- 1Universidade de Brasília, Brasília, Brazil
- 2Embrapa Recursos Genéticos e Biotecnologia, Brasília, Brazil
- 3Department of Natural Sciences, Bowie State University, Bowie, MD, United States
- 4Escuela de Biología and Centro de Investigaciones en Productos Naturales CIPRONA, Universidad de Costa Rica, San José, Costa Rica
Trichoderma spp. are important biological control agents and plant growth promoters. However, only a limited number of species are used in biological control even though the genus contains more than 400 species, with most of them being mycotrophic. In this study, 97 Trichoderma isolates preserved at the EMBRAPA collection (an important source for biocontrol agents) and previously collected from several areas in Brazil were characterized which were identified using various molecular markers (internal transcribed spacers (its), translation elongation factor (tef1α), RNA polymerase II subunit (rpb2), actin (act), and calmodulin (cal). Of these, 54 isolates were found to group in the Harzianum species complex and 32 in Sect. Trichoderma. Others were distributed in the following clades: Strictipilosa complex (one isolate), Longibrachiatum (four isolates), and Brevicompactum (seven isolates). Most of the isolates were identified within 17 known species, whereas Trichoderma inhamatum and T. dorothopsis were synonymized under T. lentiforme and T. koningiopsis, respectively, based on multi-locus phylogenetic analysis and GCPRS criteria. However, two isolates formed a clade apart from previously identified species from Sect. Trichoderma and identified as a new species: T. cerradensis sp. nov. The multigenic characterization of isolates deposited in fungal culture collections is crucial for accurate identification and reveals a diverse range of Trichoderma species in Brazil.
1 Introduction
Trichoderma Persoon (1794) (=Hypocrea) contains mycotrophic and saprotrophic fungal species that can be found in diverse habitats such as leaf-cutting ant nests, soil, rhizosphere, decomposing plant material, fungal sporocarps, and as endophytes, and in multiple geographic regions, from the Arctic to the Tropics (Chaverri and Samuels, 2003; Hughes et al., 2007; Montoya et al., 2016). These fungi exhibit a myriad of applications, owing to the diverse array of research fields that have utilized them over the past century (Dou et al., 2020). Several species are being studied for use in environmental bioremediation processes and production of heterologous enzymes of scientific and industrial interest (Tomico-Cuenca et al., 2021; Mukherjee et al., 2013). However, this genus is especially important in agriculture as biological control agents and plant growth promoters and is known to increase drought tolerance in plants (Weindling, 1932, 1934; López-Bucio et al., 2015; Mukesh et al., 2016; Ben M’henni et al., 2022). A few species have been reported to cause human diseases, especially in immunocompromised patients, while others are etiologic agents of green mold disease in mushroom cultivation (Chen and Zhuang, 2017).
The cosmopolitan nature of Trichoderma is evident in its widespread distribution. Its species can be present in the most diverse habitats, almost without restriction of environmental conditions. Studies have reported their ability to survive in various geographical areas of the planet and on different continents, i.e., Africa (Allsopp et al., 1987; du Plessis et al., 2018; del Carmen et al., 2021), Oceania (Jaklitsch and Voglmayr, 2014), Europe (Jaklitsch, 2009), Asia (Qiao et al., 2018), and America (Chaverri et al., 2015; Almeida et al., 2018; Hanada et al., 2008; Bustamante et al., 2021). There are also reports of the presence of Trichoderma in extreme conditions, such as T. koningii in Antarctic soil (Hughes et al., 2007), T. viride in ice tunnels at the North Pole (Jacobs et al., 1964), and in highly polluted environments or in kerosene tanks (Klein and Eveleigh, 1998; Druzhinina et al., 2012).
Even though Brazil is one of the most important biodiversity hotspots, few studies have assessed and characterized its Trichoderma diversity. One study identified many Trichoderma species from soil samples of garlic and onion (Inglis et al., 2020). Recently, four new species were described from Brazilian Amazon (Brito et al., 2023), while several isolates were characterized with sequences of the translation elongation factor 1-α gene region (Oliveira et al., 2023). Therefore, it is difficult to estimate the number of species present in Brazil. In a study carried out in China by Hu et al. (2020), covering 1,236 samples collected from 40 locations with diverse climatic conditions and ecosystems, 919 isolates belonging to 39 species in 9 complexes were identified, in addition to revealing another 317 isolates as potentially new species. Another study conducted in Europe with more than 650 isolates revealed greater genetic variation than previously observed. These authors found 96 species, among which 17 were considered new species (Jaklitsch and Voglmayr, 2015). Studies with this impact are important to know the variability and distribution of fungi of this genus, in addition to providing information on the ideal environmental conditions for survival of each species. Furthermore, the correct identification of the species may relate to their biological applications (Hoyos-Carvajal and Bisset, 2011).
Through sequencing of actin (act), calmodulin (cal), internal transcribed spacers (its), RNA polymerase II subunit 2 (rpb2), and translation elongation factor 1-α (tef1α), commercial isolates registered as T. harzianum in North America and Europe were reanalyzed by Chaverri et al. (2015). These authors concluded that such products were, in fact, T. afroharzianum, T. guizhouense, and T. simmonsii. No isolates of Trichoderma harzianum sensu stricto were identified among the analyzed samples. In Brazil, there are 21 Trichoderma-based biofungicides available, and 14 of these were registered as T. harzianum, 5 as T. asperellum, 1 as T. koningiopsis, and 1 as T. stromaticum (Bettiol et al., 2012, 2019). According to the product labels, T. harzianum is present as an active component in 38.8% of the products, 50% when mixed with other Trichoderma species, and up to 60% if considered in a mixture with other microorganisms (Bettiol et al., 2019). This confusion in the identification of Trichoderma species may be underestimated, considering the products currently registered as T. harzianum and the mistaken identification in other countries (Chaverri et al., 2015; Bettiol et al., 2019). Probably, the identification of such isolates occurred at times before the emergence of modern sequencing techniques and multi-loci analysis and was based primarily on morphological characteristics. Therefore, a review of the identification and the reclassification of these isolates is urgently needed. Especially in the case of species that will compose new commercial products, at least the tef1α and rpb2 (Atanasova et al., 2013) should be used to confidently identify the active ingredients. In view of the above, the objective of this study was to correctly identify and characterize the Trichoderma isolates kept in the Empresa Brasileira de Pesquisa Agropecuaria (EMBRAPA) collection, an important source of biocontrol agents, which were previously isolated from different locations in Brazil.
2 Materials and methods
2.1 Source of isolates
Ninety-seven Trichoderma isolates from the EMBRAPA Biological Control Agents Collection were used (Table 1). The isolates were collected from different geographical areas of Brazil. The cultures were kept in liquid nitrogen. For this study, they were reactivated in potato dextrose agar (PDA) and then stored for a short period at 10°C in test tubes containing 20 mL of PDA.
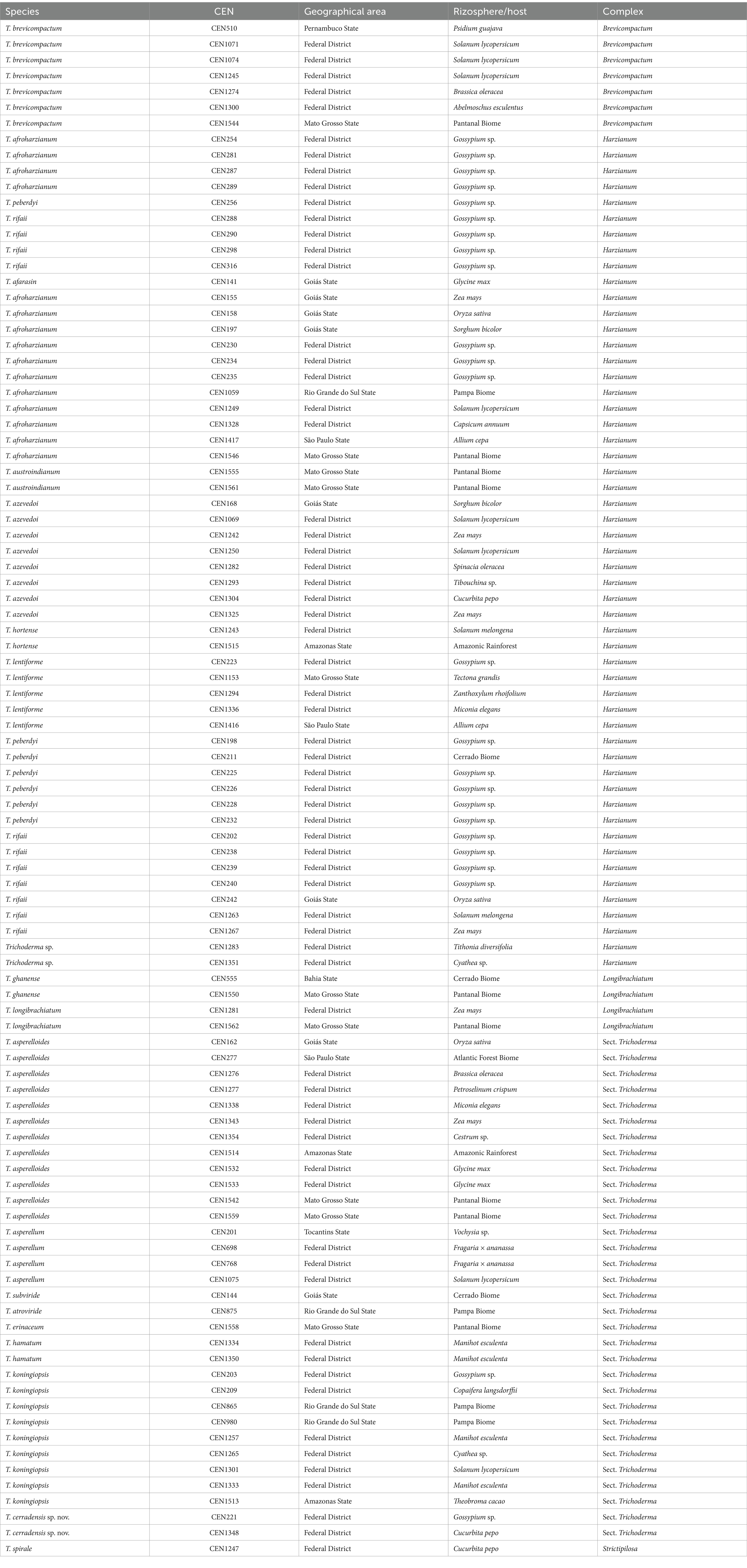
Table 1. Trichoderma species from biological control collection on EMBRAPA genetic resources and biotechnology characterized in this study.
