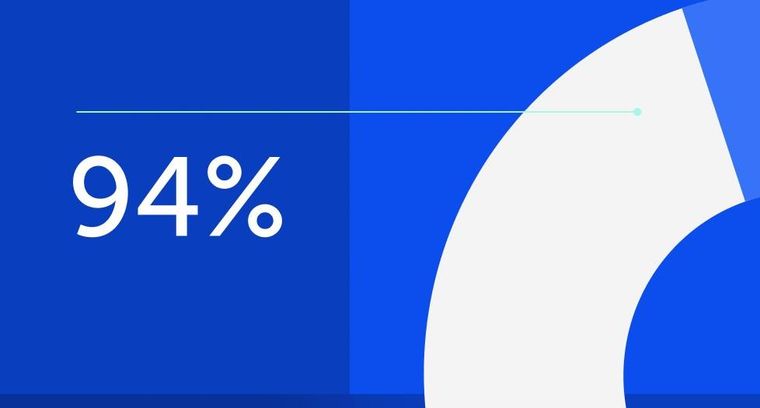
94% of researchers rate our articles as excellent or good
Learn more about the work of our research integrity team to safeguard the quality of each article we publish.
Find out more
ORIGINAL RESEARCH article
Front. Microbiol., 07 January 2025
Sec. Antimicrobials, Resistance and Chemotherapy
Volume 15 - 2024 | https://doi.org/10.3389/fmicb.2024.1532743
Introduction: Glaesserella parasuis causes Glässer’s disease in pigs, a leading cause of death in swine herds and a major contributor to economic losses in the global swine industry. Although several studies have investigated antimicrobial resistance in G. parasuis, the correlation between phenotypic and genotypic resistance remains unclear due to incomplete genetic resistance mechanisms detection.
Methods: The susceptibility of 117 clinical G. parasuis isolates to 7 antimicrobials was determined using a broth microdilution method. The sequences of 48 resistant isolates were obtained by whole-genome sequencing. Resistance genes, mutations, and group 1 vtaAs were detected based on whole-genome sequence data. Sequence types (STs) were identified by multilocus sequence typing (MLST).
Results: Phenotypic analysis showed that most isolates were susceptible to the tested antibiotics; resistance was most common against tetracycline (27%) and enrofloxacin (20%). All isolates were susceptible to ceftiofur. Analysis of whole-genome sequences revealed that resistance to tetracycline, amoxicillin, erythromycin, florfenicol, and chloramphenicol was frequently associated with the resistance genes tet(B) or tet(H), blaROB–1, erm(T), floR, and catA3, and enrofloxacin resistance was associated with mutations in GyrA, ParC, and ParE. MLST identified 25 STs, of which, 14 were novel. The sequenced strains were divided into two primary lineages, LI and LII. Group 1 vtaA genes were detected in 87.5% (n = 42) of the isolates.
Conclusion: This study provides comprehensive insights into the molecular mechanisms responsible for drug resistance in G. parasuis, the characteristics of molecular epidemiology, and the virulence of resistant groups. Our findings can aid in the development of G. parasuis-specific clinical breakpoints and inform strategies for managing antimicrobial resistance in swine herds.
Glaesserella parasuis commonly colonizes the upper respiratory tract of pigs and can cause Glässer’s disease in immunocompromised hosts (Zhang et al., 2019; Costa-Hurtado et al., 2020). Discovering virulence factors is key to understanding the pathogenesis of G. parasuis. Several putative virulence-associated genes have been reported, such as those encoding capsule, lipopolysaccharides, trimeric autotransporters, and outer membrane proteins, among others. Among them, virulence-associated trimeric autotransporter (vtaA) genes are the most important virulence factors, as some of the group 1 vtaAs are more frequently used in diagnostic settings to predict the virulence potential of strains (Mugabi et al., 2023). Certain sequence types (STs) of G. parasuis isolates are responsible for outbreaks of infections (Mugabi et al., 2023). Multilocus sequence typing (MLST) has achieved high levels of discrimination and is useful for understanding the evolution and epidemiology of G. parasuis.
