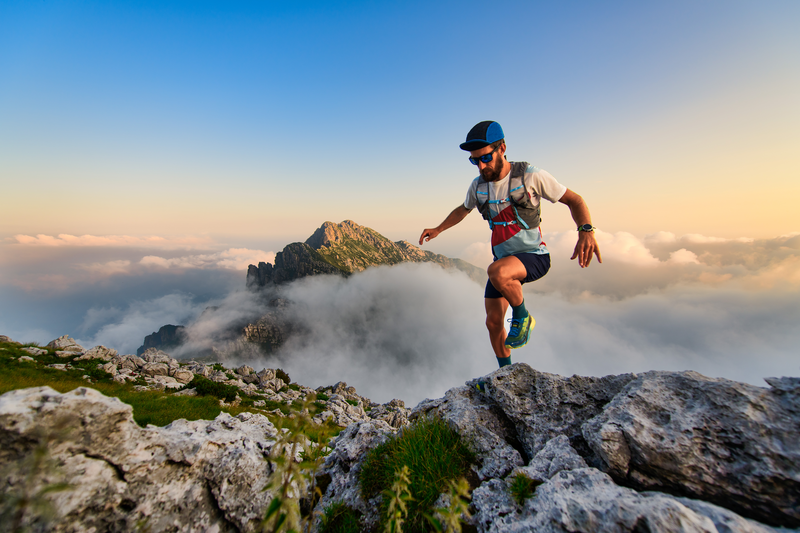
94% of researchers rate our articles as excellent or good
Learn more about the work of our research integrity team to safeguard the quality of each article we publish.
Find out more
ORIGINAL RESEARCH article
Front. Microbiol.
Sec. Microorganisms in Vertebrate Digestive Systems
Volume 16 - 2025 | doi: 10.3389/fmicb.2025.1504264
This article is part of the Research Topic Gut Microbiota Modulation to Mitigate Stress-Induced Functional Changes View all 10 articles
The final, formatted version of the article will be published soon.
You have multiple emails registered with Frontiers:
Please enter your email address:
If you already have an account, please login
You don't have a Frontiers account ? You can register here
Long photoperiods are often characterized by enhanced oxidative stress-induced damage to skeletal muscle, reduced melatonin (MT) levels and intestinal microbiota dysfunction in broilers. In this study, we aimed to investigate the association of breast muscle morphology with melatonin levels and the cecal microbiota of broilers under different photoperiods. A total of 216 healthy 5-day-old Arbor Acres (AA) male broilers were randomly assigned to 12L:12D, 18L:6D and 24L:0D photoperiods for 4 weeks (L = hours of light, D = hours of darkness). The concentration of inflammatory factors and MT concentrations was measured using ELISA kits, whereas breast muscle morphology was examined through the hematoxylin (H) and eosin (E) staining, and microbiota composition was identified through 16s rRNA analysis. Extended light exposure significantly improved the growth rate of broilers, but significantly decreased feed efficiency (FE). Furthermore, it upregulated the concentration of IL-1a, IL-6 and TNF-a and induced an abnormal breast muscle morphology. Extended light exposure significantly decreased MT levels in the hypothalamus, cecum and breast muscle, while triggering the cecal microbiota composition disorder. Specifically, there was significant alteration to the dominant bacterial phylum, following exposure to long photoperiods, with the abundance of Firmicutes decreasing and the abundance of Bacteroidota increasing. Notably, the relative abundance of Lactobacillus showed a positive correlation with MT levels and a negative correlation with inflammatory cytokines. In conclusion, the present findings indicated that extended light exposure reduced the MT levels, which were related to disturbed cecal microbiota, damaging breast muscle morphology and inducing breast muscle inflammation in broilers.
Keywords: photoperiod, Melatonin, Cecal microbiota, breast muscle morphology, Inflammation
Received: 30 Sep 2024; Accepted: 10 Mar 2025.
Copyright: © 2025 Yu, Xu, Wang, Feng and Zhang. This is an open-access article distributed under the terms of the Creative Commons Attribution License (CC BY). The use, distribution or reproduction in other forums is permitted, provided the original author(s) or licensor are credited and that the original publication in this journal is cited, in accordance with accepted academic practice. No use, distribution or reproduction is permitted which does not comply with these terms.
* Correspondence:
Minhong Zhang, Institute of Animal Sciences, Chinese Academy of Agricultural Sciences, Beijing, China
Disclaimer: All claims expressed in this article are solely those of the authors and do not necessarily represent those of their affiliated organizations, or those of the publisher, the editors and the reviewers. Any product that may be evaluated in this article or claim that may be made by its manufacturer is not guaranteed or endorsed by the publisher.
Research integrity at Frontiers
Learn more about the work of our research integrity team to safeguard the quality of each article we publish.