2.2 DNA extraction, PCR, and sequencing
The isolates were grown in 50 mL Falcon tubes containing potato dextrose broth (PDB) medium (at 25°C for 5 days on a shaker Adicione one). Then, the mycelium was collected with a sterile toothpick and deposited in 1.5 mL microtubes containing 20 μL of Tris-EDTA (TE) buffer. DNA extraction was done using a Wizard Genomic DNA Purification Kit (Promega®) according to the protocol adapted by Pinho et al. (2012). The presence and the quality of genomic DNA were assessed in 1% agarose gel electrophoresis, stained with GelRed (Biotium®), and visualized under UV light. The genomic DNA was stored at −20°C for later use.
Part of the gene encoding translation elongation factor 1-alpha (TEF1α; ca. 700 bp) was amplified and sequenced for a preliminary identification as it is considered a useful secondary barcode (Druzhinina et al., 2012; Chaverri et al., 2015). After this preliminary identification, internal transcribed spacers of the nuclear ribosomal DNA (its, ca. 1,500 bp), RNA polymerase II (rpb2; ca. 900 bp), calmodulin (cal; 700 bp), and actin (act; 700 bp) were sequenced for selected isolates. The primers used are listed in Table 2. Polymerase chain reactions (PCRs) were performed in a final volume of 12.5 μL: 6.25 μL of MyTaq MasterMix 2x (Bioline, EUA), 0.3 μL (10 pmol/μL) of each primer, 4.25 μL of nuclease-free water, and 1 μL of template DNA (25 ng/μL). The cycle conditions for all markers were as follows: initial denaturation at 96°C for 5 min followed by 30 cycles at 90°C for 30 s, annealing temperature (according to Table 2) for 45 s, and 72°C for 45 s, and a final extension at 72°C for 5 min. The PCR products were purified and bidirectionally Sanger-sequenced.
2.3 Phylogenetic analyses
The quality of the sequences and contig assembly were checked, with subsequent ambiguity analysis, and, when necessary, adjusted by comparing the sense and antisense strands through DNA Dragon software.1 The TEF1α sequences were submitted to the BLASTn algorithm (Altschul et al., 1990) within the NCBI platform and compared with sequences downloaded from the GenBank nucleotide database2 found in publications to determine the origin of the species complex. Phylogenetic analyses were first performed for each gene separately. Then, multi-loci analyses were performed for each Trichoderma clade separately (e.g., Harzianum, Longibrachiatum, Brevicompactum, sect. Trichoderma, and Strictipilosa). Sequences were aligned in MAFFT v.7 (Katoh and Standley, 2013) and manually refined in MEGA v.7 software (Kumar et al., 2016). For Bayesian inference (BI), the best nucleotide substitution models for each partition were determined with MrModeltest 2.3 (Nylander, 2004). The model selected for maximum likelihood (ML) analysis was GTR + G (Stamatakis, 2014). The models were added to a command block in each corresponding matrix, which was later concatenated into a single supermatrix. To construct phylogenetic trees, MrBayes 3.1.2 was used for BI (Ronquist and Huelsenbeck, 2003) and RAxML-HPC2 8.2.12 for ML (Stamatakis, 2014) within the CIPRES Portal (Miller et al., 2010). For BI, 10 million generations were run, with sampling every 1,000 and subsequent removal of the 25% first trees in the analysis (burn-in), followed by the assembly of consensus tree using the 7,500 remaining trees and calculation of posterior probability (PP). The convergence of the log likelihoods was confirmed using TRACER v1.7.1 (Rambaut and Drummond, 2018). For ML, 1,000 bootstrap (BS) replicates were used. The trees were visualized using FigTree v.1.4 (Rambaut, 2018) and edited in graphics programs. For species attribution, the criteria of Genealogical Concordance Phylogenetic Species Recognition (GCPSR) were employed. The criteria of genealogical concordance, which is satisfied when the clade is present in the majority of individual trees, and genealogical non-discordance, which is achieved when the clade is strongly supported (≥70% for ML and ≥ 0.95 for BI), were analyzed. The new species and their synonymization were recognized when the GCPSR criteria were met.
2.4 Morphological characterization
The morphological characteristics of the colonies were determined only for the isolate CEN221, the holotype of T. cerradensis sp. nov. The growth trials were done in 90 mm diameter Petri dishes, containing 20 mL of PDA, cornmeal dextrose (CMD) agar, and synthetic nutrient agar (SNA) medium without a filter paper (Nirenberg, 1976), according to Chaverri et al. (2015). The cultures were incubated at 25°C under alternating 12-h/12-h light/darkness. Growth rate measurements (radius in mm) were recorded daily for 5 days. Culture characteristics were observed after 7 days. The micromorphological characteristics were performed based on a microculture technique, from 3-day-old colonies grown on SNA at 25°C, and conidia and conidiophores were analyzed by mounting semi-permanent slides in lactoglycerol. Thirty-five measurements for each of these morphological parameters were done at a magnification of ×1,000, using a Leica DM2500 light microscope equipped with a Leica DFC 490 digital camera, coupled to a computer containing Leica Qwin-Plus software. The average, standard deviation, and maximum and minimum values were calculated for the measurements. The dried culture of the holotype was stored at the Brasilia University (UnB) Herbarium, while ex-type cultures preserved in liquid nitrogen were kept in the Biocontrol Agents Collection at EMBRAPA Genetic Resources and Biotechnology (CENARGEN), Brasília, Federal District.
3 Results
The studied isolates were distributed in the following clades: Harzianum complex (54 isolates), Section Trichoderma (32), Brevicompactum complex (7), Longibrachiatum complex (4), and Strictipilosa complex (1). The sequences were deposited in GenBank with the following codes: its (OM515005–OM515101); tef (ON101407–ON101503); rpb2 (PP805906–PP854205, PQ149256–PQ149277, and PQ720596– PQ720597); cal (ON241149–ON241245); and act (ON311008–ON311104).
After analyzing all the single-gene phylogenetic trees for each complex (Supplementary material), it was observed that the tef1α and rpb2 regions exhibited greater species segregation. While cal and act regions could delineate certain species, they were insufficient for comprehensive species differentiation. In addition, these two regions had the fewest sequences available for comparison in GenBank. Finally, the its region failed to adequately segregate species across all complexes. When focusing solely on the isolates analyzed in this study, act, cal, rpb2, and tef1α regions effectively defined the clades, whereas its did not perform as well.
The multi-loci analysis was done for all species in each species complex. One of these, which was compared to 27 sequences available on GenBank, including accessions from Stromaticum clade, was shown to be part of the Strictipilosa complex, considering the proximity between these two species complexes. The out-group used for rooting was T. semiorbis. The concatenated gene matrix comprised 2,477 total characters, distributed among the rpb2 (811 bp), its (602 bp), tef1α (548 bp), cal (471 bp), and act (716 bp) regions, including gaps. Of the total character numbers in the concatenated matrix, 2,115 sites were conserved, 968 were variable, and 477 were phylogenetically informative. The evolutionary models selected for Bayesian inference were GTR + I + G, HKY + G, GTR + I + G, K80 + G, and GTR + I for its, tef1α, rpb2, cal, and act, respectively. In the multi-loci tree of this complex, one isolate (CEN1247) had grouped with T. spirale specimens with a high PP and BS support (Figure 1).