Antibiotics are widely administered to animals to treat bacterial infections, prevent diseases, and promote growth (Kimera et al., 2020). However, the overuse and misuse of antimicrobial agents in animals contribute to the growing threat of antimicrobial resistance (AMR) (Mudenda et al., 2023). Under the pressure of antimicrobial selection, antimicrobial-resistant bacterial strains acquire a greater ability to proliferate in animals, reducing the effectiveness of antimicrobial drugs used in animal-derived food production (Velazquez-Meza et al., 2022). Continuous monitoring of AMR is essential to address this threat and can help guide empirical treatment recommendations (Gulumbe et al., 2022). Antimicrobial susceptibility testing and polymerase chain reaction (PCR) analysis are primary methods for routine phenotypic and genotypic resistance monitoring. While PCR may not detect all resistance mechanisms, whole-genome sequencing (WGS) provides comprehensive insights into known resistance-related genes and mutations in resistant strains (Yang et al., 2023).
In China, G. parasuis strains remain susceptible to most antibiotics; however, an increasing trend of AMR is observed (Zhang et al., 2019). Most studies have focused on the phenotypic susceptibility of G. parasuis isolates (Zhou et al., 2010; Brogden et al., 2018). Few studies have used the traditional PCR method to determine the genes or mutations responsible for phenotypic resistance (Dayao D. et al., 2016). As WGS has become more affordable in recent years, it has been used to detect resistance elements in various bacteria (McDermott et al., 2016; Bossé et al., 2017; Fen et al., 2023). In a recent study, we applied WGS to gain genomic insights into the diversity of G. parasuis strains found worldwide (Gong et al., 2024). However, without antimicrobial susceptibility data, resistance mechanisms cannot be linked to phenotypic resistance. Therefore, we primarily aimed to obtain comprehensive information on the determinants of resistance in resistant G. parasuis isolates. The susceptibility of 117 G. parasuis isolates to 7 antimicrobial compounds was tested in this study. Resistant isolates were screened for associated resistance mechanisms using WGS. Moreover, we also characterized the molecular epidemiology and virulence of the resistant isolates.
A total of 117 G. parasuis isolates, supplied by the National Reference Laboratory of Veterinary Drug Residues (Guangzhou, China), were isolated from pigs with Glässer’s disease in South China from 2010 to 2017. Isolates were cultured on tryptic soy agar supplemented with 10 μg/ml of nicotinamide adenine dinucleotide and 5% (v/v) fetal calf serum for 18 h at 37°C. Bacterial species were identified using 16S rRNA diagnostic PCR as described previously (Oliveira et al., 2001).
In vitro antimicrobial susceptibility testing was performed using the broth microdilution method, as described previously (Prüller et al., 2017). The following antimicrobial agents and test ranges were used: amoxicillin (AMX, 0.12–256 μg/ml), ceftiofur (CEF, 0.12–128 μg/ml), enrofloxacin (ENR, 0.12–128 μg/ml), erythromycin (ERY, 0.25–256 μg/ml), florfenicol (FFC, 0.12–128 μg/ml), tetracycline (TET, 0.12–128 μg/ml), and chloramphenicol (CHL, 0.5–128 μg/ml). Quality control was performed using Escherichia coli ATCC 25922 and Staphylococcus aureus ATCC 29213. As resistance breakpoints specific to G. parasuis have not been reported, the breakpoints used in this study were derived from previous studies when available (Zhou et al., 2010). For AMX, if the minimum inhibitory concentrations (MICs) showed a bimodal distribution, isolates with high MICs were considered “resistant” in this study.
G. parasuis isolates that were resistant to at least one of the tested antibiotics were subjected to WGS. Briefly, colonies were scraped from tryptic soy agar plates supplemented with 10 μg/ml nicotinamide adenine dinucleotide and 5% (v/v) fetal calf serum, which were cultured at 37°C for 18 h. Genomic DNA was extracted using the TIANamp Bacteria DNA Kit (Tiangen, Beijing, China) following the manufacturer’s instructions. WGS was performed with 250-bp reads on the MiSeq platform (Illumina, San Diego, CA, USA). Trimmed reads were assembled using SPAdes v3.6.2 (Bankevich et al., 2012).
Single nucleotide polymorphism (SNP) phylogenetic analysis was performed using Parsnp v1.7.4,1 and the conserved regions were then filtered out with Pandas v2.0.3 using in-house scripts. A core-genome SNP phylogenetic tree was generated and then visualized using the online tool iTOL.2
Sequence types (STs) were determined by querying the assembled sequences using the G. parasuis pubMLST typing platform.3 Novel alleles and STs were assigned by submitting the data to the pubMLST G. parasuis database. The strains were divided into different groups according to their allelic profiles using BURST analysis of PubMLST plugins.4 A clone complex (CC) was defined if these strains contained at least five identical alleles; otherwise, the STs were considered singletons.
