- 1Postdoctoral Research Station, Guangzhou Bay Area Institute of Biomedicine, Guangzhou, China
- 2School of Basic Medical Sciences, Southwest Medical University, Luzhou, China
- 3Sichuan Provincial Key Laboratory for Human Disease Gene Study and Department of Laboratory Medicine, Sichuan Provincial People’s Hospital, University of Electronic Science and Technology of China, Chengdu, China
- 4Department of Dermatology, Southwest Hospital, Third Military Medical University (Army Medical University), Chongqing, China
- 5State Key Laboratory of Ultrasound in Medicine and Engineering, College of Biomedical Engineering, Chongqing Medical University, Chongqing, China
Currently, multidrug-resistant (MDR) bacterial wound infections (WIs) are an extremely challenging clinical problem for physicians. Recently, compared to traditional single liquid delivery drugs, the study of five novel drug delivery systems (i.e., hydrogel, liposomes, electrospun fibers, nanoparticles and nanoemulsion) for phages and their encoded lysins in WI management has become a hot topic. To assess the current landscape of these emerging technologies, we conducted a comprehensive literature search across PubMed, Scopus and Web of Science up to July 2024, using terms such as “phage,” “lysin,” “wound,” “hydrogel,” “liposomes,” “fibers,” “nanoparticles,” and “nanoemulsion.” The criteria included original studies of five novel delivery systems for phages and lysins in WI management. The findings highlighted the positive effects of the five novel delivery systems for phages and lysins in WI management, significantly reducing wound bacterial populations, and accelerating healing at the injury site. However, the available literature on novel delivery systems for phages and lysins remains limited, particularly for lysins. In conclusion, the application of novel drug delivery systems for phages and lysins showed great potential in combating MDR bacterial WIs.
1 Introduction
Currently, the treatment of multidrug-resistant (MDR) bacterial wound infections (WIs) faces significant challenges. The American Academy of Dermatology reported that the total number of patients seeking treatment for burns, ulcers, wounds and skin infections in the U.S. exceeded 50 million in 2013, and the total cost of treatment was approximately $19.9 billion (American Academy of Dermatology Association, 2018). With the considerable overuse of antibiotics to treat WIs, the emergence of MDR bacterial WIs has become a headache for clinicians and the public health system. Due to the tightening of financial strategies of global pharmaceutical companies and slow progress in antibiotic development, the search for alternatives to antibiotics to treat MDR bacteria has become particularly urgent (Leptihn, 2019; Manohar et al., 2020).
In recent years, phage therapy and phage-encoded lysins as new antimicrobial agents provide the revitalied weapons to address the antibiotic resistance dilemma (Dedrick et al., 2019; Sweere et al., 2019; Rahman et al., 2021; Feng et al., 2024). Phages use bacteria as parasitic hosts, proliferate inside the host bacteria and release them outside the host bacteria, resulting in bacterial lysis and death. Generally speaking, the phage life cycle is as follows (Briot et al., 2022; Liang et al., 2023): (1) Adsorption: the phage achieves tight adsorption to the host bacteria through the specific binding of its tail fibers to the surface of the host bacteria. (2) Invasion: after tightly adhering to the host bacteria, the phage injects its genes into the host bacteria. (3) Proliferation: the phage uses structural components of the host bacteria to synthesize progeny phage proteins and nucleic acids. (4) Assembly: the synthesized phage components are assembled together according to a certain procedure. (5) Release: upon completion of assembly, the phage cleaves the cell wall through its secreted lytic enzyme, and then releases it into the extracellular space of the host bacteria. Through the repeated cycles of the above steps, the phage kills a large number of host bacteria, thus exerting its powerful bactericidal effect. Therefore, microbiologists and clinicians often isolate and screen phages from natural environments to produce phage preparations for antimicrobial treatments known as phage therapy. In fact, phages rely mainly on their derived enzymes to exert their antibacterial properties. As one of the most important phage-derived enzymes, endolysin kills bacteria by degrading the peptidoglycan structure of the bacterial cell wall during phage-mediated internal lysis –“endo” meaning “inside”-which occurs at the end of the phage infection cycle (Rahman et al., 2021; Chang et al., 2022). Based on the above description, attention in this review is therefore focussed on the research of endolysins (lysins). Although phages are obtained from natural rapid isolation, phage-derived lsyins need to be produced by recombination in a bacterial or yeast expression system. With the rapid development of genetic engineering, large quantities of purified phage-derived lysins can be readily obtained, thus opening up the possibility of large-scale clinical therapy.
The research of phage therapy as an alternative to conventional antibiotics in the treatment of MDR-refractory WIs such as dermatoses, burns and chronic wound/ulcer infections is again of great interest to traumatologists worldwide (Ding et al., 2022; Wang et al., 2024). Early phage therapy for the treatment of superficial bacterial infections, particularly dermatological diseases, burns and chronic wound/ulcer infections, attracted considerable attention. Despite the excellent antibacterial efficacy of phage therapy, phage therapy only has antibacterial properties against a single pathogenic bacteria, which is one of the reasons why phage therapy was difficult to be applied on a wide clinical scale at that time. Soon after, with the discovery of broad-spectrum antibiotic penicillin, phage therapy came to an abrupt end in most Western countries. Surprisingly, phage therapy is still in use at institutions such as Hirszfeld (Wrocław, Poland) and Eliava (Tbilisi, Georgia). Nowadays, phages or their derived lysins modified by genetic engineering and in vivo recombinant engineering can remove unwanted properties, thus potentially altering specificity or improving therapeutic potential. Peng et al. (2022) replaced the receptor-binding structural domain of phage M13 (the N-terminal structural domain of g3p) with the corresponding structural domain of phage Pf1, which enabled the chimeric M13-g3p (Pf1) to (Pf1) to attach to Pseudomonas aeruginosa (P. aeruginosa) via type IV pili. Moreover, there has been an increasing interest in improved lysins with new properties. Modified lysins are formed by binding of natural lysin fragments to other proteins or by rearrangement of the catalytic N-terminal structural domain and cell wall binding structural domain. The fusion of an outer membrane permeable peptide or cationic peptide with phage lysins can sensitize Gram-negetive (G–) bacteria to its lysogenic effect or enhance the bactericidal effect against Streptococcus spp. (Briers et al., 2014a; Briers et al., 2014b; Rodriguez-Rubio et al., 2016). Yang et al. (2015) constructed a novel modified lysin, ClyR, by fusing the CHAP structural domain of the PlyC lysin to the CBD of the PlySs2 lysin. It has potent bactericidal activity and a broad streptococcal host range, including a wide variety of streptococci as well as representative enterococci and staphylococci (including MRSA and VRSA). Based on the above, phage and lysin therapies for WIs caused by MDR bacteria are once again showing a very tempting prospect, along with the continuous modification of phages and lysins and the emergence of MDR bacteria (De Maesschalck et al., 2020; Prokopczuk et al., 2023; Karn et al., 2024).
Hitherto, the U.S. Food and Drug Administration has granted consecutive approvals for the phage cocktail Sb-1, and the phage-derived lysins N-Rephasin® SAL200, and CF-301 for use in clinical emergencies and special situations. Staphefekt SA.100 is designated as a Class 1 medical device in Europe for the treatment of superficial skin lesions infected with Staphylococcus aureus (S. aureus) (de Wit et al., 2019). The best route of administration for the phage and its encoded lysin for the treatment of WIs is likely to be topical to ensure that the drug is delivered directly to the infected wound site, thus avoiding its destruction by the host immune and digestive systems. Topical administration is a safer, more cost-effective and convenient route of administration compared to intravenous and oral administrations. Table 1 summarizes the current clinical trial landscape of phage and lysin formulations for the treatment of WIs. It can be seen that liquid or creams is the main delivery mode of phage and lysin formulations for the treatment of WIs. Liquid phage preparations are usually formulated in sterile buffer solutions such as phosphate buffered saline (PBS) or Tris-buffered saline-magnesium buffer (SMB) (Chang et al., 2020). As a result, liquid phage formulations are simple to prepare and relatively minimal formulation development is required for phage stability (Chang et al., 2020). Liquid preparations are usually impregnated in dressings, gauze and bandages for covering wounds (Pinto et al., 2020; Steele et al., 2020). However, phages may become stuck in the gauze, hindering phage release and subsequent bacteriolysis. In an impressive PhagoBurn study, jointly organized by France, Belgium and Switzerland, the efficacy of phage cocktail (PP1131) in the treatment of burn wounds failed to meet expectations, mainly due to the rapid decrease in the total titre of the phage cocktail to 104–105 PFU/mL over a period of 6 months (Jault et al., 2019). The instability of PP1131 may be due to electrostatic interactions, adsorption on the surface of the storage container, oxidation, or water chemistry interference (Jault et al., 2019; Mutti and Corsini, 2019; Chang et al., 2020). In addition, thicker phage cream preparations may have added ingredients that can limit phage movement, but the presence of preservatives in creams, especially those with an acidic pH, can negatively affect phage effectiveness (Merabishvili et al., 2017; Pinto et al., 2020). Cream delivery of lysins also does not perfectly improve the survival of lysins at pH values close to 5 and is thermally triggered at temperatures similar to those of infected wounds (Pinto et al., 2021). This makes it difficult to obtain satisfactory stability, long shelf-life and definitive therapeutic effect of lysin cream formulations (Pinto et al., 2021). To date, a number of novel delivery systems (e.g., hydrogel, liposomes, electrospun fibers, nanoparticles and nanoemulsions) for the delivery of phages and lysins have received more and more keen attention from scholars, as illustrated in Figure 1 (Chang et al., 2020; Pinto et al., 2020; Pinto et al., 2021; Briot et al., 2022). As far as the currently available data are concerned, there has not yet been a comprehensive and detailed review on the use of these novel delivery systems for phages and lysins in the topical management of WIs. Here, we will focus on an overview of the literature on these five novel drug delivery systems for the treatment of WIs, with a view to providing important implications for researchers working in this field.