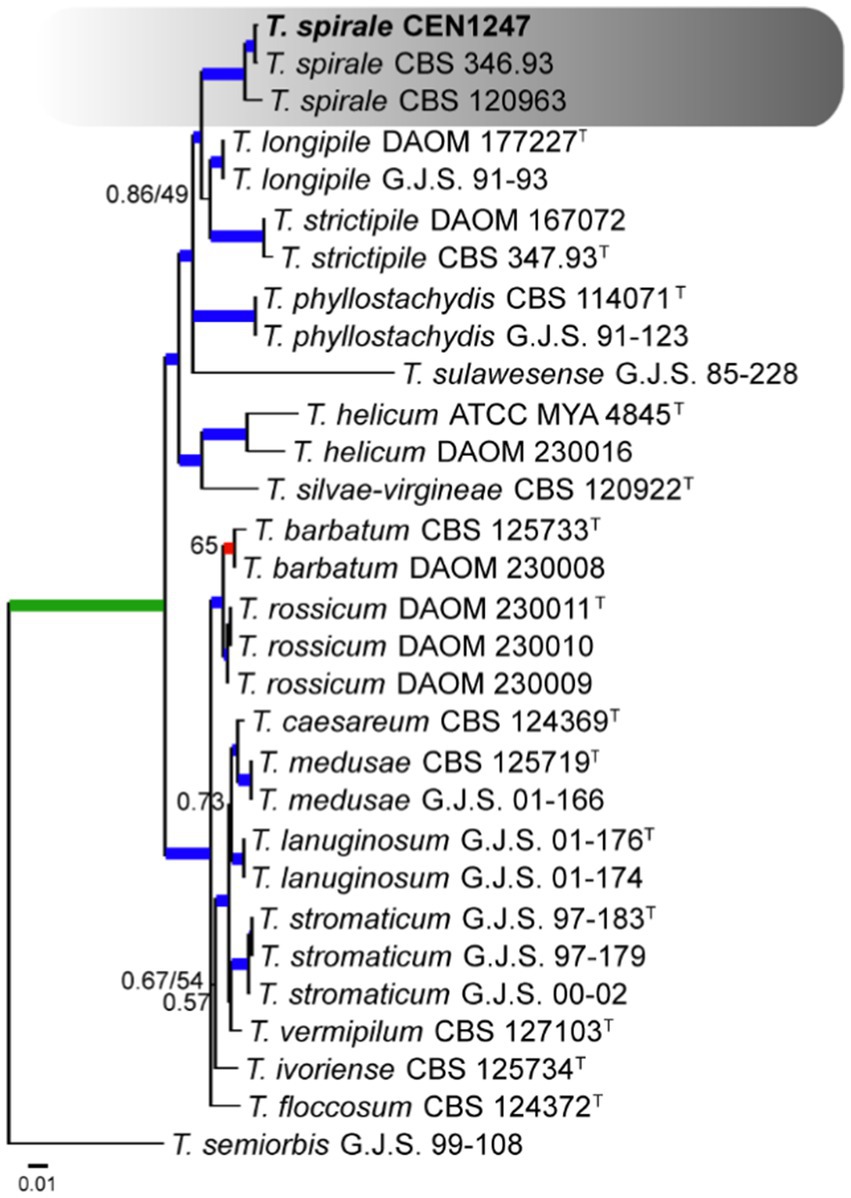
Figure 1. Bayesian phylogenetic tree based on concatenated sequences (TEF1α, ITS, RPB2, CAL, and ACT) of Strictipilosa and Stromaticum complexes. Bayesian posterior probability and maximum likelihood bootstrap support values are indicated at the nodes, and the scale bar represents the number of expected changes per site. Thickened blue lines indicate PP ≥ 0.99 and BS ≥ 95, red color indicates PP ≥ 0.99, and green color indicates BS ≥ 95. The specimen T. semiorbis G.J.S. 99–108 was used as the out-group. The strains reported here are highlighted in bold (T = Type specimen).
For the Longibrachiatum complex, four Trichoderma isolates were identified. The tree was rooted with T. barbatum. The concatenated matrix had 2,988 sites, distributed among act (718 bp), rpb2 (703 bp), tef1α (590 bp), its (572 bp), and cal (400 bp), including gaps. Of these sites, 2,104 were conserved, 840 were variable, and 647 were phylogenetically informative. The selected nucleotide substitution models for its, tef1α, rpb2, cal, and act were GTR + I, GTR + I + G, GTR + I + G, and GTR + I + G, respectively, and GTR + I under Bayesian inference and GTR + G under maximum likelihood. It was possible to identify two isolates positioned in a clade with T. longibrachiatum and two others in T. ghanense, both with PP and BS more than 0.99 and 95%, respectively (Figure 2).
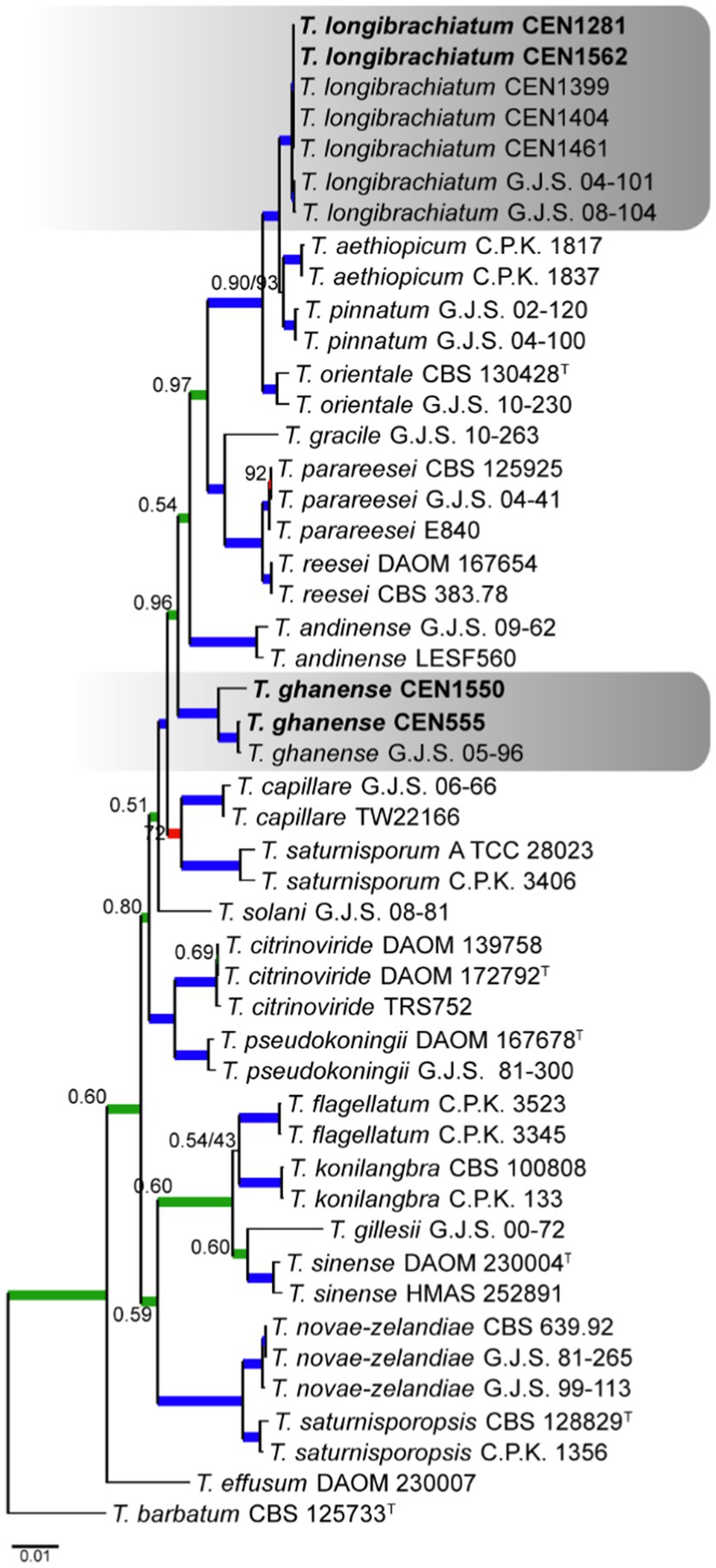
Figure 2. Bayesian phylogenetic tree based on concatenated sequences (TEF1α, ITS, RPB2, CAL, and ACT) of the Longibrachiatum complex. Bayesian posterior probability and maximum likelihood bootstrap support values are indicated at the nodes, and the scale bar represents the number of expected changes per site. Thickened blue lines indicate PP ≥ 0.99 and BS ≥ 95, red color indicates PP ≥ 0.99, and green color indicates BS ≥ 95. The specimen T. barbatum C.B.S. 125733 was used as the out-group. The strains reported here are highlighted in bold (T = Type specimen).
Seven isolates were placed in the Brevicompactum complex, for which T. minutisporum was used as the out-group. The total number of characters in the concatenated matrix was 2,970, including gaps, with 751 bp for rpb2, 652 for act, 590 bp for tef1α, 565 bp for its, and 409 bp for cal. Of the total number of characters in the matrix, 2,374 were conserved sites, 542 were variable, and 299 were phylogenetically informative. The evolutionary models selected for Bayesian inference were HKY + I, GTR + G, SYM + I, K80, and HKY, for its, tef1α, rpb2, cal, and act, respectively, while GTR + G was selected for maximum likelihood. As shown in Figure 3, it was found that all seven isolates clustered close to T. brevicompactum with high PP and BS values.
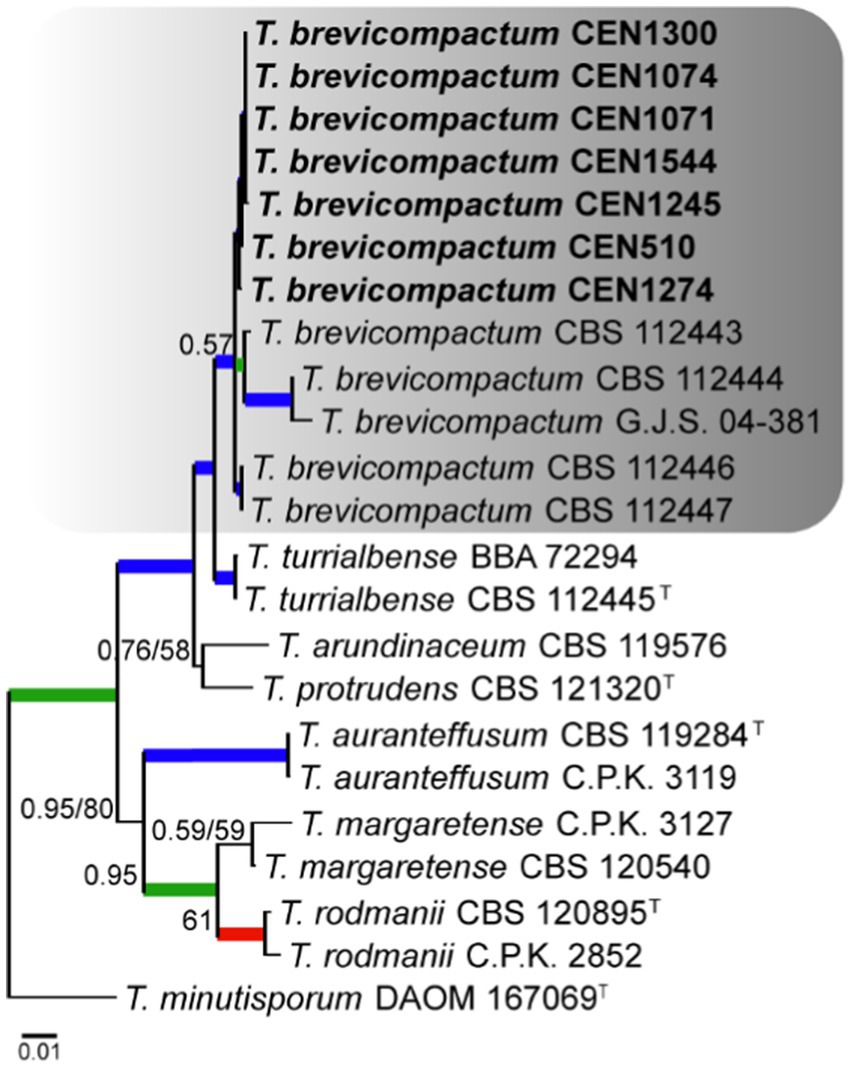
Figure 3. Bayesian phylogenetic tree based on concatenated sequences (TEF1α, ITS, RPB2, CAL, and ACT) of the Brevicompactum complex. Bayesian posterior probability and maximum likelihood bootstrap support values are indicated at the nodes, and the scale bar represents the number of expected changes per site. Thickened blue lines indicate PP ≥ 0.99 and BS ≥ 95, red color indicates PP ≥ 0.99, and green color indicates BS ≥ 95. The specimen T. minutisporum DAOM 107069 was used as the out-group. The strains reported here are highlighted in bold (T = Type specimen).
