretion in healthy Japanese subjects. Lipids Health Dis. 14:20. doi: 10.1186/s12944-015-0019-0
Parada, J. L., Caron, C. R., Medeiros, A. B. P., and Soccol, C. R. (2007). Bacteriocins from lactic acid bacteria: purification, properties and use as biopreservatives. Braz. Arch. Biol. Technol. 50, 512–542. doi: 10.1590/S1516-89132007000300018
Parada Venegas, D., De la Fuente, M. K., Landskron, G., Gonzalez, M. J., Quera, R., Dijkstra, G., et al. (2019). Short chain fatty acids (SCFAs)-mediated gut epithelial and immune regulation and its relevance for inflammatory bowel diseases. Front. Immunol. 10:277. doi: 10.3389/fimmu.2019.00277
Peralta-Sánchez, J. M., Martín-Platero, A. M., Ariza-Romero, J. J., Rabelo-Ruiz, M., Zurita-González, M. J., Baños, A., et al. (2019). Egg production in poultry farming is improved by probiotic bacteria. Front. Microbiol. 10:1042. doi: 10.3389/fmicb.2019.01042
Qiao, Y., Qiu, Z., Tian, F., Yu, L., Zhao, J., Zhang, H., et al. (2021). Pediococcus acidilactici strains improve constipation symptoms and regulate intestinal flora in mice. Front. Cell Infect. Microbiol. 11:655258. doi: 10.3389/fcimb.2021.655258
Quarantelli, A., Righi, F., Agazzi, A., Invernizzi, G., Ferroni, M., and Chevaux, E. (2008). Effects of the administration of Pediococcus acidilactici to laying hens on productive performance. Vet. Res. Commun. 32, S359–S361. doi: 10.1007/s11259-008-9148-5
Ramasamy, K., Abdullah, N., Jalaludin, S., Wong, M., and Ho, Y. W. (2008). Effects of Lactobacillus cultures on performance of laying hens, and total cholesterol, lipid and fatty acid composition of egg yolk. J. Sci. Food Agric. 89, 482–486. doi: 10.1002/jsfa.3477
Rodjan, P., Soisuwan, K., Thongprajukaew, K., Theapparat, Y., Khongthong, S., Jeenkeawpieam, J., et al. (2018). Effect of organic acids or probiotics alone or in combination on growth performance, nutrient digestibility, enzyme activities, intestinal morphology and gut microflora in broiler chickens. J. Anim. Physiol. Anim. Nutr. 102, e931–e940. doi: 10.1111/jpn.12858
Saleh, A. A., Gálik, B., Arpášová, H., Capcarová, M., Kalafová, A., Šimko, M., et al. (2016). Synergistic effect of feeding Aspergillus awamori and lactic acid bacteria on performance, egg traits, egg yolk cholesterol and fatty acid profile in laying hens. Ital. J. Anim. Sci. 16, 132–139. doi: 10.1080/1828051X.2016.1269300
Saleh, A. A., Hayashi, K., and Ohtsuka, A. (2013). Synergistic effect of feeding Aspergillus awamori and Saccharomyces cerevisiae on growth performance in broiler chickens; promotion of protein metabolism and modification of fatty acid profile in the muscle. J. Poult. Sci. 50, 242–250. doi: 10.2141/jpsa.0120153
Schaub, A. C., Schneider, E., Vazquez-Castellanos, J. F., Schweinfurth, N., Kettelhack, C., Doll, J. P., et al. (2022). Clinical, gut microbial and neural effects of a probiotic add-on therapy in depressed patients: a randomized controlled trial. Transl. Psychiatry 12:227. doi: 10.1038/s41398-022-01977-z
Shanmugam, S., Barbe, F., Park, J. H., Chevaux, E., and Kim, I. H. (2024). Supplemental effect of Pediococcus acidilactici CNCM I-4622 probiotic on the laying characteristics and calcium and phosphorus metabolism in laying hens. Sci. Rep. 14:12489. doi: 10.1038/s41598-024-62779-5
Sherman, P. M., Johnson-Henry, K. C., Yeung, H. P., Ngo, P. S., Goulet, J., and Tompkins, T. A. (2005). Probiotics reduce enterohemorrhagic Escherichia coli O157:H7- and enteropathogenic E. coli O127:H6-induced changes in polarized T84 epithelial cell monolayers by reducing bacterial adhesion and cytoskeletal rearrangements. Infect. Immun. 73, 5183–5188. doi: 10.1128/IAI.73.8.5183-5188.2005