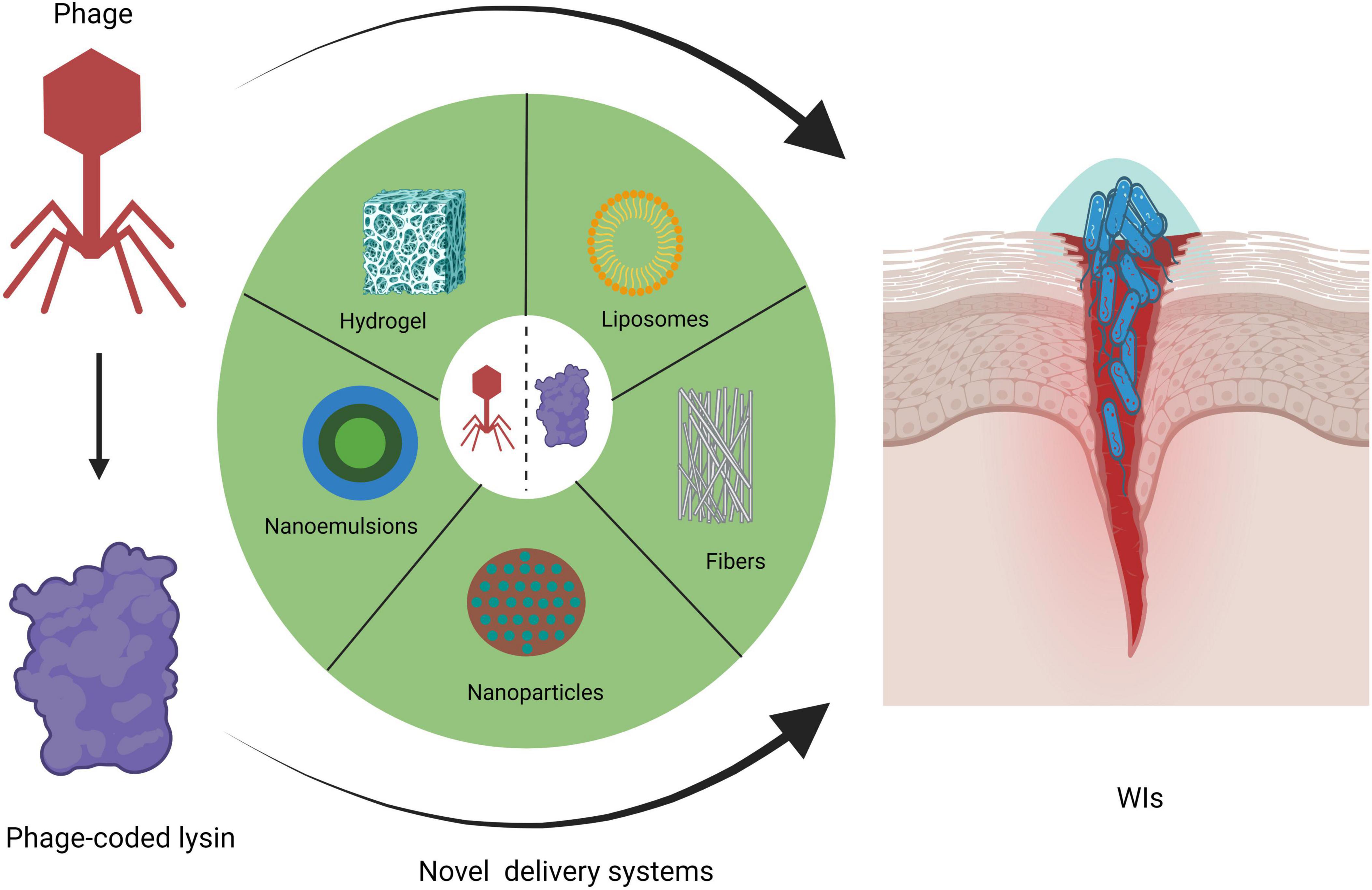
Figure 1. Novel delivery systems for phages and lysins in topical WI management (created by BioRender.com, 2024).
2 Methods
2.1 Search strategy
Three electronic databases were searched, without limits, for articles published up to 25th July 2024: the PubMed, Scopus and Web of Science databases. The search was performed using the following terms: “phage” AND “hydrogel” OR “liposomes” OR “fibers” OR “nanoparticles” OR “nanoemulsion” AND “wound” or “lysin” AND “hydrogel” OR “liposomes” OR “fibers” OR “nanoparticles” OR “nanoemulsion” AND “wound.”
2.2 Study inclusion and exclusion criteria
The selection of studies for inclusion was limited to original research, particularly in vitro and in vivo animal experimental studies on infected wounds. The exclusion criteria were strictly defined to ensure relevance and quality; we omitted reviews, short communication, encyclopedias, editorials, conference information, conference abstracts and book chapters. The first step in the research methodology was to identify and exclude duplicate study using Endnote (version X8) from the database to avoid redundancy. Moreover, additional literature was included to enrich the manuscript and deepen the thematic structure of the article during the manuscript writing process. Title and abstract screening was performed independently by two authors (PY, Xiumei Ma), with discrepancies resolved by a third author (NH). Full-text screening was performed independently by two authors (PY, Xiumei Ma), with discrepancies resolved by a third author (NH).
2.3 Data extraction
The following information was extracted from each study: authors; date of publication; location of the study; type of wound; synthetic material for the novel delivery system; causative organisms; phage and lysin names and their dosages; type of study design; therapeutic efficacy (including change in bacterial counts in wounds, survival in mice, wound contraction, wound tissue healing, and change in inflammation in wound tissue). Comments or data on the safety, stability, and adverse effects of the formulations were also collected. Data were extracted independently by two authors (PY, Xiumei Ma), and inconsistencies were resolved by a third author (NH). This review was conducted in accordance with the PRISMA (Preferred Reporting Items for Systematic Reviews and Meta-Analyses) guidelines (Stroup et al., 2000).
3 Results
We conducted a relative comprehensive literature search of PubMed, Scopus and Web of Science, retrieving in total 4,180 articles, distributed as follows: 95 from PubMed, 4,047 from Scopus and 38 from Web of Science. Our methodologically rigorous and comprehensive screening process, as shown in Figure 2, provides the reader with a clear overview of the methodology and results. Through meticulously removing duplicates, filtering irrelevant article types and performing in-depth relevance screening of titles and abstracts, we obtained 30 research articles that were closely related to our review’s scope, as shown in the tables below (Kumari et al., 2010, 2011; Esteban et al., 2014; Hathaway et al., 2015; Chadha et al., 2017; Hathaway et al., 2017; Sarhan and Azzazy, 2017; Chhibber et al., 2018; Bai et al., 2019; Kaur et al., 2019; Portilla et al., 2020; Zhang et al., 2020; Beschastnov et al., 2021; Sarhan et al., 2021; Shen et al., 2021; Yan et al., 2021; Ilomuanya et al., 2022; Li et al., 2022; Morais et al., 2022; Mabrouk et al., 2022; Dehari et al., 2023; Kielholz et al., 2023; Mukhopadhyay et al., 2023; Shafigh Kheljan et al., 2023; Ilomuanya et al., 2024; Khazani Asforooshani et al., 2024; Narayanan et al., 2024; Shiue et al., 2024; Suchithra et al., 2024; Vacek et al., 2024). According to the currently available literature, novel encapsulated phage delivery systems for the treatment of WIs have been studied much more than lysins (Figure 3). To provide a broader perspective and enhance the organization of the review, we included 68 additional articles in our text.
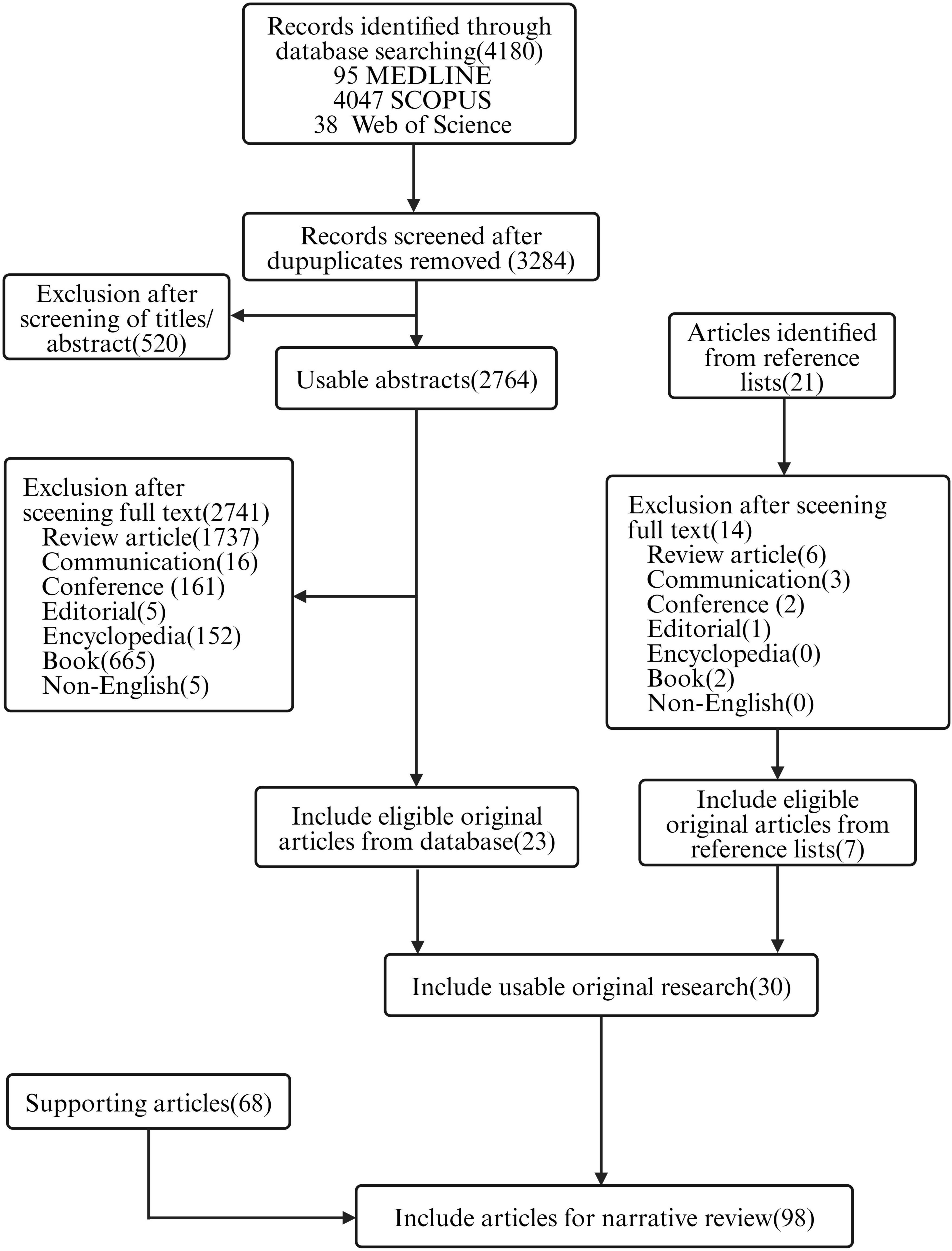
Figure 2. Flowchart of the review (created by BioRender.com, 2024).