According to the initial screening done with the TEF1α region, 54 isolates grouped within the Harzianum complex using T. viride as the out-group. The concatenated matrix had 2,937 characters, with 760 for rpb2, 621 for act, 577 for tef1α, 528 for its, and 451 for cal. Of the total number of characters 1,940 were conserved sites, 894 were variable, and 580 were phylogenetically informative. The evolutionary models chosen for Bayesian inference were GTR + I + G, HKY + G, SYM + I + G, K80 + I + G, and GTR + I + G for its, tef1α, rpb2, cal, and act, respectively, and GTR + G for maximum likelihood. Interestingly, T. inhamatum CBS 273.78 was reclassified as T. lentiforme in our analysis. The isolates were identified to be clustering with T. afroharzianum (15 isolates), T. rifaii (11 isolates), T. azevedoi (8 isolates), T. peberdyi (7 isolates), T. lentiforme (5 isolates), T. austroindianum (2 isolates), T. hortense (2 isolates), and T. afarasin (one isolate) (Figure 4). Here, many of clades showed low PP values over 0.75.
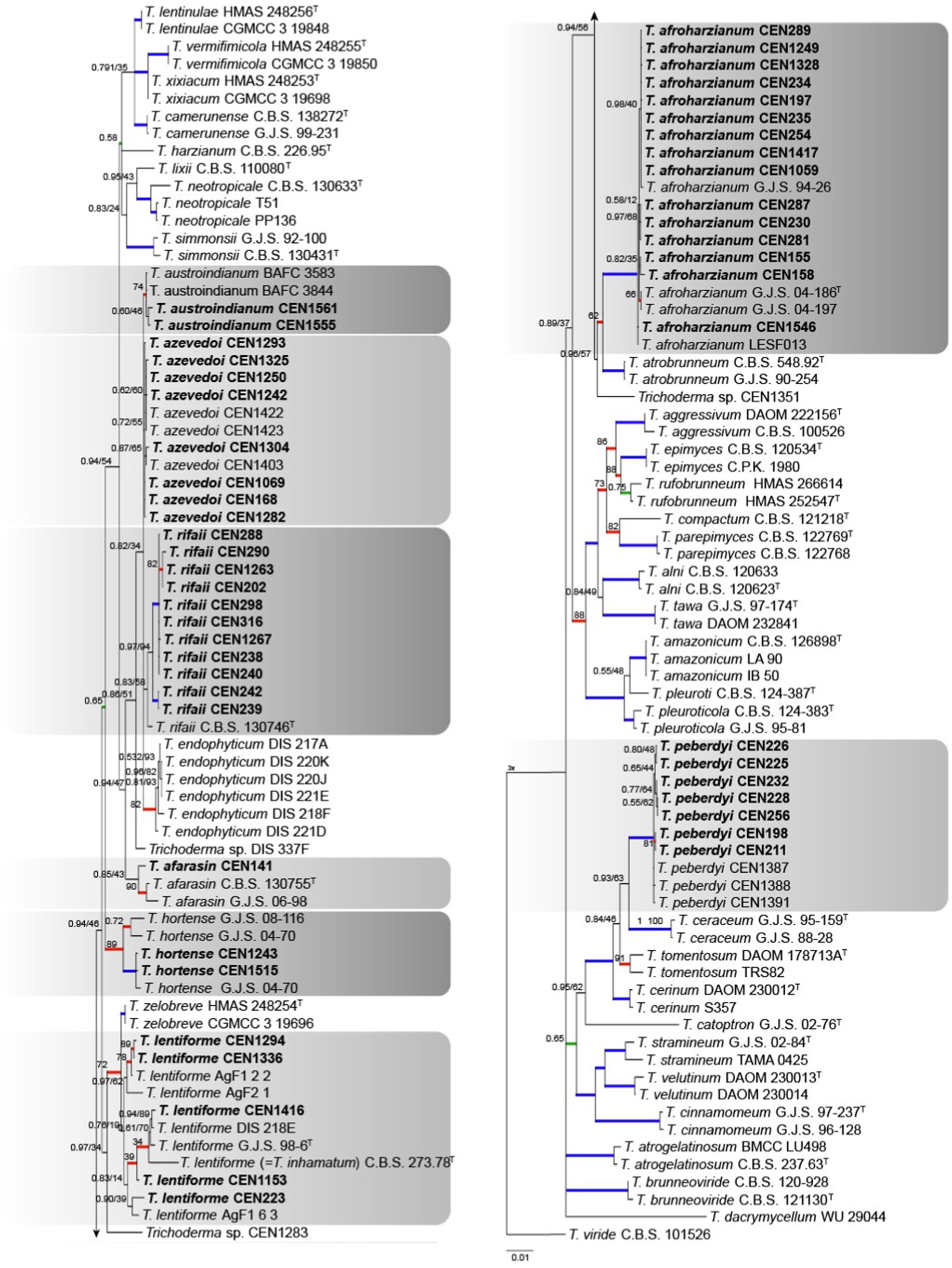
Figure 4. Bayesian phylogenetic tree based on concatenated sequences (TEF1α, ITS, RPB2, CAL, and ACT) of the Harzianum complex. Bayesian posterior probability and maximum likelihood bootstrap support values are indicated at the nodes, and the scale bar represents the number of expected changes per site. Thickened blue lines indicate PP ≥ 0.99 and BS ≥ 95, red color indicates PP ≥ 0.99, and green color indicates BS ≥ 95. The specimen T. viride CBS 101526 was used as the out-group. The strains reported here are highlighted in bold (T = Type specimen).
For sect. Trichoderma, 32 isolates were identified. In this case, T. minutisporum was used as the out-group. The combined gene matrix formed has 2,976 characters and is subdivided into rpb2 (728 bp), act (681 bp), its (544 bp), tef1α (605 bp), and cal (418 bp). In this analysis, the number of conserved sites, variable sites, and informational sites to parsimony is 1,392, 899, and 697, respectively. The chosen nucleotide substitution models were GTR + I + G for its, GTR + I + G for tef1α, SYM + G for rpb2, SYM + G for cal, and GTR + I + G for act in the Bayesian inference analysis and GTR + G for maximum likelihood. The species T. dorothopsis was reclassified in T. koningiopsis due its older name. It was identified in the tree (Figure 5) that the isolates grouped into clades (PP ≥ 0.99 and BT ≥ 95) of T. asperelloides (12 isolates), T. koningiopsis (9 isolates), T. asperellum (4 isolates), T. hamatum (2 isolates), T. atroviride (1 strain), T. erinaceum (1 strain), and T. subviride (1 strain). A clade independent of the known species was formed (PP ≥ 0.99 and BT ≥ 95), and this is being proposed as T. cerradensis.
