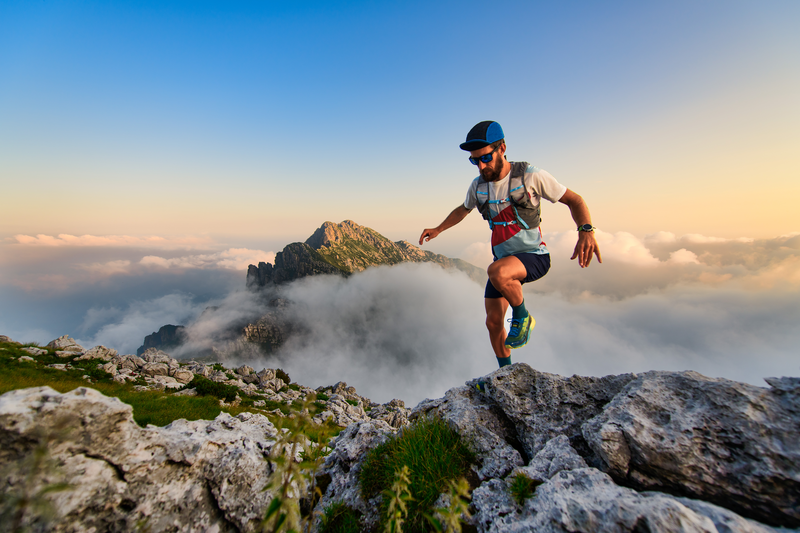
94% of researchers rate our articles as excellent or good
Learn more about the work of our research integrity team to safeguard the quality of each article we publish.
Find out more
ORIGINAL RESEARCH article
Front. Microbiol.
Sec. Virology
Volume 16 - 2025 | doi: 10.3389/fmicb.2025.1468530
This article is part of the Research Topic Transmission and Infection of Arboviruses – Volume II View all 10 articles
The final, formatted version of the article will be published soon.
You have multiple emails registered with Frontiers:
Please enter your email address:
If you already have an account, please login
You don't have a Frontiers account ? You can register here
The West Nile virus (WNV) is a mosquito-borne virus of the Flaviviridae family that is transmitted through the mosquito-migratory bird-mosquito cycle. Currently, WNV infection is widespread in the Americas, Europe, and Africa, and is one of the most important global epidemic infectious diseases. Although migratory birds play an important role in the spread of WNV, monitoring of migratory birds carrying the WNV remains limited. Here, we developed a new nucleic acid test for detecting migratory birds carrying WNV, which uses amplicons of WNV to test fecal samples from migratory birds. This new method was validated by using full-length WNV genomic plasmid. With this amplicon method, we tested the migratory bird droppings collected in different locations. The results indicated that the positive rate of WNV nucleic acid in migratory bird droppings was over 39%, which provides clues to the fact that migratory birds may carry the WNV in Xinjiang, China.
Keywords: West Nile virus, migratory bird, amplicon, Fecal samples, Epidemiology
Received: 22 Jul 2024; Accepted: 07 Mar 2025.
Copyright: © 2025 Kunsheng, He, Zhang, Li, Donghua, Wei, Li, Qiu, Ma and Liu. This is an open-access article distributed under the terms of the Creative Commons Attribution License (CC BY). The use, distribution or reproduction in other forums is permitted, provided the original author(s) or licensor are credited and that the original publication in this journal is cited, in accordance with accepted academic practice. No use, distribution or reproduction is permitted which does not comply with these terms.
* Correspondence:
Ke Liu, Shanghai Veterinary Research Institute, Chinese Academy of Agricultural Sciences, Shanghai, China
Disclaimer: All claims expressed in this article are solely those of the authors and do not necessarily represent those of their affiliated organizations, or those of the publisher, the editors and the reviewers. Any product that may be evaluated in this article or claim that may be made by its manufacturer is not guaranteed or endorsed by the publisher.
Research integrity at Frontiers
Learn more about the work of our research integrity team to safeguard the quality of each article we publish.