Acquired antimicrobial resistance genes were identified using ResFinder v4.6.05 with default settings. The threshold for %ID was 90% and the minimum length was 60%. The 16-membered macrolides resistance gene estT was not present in the database; therefore, we downloaded its reference sequence for Sphingobacterium faecium strain WB1 plasmid pWB1 (CP094932.1) from NCBI. Chromosomal mutations associated with AMR were determined in gyrA, gyrB, parC, parE, rplD (L4), rplV (L22), and 23S rRNA using online BLAST software6 (Piddock, 2002; Eaves et al., 2004). G. parasuis Nagasaki (CP018034.1) was used as the reference strain.
The 406-bp amplicon sequence of Group1 vtaAs was extracted from the complete sequence of G. parasuis strain Nagasaki (CP018034.1) using the primers reported in a previous study. Blastn was used to screen for vtaAs in the assembled sequences by querying the 406-bp amplicon.
The core-genome SNP phylogenetic tree showed high genetic diversity and was divided into two primary lineages (LI and LII) based on the structure of the phylogenetic tree (Figure 1). The lineage of LI contained 31 isolates, larger than LII, which contained 17 isolates.
Figure 1. Core-genome phylogenetic tree of 48 sequenced genomes. Color shades indicate the primary lineages; LI and LII.
A total of 25 STs were identified within the collection of 48 sequenced isolates, among which, 14 were novel (Figure 2). The most common STs were ST254 (n = 8) and ST362 (n = 6), associated with LI. ST792 (n = 4) was the dominant ST within LII, attributed to clonal dissemination according to core genome analysis. More than half of the isolates (n = 26) were divided into 6 CCs, and the others belonged to 22 singletons. The most prevalent CC (CC6) included 2 sequence types, ST362 and ST541, and was part of LI.
Figure 2. Distribution of STs, CCs, group 1 vtaAs, and phenotypic resistance. Core-genome phylogenetic tree was constructed based on core-genome SNPs. STs, sequence types; CCs, clonal complexes; AMX, amoxicillin; ENR, enrofloxacin; ERY, erythromycin; TET, tetracycline; CHL, chloramphenicol. FFC, florfenicol.
Group 1 vtaAs were identified in 87.5% (n = 42) of the isolates. In detail, 93.5% (n = 29) of the LI isolates were positive for group 1 vtaAs, but only 76.4% (n = 13) of the LII isolates contained group 1 vtaAs (Figure 2).
Each of the 117 G. parasuis isolates was tested for susceptibility to seven antimicrobial agents. Among these isolates, 41% (n = 48) displayed resistance to at least one of the tested antibiotics. The most common resistances were to TET (27%, n = 27), ENR (20%, n = 23), and AMX (11%, n = 13), followed by FFC (6%, n = 7), ERY (4%, n = 5), and CHL (2%, n = 2). All 117 isolates were susceptible to CEF (Table 1). No correlation between phenotypic resistance and STs, lineages, or virulence factors was found in this study (Figure 2).
Among the 117 G. parasuis isolates, 59% (n = 69) showed no resistance to any of the tested antimicrobial agents and 6.8% (n = 8) displayed resistance to at least three antibiotics. The most common resistance phenotype was ENR (n = 13), followed by TET (n = 11), AMX+TET (n = 6), ENR+TET (n = 3), TET+FFC (n = 3), and AMX+ENR+TET+CHL (n = 3) (Table 2).
A total of 12 known resistance genes and 6 mutations were identified in our WGS isolates, which predicted AMR to the 7 tested antibiotics and additional drugs (sul2 for sulfonamide, strA and strB for streptomycin, aph(3′)-Ic for gentamicin, and lnu(C) for lincomycin). Among these resistance genes and mutations, 12 were associated with a decreased susceptibility to the tested antimicrobial compounds. Of the 48 resistant isolates evaluated using WGS, 31 were resistant to TET and harbored tet(B) (n = 27) or tet(H) (n = 2) (Table 3). Nineteen isolates were susceptible to TET and were negative for tet alleles. However, two isolates were susceptible to TET positive for tet(B) and had MICs of 2 and 4 μg/ml.