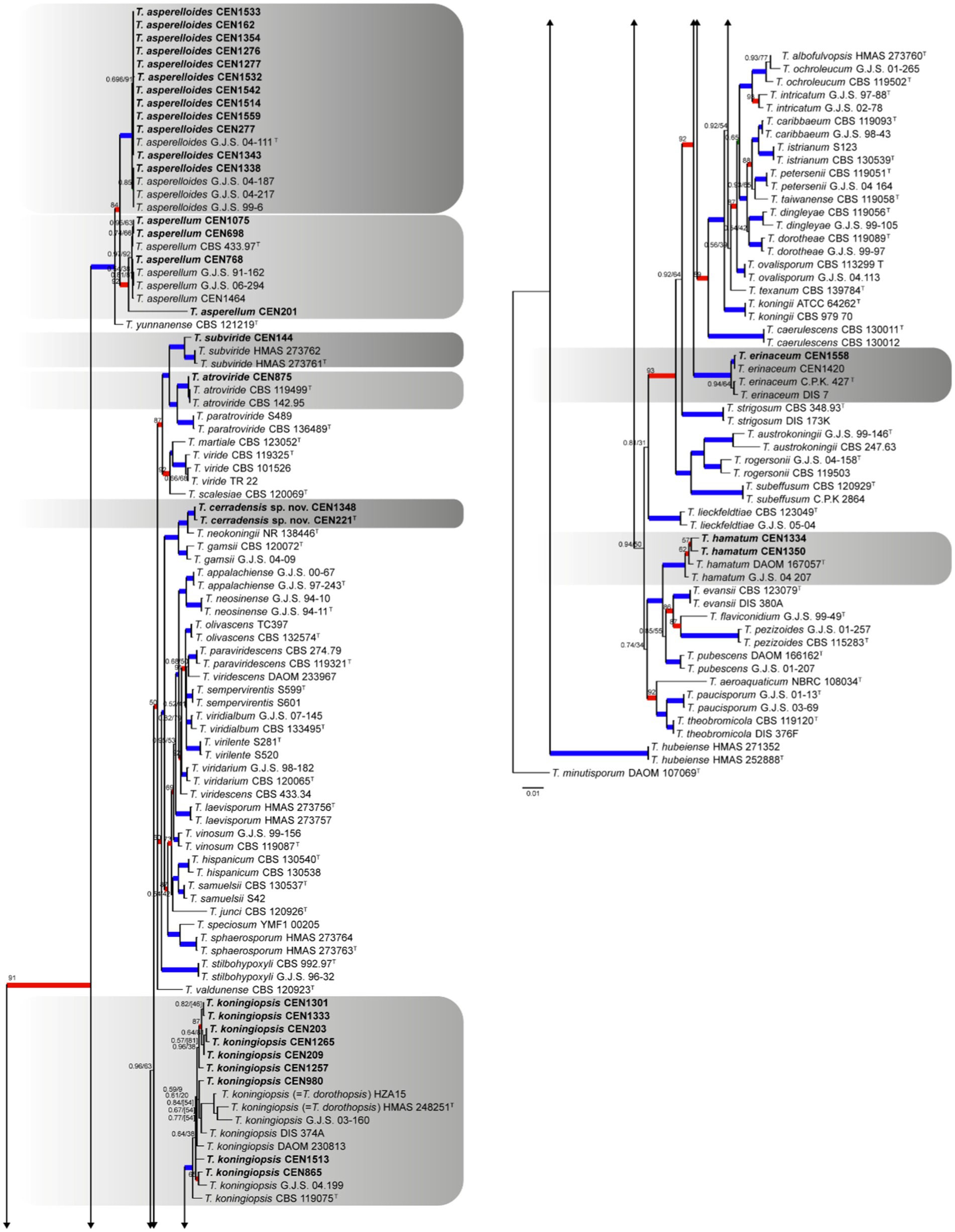
Figure 5. Bayesian phylogenetic tree based on concatenated sequences (TEF1α, ITS, RPB2, CAL, and ACT) of the Sect. Trichoderma complex. Bayesian posterior probability and maximum likelihood bootstrap support values are indicated at the nodes, and the scale bar represents the number of expected changes per site. Thickened blue lines indicate PP ≥ 0.99 and BS ≥ 95, red color indicates PP ≥ 0.99, and green color indicates BS ≥ 95. The specimen T. minutisporum DAOM 107069 was used as the out-group. The strains reported here are highlighted in bold (T = Type specimen).
3.1 Taxonomy
Trichoderma koningiopsis Samuels, C. Suarez & H.C. Evans, Studies in Mycology 56: 117. 2006.
Basionym: Hypocrea koningiopsis Samuels, Studies in Mycology 56: 117. 2006.
Synonyms: Trichoderma dorothopsis A.A. Tomah & J.Z. Zhang, Biological Control 145: 6. 2020.
Notes: Considering the available sequences of T. dorothopsis (tef1α, rpb2, and its), it positioned within the T. koningiopsis clade, showing high support in both the multigene and single-gene analyses of tef1α and rpb2, meeting the GCPSR criteria. The species T. dorothopsis (Tomah et al., 2020) was synonymized under T. koningiopsis (Samuels et al., 2006) due its older description.
Trichoderma lentiforme (Rehm) P. Chaverri, Samuels & F.B. Rocha, Mycologia 107: 577. 2015.
Basionym: Hypocrea lentiformis Rehm, Hedwigia 37: 193. 1898.
Synonyms: Trichoderma inhamatum Veerkamp & W. Gams, Caldasia 13: 710. 1983.
Notes: Trichoderma inhamatum was synonymized under T. lentiforme due to the type of the two species grouping in the same clade of the multi-loci phylogenetic tree. The same results were obtained for the cal, its, and rpb2 individual trees (Supplementary Material). In addition, T. lentiforme and T. inhamatum were morphologically similar and some authors considered these taxa conspecific (Chaverri et al., 2015).
Trichoderma cerradensis Peixoto, Pinho, P. Chaverri, & S.C.M. Mello sp. Nov. Figure 6.
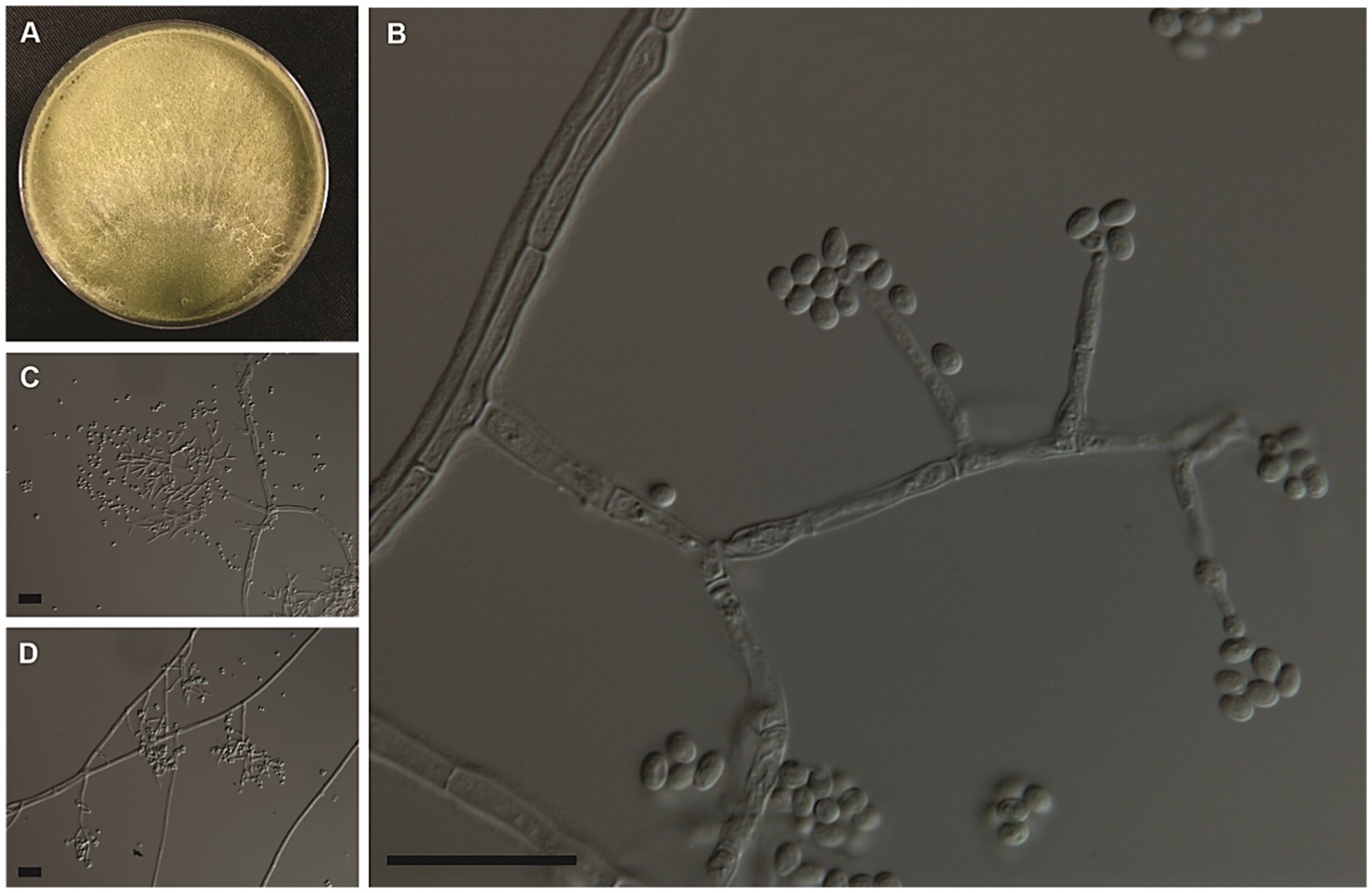
Figure 6. Colony morphologies of the Trichoderma cerradensis strain CEN221 formed on PDA after 7 days (A). Morphological structures of T. cerradensis formed on PDA after 3 days by microculture technique showing conidiophore, phialides, and globose, subglobose to ovoid conidia (B–D). Bars: 20 μm.
MycoBank: 851480.
Typification: BRAZIL, Federal District: Brasília, from the soil cultivated with Gossypium sp., March 2002, coll. F. G. V. Schmidt (holotype UB24548 permanently preserved in a metabolically inactive state, ex-type living culture CEN221).
Teleomorph: Unknown.
GenBank: tef1α = ON101458; its = OM515056; rpb2 = PQ149277; cal = ON241200; and act = ON311059.
Etymology: The name refers to the Brazilian biome, Cerrado, where this species was isolated.
Cultural and micrometric characteristics: Colony radius after 72 h at 25°C on PDA and SNA measuring 63–80 mm, growing more slowly on SNA than on PDA and CMD. The Petri dishes (80 mm Ø) were filled with the CEN221 colony on all culture media after 96 h at 25°C. On PDA, colony radius measured 73–80 mm after 96 h at 30°C. Mycelium cottony with sparse aerial hyphae covering the entire plate, conidia forming abundantly under cottony hyphae after 96 h at 25 and 30°C. No diffusible pigments or distinctive odors observed. On SNA, the colonies measured 77–78 mm in radius after 96 h at 30°C. Colony white with disperse cottony hyphae and dense sporulation at 25°C. Little growth was observed at 15°C and 35°C after 120 h. On CMD, colonies 80 mm in radius after 96 h at 30°C. They were hyaline with disperse cottony hyphae. Masses of green conidia covering the entire plate after 120 h at 25°C. Lowest growth (13–35 mm in radius after 168 h) observed on all culture media at 35°C. Conidiophores pyramidal, with single or opposing branches, terminating in groups of two to three phialides, or solitary. Phialides lageniform, measuring 9.5 ± 1.0 × 3.5 ± 0.5 μm (overall range: 5.5–12.5 × 2.5–4.0 μm), base 1.5–2.5 μm (mean 2.0 μm). Conidial masses olive to green formed by conidia globose, subglobose to ovoid 3.5 ± 0.5 × 3.0 ± 0.5 μm (overall range: 2.5–5.0 × 2.0–4.0 μm) (Table 3). Chlamydospores not observed.