Upadhaya, S. D., Hossiendoust, A., and Kim, I. H. (2016). Probiotics in Salmonella-challenged Hy-Line brown layers. Poult. Sci. 95, 1894–1897. doi: 10.3382/ps/pew106
van der Hee, B., and Wells, J. M. (2021). Microbial regulation of host physiology by short-chain fatty acids. Trends Microbiol. 29, 700–712. doi: 10.1016/j.tim.2021.02.001
Vang, S., Cochran, P., Sebastian Domingo, J., Krick, S., and Barnes, J. W. (2022). The glycobiology of pulmonary arterial hypertension. Metabolites 12:316. doi: 10.3390/metabo12040316
Vicente, J. L., Torres-Rodriguez, A., Higgins, S. E., Pixley, C., Tellez, G., Donoghue, A. M., et al. (2008). Effect of a selected Lactobacillus spp.-based probiotic on Salmonella enterica serovar enteritidis-infected broiler chicks. Avian Dis. 52, 143–146. doi: 10.1637/7847-011107-ResNote
Von-Hafe, M., Borges-Canha, M., Vale, C., Leite, A. R., Sergio Neves, J., Carvalho, D., et al. (2022). Nonalcoholic fatty liver disease and endocrine axes—a scoping review. Metabolites 12:298. doi: 10.3390/metabo12040298
Wang, D., Guo, S., He, H., Gong, L., and Cui, H. (2020). Gut microbiome and serum metabolome analyses identify unsaturated fatty acids and butanoate metabolism induced by gut microbiota in patients with chronic spontaneous urticaria. Front. Cell. Infect. Microbiol. 10:24. doi: 10.3389/fcimb.2020.00024
Wang, W. W., Wang, J., Zhang, H. J., Wu, S. G., and Qi, G. H. (2020). Supplemental Clostridium butyricum modulates lipid metabolism through shaping gut microbiota and bile acid profile of aged laying hens. Front. Microbiol. 11:600. doi: 10.3389/fmicb.2020.00600
Wang, X., Wu, X., Shang, Y., Gao, Y., Li, Y., Wei, Q., et al. (2022). High-altitude drives the convergent evolution of alpha diversity and indicator microbiota in the gut microbiomes of ungulates. Front. Microbiol. 13:953234. doi: 10.3389/fmicb.2022.953234
Wang, Y., and Gu, Q. (2010). Effect of probiotic on growth performance and digestive enzyme activity of Arbor Acres broilers. Res. Vet. Sci. 89, 163–167. doi: 10.1016/j.rvsc.2010.03.009
Wang, Y., Heng, C., Zhou, X., Cao, G., Jiang, L., Wang, J., et al. (2021). Supplemental Bacillus subtilis DSM 29784 and enzymes, alone or in combination, as alternatives for antibiotics to improve growth performance, digestive enzyme activity, anti-oxidative status, immune response and the intestinal barrier of broiler chickens. Br. J. Nutr. 125, 494–507. doi: 10.1017/S0007114520002755
Wijnands, K. A., Castermans, T. M., Hommen, M. P., Meesters, D. M., and Poeze, M. (2015). Arginine and citrulline and the immune response in sepsis. Nutrients 7, 1426–1463. doi: 10.3390/nu7031426
Wu, Y., Wang, W., Kim, I. H., and Yang, Y. (2022). Dietary hydrolyzed wheat gluten supplementation ameliorated intestinal barrier dysfunctions of broilers challenged with Escherichia coli O78. Poult. Sci. 101:101615. doi: 10.1016/j.psj.2021.101615
Xu, Y., Yu, Y., Shen, Y., Li, Q., Lan, J., Wu, Y., et al. (2021). Effects of Bacillus subtilis and Bacillus licheniformis on growth performance, immunity, short chain fatty acid production, antioxidant capacity, and cecal microflora in broilers. Poult. Sci. 100:101358. doi: 10.1016/j.psj.2021.101358
Xu, Z. R., Hu, C. H., Xia, M. S., Zhan, X. A., and Wang, M. Q. (2003). Effects of dietary fructooligosaccharide on digestive enzyme activities, intestinal microflora and morphology of male broilers. Poult. Sci. 82, 1030–1036. doi: 10.1093/ps/82.6.1030
Yang, C. M., Cao, G. T., Ferket, P. R., Liu, T. T., Zhou, L., Zhang, L., et al. (2012). Effects of probiotic, Clostridium butyricum, on growth performance, immune function, and cecal microflora in broiler chickens. Poult. Sci. 91, 2121–2129. doi: 10.3382/ps.2011-02131