No acquired genes associated with quinolone resistance were detected among the sequenced isolates, whereas genomes of all G. parasuis isolates contained at least one mutation within the quinolone resistance-determining regions (QRDRs) of GyrA, ParC, and ParE (Table 4). Mutations were absent in GyrB. Fourteen of the twenty-three resistant isolates contained two mutations in GyrA (Ser83 and Asp87) and mutations in the QRDRs of ParC and ParE. Three resistant isolates contained two mutations at the Ser83 and Asp87 codons of GyrA and a Glu84Lys substitution in ParC. Isolates containing only one substitution at Ser83 of GyrA, with or without mutations in ParC and ParE, were susceptible to ENR. Substitutions at codons Ser83 and Asp87 combined with additional mutations in ParC were found in both resistant and susceptible isolates.
Table 4. MICs for enrofloxacin and resistance mutations in QRDRs of 48 sequenced Glaesserella parasuis isolates.
Four of the erythromycin-resistant isolates, contained known macrolide resistance genes or mutations, with MICs of 64 μg/ml (Table 3). Three resistant isolates harbored erm(T), which encodes a methyltransferase that confers macrolide resistance. One isolate contained the mutation A2059G in the 23S rRNA gene. All genomes were also screened for mutations in the L4 and L22 proteins, encoded by the rplD and rplV genes. Thirteen sequenced isolates harbored at least one mutation in L4 or L22. These mutations were identified in both the susceptible and resistant isolates.
Each AMX-resistant isolate harbored blaROB–1, which encodes a beta-lactamase. Seven isolates were resistant to FFC, all harboring the resistance-associated floR gene. Only two isolates were resistant to CHL and contained the catA3 resistance gene. Notably, isolates with CHL MICs of 4 μg/ml contained the floR gene (Table 3).
G. parasuis infections are common in modern swine production, resulting in economic losses. To control infections, antimicrobial treatment is one of the most important approaches. As antimicrobials were widely used as feed additives in China to promote growth before 2020, AMR in various bacteria, including G. parasuis, is increasing (Zhang et al., 2019; Shao et al., 2021). Therefore, the rise of AMR in G. parasuis is a growing concern, and the characterization of phenotypic and complete genotypic AMR represents a vital strategy for eliminating resistant strains.
This study sequenced 48 resistant G. parasuis isolates using WGS. Phylogenetic analysis indicated 2 primary lineages, LI and LII. This is similar to another study conducted in China, which was different from that reported in other countries, which showed that G. parasuis isolates were clustered into 3 primary lineages (Wan et al., 2020; Mugabi et al., 2023).
MLST is now integrated with WGS, which contributes to our understanding of the evolution and molecular epidemiology of G. parasuis. We identified 25 STs within the sequenced isolates, of which 14 were novel. In addition, almost half of the isolates had singleton STs, highlighting the heterogeneity and instability of G. parasuis strains. Other studies have also observed the diverse nature of G. parasuis (Wang et al., 2016; Turni et al., 2018; Gong et al., 2024). Most of the isolates with identical STs showed a close evolutionary relationship in our study, suggesting clonal dissemination of G. parasuis in certain farms and areas, which was reported in previous studies (Wu et al., 2023).
Several putative virulence genes are associated with the pathogenicity of G. parasuis. However, detection of all the putative virulence genes might lead to confusing results, as over half were conserved in G. parasuis. Group 1 vtaAs were considered good predictors of virulence, and some of them had been experimentally verified based on previous research (Olvera et al., 2012). In the current study, we chose group 1 vtaAs as the only virulence marker and screened them through our collection. Group 1 vtaAs were identified in most of the isolates in this study, which might be explained by the fact that all the strains were isolated from diseased pigs. In the present study, a larger number of LI isolates were observed containing group 1 vtaAs compared with those in LII. Mugabi et al. (2023) also found that LI and LII isolates carried a higher number of group 1 vtaA genes on average than LIII isolates.
Most G. parasuis isolates were susceptible to the tested antimicrobials. Only 7% of the isolates were resistant to at least three antibiotics. Our study confirms the high prevalence of antimicrobial-susceptible strains (Dayao et al., 2014). As antimicrobials such as gentamycin, streptomycin, and the combination of trimethoprim/sulphamethoxazole were not tested in this study, the frequency of multidrug resistance was lower than that previously reported in China (13%) (Zhou et al., 2010).