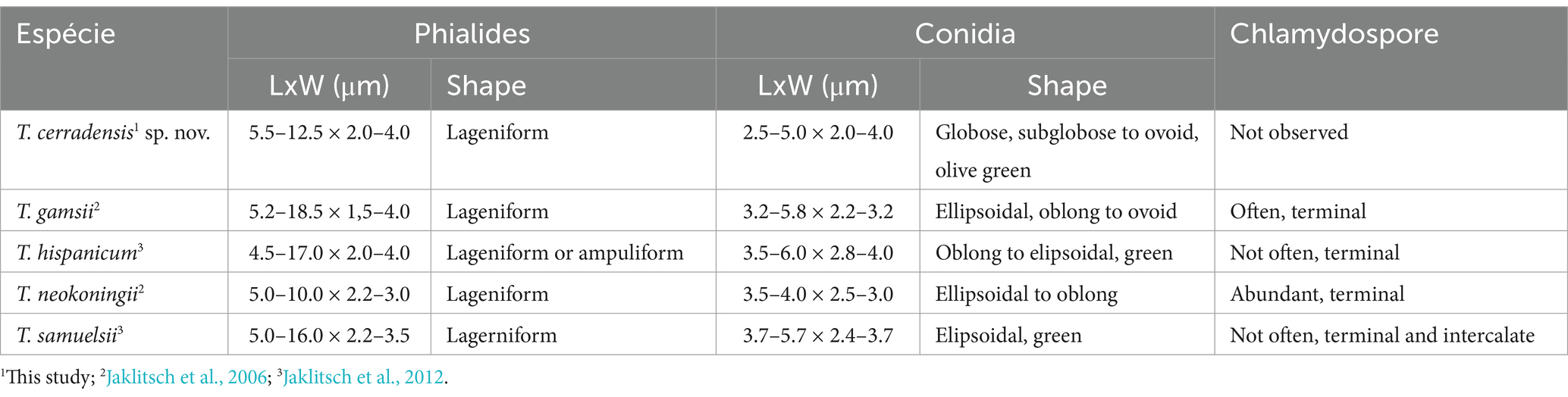
Table 3. Morphological characteristics of Trichoderma cerradensis sp. nov. compared to closely related strains of Trichoderma species.
Additional specimen examined: BRAZIL, Federal District: Rural Nucleus of “Rajadinha,” Brasília, from soil cultivated with Cucurbita pepo, February 2012, coll. J.B.T. da Silva (culture CEN1348).
Known substrate: Soil under Gossypium sp. and Cucurbita pepo.
Known geographic distribution: Brasília, Brazil.
Notes: Considering the GCPSR criteria, the new species was assigned, where in all individual trees, the T. cerradensis clade was highlighted with high support in ML and BI, thus meeting the criteria. Trichoderma cerradensis was closely related to T. gamsii, T. hispanicum, T. neokoningii, and T. samuelsii. Although there is an overlap in phialides and conidial measures, in comparison with T. gamsii, T. hispanicum, and T. samuelsii, phialides of T. cerradensis are shorter. Trichoderma cerradensis conidia were globose to ovoid, while T. gamsii, T. hispanicum, T. neokoningii and T. samuelsii conidia were ellipsoidal, oblong, and sometimes ovoid. Chlamydospores were not observed in Trichoderma cerradensis sp. nov. In contrast, T. gamsii and T. neokoningii often produced terminal chlamydospores, T. hispanicum sometimes produced terminal, and T. samuelsii exhibited terminal and intercalate chlamydospores. Trichoderma cerradensis sp. nov. was distinguished from all other Trichoderma species and well supported in the phylogenetic analyses (Figure 5).
4 Discussion
The present study demonstrates that within a relatively small collection of Trichoderma isolates for biological control, there are more species than previously reported. Many biocontrol isolates were typically classified in the T. harzianum or T. atroviride species complexes (Jaklitsch et al., 2006; 2012). This study identified 19 species of Trichoderma, including the new species T. cerradensis. In addition to T. cerradensis, some of the other species identified were T. afarasin, T. asperelloides, T. asperellum, T. atroviride, T. austroindianum, T. azevedoi, T. brevicompactum, T. erinaceum, T. ghanense, T. hamatum, T. hortense, T. koningiopsis, T. lentiforme, T. longibrachiatum, T. peberdyi, T. rifaii, T. spirale, and T. subviride.
Trichoderma austroindianum and T. hortense were recently identified from Argentinian soils (Barrera et al., 2021). Trichoderma brevicompactum has been reported in Brazil as an endophyte in healthy Theobroma cacao tissues (Novais Bastos, 2012), unlike the specimens recovered from soils in this study (Table 1). Trichoderma asperellum, T. koningiopsis, and T. erinaceum were identified from soil samples under common bean (Phaseolus vulgaris) crops and rubber trees native to the Brazilian Amazon (Lopes et al., 2012; Brito et al., 2023). The isolates characterized in the present study were from natural habitats in the Cerrado (i.e., T. asperellum and T. koningiopsis), Amazon Forest (i.e., T. koningiopsis), and Pantanal of Mato Grosso (i.e., T. erinaceum). Therefore, the data obtained in the present study suggest the versatility of Trichoderma in terms of its ability to colonize different substrata, habitats, and regions.
The data obtained here also provide additional records for species, e.g., T. longibrachiatum from Federal District and Mato Grosso State and T. hamatum from Federal District, that were reported in soil contaminated with textile laundry discharge in Pernambuco and from mangrove sediment in Bahia (da Silva et al., 2016). Trichoderma atroviride (from Goiás State) was previously reported from a soil sample from the Amazon Forest (Grigorevski-Lima et al., 2013) and T. spirale (from Federal District) in samples from a forest agroecosystem in Bahia (Reis et al., 2015) and Brazilian Amazon (Brito et al., 2023). Trichoderma lentiforme (São Paulo State and Federal District) has already been found colonizing palm leaves in Santa Catarina, rubber trees native in Brazilian Amazon, and as an endophyte of several tropical trees (Chaverri et al., 2015; Brito et al., 2023). Trichoderma afroharzianum (Federal District, Goiás, Mato Grosso and São Paulo) is widely distributed and was even found colonizing fungal gardens of the leaf-cutting ant Atta sexdens in São Paulo (Montoya et al., 2016). Inglis et al. (2020) described two new species, namely, T. azevedoi and T. peberdyi, from soil samples of Allium sativum and A. cepa collected from a different area in Brazil (Inglis et al., 2020). It is interesting to mention that all these isolates belong to the same EMBRAPA Culture Collection. In addition, the T. afroharzianum isolate CEN287 is the active ingredient of Habitat®, a commercial biological control product used against Sclerotinia sclerotiorum and Rhizoctonia solani.
Until the present study, T. rifaii had only been found as an endophyte in leaves and stems of tropical trees (Theobroma cacao and T. gileri) from Ecuador (Chaverri et al., 2015). Interestingly, the results obtained here show the presence of this species recovered from samples of native and cultivated soil (different crops) from the Federal District, from soil under cultivated rice and sorghum in Goiás State, and soil from the Pantanal in Mato Grosso State (Table 1). However, this is the first report of this species in Brazil. According to Chaverri et al. (2015), T. rifaii has its sequences identical to T. endophyticum, which explains the proximity of the clades that define these species.
According to a survey carried out by Menolli and Sánchez-García (2020), there are currently 289 its sequences of Trichoderma isolates from Brazil in GenBank. In addition, according to these authors, many sequences in GenBank do not have information regarding the country of origin in their metadata; therefore, the number of Trichoderma species in Brazil may be greatly underestimated.
The present study not only demonstrates the usefulness of fungal culture collections but also increases the cataloging and correct identification of fungal biodiversity that may be applied in organic or sustainable agriculture. In addition, the present study confirms that its is useless in accurate identification of Trichoderma and that, in contrast, tef1α and rpb2 continue to be useful as secondary barcodes and for biodiversity discovery (Chaverri and Samuels, 2003; Chaverri et al., 2015; Cai and Druzhinina, 2021).
Data availability statement
The datasets presented in this study can be found in online repositories. The names of the repository/repositories and accession number(s) can be found in the article/Supplementary material.
Author contributions
GP: Conceptualization, Data curation, Formal analysis, Investigation, Writing – original draft. RS: Conceptualization, Investigation, Methodology, Writing – review & editing. AZ: Data curation, Formal analysis, Investigation, Writing – review & editing. TS: Data curation, Formal analysis, Investigation, Writing – review & editing. PC: Conceptualization, Investigation, Methodology, Writing – review & editing. DP: Conceptualization, Investigation, Methodology, Writing – review & editing. SM: Conceptualization, Project administration, Resources, Writing – review & editing.
Funding
The author(s) declare that financial support was received for the research, authorship, and/or publication of this article. This study received funding from the Embrapa Genetic Resources and Biotechnology and Conselho Nacional de Desenvolvimento Científico e Tecnológico – CNPq.