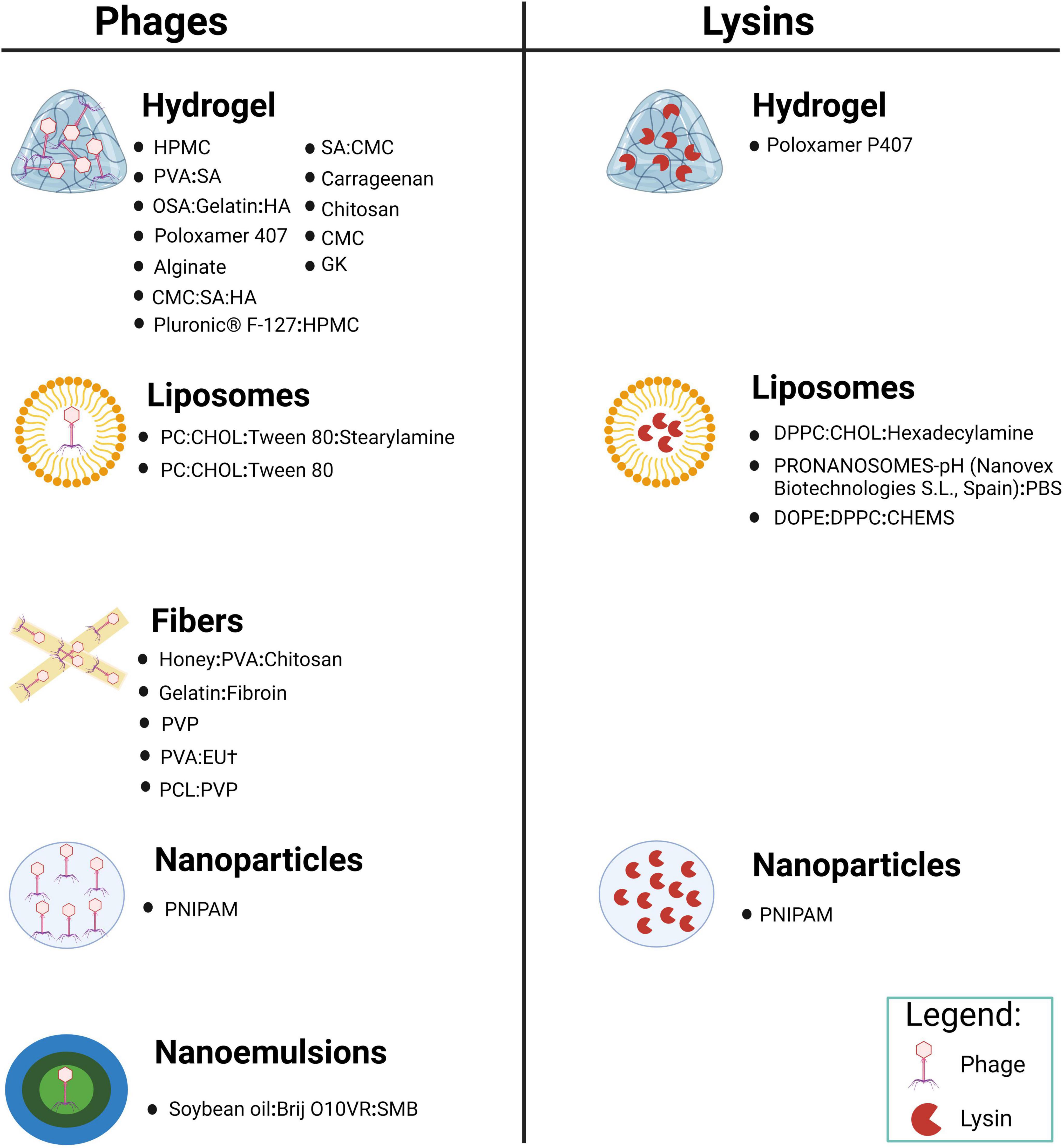
Figure 3. Novel delivery systems encapsulating phage and its encoded lysin in various materials prepared for the treatment of WIs (created by BioRender.com, 2024).
4 Hydrogel
Hydrogels are the most commonly used carriers for delivery of biomolecules in tissue engineering. Hydrogels are highly hygroscopic and capable of retaining large amounts of water, making them suitable for phage delivery. They help protect the skin from excessive fluid loss while absorbing wound excretions (Merabishvili et al., 2017). Hydrogel formulations not only improve the hydration balance at the wound site, but also stabilize the phage by allowing hydrogen bond formation between water and phage proteins (Jones and Vaughan, 2005).
Recently, many animal studies have shown that hydrogels for delivering phages can be used for WIs with MDR bacteria (Table 2). The study implemented by Kumari et al. strongly suggested that hydrogels containing phage Kpn5 have a therapeutic role in the treatment of murine burn WIs (Kumari et al., 2011). Using a mouse model, a phage-loaded polyvinyl alcohol (PVA)-sodium alginate (SA) hybrid dressing was shown to display some characteristics of bacterial pathogen killing and promoting burn wound healing (Kaur et al., 2019). In a recent study of P. aeruginosa-infected rats with thermal skin injury, phage vB_Pae_SMP1 or vB_Pae_SMP5 or a biphage cocktail mixed with 5% w/v carboxymethylcellulose (CMC) hydrogel could control P. aeruginosa infections in burn wounds, promote dermal collagen fiber formation and accelerate healing at the site of injury, as evidenced by histopathological examination (Mabrouk et al., 2022).
The addition of other substances to hydrogels has similarly been demonstrated to have positive effects on wound healing. An injectable hydrogel encapsulating acidic fibroblast growth factor (aFGF) and phage had significant bacteriostatic activity against MDR Escherichia coli (E. coli), and significantly increased the VEGF, α-SMA and collagen I expression levels in regenerated tissues at day 7, indicating that hydrogels containing aFGF can effectively improve tissue regeneration, enhance angiogenic activity, promote skin regeneration and prevent bacterial infections (Zhang et al., 2020). Moreover, the use of phage preparations in combination with anaesthetics may provide a painless solution for wound treatment. Beschastnov et al. (2021) produced hydrogel wound dressings with the optional addition of lidocaine. The results of in vitro studies showed that phages immobilized in hydrogels retained their viability and solubilizing activity, and phage activity was maintained when combined with lidocaine.
Notably, phages were well tolerated and remained stable in hydrogels formulated with neutral polymers (Chang et al., 2020; Kim et al., 2021). At physiological pH, the caps of phages exhibit a net negative charge, whereas the tails exhibit a net positive charge (Anany et al., 2011). Anionic polymers will interact with the positively charged phage tails by electrostatic attraction, blocking the binding of the tail fibers to the host bacterial receptor, resulting in the phage no longer being infectious to the host bacteria. Osborne et al. (2018) prepared phage-containing hydrogels using nonionic and anionic polymers. Phages formulated in carbomer (anionic polymer) hydrogels displayed a 99.95% decrease in titre after 4 weeks of storage at 4°C, whereas phages formulated in hydroxyethylcellulose gel (nonionic) hydrogels remained biostable (Osborne et al., 2018). The limited number of studies mentioned above highlights the importance of selecting neutral polymers that minimize charge-induced phage inactivation and thus maintain phage stability. Phages PEV1 and PEV31 remained stable in aqueous gels formulated with poly(ethylene oxide) (PEO), PVA and hydroxypropyl methylcellulose (HPMC) (Chang et al., 2021). Due to the limited number of hydrogels tested for phage stability, neutral polymer hydrogels such as guar gum, agarose, polyvinylpyrrolidone or polyacrylamide can be investigated in the future.
Currently, there is a lack of research in the field on how to maintain the stability of phage hydrogels for long-term storage. The stability of phages depends on many of the following factors: pH, temperature, formulation composition, and light (Jonczyk-Matysiak et al., 2019). There are only a few studies on the short-term storage of hydrogel to maintain the stability of phages. The stability of Klebsiella phage Kpn5 in a 3% HPMC hydrogel at 37°C was only seven days (Kumari et al., 2010, 2011). Klebsiella phage Kpn5, Pseudomonas phage PA5 and Staphylococcus phage MR10 remained active after 28 days of storage at room temperature in PVA-SA hydrogels (Kaur et al., 2019). It is clear that maintaining long-term phage stability in hydrogels deserves more work to improve.
Hydrogels are also an effective delivery system for sustained lysin release to the site of infection, as illustrated in Table 2. A thermosensitive hydrogel containing the G– lysin LysP53 was safe, non-cytotoxic and hydrolysed the resting Acinetobacter baumannii (A. baumannii) peptidoglycan, resulting in bactericidal activity (Li et al., 2022). LysP53-loaded hydrogels may provide a novel solution for the treatment of A. baumannii-induced WIs (Li et al., 2022). Thermostable polymers should be considered for investigation. Following infection, the skin temperature increases, which has been observed in chronic leg ulcers. A cocktail consisting of cysteine, the CHAP structural domain of phage K lysin and lysostaphin was added to a poly (N-isopropylacrylamide) (PNIPAM) gel (Hathaway et al., 2017). An increase in temperature successfully triggered their release. In addition, the lysin-loaded hydrogel had the properties of killing pathogenic bacteria in fracture-associated infections and promoting fracture healing. Yao et al. (2021) designed a chimeric ClyC-loaded alginate hydrogel (ClyC-AH), which was non-cytotoxic and retained the stability and activity of ClyC, while providing sustained ClyC release and antimicrobial efficacy against S. aureus. In a mouse model of osteomyelitis, ClyC-AH reduced the number of S. aureus in the femur and surrounding tissues. However, none of these studies had yet assessed the long-term storage stability of lysin in hydrogels, which is essential for the development of commercially viable lysin products. This shows that it is a major challenge to maintain the long-term stability of lysin in hydrogels.
5 Liposomes
Liposomes are spherical particles consisting of natural lipid bilayers that can contain drugs; hydrophobic or amphiphilic molecular drugs are present within the membrane or at the interface, respectively (Sercombe et al., 2015; Kuhn et al., 2017). Liposomes can be prepared as multi- or unilamellar vesicles of various sizes. The non-immunogenicity, biodegradability and biocompatibility of liposomes make them a suitable phage delivery system (Colom et al., 2015).
A summary of studies on the application of liposome delivery systems encapsulating phage for WIs is presented in Table 3. Liposomes help to improve phage survival, stability and retention time at the site of WI. Chadha et al. (2017) evaluated the potential of liposomes as phage delivery vehicles for the treatment of burn WIs. The results showed that liposome delivery of phages increased phage retention time in the body and enhanced efficacy compared to phage use alone, which in turn resulted in greater bacterial volume reductions in the bloodstream and major organs of mice treated with the contained cocktail of phage liposomes, and consequently a more rapid rate of infection regression. Diminished phage immunogenicity reduced cytokine levels (interleukin-1β and tumor necrosis factor-α) compared to baseline (Chadha et al., 2017). In addition, liposomes protect phages from pH-induced inactivation, which is particularly important in acidic environments such as wound infection sites. Chhibber et al. (2018) evaluated the ability of a liposome-encapsulated cocktail of phages to resolve diabetic excisional WIs induced by S. aureus. The results of in vitro stability studies and in vivo phage titre assays indicated that WI treatment with phage-loaded liposomes improved phage persistence in vitro and in vivo, suppressed bacterial counts and inflammation levels in wound tissue and promoted wound tissue collagen fiber maturation, improving wound healing.
Not to be overlooked is the fact that cationic liposome are well suited for delivery of phages. Cationic liposomes can also be used to enhance interactions with negatively charged bacteria, because there is a higher affinity between positively charged liposomes and bacteria. In addition, cationic liposomes have mucoadhesive properties that prolong the drug retention time at the administration site, resulting in controlled release and improved therapeutic efficacy (Shaikh et al., 2011). A myxoma virus and two Podoviridae viruses did not degrade when delivered in cationic liposome particles, thus prolonging their residence time in animals (Colom et al., 2015). The obstacles observed so far, such as low encapsulation efficiency, difficulty in controlling liposome size, and loss of active phage during preparation, suggest that liposomes are not perfect delivery vehicles. Therefore, rigorous testing is needed to determine the general suitability of delivery vectors, i.e., whether liposomes can be used and which types of lipids may be suitable.