We observed a high level of TET resistance (27%), which was similar to that reported in another study (29%) (Zhang et al., 2019). TET resistance was predominantly conferred by tet(B) (27/29) and less commonly by tet(H) (2/29) in the present study. In a global investigation of G. parasuis, two TET resistance determinants, tet(B) and tet(H), have been detected, with the dominant tet allele being tet(B) (Lancashire et al., 2005; Gong et al., 2024). Two isolates that contained tet(B) had MICs that did not reach the resistance breakpoint. This finding suggests that a TET resistance breakpoint or an intermediate MIC cut-off specific to G. parasuis may be established.
We identified many ENR-resistant G. parasuis isolates as reported in other studies (Guo et al., 2011). Quinolone resistance was usually conferred by QRDR mutations and/or plasmid-mediated quinolone resistance genes. In this study, isolates possessing multiple mutations (two in GyrA, one in ParC, and others in ParE) within the QRDRs were resistant to ENR. However, a single substitution of Ser83 in GyrA, along with other QRDR mutations in ParC and/or ParE did not confer ENR resistance. Two G. parasuis isolates with a single mutation in GyrA exhibit moderate resistance to ENR (Guo et al., 2011). These two isolates contain plasmid-mediated quinolone resistance genes [qnrB6 or aac(6′)-Ib-cr]. No substitutions were observed in the GyrB protein of all the sequenced isolates.
According to the Annual Report on the Development of Veterinary Medicine Industry in China (for 2013 and 2014), doxycycline and enrofloxacin have become two of the four leading antimicrobial agents used in veterinary medicine, which might explain the high prevalence of TET- and ENR-resistant G. parasuis isolates.
Five G. parasuis isolates showed resistance to ERY. The frequency of ERY-resistant isolates in the present study was similar to that in another study in China in 2010 (Zhou et al., 2010) but considerably lower than that in a recent study (Zhang et al., 2019). These results suggest an increasing macrolide resistance in G. parasuis. Several studies have reported susceptibility data for macrolides; however, reports on the underlying resistance mechanisms are less common. The resistance gene erm(T) and the A2059G mutation in 23S rRNA are responsible for macrolide resistance in G. parasuis (Dayao D. A. E. et al., 2016). In the present study, we found that three resistant isolates contained the erm(T) gene, and one resistant isolate harbored the A2059G mutation in the 23S rRNA. However, no resistance genes or mutations were found in one resistant isolate, suggesting an uncharacterized mechanism contributing to macrolide resistance in G. parasuis. Amino acid substitutions in ribosomal proteins L4 and L22 were observed in both resistant and susceptible isolates. Therefore, these substitutions alone may not be responsible for the high level of ERY resistance.
A small number of isolates showed resistance to FFC, CHL, and AMX, which agrees with previous findings (Zhou et al., 2010). The resistance genes floR, catA3, and blaROB–1 were responsible for the resistance to FFC (floR), CHL (catA3 and floR), and AMX (blaROB–1), which has also been observed in previous studies (San Millan et al., 2007; Li et al., 2015).
Although this study presents some credible findings, its limitations must be considered. First, resistance breakpoints used in the present study were not species-specific but were obtained from previous reports that largely referred to other porcine respiratory bacterial species in the Clinical and Laboratory Standards Institute (CLSI) standards. Nevertheless, the lack of a G. parasuis-specific resistance breakpoint partly confirmed the strength of our findings. Second, we did not obtain sequencing data from the susceptible isolates (isolates susceptible to all the drugs tested). However, as the main aim of this study was to detect the underlying resistance mechanisms in resistant isolates, we prioritized sequencing the resistant group. In our future work, we aim to sequence the susceptible group.
In conclusion, G. parasuis isolates showed susceptibility to most of the seven antimicrobials tested. The present study demonstrated a clear association between phenotypic and genotypic resistance and revealed the heterogeneity and instability of G. parasuis. Our findings highlight the WGS as a valuable tool for fighting AMR and will be useful for establishing the G. parasuis-specific clinical breakpoints.
WGS data included in this study were submitted to NCBI under BioProject accession number 279 PRJNA1184024.
The manuscript presents research on animals that do not require ethical approval for their study.
QG: Conceptualization, Data curation, Investigation, Methodology, Validation, Visualization, Writing – original draft. LF: Methodology, Resources, Writing – review and editing. YY: Methodology, Resources, Supervision, Writing – review and editing. RS: Methodology, Resources, Writing – review and editing. XL: Supervision, Writing – review and editing. PZ: Conceptualization, Data curation, Funding acquisition, Methodology, Validation, Visualization, Writing – original draft, Writing – review and editing.