Acknowledgments
The authors thank M.Sc. Irene Martins (Embrapa) and Ph.D. João Batista Tavares da Silva for their technical support. We also acknowledge the the Conselho Nacional de Desenvolvimento Científico e Tecnológico (CNPq, Brazil) for providing a scholarship to Gustavo H. S. Peixoto and research productivity fellowships to Danilo B. Pinho and Fundação de Apoio a Pesquisa do Distrito Federal (FAP-DF) for the financial resources. Thanks to Universidade de Brasília through grants Edital nº 001/2025 DPI/BCE/UnB.
Conflict of interest
The authors declare that the research was conducted in the absence of any commercial or financial relationships that could be construed as a potential conflict of interest.
Publisher’s note
All claims expressed in this article are solely those of the authors and do not necessarily represent those of their affiliated organizations, or those of the publisher, the editors and the reviewers. Any product that may be evaluated in this article, or claim that may be made by its manufacturer, is not guaranteed or endorsed by the publisher.
Supplementary material
The Supplementary material for this article can be found online at: https://www.frontiersin.org/articles/10.3389/fmicb.2025.1279142/full#supplementary-material
Footnotes
References
Allsopp, N., Olivier, D. L., and Mitchell, D. T. (1987). Fungal populations associated with root systems of proteaceous seedlings at a lowland fynbos site in South Africa. South Afr. J. Bot. 53, 365–369. doi: 10.1016/s0254-6299(16)31398-9
Almeida, K. A., Armesto, C., Monteiro, F. P., and de Souza, J. T. (2018). Diversity of Trichoderma species isolated from dead branches and sapwood of Theobroma cacao trees. Trop. Plant Pathol. 43, 90–94. doi: 10.1007/s40858-017-0191-z
Altschul, S. F., Gish, W., Miller, W., Myers, E. W., and Lipman, D. J. (1990). Basic local alignment 359 search tool. J. Mol. Biol. 215, 403–410. doi: 10.1016/S0022-2836(05)80360-2
Atanasova, L., Druzhinina, I. S., and Jaklitsch, W. M. (2013). “Two hundred Trichoderma species recognized on the basis of molecular phylogeny” in Trichoderma: Biology and applications. eds. M. Mukherjee, P. K. Horwitz, B. A. Mukherjee, and M. Schmoll (Boston, MA: CAB International), 10–42.
Barrera, V. A., Iannone, L., Romero, A. I., and Chaverri, P. (2021). Expanding the Trichoderma harzianum species complex: three new species from argentine natural and cultivated ecosystems. Mycologia 113, 1136–1155. doi: 10.1080/00275514.2021.1947641
Ben M’henni, Y., Salem, I. B., Souli, M., Tounsi, S., Debieu, D., Fillinger, S., et al. (2022). Biocontrol and growth promotion potential of combined application of Trichoderma simmonsii and aspergillus westerdijkiae against apple tree dieback disease. PhytoFrontiers™ 2, 268–279. doi: 10.1094/phytofr-01-22-0005-r
Bettiol, W., Morandi, M. A. B., Pinto, Z. V., Corrêa, É. B., Moura, A. B., Lucon, C. M. M., et al. (2012). Produtos comerciais à base de agentes de biocontrole de doenças de plantas. EMBRAPA - Bol. Pesqui. e Desenvolv. 1:155.
Bettiol, W., Pinto, Z. V., Silva, J. C., Forner, C., Faria, M. R., Pacifico, M. G., et al. (2019). “Produtos comerciais à base de Trichoderma” in Trichoderma: uso na agricultura. eds. J. C. Meyer and M. C. Mazaro (Brasília: Embrapa Soja), 45–160.
Brito, V. N., Lana Alves, J., Sírio Araújo, K., de Souza Leite, T., Borges de Queiroz, C., Pereira, O. L., et al. (2023). Endophytic Trichoderma species from rubber trees native to the Brazilian Amazon, including four new species. Front. Microbiol. 14:1095199. doi: 10.3389/fmicb.2023.1095199
Bustamante, D. E., Calderon, M. S., Leiva, S., Mendoza, J. E., Arce, M., and Oliva, M. (2021). Three new species of Trichoderma in the Harzianum and Longibrachiatum lineages from Peruvian cacao crop soils based on an integrative approach. Mycologia 113, 1056–1072. doi: 10.1080/00275514.2021.1917243
Cai, F., and Druzhinina, I. S. (2021). In honor of John Bissett: authoritative guidelines on molecular identification of Trichoderma. Fungal Div. 107, 1–69. doi: 10.1007/s13225-020-00464-4
Carbone, I., and Kohn, L. M. (1999). A method for designing primer sets for speciation studies in filamentous ascomycetes. Mycologia 91, 553–556. doi: 10.1080/00275514.1999.12061051
Chaverri, P., Branco-Rocha, F., Jaklitsch, W., Gazis, R., Degenkolb, T., and Samuels, G. J. (2015). Systematics of the Trichoderma harzianum species complex and the re-identification of commercial biocontrol strains. Mycologia 107, 558–590. doi: 10.3852/14-147
Chaverri, P., and Samuels, G. J. (2003). Hypocrea/Trichoderma (Ascomycota, Hypocreales, Hypocreaceae): Species with green ascospores. Utrecht: Centraalbureau voor Schimmelcultures, 1–35.
Chen, K., and Zhuang, W. Y. (2017). Discovery from a large-scaled survey of Trichoderma in soil of 394 China. Sci. Rep. 7, 1–37. doi: 10.1038/s41598-017-07807-3
da Silva, J. A. T., de Medeiros, E. V., da Silva, J. M., Tenório, D. A., Moreira, K. A., Nascimento, T. C. E. S., et al. (2016). Trichoderma aureoviride URM 5158 and Trichoderma hamatum URM 6656 are biocontrol agents that act against cassava root rot through different mechanisms. J. Phytopathol. 164, 1003–1011. doi: 10.1111/jph.12521
De Hoog, G. S., and Van den Ended, A. H. G. G. (1998). Molecular diagnostics of clinical strains of filamentous Basidiomycetes. Mycoses 41, 183–189. doi: 10.1111/j.1439-0507.1998.tb00321.x
del Carmen, H., Rodríguez, M., Evans, H. C., and de Abreu, L. M. (2021). New species and records of Trichoderma isolated as mycoparasites and endophytes from cultivated and wild coffee in Africa. Sci. Rep. 11:5671. doi: 10.1038/s41598-021-84111-1
Dou, K., Lu, Z., Wu, Q., Ni, M., Yu, C., Wang, M., et al. (2020). MIST: a multilocus identification system for Trichoderma. Appl. Environ. Microbiol. 86:20. doi: 10.1128/AEM.01532-20
Druzhinina, I. S., Shelest, E., and Kubicek, C. P. (2012). Novel traits of Trichoderma predicted through the analysis of its secretome. FEMS Microbiol. Lett. 337, 1–9. doi: 10.1111/j.1574-6968.2012.02665.x
Du Plessis, I. L., Druzhinina, I. S., Atanasova, L., Yarden, O., and Jacobs, K. (2018). The diversity of Trichoderma species from soil in South Africa, with five new additions. Mycologia 110, 559–583. doi: 10.1080/00275514.2018.1463059
Grigorevski-Lima, A. L., De Oliveira, M. M. Q., Do Nascimento, R. P., Da Silva Bon, E. P., and Coelho, R. R. R. (2013). Production and partial characterization of cellulases and xylanases from Trichoderma atroviride 676 using lignocellulosic residual biomass. Appl. Biochem. Biotechnol. 169, 1373–1385. doi: 10.1007/s12010-012-0053-6
Groenewald, J. Z., Nakashima, C., Nishikawa, J., Shin, H. D., Park, J. H., Jama, A. N., et al. (2013). Species concepts in Cercospora: spotting the weeds among the roses. Stud. Mycol. 75, 115–170. doi: 10.3114/sim0012
Hanada, R. E., de Jorge Souza, T., Pomella, A. W., Hebbar, K. P., Pereira, J. O., Ismaiel, A., et al. (2008). Trichoderma martiale sp. nov., a new endophyte from sapwood of Theobroma cacao with a potential for biological control. Mycol. Res. 112, 1335–1343. doi: 10.1016/j.mycres.2008.06.022
Hoyos-Carvajal, L., and Bisset, J. (2011). “Biodiversity of Trichoderma in neotropics” in The dynamical processes of biodiversity - case studies of evolution and spatial distribution. ed. G. Grillo (Rijeka: IntechOpen), 303–320.