At the same time, alternatives to liposomes, such as so-called transferosomes, i.e., liposomes containing detergents, must be explored. Transferosomes are cationic liposomes consisting of phosphatidylcholine, tween-80 and stearyl amine, which are used to improve permeability (Chhibber et al., 2017). Chhibber et al. (2017) evaluated the use of transferosomes as a transdermal delivery system for encapsulating MRSA phage cocktails. The results of in vitro stability and in vivo phage titre experiments showed that the transporter-delivered cocktail phage had better persistence and stability compared to free phage. Thigh infections in rats treated with transposome-delivered cocktail phage resolved within 7 days, compared to 20 days in untreated animals. In addition, this study found that transposome-delivered phage had better therapeutic advantages over free phage in the treatment of skin and soft tissue infections. Unlike free phage, the transferosome-delivered phage cocktail protected all test animals (no deaths) even when administered with a 12-hour delay after infection. However, Niosomes composed of nonionic surfactants and other amphiphilic molecules as well as cholesterol face similar challenges as all amphiphilic vesicle particles (Marianecci et al., 2014).
Lysin-loaded liposomes are also a very attractive option. To date, there are three examples of liposome-delivered phage lysins, as summarized in Table 3. For example, Hajiahmadi et al. (2019) prepared a number of vancomycin-loaded nanoliposomes conjugated with anti-staphylococcal proteins (lysozyme) and evaluated their in vitro and in vivo efficacy as topical therapy for MRSA. Targeted liposomes inhibited bacterial infections in vitro and in vivo more effectively than non-targeted vancomycin liposomes. The results suggest that a novel nanocarrier (lysostaphin-conjugated coupled liposomal vancomycin) can be used as an optimal topical antibacterial construct for the treatment of MRSA skin infections (Hajiahmadi et al., 2019). Here, Portilla et al. (2020) also found that pH-sensitive liposomes can be used to deliver the endolysin LysRODI, which eliminates S. aureus planktonic and biofilm populations in weakly acidic environments. This included different human bodily regions, such as the skin or vagina, as well as certain food or surgical sites where staphylococcal contamination may be a problem. Notably, S. aureus biofilm formation is enhanced at ambient pH values between 4.75 and 5.5, which is the range of content release of the liposomes used in the study performed by Foulston et al. (2014). Nevertheless, although liposome-encapsulated LysRODI was effective against biofilm cells, its activity was significantly hampered by the biofilm matrix compared to the free lysin. More research in the future should overcome it by altering the composition of lipid vesicles or trying combinatorial strategies with matrix-degrading enzymes. Another interesting strategy to explore wounding is to dope the surface of liposomes with antibodies or other proteins that can interact with biofilm molecules and thus facilitate the penetration of lipid vesicles into these robust structures (Portilla et al., 2020).
6 Electrospun fibers
In addition to the use of liposomes, phages can be delivered in electrospun fibers. Phages can withstand an electric field of up to 40 kV/cm for 5 min and can be embedded in fibers during the electrospinning process, and a variety of materials (cellulose diacetate, PEO, PVP, polyester urea) have been demonstrated to be suitable for this method (Korehei and Kadla, 2013; Dai et al., 2014; Korehei and Kadla, 2014; D rehei and Kadla). The key point is that a large number of synthetic agents (anticancer, anti-inflammatory and antimicrobial drugs), bioactive agents (DNA, proteins, enzymes) and living cells (viruses, algae) can be doped during the electrostatic spinning process to develop electrospun fibers that can be further used as suitable carrier systems (Kaur et al., 2021).
Fiber production may expose the phage particles to damage. Loss of phage viability during electrostatic spinning due to rapid solvent evaporation and drastic changes in osmotic pressure is found. While fibers can still be produced using pure distilled water, such compositions are not ideal for electrospinning, phage delivery or long-term storage. By contrast, the morphology produced by fibers composed of buffer solutions has been shown to provide a thermodynamically favorable microenvironment in which phages will be encapsulated, thus maintaining their infectivity for up to 8 weeks (Dai et al., 2014). Furthermore, the addition of sugars (e.g., tohalose) or solvent components can stabilize phages and reduce the formation of salt crystals, thus preventing complete phage inactivation (Dai et al., 2014). Despite the protective effect of the addition of alginate, buffer on the high pressure and spinning process, partial loss of phage activity was observed (Zussman, 2011; Dai et al., 2014).
Studies of phage-loaded electrospun fibers applied to WIs are summarized in Table 4. There are two general types of electrostatic spinning: emulsion and coaxial electrostatic spinning. These two types of electrostatic spinning can solve the disadvantages of electrostatic spinning mentioned above. Emulsion electrospinning involves pre-encapsulating phage in alginate nanoparticles, freeze-drying them, suspending them in a polymer-containing solvent and electrostatically spinning them. Doping of emulsion electrospun fibers containing T4 phage into alginate capsules provided a shielding barrier against rapid dehydration stress, and only a slight decrease in phage activity was observed (Korehei and Kadla, 2013). Phage-loaded gelatine/silk fibroin fibers prepared by Sarhan et al. had an amorphous structure, good thermal stability at temperatures up to 300°C, good biocompatibility, promoted fibroblast proliferation and displayed significant inhibition of MDR P. aeruginosa (Sarhan et al., 2021). This suggested that the electrospun fibers are capable of maintaining phage stability, and are a promising dressing for MDR P. aeruginosa infected wounds (Sarhan et al., 2021). In contrast, coaxial electrospinning involves incorporating phage in the core of a core-shell fiber structure, thereby encapsulating the phage in the core-shell fiber. Coaxial spinning produced a continuous core/shell morphology with a more homogeneous distribution of phage in the fiber core and no major changes in the permeation environment, thus maintaining full solubilization activity (Korehei and Kadla, 2013). Kielholz et al. (2023) employed a coaxial electrostatic spinning technology approach to fabricate functional fibers with phage core/PVP shells with mechanical properties that are well suited for P. aeruginosa and S. aureus-infected wound applications. This core-shell fiber has good rapid-release kinetics, biocompatibility and antimicrobial activity. On the basis of the above features, this fiber prepared by coaxial electrostatic spinning is suitable for WIs (Kielholz et al., 2023). Unfortunately, the storage time of these phage-loaded electrospun fibers was short, with the antimicrobial properties remaining unchanged for 90 days at 4°C and 60 days at 25°C. These factors limit their wide clinical application (Kielholz et al., 2023; Ilomuanya et al., 2024).
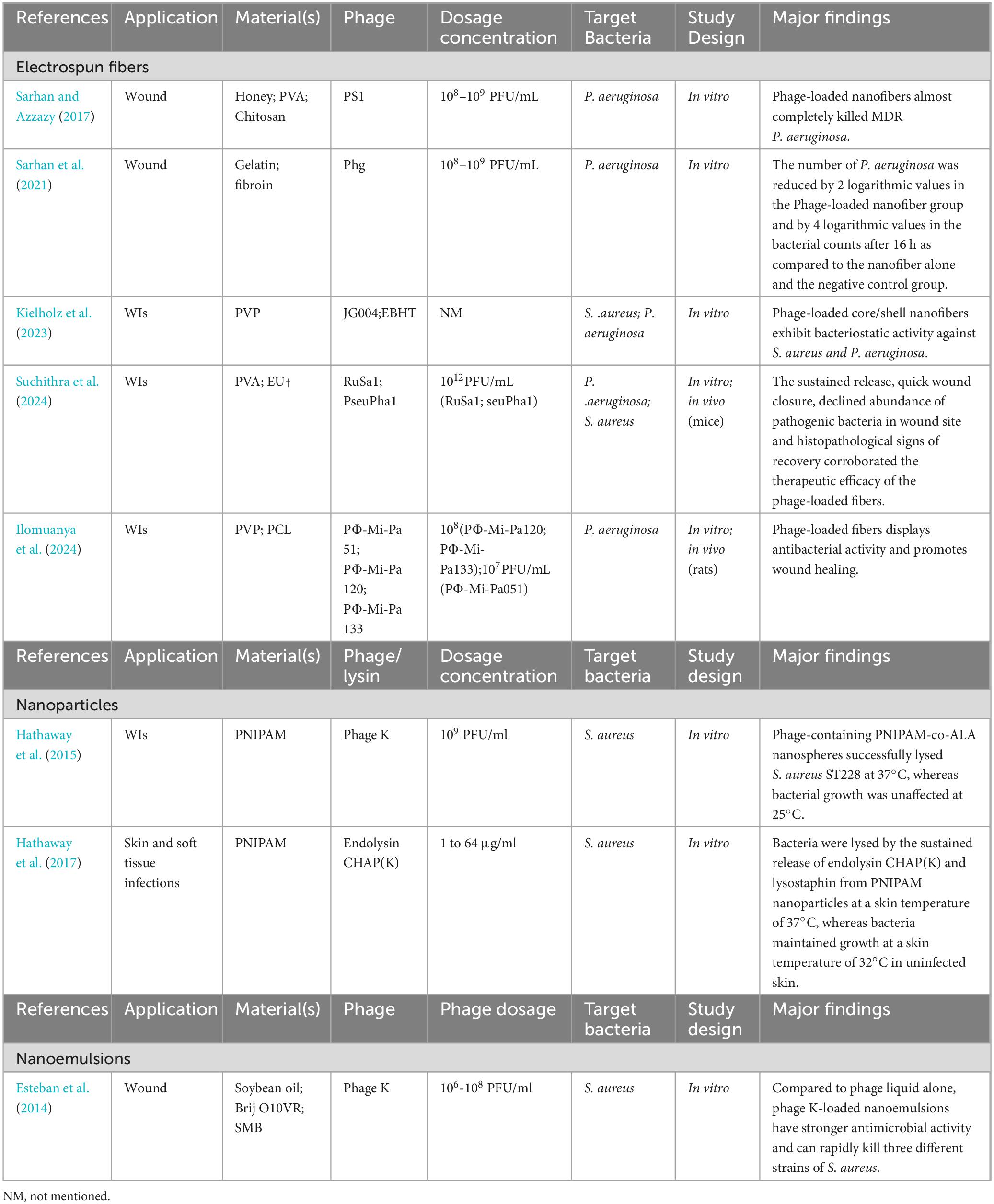
Table 4. Summary study of other novel delivery systems for phages and lysins in the topical management of WIs.
By contrast, little research has been published on the application of fiber delivery systems encapsulating phage-derived lytic lysins for infected wounds. Taking inspiration from nature, Miao et al. (2011) used lysostaphin (Lst), a cytolytic enzyme with specific bactericidal activity against S. aureus, to create an anti-infective bandage. Lst was immobilized in a cellulose binding module consisting of the room temperature ionic liquid 1-ethyl-3-methylimidazolium acetate electrospun cellulose, cellulose-chitosan and cellulose-poly(methyl methacrylate) in homogeneous solution to generate biocompatible fibers. The fibers were chemically treated to generate aldehyde groups for covalent immobilization of Lst. The Lst-functionalised cellulose fibers obtained from the processing could be made into bandage formulations, which displayed antistaphylococcal activity against S. aureus in an in vitro skin model and low toxicity for keratinocytes, suggesting that these materials are biocompatible and have a good potential to serve as an antimicrobial matrix in wound healing applications. This approach provides a new idea for the development of a fiber delivery system encapsulating phage-encoded lysins.