Yang, J., Zhan, K., and Zhang, M. (2020). Effects of the use of a combination of two Bacillus species on performance, egg quality, small intestinal mucosal morphology, and cecal microbiota profile in aging laying hens. Probiotics Antimicrob. Proteins 12, 204–213. doi: 10.1007/s12602-019-09532-x
Zhang, J., Feng, D., Law, H. K., Wu, Y., Zhu, G. H., Huang, W. Y., et al. (2021). Integrative analysis of gut microbiota and fecal metabolites in rats after prednisone treatment. Microbiol. Spectr. 9:e006502. doi: 10.1128/Spectrum.00650-21
Zhang, J. L., Xie, Q. M., Ji, J., Yang, W. H., Wu, Y. B., Li, C., et al. (2012). Different combinations of probiotics improve the production performance, egg quality, and immune response of layer hens. Poult. Sci. 91, 2755–2760. doi: 10.3382/ps.2012-02339
Zhang, L., Zhang, L., Zhan, X., Zeng, X., Zhou, L., Cao, G., et al. (2016). Effects of dietary supplementation of probiotic, Clostridium butyricum, on growth performance, immune response, intestinal barrier function, and digestive enzyme activity in broiler chickens challenged with Escherichia coli K88. J. Anim. Sci. Biotechnol. 7:3. doi: 10.1186/s40104-016-0061-4
Zhang, X., Coker, O. O., Chu, E. S., Fu, K., Lau, H. C. H., Wang, Y. X., et al. (2021). Dietary cholesterol drives fatty liver-associated liver cancer by modulating gut microbiota and metabolites. Gut 70, 761–774. doi: 10.1136/gutjnl-2019-319664
Zhang, Y., Huang, Y., Chen, R., Chen, S., and Lü, X. (2022). The interaction mechanism of nickel ions with L929 cells based on integrative analysis of proteomics and metabolomics data. Regen. Biomater. 9. doi: 10.1093/rb/rbac040
Zhao, M. J., Cai, H. Y., Liu, M. Y., Deng, L. L., Li, Y., Zhang, H., et al. (2019). Effects of dietary glycerol monolaurate on productive performance, egg quality, serum biochemical indices, and intestinal morphology of laying hens. J. Zhejiang. UNIV-SC. B. 20, 877–890. doi: 10.1631/jzus.B1800530
Zhu, S., Han, M., Liu, S., Fan, L., Shi, H., and Li, P. (2022c). Composition and diverse differences of intestinal microbiota in ulcerative colitis patients. Front. Cell Infect. MI. 12, 953962. doi: 10.3389/fcimb.2022.953962
Zhu, D. H., Nie, F. H., Zhang, M., Wei, W., Song, Q. L., Hu, Y., et al. (2022a). Effects of Aroclor 1254 on intestinal immunity, metabolism, and microflora in zebrafish. Front. Nutr. 9, 929925 doi: 10.3389/fnut.2022.929925
Keywords: Pediococcus acidilactici, egg production, gut microbiota, aged laying hens, metabolome
Citation: Dong A, Ding X, Wang J, Zeng Q, Bai S, Liu Y, Xuan Y, Li S, Mu Y, Peng H and Zhang K (2025) Dietary Pediococcus acidilactici improves the intestinal functions by regulating the expression of intestinal inflammatory genes and intestinal microbiota in aged laying hens at 80–91 weeks of age. Front. Microbiol. 16:1530319. doi: 10.3389/fmicb.2025.1530319
Edited by:
Wen-Chao Liu, Guangdong Ocean University, ChinaReviewed by:
Hongbin Pan, Yunnan Agricultural University, ChinaShimeng Huang, China Agricultural University, China
Copyright © 2025 Dong, Ding, Wang, Zeng, Bai, Liu, Xuan, Li, Mu, Peng and Zhang. This is an open-access article distributed under the terms of the Creative Commons Attribution License (CC BY). The use, distribution or reproduction in other forums is permitted, provided the original author(s) and the copyright owner(s) are credited and that the original publication in this journal is cited, in accordance with accepted academic practice. No use, distribution or reproduction is permitted which does not comply with these terms.
*Correspondence: Keying Zhang, emtleWluZ0BzaWNhdS5lZHUuY24=