Hu, J., Zhou, Y., Chen, K., Li, J., Wei, Y., Wang, Y., et al. (2020). Large-scale Trichoderma diversity was associated with ecosystem, climate and geographic location. Environ. Microbiol. 22, 1011–1024. doi: 10.1111/1462-2920.14798
Hughes, K. A., Bridge, P., and Clark, M. S. (2007). Tolerance of antarctic soil fungi to hydrocarbons. Sci. Total Environ. 372, 539–548. doi: 10.1016/j.scitotenv.2006.09.016
Inglis, P. W., Mello, S. C. M., Martins, I., Silva, J. B. T., Macêdo, K., Sifuentes, D. N., et al. (2020). Trichoderma from Brazilian garlic and onion crop soils and description of two new species: Trichoderma azevedoi and Trichoderma peberdyi. PLoS One 15, e0228485–e0228423. doi: 10.1371/journal.pone.0228485
Jacobs, K., Bergdahl, D. R., Wingfield, M. J., Halik, S., Seifert, K. A., Bright, D. E., et al. (2004). Leptographium wingfieldii introduced into North America and found associated with exotic Tomicus piniperda and native bark beetles. Mycol. Res. 108, 411–418. doi: 10.1017/S0953756204009748
Jacobs, P. H., Taylor, H. C., and Shafer, J. C. (1964). Studies of fungi at Amundsen-Scott IGY South pole base. Arch. Dermatol. Res. 89, 117–123. doi: 10.1001/archderm.1964.01590250123021
Jaklitsch, W. M. (2009). European species of Hypocrea part I the green-spored species. Stud. Mycol. 63, 1–91. doi: 10.3114/sim.2009.63.01
Jaklitsch, W. M., Samuels, G. J., Dodd, S. L., Lu, B. S., and Druzhinina, I. S. (2006). Hypocrea rufa/Trichoderma viride: a reassessment, and description of five closely related species with and without warted conidia. Stud. Mycol. 56, 135–177. doi: 10.3114/sim.2006.56.04
Jaklitsch, W. M., Stadler, M., and Voglmayr, H. (2012). Blue pigment in Hypocrea caerulescens sp. nov. and two additional new species in sect. Trichoderma. Mycologia 104, 925–941. doi: 10.3852/11-327
Jaklitsch, W. M., and Voglmayr, H. (2014). Europe PMC funders group new combinations in Trichoderma (Hypocreaceae, Hypocreales). Mycotaxon 126, 143–156. doi: 10.5248/126.143.New
Jaklitsch, W. M., and Voglmayr, H. (2015). Biodiversity of Trichoderma (Hypocreaceae) in southern Europe and Macaronesia. Stud. Mycol. 80, 1–87. doi: 10.1016/j.simyco.2014.11.001
Katoh, K., and Standley, D. M. (2013). MAFFT multiple sequence alignment software version 7: improvements in performance and usability. Mol. Biol. Evol. 30, 772–780. doi: 10.1093/molbev/mst010
Klein, D., and Eveleigh, D. E. (1998). “Ecology of Trichoderma” in Trichoderma & Gliocladium: Basic biology, taxonomy and genetics. eds. G. E. Kubicek and C. P. Harman (New York, NY: Taylor & Francis), 57–69.
Kumar, S., Stecher, G., and Tamura, K. (2016). MEGA7: molecular evolutionary genetics analysis version 7.0 for bigger datasets. Mol. Biol. Evol. 33, 1870–1874. doi: 10.1093/MOLBEV/MSW054
Liu, Y. J., Whelen, S., and Hall, B. D. (1999). Phylogenetic relationships among ascomycetes: evidence from an RNA polymerase II subunit. Mol. Biol. Evol. 16, 1799–1808. doi: 10.1093/oxfordjournals.molbev.a026092
Lopes, F. A. C., Steindorff, A. S., Geraldine, A. M., Brandão, R. S., Monteiro, V. N., Júnior, M. L., et al. (2012). Biochemical and metabolic profiles of Trichoderma strains isolated from common bean crops in the Brazilian Cerrado, and potential antagonism against Sclerotinia sclerotiorum. Fungal Biol. 116, 815–824. doi: 10.1016/j.funbio.2012.04.015
López-Bucio, J., Pelagio-Flores, R., and Herrera-Estrella, A. (2015). Trichoderma as biostimulant: exploiting the multilevel properties of a plant beneficial fungus. Sci. Hortic. 196, 109–123. doi: 10.1016/j.scienta.2015.08.043
Menolli, N., and Sánchez-García, M. (2020). Brazilian fungal diversity represented by DNA markers generated over 20 years. Braz. J. Microbiol. 51, 729–749. doi: 10.1007/s42770-019-00206-y
Miller, M. A., Pfeiffer, W., and Schwartz, T. (2010). Creating the CIPRES science gateway for inference of large phylogenetic trees. Gatew Comput. Environ. Work. GCE 2010, 1–8. doi: 10.1109/GCE.2010.5676129
Montoya, Q. V., Meirelles, L. A., Chaverri, P., and Rodrigues, A. (2016). Unraveling Trichoderma species in the attine ant environment: description of three new taxa. Antonie van Leeuwenhoek Int. J. Gen. Mol. Microbiol. 109, 633–651. doi: 10.1007/s10482-016-0666-9
Mukesh, S., Vipul, K., Mohammad, S., Sonika, P., and Anuradha, S. (2016). Trichoderma- a potential and effective bio fungicide and alternative source against notable phytopathogens: a review. African J. Agric. Res. 11, 310–316. doi: 10.5897/ajar2015.9568
Mukherjee, P. K., Horwitz, B. A., Singh, U. S., Mukherjee, M., and Schmoll, M. (2013). “Trichoderma in agriculture, industry and medicine: an overview,” in Trichoderma: Biology and applications, ed. M. Mukherjee, Horwitz, B.A., and Mukherjee, M. (Boston, MA: CAB International, 1–9.
Nirenberg, H. I. (1976). Untersuchungen über die morphologische und biologische differenzierung in der Fusarium-Sektion Liseola. Mitt Biol Bundesanst Land-Forstw Berlin-Dahlem 169, 1–117.
Novais Bastos, C. (2012). Isolate of Trichoderma brevicompactum for the control of cocoa witches’ broom disease: preliminary results. Agrotrópica 24, 21–26. doi: 10.21757/0103-3816.2012v24n1p21-26
Nylander, J. A. A. (2004). MrModeltest v2. Program distributed by the author. Evol. Biol. Cent. Uppsala Univ. 2, 1–2.
Oliveira, L. G., Kettner, M. G., Lima, M. L. S., Leão, M. P. C., da Santos, A. C., and Costa, A. F. (2023). Trichoderma species from soil of Pernambuco state, Brazil. Curr. Microbiol. 80:289. doi: 10.1007/s00284-023-03401-1
Pinho, D. B., Firmino, A. L., Ferreira-Junior, W. G., and Pereira, O. L. (2012). An efficient protocol for DNA extraction from Meliolales and the description of Meliola centellae sp. nov. Mycotaxon 122, 333–345. doi: 10.5248/122.333
Qiao, M., Du, X., Zhang, Z., Xu, J. P., and Yu, Z. F. (2018). Three new species of soil-inhabiting Trichoderma from Southwest China. MycoKeys 44, 63–80. doi: 10.3897/mycokeys.44.30295
Rambaut, A. (2018). FigTree. Available at: http://tree.bio.ed.ac.uk/software/figtree/.
Rambaut, A., and Drummond, A. (2018). Tracer MCMC trace analysis tool. Available at: http://tree.bio.ed.ac.uk/software/tracer/ (Accessed April 14, 2023).
Reis, B. M., Silva, A., Alvarez, M. R., de Oliveira, T. B., and Rodrigues, A. (2015). Fungal communities in gardens of the leafcutter ant Atta cephalotes in forest and cabruca agrosystems of southern Bahia state (Brazil). Fungal Biol. 119, 1170–1178. doi: 10.1016/j.funbio.2015.09.001
Ronquist, F., and Huelsenbeck, J. P. (2003). MrBayes 3: Bayesian phylogenetic inference under mixed models. Bioinformatics 19, 1572–1574. doi: 10.1093/bioinformatics/btg180
Samuels, G. J., Dodd, S., Lu, B. S., Petrini, O., Schroers, H. J., and Druzhinina, I. (2006). The Trichoderma koningii aggregate species. Stud. Mycol. 56, 67–133. doi: 10.3114/sim.2006.56.03
Stamatakis, A. (2014). RAxML version 8: a tool for phylogenetic analysis and post-analysis of large phylogenies. Bioinformatics 30, 1312–1313. doi: 10.1093/bioinformatics/btu033
Sung, G. H., Sung, J. M., Hywel-Jones, N. L., and Spatafora, J. W. (2007). A multi-gene phylogeny of Clavicipitaceae (Ascomycota, Fungi): identification of localized incongruence using a combinational bootstrap approach. Mol. Phylogenet. Evol. 44, 1204–1223. doi: 10.1016/j.ympev.2007.03.011
Tomah, A. A., Alamer, I. S. A., Li, B., and Zhang, J. Z. (2020). A new species of Trichoderma and gliotoxin role: a new observation in enhancing biocontrol potential of T. virens against Phytophthora capsici on chili pepper. Biol. Control 145:104261. doi: 10.1016/j.biocontrol.2020.104261
Tomico-Cuenca, I., Mach, R. L., Mach-Aigner, A. R., and Derntl, C. (2021). An overview on current molecular tools for heterologous gene expression in Trichoderma. Fungal Biol. Biotechnol. 8, 11–17. doi: 10.1186/s40694-021-00119-2
Vilgalys, R., and Hester, M. (1990). Rapid genetic identification and mapping of enzymatically amplified ribosomal DNA from several Cryptococcus species. J. Bacteriol. 172, 4238–4246. doi: 10.1128/jb.172.8.4238-4246.1990
Weindling, R. (1932). Trichoderma lignorum as a parasite of others soil fungi. Phytopathology 2, 1–10. doi: 10.4236/oalib.1101706
Keywords: Hypocreales, Hypocreaceae, multigenic, new taxon, biological control
Citation: Peixoto GHS, da Silva RAF, Zacaroni AB, Silva TF, Chaverri P, Pinho DB and de Mello SCM (2025) Trichoderma collection from Brazilian soil reveals a new species: T. cerradensis Sp. nov. Front. Microbiol. 16:1279142. doi: 10.3389/fmicb.2025.1279142
Edited by:
K. M. Golam Dastogeer, Bangladesh Agricultural University, BangladeshReviewed by:
Karin Jacobs, Stellenbosch University, South AfricaAbolfazl Narmani, University of Tabriz, Iran
Copyright © 2025 Peixoto, da Silva, Zacaroni, Silva, Chaverri, Pinho and de Mello. This is an open-access article distributed under the terms of the Creative Commons Attribution License (CC BY). The use, distribution or reproduction in other forums is permitted, provided the original author(s) and the copyright owner(s) are credited and that the original publication in this journal is cited, in accordance with accepted academic practice. No use, distribution or reproduction is permitted which does not comply with these terms.
*Correspondence: Sueli Corrêa Marques de Mello, c3VlbGkubWVsbG9AZW1icmFwYS5icg==; Danilo Batista Pinho, ZGFuaWxvcGluaG9AdW5iLmJy