7 Nanoparticles
Recent advances in nanotechnology have opened new frontiers for the application of small molecules, proteins, viruses and other pharmaceutically active substances for chronic wound healing (Peng et al., 2022; Liu et al., 2023; Wang et al., 2023). The size and physicochemical properties of nanostructures allow intracellular delivery of actives, their protection from degradation and their enhanced penetration into wounds. In addition, delivery of active substances in nanocarriers enables different drug release profiles, thus meeting the requirements of wound healing (Liu et al., 2023).
Nanoparticles are normally small-sized nanospheres constructed at the molecular level. These spheres can be used to deliver actives drug that degrade in the environment. Phage delivery processes need to be carefully designed to prevent damage to the viral capsid and DNA/RNA components, and to prevent a decrease in phage activity during production. Hathaway et al. (2015) developed nanospheres consisting of PNIPAM, to which S. aureus phage K was incorporated, as shown in Table 4. When the temperature rises (usually at the site of bacterial skin infection), the nanospheres dissolve, releasing active phage cargo, which inhibits bacterial growth (Hathaway et al., 2015). Hyaluronic acid (HA) methacrylate is coated on the agarose gel containing S. aureus phage K, essentially creating a two-layer hydrogel. During infection, the outer layer of HA is solubilized by enzymes produced by the pathogen, and phages are released in the vicinity of the infection (Bean et al., 2014).
Nanoparticle delivery of lysins protects the proteins from degradation, provides sustained release and aids in achieving better bacteriolysis. Kaur et al. (2020) evaluated the antibacterial characteristics of alginate-chitosan nanoparticles (Alg-Chi NPs) loaded with LysMR-5 against S. aureus. Alg-Chi Nps loaded with LysMR-5 displayed enhanced bactericidal activity against S. aureus relative to single Alg-Chi Nps. The nanoparticle diameters of the LysMR-5-loaded formulations remain essentially unchanged for about 9 weeks at 4°C, but will change significantly at 25°C (Kaur et al., 2020). This shows that the long-term stability of nanoparticle formulations still requires us to be worthy of vigilance. Hathaway et al. (2017) delivered the truncated endolysin CHAP(K) and bacteriocin lysostaphin within PNIPAM nanoparticles, which at the specific temperature of 37°C release and treat skin infections caused by S. aureus, as shown in Table 4. In addition, the combination of CHAP(K) and bacteriocin lysostaphin acted synergistically to accelerate control and the response time to MRSA treatment (Hathaway et al., 2017). The use of nanoparticles as delivery vehicles for lysins is a novel approach in making lysins a viable treatment for infections by utilizing and developing the physical and chemical properties of new and existing nanoparticles.
8 Nanoemulsions
Nanoemulsions are colloidal spherical systems with amorphous and lipophilic (negatively charged) surfaces of average particle sizes between 10 and 1,000 nm (Jaiswal et al., 2015). Nanoemulsification technology allows for the formation of homogeneous nanoemulsions by adding a mixture of microorganisms, cells, enzymes and drugs, as well as compatible polymers to the oil, and then further dispersing the mixture into another phase of vegetable oil (McClements and Rao, 2011). The nanoemulsion may be water-in-oil (W/O), but may also be oil-in-water (O/W), and in some cases a third phase may be present (Puapermpoonsiri et al., 2009; Esteban et al., 2014). Nanoemulsions can be further stabilized by the addition of emulsifiers and stabilizers. Therefore, nanoemulsions provide a new way to encapsulate sensitive molecules such as proteins, enzymes and phages, among others, in a nanoporous matrix. Nanoemulsion formulations can facilitate transdermal absorption by varying droplet size, altering emollients and/or emulsifiers and adding particulate components to the mixture (Otto et al., 2009). This makes them particularly effective in treating deep skin infections.
Multiple emulsions prepared by microfluidic methods, such as W1/O/W2 emulsions (where the intermediate phase is immiscible with the inner and outer phases), have a high degree of precision and controllable droplet size and shape (Dickinson, 2011). Depending on the number of immiscible phases, different amounts of multiple active ingredients can be prepared (e.g., phage co-encapsulated with antibiotics or phage co-encapsulated with antimicrobial peptides or lysin), with the release sequence selected as desired. Such multishell droplets can maximize the protection of highly sensitive phages or phage enzymes from external stressful environments. For example, the outer shell protects the phage from the pH of gastric acid because it is acid-resistant. The next layer of the inner shell may subsequently be used for burst release to act immediately in releasing a large number of phages for immediate bacterial population control. The final layer or inner shell can be used for sustained release of phages or antibacterial substances to maintain a slow but sustained release pattern, which is particularly important for treating chronic infections, and also reduces or avoids the need to reuse phages or antibacterial substances.
Nanoemulsions can be considered to be a new reliable option for phage delivery. To provide maximum protection to highly sensitive phages from external stressful environments, nanoemulsification technology can deliver single or multiple active ingredients with sequential release, depending on the desired regimen (Kaur et al., 2021). Esteban et al. (Esteban et al., 2014) used nanoemulsion encapsulation to stabilize phage K lysates, which were subsequently tested in vitro against three species of S. aureus, as shown in Table 4. Here, the phage surrounded the nanodroplets instead of being encapsulated in them. Phages in the emulsion had higher activity than unemulsified phages over a period of 10 days at 4°C and room temperature. Phage emulsion formulations were also effective in killing three S. aureus strains within 2–5 h relative to liquid phage controls. Because both phages and bacteria have a very negative “charge,” the presence of nanoemulsions reduces the electrostatic repulsion between them and facilitates their interaction, thus enhancing the anti-microbial or killing effect (Esteban et al., 2016). In addition, due to less surfactants required in the preparation process, such O/W nanoemulsions are more permeable transdermally and more biocompatible with skin tissues (Azeem et al., 2009). Nanoemulsified phage formulations are used for superficial and deep skin infections as well as wound application/dressings, resulting in sustained active phage release. However, the activity of the phage emulsion did not change significantly over a 10-day period, whereas the lysogenic activity of the phage appeared to be erratic, with different results for each day of treatment and each storage temperature (Esteban et al., 2014). To further deepen the research on nanoemulsion formulations for delivery of phage, the above issues need to be thoroughly addressed.
Strategies to enhance antimicrobial efficacy through nanoemulsions also include the incorporation of lysin into antimicrobial matrices and systems that extend the duration of lysin administration. One approach to prolong lysin administration at the site of infection is nanoemulsion delivery of lysin. These thermodynamically stable particles adhere well to the skin, which improves bioavailability (Tayeb and Sainsbury, 2018). Recombinant lysostaphin has been formulated in a nanogel to extend the release time up to 8 h. A treatment regimen of three topical applications per day was sufficient to cure MRSA-infected abscesses in mouse skin (Nour El-Din et al., 2020). Although lysozyme is a bacteriocin, similar applications could be envisaged using phage-derived or engineered lysin.
9 Discussion
The treatment of MDR bacterial WIs raises many challenges and new insights are urgently needed in this field (Raducu et al., 2024). Phage and lysin formulations may be a promising strategy to treat MDR bacterial WIs (Moghadam et al., 2024; Wang et al., 2024). Novel delivery systems (e.g., hydrogel, liposomes, electrospun fibers, nanoparticles, nanoemulsions) in combination with phages or lysins provide a rich toolbox to treat MDR bacterial WIs. This article focuses on a review of some recent advances in novel delivery systems that deliver phages or lysins for topical application to WIs.
A good drug formulation must be able to encapsulate a specific drug and protect it from the surrounding environment, such as pH and temperature, among others, while maintaining the biological activity of the drug, particularly its antimicrobial properties (Rosner and Clark, 2021; Briot et al., 2022). In addition, the chosen drug delivery system must be biocompatible and capable of releasing the drug in an orderly and slow manner at the desired infection site (Rosner and Clark, 2021; Briot et al., 2022).
Existing literature on novel delivery systems encapsulating phages and lysins remains scarce, particularly on novel drug delivery systems encapsulating lysins. Phages are mostly administered as suspensions or, in the case of skin infections, associated with dressings (Pinto et al., 2020; Pinto et al., 2021). Most of the novel drug delivery systems regarding encapsulated phages were developed during just more than the last decade. It is of note that novel drug delivery systems for lysins started in 2017 (Pinto et al., 2021).
Recently, novel delivery systems encapsulating phages or lysins, such as hydrogel, liposomes, electrospun fibers, nanoparticles and nanoemulsions, have been reported, and these studies have confirmed that novel delivery systems encapsulating therapeutic substances are beneficial for healing infected wounds (Lin et al., 2024). However, each novel delivery system has its own limitations and challenges, such as the complexity of its manufacture, the potential loss of antimicrobial activity during processing, and the difficulty of stability of the formulation over long periods of storage. In addition, these studies rarely mention in detail the interactions between carriers and drugs and the level of comparison with other types of systems. Consequently, there are no clear conclusions as to which drug delivery system performs the best.
To date, various in vitro and in vivo studies have demonstrated the great therapeutic potential of novel delivery systems encapsulating phages or lysins for the treatment of WIs, and most of these studies have demonstrated their therapeutic benefit through animal models. However, few studies have considered the irregular shape and varying depths of clinical infected wounds; animal models of infected wounds are normally a simple and regular situation.
Despite the amount of research focusing on the therapeutic potential of novel drug delivery systems, there remains many practical challenges in treating patients with WIs. Because of the current gaps, greater attention needs to be paid to clinical specifics from different perspectives. First, infected wounds in patients are frequently associated with uncontrolled hyperglycaemia or vascular pathology (Liu et al., 2023). Ideally, novel delivery systems should encapsulate drugs that can lower blood glucose or ameliorate vasculopathy, in addition to phages or lysins. Second, the relationship between the action of the loaded drug and the pathological stage of the infected wound should also be elaborated. In addition, the strains in chronic infected wounds are diverse and change dynamically over time (Nahid et al., 2021). Because no single drug works optimally or is suitable for all types of infected wound conditions, future studies may also consider the development of novel drug delivery systems loaded with multiple active drugs to provide synergistic effects at different stages and for varying pathological tissue types as well as in multi-strain infections. Preparation techniques for novel drug delivery systems are also important, and these should be scalable, reproducible and cost-effective to allow their use for a wide range of clinical applications. Clinical validation of these drug delivery systems requires more extensive in vivo studies and clinical trials to confirm their safety, efficacy and performance in the real world.
10 Conclusion
Currently, WIs associated with MDR bacteria pose many difficulties. In the future, phage and lysin therapies will remain a popular research topic for the treatment of MDR-infected wounds. Phage and lysin formulations have evolved from traditional liquid formulations to novel delivery systems that have been demonstrated in different laboratory models. Limited experimental data suggest that novel delivery systems such as hydrogel, liposomes, electrospun fibers, nanoparticles and nanoemulsions encapsulating phages and lysins for the treatment of WIs have significant bacteriostatic properties and promote wound healing. Continuous improvement and innovation of novel drug delivery systems could bring closer the full realization of the therapeutic potential of phages and lysins, ultimately leading to improved wound care strategies and better patient outcomes for MDR bacterial WIs.
