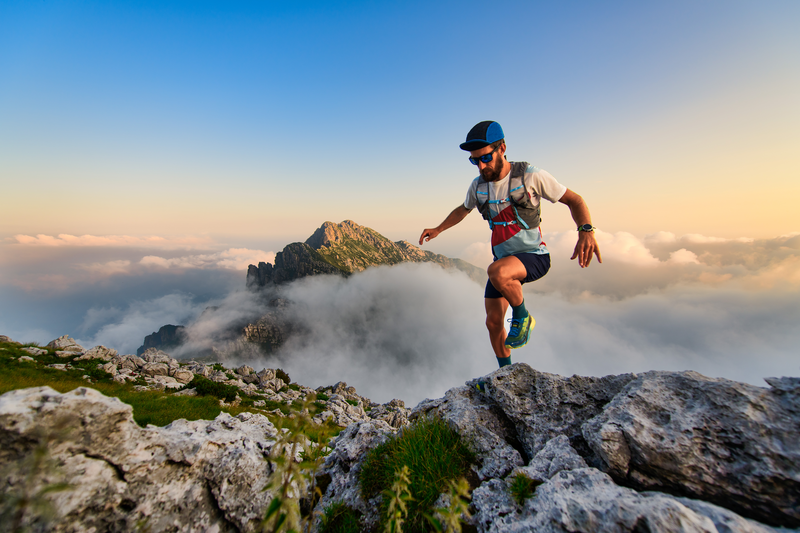
94% of researchers rate our articles as excellent or good
Learn more about the work of our research integrity team to safeguard the quality of each article we publish.
Find out more
ORIGINAL RESEARCH article
Front. Microbiol.
Sec. Terrestrial Microbiology
Volume 16 - 2025 | doi: 10.3389/fmicb.2025.1496385
This article is part of the Research Topic Microorganisms in Agricultural Soil: Advances and Challenges of Biological Health View all 8 articles
The final, formatted version of the article will be published soon.
You have multiple emails registered with Frontiers:
Please enter your email address:
If you already have an account, please login
You don't have a Frontiers account ? You can register here
As is well known, continuous cropping can lead to a decrease in crop yield and quality. Despite this, continuous cropping remains prevalent in practical agricultural production, particularly in the case of tobacco cultivation, owing to its high economic value.The samples for this study were collected from a flue-cured tobacco planting base located in Huili County, Liangshan Yi Autonomous Prefecture, Sichuan Province, China. After years of continuous planting, the yield of tobacco in this base has significantly decreased. In order to explain the microecological causes of this phenomenon, we collected non-continuous cropping, continuous cropping for 5 years, and continuous cropping for 10 years of tobacco rhizosphere soil, and analyzed the effects of long-term continuous cropping on nutrients, enzyme activities, microbial community structure and function of tobacco rhizosphere soil. The results showed that with the continuous cropping, the majority nutrients (except for phosphorus and manganese) in rhizosphere soil decreased significantly, and the rhizosphere microbial community structure changed significantly. Correlation network analysis results showed that changes in the rhizosphere microbial community of tobacco were closely related to soil urease, active organic carbon, and available iron content. The results of functional analysis based on microorganisms and genes showed that the rhizosphere microbiota may change the content of soil nutrients through iron_respiration, sulfur_respiration and Carbon fixation in prokaryotes pathways. The results of the correlation network analysis and the functional analysis mutually confirmed each other, both emphasizing the important role of soil carbon and iron in shaping the structure of the tobacco rhizosphere microbial community. Based on the results of this study, we propose to improve the microbial community structure of tobacco rhizosphere soil by increasing the levels of readily oxidizable organic carbon, available iron, and soil urease activity in the future, so as to alleviate the negative impact of continuous cropping on crop yield. The results of this study provide theoretical support for modifying the rhizosphere microbial environment through nutrient regulation, thereby enhancing plant growth in the context of continuous tobacco cropping.
Keywords: continuous cropping, Metagenomics, Soil nutrients and enzymes, soil microbial community, Soil microbial function
Received: 14 Sep 2024; Accepted: 18 Feb 2025.
Copyright: © 2025 Yang, Feng, Jiang, Chen, Ding, Dai, Zhai, Yang, Liang and Zhang. This is an open-access article distributed under the terms of the Creative Commons Attribution License (CC BY). The use, distribution or reproduction in other forums is permitted, provided the original author(s) or licensor are credited and that the original publication in this journal is cited, in accordance with accepted academic practice. No use, distribution or reproduction is permitted which does not comply with these terms.
* Correspondence:
Yanling Zhang, Zhengzhou Tobacco Research Institute of CNTC, Zhengzhou, China
Disclaimer: All claims expressed in this article are solely those of the authors and do not necessarily represent those of their affiliated organizations, or those of the publisher, the editors and the reviewers. Any product that may be evaluated in this article or claim that may be made by its manufacturer is not guaranteed or endorsed by the publisher.
Research integrity at Frontiers
Learn more about the work of our research integrity team to safeguard the quality of each article we publish.