The authors declare that financial support was received for the research, authorship, and/or publication of this article. This study was supported by the China Postdoctoral Science Foundation (grant number: 2020M682738).
We would like to thank our colleagues at the National Risk Assessment Laboratory for Antimicrobial Resistance of Animal Original Bacteria, South China Agricultural University, Guangzhou, China.
The authors declare that the research was conducted in the absence of any commercial or financial relationships that could be construed as a potential conflict of interest.
The authors declare that no Generative AI was used in the creation of this manuscript.
All claims expressed in this article are solely those of the authors and do not necessarily represent those of their affiliated organizations, or those of the publisher, the editors and the reviewers. Any product that may be evaluated in this article, or claim that may be made by its manufacturer, is not guaranteed or endorsed by the publisher.
Bankevich, A., Nurk, S., Antipov, D., Gurevich, A.A., Dvorkin, M., Kulikov, A.S., et al. (2012). SPAdes: A new genome assembly algorithm and its applications to single-cell sequencing. J. Comput. Biol. 19, 455–477. doi: 10.1089/cmb.2012.0021
Bossé, J. T., Li, Y., Rogers, J., Fernandez Crespo, R. F., Li, Y., Chaudhuri, R. R., et al. (2017). Whole genome sequencing for surveillance of antimicrobial resistance in Actinobacillus pleuropneumoniae. Front. Microbiol. 8:311. doi: 10.3389/fmicb.2017.00311
Brogden, S., Pavlović, A., Tegeler, R., Kaspar, H., De Vaan, N., and Kehrenberg, C. (2018). Antimicrobial susceptibility of Haemophilus parasuis isolates from Germany by use of a proposed standard method for harmonized testing. Vet. Microbiol. 217, 32–35. doi: 10.1016/j.vetmic.2018.02.017
Costa-Hurtado, M., Barba-Vidal, E., Maldonado, J., and Aragon, V. (2020). Update on Glässer’s disease: How to control the disease under restrictive use of antimicrobials. Vet. Microbiol. 242:108595. doi: 10.1016/j.vetmic.2020.108595
Dayao, D. A. E., Kienzle, M., Gibson, J. S., Blackall, P. J., and Turni, C. (2014). Use of a proposed antimicrobial susceptibility testing method for Haemophilus parasuis. Vet. Microbiol. 172, 586–589. doi: 10.1016/j.vetmic.2014.06.010
Dayao, D. A. E., Seddon, J. M., Gibson, J. S., Blackall, P. J., and Turni, C. (2016). Whole genome sequence analysis of pig respiratory bacterial pathogens with elevated minimum inhibitory concentrations for macrolides. Microb. Drug Resist. 22, 531–537. doi: 10.1089/mdr.2015.0214
Dayao, D., Gibson, J. S., Blackall, P. J., and Turni, C. (2016). Antimicrobial resistance genes in Actinobacillus pleuropneumoniae, Haemophilus parasuis and Pasteurella multocida isolated from Australian pigs. Aust. Vet. J. 94, 227–231. doi: 10.1111/avj.12458
Eaves, D. J., Randall, L., Gray, D. T., Buckley, A., Woodward, M. J., White, A. P., et al. (2004). Prevalence of mutations within the quinolone resistance-determining region of gyrA, gyrB, parC, and parE and association with antibiotic resistance in quinolone-resistant Salmonella enterica. Antimicrob. Agents Chemother. 48, 4012–4015. doi: 10.1128/AAC.48.10.4012-4015.2004
Fen, S. H. Y., Tandhavanant, S., Phunpang, R., Ekchariyawat, P., Saiprom, N., Chewapreecha, C., et al. (2023). Antibiotic susceptibility of clinical Burkholderia pseudomallei isolates in northeast Thailand during 2015–2018 and the genomic characterization of β-lactam-resistant isolates. Antimicrob. Agents Chemother. 95:e2230-20. doi: 10.1128/AAC.02230-20
Gong, X., Cui, Q., Zhang, W., Shi, Y., Zhang, P., Zhang, C., et al. (2024). Genomic insight into the diversity of Glaesserella parasuis isolates from 19 countries. mSphere 9:e0023124. doi: 10.1128/msphere.00231-24