Author contributions
PY: Data curation, Writing – original draft. JL: Writing – original draft. XM: Software, Writing – review & editing, Data curation NH: Writing – review & editing, Data curation. ZS: Formal analysis, Writing – review & editing. BC: Conceptualization, Formal analysis, Funding acquisition, Project administration, Resources, Supervision, Visualization, Writing – review & editing, Investigation. SL: Conceptualization, Funding acquisition, Investigation, Methodology, Project administration, Resources, Supervision, Validation, Visualization, Writing – review & editing.
Funding
The author(s) declare that financial support was received for the research, authorship, and/or publication of this article. This work was supported by the National Key Research Programme Project (No. 2023YFE0204500), Guangdong Key Research Programme Project (No. 20200401), and Natural Science Foundation Project of Chongqing Science & Technology Commission (No. CSTB2024NSCQ-MSX0770).
Acknowledgments
The years turn and the stars change. On the occasion of her birthday, Pan Yang wishes Shizhu Li a happy birthday, youthfulness and beauty, and success in all her endeavors.
Conflict of interest
The authors declare that the research was conducted in the absence of any commercial or financial relationships that could be construed as a potential conflict of interest.
Generative AI statement
The authors declare that no Generative AI was used in the creation of this manuscript.
Publisher’s note
All claims expressed in this article are solely those of the authors and do not necessarily represent those of their affiliated organizations, or those of the publisher, the editors and the reviewers. Any product that may be evaluated in this article, or claim that may be made by its manufacturer, is not guaranteed or endorsed by the publisher.
Abbreviations
MDR, Multidrug-resistant bacteria; WIs, Wound infections; E. faecalis, Enterococcus faecalis; S. aureus, Staphylococcus aureus; K. pneumonia, Klebsiella pneumoniae; A. baumannii, Acinetobacter baumannii; P. aeruginosa, Pseudomonas aeruginosa; G+/–, Gram-positive/nagetive; MRSA, Methicillin-resistant S. aureus VRSA, Vancomycin-resistant S. aureus; DFU, Diabetic foot ulcers; E. coli, Escherichia coli; AD, Atopic dermatitis; PBS, Phosphate buffered saline; SMB, Tris-buffered saline-magnesium buffer; PEO, Polyethylene oxide; PVA, Polyvinyl alcohol; PVP, Polyvinylpyrrolidone; HPMC, Hydroxypropyl methylcellulose; SA, Sodium alginate; PVA-SA, Polyvinyl alcohol-sodium alginate; CMC, carboxymethylcellulose; OSA, Oxidized sodium alginate; PNIPAM, poly (N-isopropylacrylamide); DPPC, Dipalmitoyl phosphatidyl choline; DOPE, Dioleoyl phosphatidylethanolamine; CHEMS, Cholesteryl hemisuccinate; CHOL, Cholesterol; GK, Gum karaya; EUK, Eudragit; PCL, Polycaprolactone; PCm Phosphatidylcholine; HA, Hyaluronic acid; aFGF, acidic fibroblast growth factor; ClyC-AH, ClyC-loaded alginate hydrogel; Lst, Lysostaphin; Alg-Chi NPs, Alginate-chitosan nanoparticles; W/O, Water-in-oil; MOI, Multiplicity of infection.
References
American Academy of Dermatology Association, (2018). Skin Ulcers, Wounds, and Burns by the Numbers. Available online at: https://assets.ctfassets.net/1ny4yoiyrqia/7dFgDh1R3VVMqtAeAfkQi1/7f5c0925b933ca8bc4afb822c9568daa/16-933-bds-briefs-Skin-ulcers-wounds-and-burns_final.pdf (accessed May 5, 2018).
Anany, H., Chen, W., Pelton, R., and Griffiths, M. W. (2011). Biocontrol of Listeria monocytogenes and Escherichia coli O157:H7 in meat by using phages immobilized on modified cellulose membranes. Appl. Environ. Microbiol. 77, 6379–6387. doi: 10.1128/AEM.05493-11
Azeem, A., Rizwan, M., Ahmad, F. J., Iqbal, Z., Khar, R. K., Aqil, M., et al. (2009). Nanoemulsion components screening and selection: A technical note. AAPS PharmSciTech 10, 69–76. doi: 10.1208/s12249-008-9178-x
Bai, J., Yang, E., Chang, P. S., and Ryu, S. (2019). Preparation and characterization of endolysin-containing liposomes and evaluation of their antimicrobial activities against gram-negative bacteria. Enzyme Microb. Technol. 128, 40–48. doi: 10.1016/j.enzmictec.2019.05.006
Bean, J. E., Alves, D. R., Laabei, M., Esteban, P. P., Thet, N. T., Enright, M. C., et al. (2014). Triggered release of bacteriophage K from agarose/hyaluronan hydrogel matrixes by Staphylococcus aureus virulence factors. Chem. Materials 26, 7201–7208.
Beschastnov, V. V., Ryabkov, M. G., Leontiev, A. E., Tulupov, A. A., Yudanova, T. N., Shirokova, I. Y., et al. (2021). Viability of bacteriophages in the complex hydrogel wound dressings in vitro. Sovrem Tekhnologii Med. 13, 32–38. doi: 10.17691/stm2021.13.2.03
Briers, Y., Walmagh, M., Grymonprez, B., Biebl, M., Pirnay, J. P., Defraine, V., et al. (2014a). Art-175 is a highly efficient antibacterial against multidrug-resistant strains and persisters of Pseudomonas aeruginosa. Antimicrob. Agents Chemother. 58, 3774–3784. doi: 10.1128/AAC.02668-14
Briers, Y., Walmagh, M., Van Puyenbroeck, V., Cornelissen, A., Cenens, W., Aertsen, A., et al. (2014b). Engineered endolysin-based “Artilysins” to combat multidrug-resistant gram-negative pathogens. mBio 5:e1379-14. doi: 10.1128/mBio.01379-14
Briot, T., Kolenda, C., Ferry, T., Medina, M., Laurent, F., Leboucher, G., et al. (2022). Paving the way for phage therapy using novel drug delivery approaches. J. Control Release 347, 414–424. doi: 10.1016/j.jconrel.2022.05.021
Chadha, P., Katare, O. P., and Chhibber, S. (2017). Liposome loaded phage cocktail: Enhanced therapeutic potential in resolving Klebsiella pneumoniae mediated burn wound infections. Burns 43, 1532–1543. doi: 10.1016/j.burns.2017.03.029
Chang, C., Yu, X., Guo, W., Guo, C., Guo, X., Li, Q., et al. (2022). Bacteriophage-mediated control of biofilm: A promising new dawn for the future. Front. Microbiol. 13:825828. doi: 10.3389/fmicb.2022.825828
Chang, R. Y. K., Morales, S., Okamoto, Y., and Chan, H. K. (2020). Topical application of bacteriophages for treatment of wound infections. Transl. Res. 220, 153–166.
Chang, R. Y. K., Okamoto, Y., Morales, S., Kutter, E., and Chan, H. K. (2021). Hydrogel formulations containing non-ionic polymers for topical delivery of bacteriophages. Int. J. Pharm. 605:120850. doi: 10.1016/j.ijpharm.2021.120850
Chhibber, S., Kaur, J., and Kaur, S. (2018). Liposome entrapment of bacteriophages improves wound healing in a diabetic mouse MRSA infection. Front. Microbiol. 9:561. doi: 10.3389/fmicb.2018.00561
Chhibber, S., Shukla, A., and Kaur, S. (2017). Transfersomal phage cocktail is an effective treatment against methicillin-resistant Staphylococcus aureus-mediated skin and soft tissue infections. Antimicrob. Agents Chemother. 61:e2146-16. doi: 10.1128/AAC.02146-16
Colom, J., Cano-Sarabia, M., Otero, J., Cortes, P., Maspoch, D., and Llagostera, M. (2015). Liposome-encapsulated bacteriophages for enhanced oral phage therapy against Salmonella spp. Appl. Environ. Microbiol. 81, 4841–4849. doi: 10.1128/AEM.00812-15
Dai, M., Senecal, A., and Nugen, S. R. (2014). Electrospun water-soluble polymer nanofibers for the dehydration and storage of sensitive reagents. Nanotechnology 25:225101. doi: 10.1088/0957-4484/25/22/225101
De Maesschalck, V., Gutierrez, D., Paeshuyse, J., Lavigne, R., and Briers, Y. (2020). Advanced engineering of third-generation lysins and formulation strategies for clinical applications. Crit. Rev. Microbiol. 46, 548–564. doi: 10.1080/1040841X.2020.1809346
de Wit, J., Totte, J. E. E., Van Mierlo, M. M. F., Van Veldhuizen, J., Van Doorn, M. B. A., Schuren, F. H. J., et al. (2019). Endolysin treatment against Staphylococcus aureus in adults with atopic dermatitis: A randomized controlled trial. J. Allergy Clin. Immunol. 144, 860–863. doi: 10.1016/j.jaci.2019.05.020
Dedrick, R. M., Guerrero-Bustamante, C. A., Garlena, R. A., Russell, D. A., Ford, K., Harris, K., et al. (2019). Engineered bacteriophages for treatment of a patient with a disseminated drug-resistant Mycobacterium abscessus. Nat. Med. 25, 730–733.
Dehari, D., Kumar, D. N., Chaudhuri, A., Kumar, A., Kumar, R., Kumar, D., et al. (2023). Bacteriophage entrapped chitosan microgel for the treatment of biofilm-mediated polybacterial infection in burn wounds. Int. J. Biol. Macromol. 253:127247. doi: 10.1016/j.ijbiomac.2023.127247
Díaz, A., Del Valle, L. J., Rodrigo, N., Casas, M. T., Chumburidze, G., Katsarava, R., et al. (2018). Antimicrobial activity of poly (ester urea) electrospun fibers loaded with bacteriophages. Fibers 6:33.
Ding, X., Tang, Q., Xu, Z., Xu, Y., Zhang, H., Zheng, D., et al. (2022). Challenges and innovations in treating chronic and acute wound infections: From basic science to clinical practice. Burns Trauma 10:tkac014. doi: 10.1093/burnst/tkac014
Esteban, P. P., Alves, D. R., Enright, M. C., Bean, J. E., Gaudion, A., Jenkins, A. T., et al. (2014). Enhancement of the antimicrobial properties of bacteriophage-K via stabilization using oil-in-water nano-emulsions. Biotechnol. Prog. 30, 932–944. doi: 10.1002/btpr.1898
Esteban, P. P., Jenkins, A. T., and Arnot, T. C. (2016). Elucidation of the mechanisms of action of Bacteriophage K/nano-emulsion formulations against S. aureus via measurement of particle size and zeta potential. Colloids Surf. B Biointerfaces 139, 87–94. doi: 10.1016/j.colsurfb.2015.11.030
Feng, Y., Fang, Q., Luo, H., Li, J., Yin, X., and Zong, Z. (2024). Safety and efficacy of a phage cocktail on murine wound infections caused by carbapenem-resistant Klebsiella pneumoniae. Int. J. Antimicrob. Agents 63:107088. doi: 10.1016/j.ijantimicag.2024.107088
Foulston, L., Elsholz, A. K., Defrancesco, A. S., and Losick, R. (2014). The extracellular matrix of Staphylococcus aureus biofilms comprises cytoplasmic proteins that associate with the cell surface in response to decreasing pH. mBio 5:e1667-14. doi: 10.1128/mBio.01667-14
Hajiahmadi, F., Alikhani, M. Y., Shariatifar, H., Arabestani, M. R., and Ahmadvand, D. (2019). The bactericidal effect of lysostaphin coupled with liposomal vancomycin as a dual combating system applied directly on methicillin-resistant Staphylococcus aureus infected skin wounds in mice. Int. J. Nanomedicine 14, 5943–5955. doi: 10.2147/IJN.S214521
Hathaway, H., Ajuebor, J., Stephens, L., Coffey, A., Potter, U., Sutton, J. M., et al. (2017). Thermally triggered release of the bacteriophage endolysin CHAP(K) and the bacteriocin lysostaphin for the control of methicillin resistant Staphylococcus aureus (MRSA). J. Control Release 245, 108–115. doi: 10.1016/j.jconrel.2016.11.030
Hathaway, H., Alves, D. R., Bean, J., Esteban, P. P., Ouadi, K., Sutton, J. M., et al. (2015). Poly(N-isopropylacrylamide-co-allylamine) (PNIPAM-co-ALA) nanospheres for the thermally triggered release of Bacteriophage K. Eur. J. Pharm. Biopharm. 96, 437–441. doi: 10.1016/j.ejpb.2015.09.013
Ilomuanya, M. O., Enwuru, N. V., Adenokun, E., Fatunmbi, A., Adeluola, A., and Igwilo, C. I. (2022). Chitosan-based microparticle encapsulated Acinetobacter baumannii phage cocktail in hydrogel matrix for the management of multidrug resistant chronic wound infection. Turk. J. Pharm. Sci. 19, 187–195. doi: 10.4274/tjps.galenos.2021.72547
Ilomuanya, M. O., Oseni, B. A., Okwuba, B. C., Abia, P., Aboh, M. I., Oluwale, O. P., et al. (2024). Evaluation of MDR-specific phage PΦ-Mi-Pa loaded mucoadhesive electrospun nanofibrous scaffolds against drug-resistant Pseudomonas aeruginosa- induced wound infections in an animal model. Int. J. Biol. Macromol. 277:134484. doi: 10.1016/j.ijbiomac.2024.134484
Jaiswal, M., Dudhe, R., and Sharma, P. K. (2015). Nanoemulsion: An advanced mode of drug delivery system. 3 Biotech 5, 123–127.
Jault, P., Leclerc, T., Jennes, S., Pirnay, J. P., Que, Y. A., Resch, G., et al. (2019). Efficacy and tolerability of a cocktail of bacteriophages to treat burn wounds infected by Pseudomonas aeruginosa (PhagoBurn): A randomised, controlled, double-blind phase 1/2 trial. Lancet Infect. Dis. 19, 35–45. doi: 10.1016/S1473-3099(18)30482-1
Jonczyk-Matysiak, E., Lodej, N., Kula, D., Owczarek, B., Orwat, F., Miedzybrodzki, R., et al. (2019). Factors determining phage stability/activity: Challenges in practical phage application. Expert Rev. Anti. Infect. Ther. 17, 583–606. doi: 10.1080/14787210.2019.1646126
Jones, A., and Vaughan, D. (2005). Hydrogel dressings in the management of a variety of wound types. J. Orthop. Nurs. 9, S1–S11.
Karn, S. L., Bhartiya, S. K., Pratap, A., Saroj, S. K., Kumar, R., Sahu, M., et al. (2024). A randomized, placebo-controlled, double-blind clinical trial of bacteriophage cocktails in chronic wound infections. Int. J. Low Extrem Wounds Online ahead of print. doi: 10.1177/15347346231226342
Kaur, J., Kour, A., Panda, J. J., Harjai, K., and Chhibber, S. (2020). Exploring endolysin-loaded alginate-chitosan nanoparticles as future remedy for staphylococcal infections. AAPS PharmSciTech 21:233. doi: 10.1208/s12249-020-01763-4
Kaur, P., Gondil, V. S., and Chhibber, S. (2019). A novel wound dressing consisting of PVA-SA hybrid hydrogel membrane for topical delivery of bacteriophages and antibiotics. Int. J. Pharm. 572:118779. doi: 10.1016/j.ijpharm.2019.118779
Kaur, S., Kumari, A., Kumari Negi, A., Galav, V., Thakur, S., Agrawal, M., et al. (2021). Nanotechnology based approaches in phage therapy: Overcoming the pharmacological barriers. Front. Pharmacol. 12:699054. doi: 10.3389/fphar.2021.699054
Khazani Asforooshani, M., Elikaei, A., Abed, S., Shafiei, M., Barzi, S. M., Solgi, H., et al. (2024). A novel Enterococcus faecium phage EF-M80: Unveiling the effects of hydrogel-encapsulated phage on wound infection healing. Front. Microbiol. 15:1416971. doi: 10.3389/fmicb.2024.1416971
Kielholz, T., Rohde, F., Jung, N., and Windbergs, M. (2023). Bacteriophage-loaded functional nanofibers for treatment of P. aeruginosa and S. aureus wound infections. Sci. Rep. 13:8330. doi: 10.1038/s41598-023-35364-5
Kim, H. Y., Chang, R. Y. K., Morales, S., and Chan, H. K. (2021). Bacteriophage-delivering hydrogels: Current progress in combating antibiotic resistant bacterial infection. Antibiotics (Basel) 10:130. doi: 10.3390/antibiotics10020130
Korehei, R., and Kadla, J. (2013). Incorporation of T4 bacteriophage in electrospun fibres. J. Appl. Microbiol. 114, 1425–1434.
Korehei, R., and Kadla, J. F. (2014). Encapsulation of T4 bacteriophage in electrospun poly(ethylene oxide)/cellulose diacetate fibers. Carbohydr. Polym. 100, 150–157. doi: 10.1016/j.carbpol.2013.03.079
Kuhn, A., Haase, M., and Leptihn, S. (2017). Assisted and unassisted protein insertion into liposomes. Biophys. J. 113, 1187–1193.
Kumari, S., Harjai, K., and Chhibber, S. (2010). Topical treatment of Klebsiella pneumoniae B5055 induced burn wound infection in mice using natural products. J. Infect. Dev. Ctries 4, 367–377.
Kumari, S., Harjai, K., and Chhibber, S. (2011). Bacteriophage versus antimicrobial agents for the treatment of murine burn wound infection caused by Klebsiella pneumoniae B5055. J. Med. Microbiol. 60, 205–210. doi: 10.1099/jmm.0.018580-0
Leptihn, S. (2019). Welcome back to the pre-penicillin era. why we desperately need new strategies in the battle against bacterial pathogens. Infectious Microbes Dis. 1:33.
Li, C., Nyaruaba, R., Zhao, X., Xue, H., Li, Y., Yang, H., et al. (2022). Thermosensitive hydrogel wound dressing loaded with bacteriophage Lysin LysP53. Viruses 14:1956. doi: 10.3390/v14091956
Liang, S., Qi, Y., Yu, H., Sun, W., Raza, S. H. A., Alkhorayef, N., et al. (2023). Bacteriophage therapy as an application for bacterial infection in China. Antibiotics (Basel) 12:417. doi: 10.3390/antibiotics12020417
Lin, Y. H., Dharmaraj, T., Chen, Q., Echterhof, A., Manasherob, R., Zheng, L. J., et al. (2024). Hydrogels for local and sustained delivery of bacteriophages to treat multidrug-resistant wound infections. bioRxiv [Preprint] doi: 10.1101/2024.05.07.593005
Liu, M., Wei, X., Zheng, Z., Li, Y., Li, M., Lin, J., et al. (2023). Recent advances in nano-drug delivery systems for the treatment of diabetic wound healing. Int. J. Nanomedicine 18, 1537–1560.
Mabrouk, S. M., Abdellatif, G. R., Abu Zaid, A. S., Aziz, R. K., and Aboshanab, K. M. (2022). In vitro and pre-clinical evaluation of locally isolated phages, vB_Pae_SMP1 and vB_Pae_SMP5, formulated as hydrogels against carbapenem-resistant Pseudomonas aeruginosa. Viruses 14:2760. doi: 10.3390/v14122760
Manohar, P., Loh, B., and Leptihn, S. (2020). Will the overuse of antibiotics during the coronavirus pandemic accelerate antimicrobial resistance of bacteria? Infectious Microbes Dis. 2, 87–88. doi: 10.1097/IM9.0000000000000034
Marianecci, C., Di Marzio, L., Rinaldi, F., Celia, C., Paolino, D., Alhaique, F., et al. (2014). Niosomes from 80s to present: The state of the art. Adv. Colloid. Interface Sci. 205, 187–206. doi: 10.1016/j.cis.2013.11.018
McClements, D. J., and Rao, J. (2011). Food-grade nanoemulsions: Formulation, fabrication, properties, performance, biological fate, and potential toxicity. Crit. Rev. Food Sci. Nutr. 51, 285–330. doi: 10.1080/10408398.2011.559558
Merabishvili, M., Monserez, R., Van Belleghem, J., Rose, T., Jennes, S., De Vos, D., et al. (2017). Stability of bacteriophages in burn wound care products. PLoS One 12:e0182121. doi: 10.1371/journal.pone.0182121
Miao, J., Pangule, R. C., Paskaleva, E. E., Hwang, E. E., Kane, R. S., Linhardt, R. J., et al. (2011). Lysostaphin-functionalized cellulose fibers with antistaphylococcal activity for wound healing applications. Biomaterials 32, 9557–9567. doi: 10.1016/j.biomaterials.2011.08.080
Moghadam, M. T., Mojtahedi, A., Salamy, S., Shahbazi, R., Satarzadeh, N., Delavar, M., et al. (2024). Phage therapy as a glimmer of hope in the fight against the recurrence or emergence of surgical site bacterial infections. Infection 52, 385–402.
Morais, D., Tanoeiro, L., Marques, A. T., Goncalves, T., Duarte, A., Matos, A. P. A., et al. (2022). Liposomal delivery of newly identified prophage Lysins in a Pseudomonas aeruginosa model. Int. J. Mol. Sci. 23:10143. doi: 10.3390/ijms231710143
Mukhopadhyay, S., To, K. K. W., Liu, Y., Bai, C., and Leung, S. S. Y. (2023). A thermosensitive hydrogel formulation of phage and colistin combination for the management of multidrug-resistant Acinetobacter baumannii wound infections. Biomater. Sci. 12, 151–163. doi: 10.1039/d3bm01383a
Mutti, M., and Corsini, L. (2019). Robust approaches for the production of active ingredient and drug product for human phage therapy. Front. Microbiol. 10:2289. doi: 10.3389/fmicb.2019.02289
Nahid, M. A., Griffin, J. M., Lustik, M. B., Hayes, J. J., Fong, K. S. K., Horseman, T. S., et al. (2021). A longitudinal evaluation of the bacterial pathogens colonizing chronic non-healing wound sites at a United States military treatment facility in the pacific region. Infect. Drug Resist. 14, 1–10. doi: 10.2147/IDR.S260708
Narayanan, K. B., Bhaskar, R., Choi, S. M., and Han, S. S. (2024). Development of carrageenan-immobilized lytic coliphage vB_Eco2571-YU1 hydrogel for topical delivery of bacteriophages in wound dressing applications. Int. J. Biol. Macromol. 259:129349.
Nour El-Din, H. T., Elhosseiny, N. M., El-Gendy, M. A., Mahmoud, A. A., Hussein, M. M. M., and Attia, A. S. (2020). A rapid Lysostaphin production approach and a convenient novel Lysostaphin loaded nano-emulgel; As a sustainable low-cost methicillin-resistant Staphylococcus aureus combating platform. Biomolecules 10:435. doi: 10.3390/biom10030435
Osborne, D., Tan, P., Varma, Y., and Carbol, J. (2018). Formulating topical products containing live microorganisms as the active ingredient. Pharmaceutical Technol. 42, 32–36.
Otto, A., Du Plessis, J., and Wiechers, J. W. (2009). Formulation effects of topical emulsions on transdermal and dermal delivery. Int. J. Cosmet. Sci. 31, 1–19.
Peng, H., Rossetto, D., Mansy, S. S., Jordan, M. C., Roos, K. P., and Chen, I. A. (2022). Treatment of wound infections in a mouse model using Zn(2+)-releasing phage bound to gold nanorods. ACS Nano 16, 4756–4774. doi: 10.1021/acsnano.2c00048
Pinto, A. M., Cerqueira, M. A., Banobre-Lopes, M., Pastrana, L. M., and Sillankorva, S. (2020). Bacteriophages for chronic wound treatment: From traditional to novel delivery systems. Viruses 12:235. doi: 10.3390/v12020235
Pinto, A. M., Silva, M. D., Pastrana, L. M., Banobre-Lopez, M., and Sillankorva, S. (2021). The clinical path to deliver encapsulated phages and lysins. FEMS Microbiol. Rev. 45:fuab019.
Portilla, S., Fernandez, L., Gutierrez, D., Rodriguez, A., and Garcia, P. (2020). Encapsulation of the antistaphylococcal endolysin LysRODI in pH-sensitive liposomes. Antibiotics (Basel) 9:242. doi: 10.3390/antibiotics9050242
Prokopczuk, F. I., Im, H., Campos-Gomez, J., Orihuela, C. J., and Martinez, E. (2023). Engineered Superinfective Pf phage prevents dissemination of Pseudomonas aeruginosa in a mouse burn model. mBio 14:e0047223. doi: 10.1128/mbio.00472-23
Puapermpoonsiri, U., Spencer, J., and Van Der Walle, C. F. (2009). A freeze-dried formulation of bacteriophage encapsulated in biodegradable microspheres. Eur. J. Pharm. Biopharm. 72, 26–33.
Raducu, L., Moraru, O. E., Gheoca-Mutu, D. E., Peligrad, T., Tigaran, A. E., Abu-Baker, A., et al. (2024). Confronting a new challenge in plastic surgery: MDR infections in patients with chronic wounds. Life (Basel) 14:444. doi: 10.3390/life14040444
Rahman, M. U., Wang, W., Sun, Q., Shah, J. A., Li, C., Sun, Y., et al. (2021). Endolysin, a promising solution against antimicrobial resistance. Antibiotics (Basel) 10:1277.
Rodriguez-Rubio, L., Chang, W. L., Gutierrez, D., Lavigne, R., Martinez, B., Rodriguez, A., et al. (2016). ‘Artilysation’ of endolysin lambdaSa2lys strongly improves its enzymatic and antibacterial activity against streptococci. Sci. Rep. 6:35382. doi: 10.1038/srep35382
Rosner, D., and Clark, J. (2021). Formulations for bacteriophage therapy and the potential uses of immobilization. Pharmaceuticals (Basel) 14:359. doi: 10.3390/ph14040359
Sarhan, W. A., and Azzazy, H. M. (2017). Apitherapeutics and phage-loaded nanofibers as wound dressings with enhanced wound healing and antibacterial activity. Nanomedicine (Lond) 12, 2055–2067. doi: 10.2217/nnm-2017-0151
Sarhan, W. A., Salem, H. G., Khalil, M. A. F., El-Sherbiny, I. M., and Azzazy, H. M. E. (2021). Fabrication of gelatin/silk fibroin/phage nanofiber scaffold effective against multidrug resistant Pseudomonas aeruginosa. Drug. Dev. Ind. Pharm. 47, 947–953. doi: 10.1080/03639045.2021.1957915
Sercombe, L., Veerati, T., Moheimani, F., Wu, S. Y., Sood, A. K., and Hua, S. (2015). Advances and challenges of liposome assisted drug delivery. Front. Pharmacol. 6:286. doi: 10.3389/fphar.2015.00286
Shafigh Kheljan, F., Sheikhzadeh Hesari, F., Aminifazl, M. S., Skurnik, M., Gholadze, S., and Zarrini, G. (2023). Design of phage-cocktail-containing hydrogel for the treatment of Pseudomonas aeruginosa-Infected wounds. Viruses 15:803. doi: 10.3390/v15030803
Shaikh, R., Raj Singh, T. R., Garland, M. J., Woolfson, A. D., and Donnelly, R. F. (2011). Mucoadhesive drug delivery systems. J. Pharm. Bioallied Sci. 3, 89–100.
Shen, H. Y., Liu, Z. H., Hong, J. S., Wu, M. S., Shiue, S. J., and Lin, H. Y. (2021). Controlled-release of free bacteriophage nanoparticles from 3D-plotted hydrogel fibrous structure as potential antibacterial wound dressing. J. Control Release 331, 154–163. doi: 10.1016/j.jconrel.2021.01.024
Shiue, S. J., Wu, M. S., Chiang, Y. H., and Lin, H. Y. (2024). Bacteriophage-cocktail hydrogel dressing to prevent multiple bacterial infections and heal diabetic ulcers in mice. J Biomed Mater Res A. 112, 1846–1859. doi: 10.1002/jbm.a.37728
Steele, A., Stacey, H. J., De Soir, S., and Jones, J. D. (2020). The safety and efficacy of phage therapy for superficial bacterial infections: A systematic review. Antibiotics (Basel) 9:754. doi: 10.3390/antibiotics9110754
Stroup, D. F., Berlin, J. A., Morton, S. C., Olkin, I., Williamson, G. D., Rennie, D., et al. (2000). Meta-analysis of observational studies in epidemiology: A proposal for reporting. meta-analysis of observational studies in epidemiology (MOOSE) group. JAMA 283, 2008–2012.
Suchithra, K. V., Hameed, A., Surya, S., Mahammad, S., and Arun, A. B. (2024). Dual phage-incorporated electrospun polyvinyl alcohol-eudragit nanofiber matrix for rapid healing of diabetic wound infected by Pseudomonas aeruginosa and Staphylococcus aureus. Drug Deliv. Transl. Res. doi: 10.1007/s13346-024-01660-4
Sweere, J. M., Van Belleghem, J. D., Ishak, H., Bach, M. S., Popescu, M., Sunkari, V., et al. (2019). Bacteriophage trigger antiviral immunity and prevent clearance of bacterial infection. Science 363:eaat9691. doi: 10.1126/science.aat9691
Tayeb, H. H., and Sainsbury, F. (2018). Nanoemulsions in drug delivery: Formulation to medical application. Nanomedicine (Lond) 13, 2507–2525.
Vacek, L., Polastik Kleknerova, D., Lipovy, B., Holoubek, J., Matyskova, D., Cerna, E., et al. (2024). Phage therapy combined with Gum Karaya injectable hydrogels for treatment of methicillin-resistant Staphylococcus aureus deep wound infection in a porcine model. Int. J. Pharm. 660:124348. doi: 10.1016/j.ijpharm.2024.124348
Wang, B., Du, L., Dong, B., Kou, E., Wang, L., and Zhu, Y. (2024). Current knowledge and perspectives of phage therapy for combating refractory wound infections. Int. J. Mol. Sci. 25:5465. doi: 10.3390/ijms25105465
Wang, J., Zhao, S., Chen, J., Liu, X., Chen, H., Lu, T., et al. (2023). Phage-Ce6-Manganese dioxide nanocomposite-mediated photodynamic, photothermal, and chemodynamic therapies to eliminate biofilms and improve wound healing. ACS Appl. Mater Interfaces 15, 21904–21916. doi: 10.1021/acsami.3c01762
Yan, W., Banerjee, P., Liu, Y., Mi, Z., Bai, C., Hu, H., et al. (2021). Development of thermosensitive hydrogel wound dressing containing Acinetobacter baumannii phage against wound infections. Int. J. Pharm. 602:120508. doi: 10.1016/j.ijpharm.2021.120508
Yang, H., Linden, S. B., Wang, J., Yu, J., Nelson, D. C., and Wei, H. (2015). A chimeolysin with extended-spectrum streptococcal host range found by an induced lysis-based rapid screening method. Sci. Rep. 5:17257. doi: 10.1038/srep17257
Yao, F., Wu, X., Liao, Y., Yan, Q., and Li, Y. (2021). Smart chimeric lysin ClyC loaded alginate hydrogel reduces Staphylococcus aureus induced bone infection. Front. Mater. 8:763297. doi: 10.3389/fmats.2021.763297
Zhang, J., Ge, J., Xu, Y., Chen, J., Zhou, A., Sun, L., et al. (2020). Bioactive multi-engineered hydrogel offers simultaneous promise against antibiotic resistance and wound damage. Int. J. Biol. Macromol. 164, 4466–4474. doi: 10.1016/j.ijbiomac.2020.08.247
Keywords: wound, phage, lysin, multidrug-resistant, therapy
Citation: Yang P, Li J, Ma X, Hu N, Song Z, Chen B and Li S (2025) Novel delivery systems for phages and lysins in the topical management of wound infections: a narrative review. Front. Microbiol. 16:1526096. doi: 10.3389/fmicb.2025.1526096
Received: 20 November 2024; Accepted: 08 January 2025;
Published: 27 January 2025.
Edited by:
Thamir A. Alandijany, King Abdulaziz University, Saudi ArabiaReviewed by:
Sudhir Kumar, Iowa State University, United StatesElena Criscuolo, Vita-Salute San Raffaele University, Italy
Copyright © 2025 Yang, Li, Ma, Hu, Song, Chen and Li. This is an open-access article distributed under the terms of the Creative Commons Attribution License (CC BY). The use, distribution or reproduction in other forums is permitted, provided the original author(s) and the copyright owner(s) are credited and that the original publication in this journal is cited, in accordance with accepted academic practice. No use, distribution or reproduction is permitted which does not comply with these terms.
*Correspondence: Bin Chen, WGlhb2NjMTk3OUAxNjMuY29t; Shizhu Li, bGlzaGl6aHVAY3FtdS5lZHUuY24=
†These authors have contributed equally to this work