Gulumbe, B. H., Haruna, U. A., Almazan, J., Ibrahim, I. H., Faggo, A. A., and Bazata, A. Y. (2022). Combating the menace of antimicrobial resistance in Africa: A review on stewardship, surveillance and diagnostic strategies. Biol. Proced. Online 24:19. doi: 10.1186/s12575-022-00182-y
Guo, L., Zhang, J., Xu, C., Zhao, Y., Ren, T., Zhang, B., et al. (2011). Molecular characterization of fluoroquinolone resistance in Haemophilus parasuis isolated from pigs in South China. J. Antimicrob. Chemother. 66, 539–542. doi: 10.1093/jac/dkq497
Kimera, Z. I., Mshana, S. E., Rweyemamu, M. M., Mboera, L. E. G., and Matee, M. I. N. (2020). Antimicrobial use and resistance in food-producing animals and the environment: An African perspective. Antimicrob. Resist. Infect. Control. 9:37. doi: 10.1186/s13756-020-0697-x
Lancashire, J. F., Terry, T. D., Blackall, P. J., and Jennings, M. P. (2005). Plasmid-encoded Tet B tetracycline resistance in Haemophilus parasuis. Antimicrob. Agents Chemother. 49, 1927–1931. doi: 10.1128/aac.49.5.1927-1931.2005
Li, B., Zhang, Y., Wei, J., Shao, D., Liu, K., Shi, Y., et al. (2015). Characterization of a novel small plasmid carrying the florfenicol resistance gene floR in Haemophilus parasuis. J. Antimicrob. Chemother. 70, 3159–3161. doi: 10.1093/jac/dkv230
McDermott, P. F., Tyson, G. H., Kabera, C., Chen, Y., Li, C., Folster, J. P., et al. (2016). Whole-genome sequencing for detecting antimicrobial resistance in nontyphoidal Salmonella. Antimicrob. Agents Chemother. 60, 5515–5520. doi: 10.1128/AAC.01030-16
Mudenda, S., Chabalenge, B., Daka, V., Mfune, R. L., Salachi, K. I., Mohamed, S., et al. (2023). Global strategies to combat antimicrobial resistance: A one health perspective. Pharmacol. Pharm. 14, 271–328. doi: 10.4236/pp.2023.148020
Mugabi, R., Silva, A.P.S.P., Hu, X., Gottschalk, M., Aragon, V., Macedo, N.R., et al. (2023). Molecular characterization of Glaesserella parasuis strains circulating in North American swine production systems. BMC Vet. Res. 19:135. doi: 10.1186/s12917-023-03698-x
Oliveira, S., Galina, L., and Pijoan, C. (2001). Development of a PCR test to diagnose Haemophilus parasuis infections. J. Vet. Diagn. Invest. 13, 495–501. doi: 10.1177/104063870101300607
Olvera, A., Pina, S., Macedo, N., Oliveira, S., Aragon, V., and Bensaid, A. (2012). Identification of potentially virulent strains of Haemophilus parasuis using a multiplex PCR for virulence-associated autotransporters (vtaA). Vet. J. 191, 213–218. doi: 10.1016/j.tvjl.2010.12.014
Piddock, L. J. V. (2002). Fluoroquinolone resistance in Salmonella serovars isolated from humans and food animals. FEMS Microbiol. Rev. 26, 3–16. doi: 10.1111/j.1574-6976.2002.tb00596.x
Prüller, S., Turni, C., Blackall, P. J., Beyerbach, M., Klein, G., Kreienbrock, L., et al. (2017). Towards a standardized method for broth microdilution susceptibility testing of Haemophilus parasuis. J. Clin. Microbiol. 55, 264–273. doi: 10.1128/JCM.01403-16
San Millan, A., Escudero, J. A., Catalan, A., Nieto, S., Farelo, F., Gibert, M., et al. (2007). β-lactam resistance in Haemophilus parasuis is mediated by plasmid pB1000 bearing blaROB–1. Antimicrob. Agents Chemother. 51, 2260–2264. doi: 10.1128/AAC.00242-07
Shao, Y., Wang, Y., Yuan, Y., and Xie, Y. (2021). A systematic review on antibiotics misuse in livestock and aquaculture and regulation implications in China. Sci. Total Environ. 798:149205. doi: 10.1016/j.scitotenv.2021.149205
Turni, C., Singh, R., and Blackall, P.J. (2018). Virulence-associated gene profiling, DNA fingerprinting and multilocus sequence typing of Haemophilus parasuis isolates in Australia. Aust. Vet. J. 96, 196–202. doi: 10.1111/avj.12705
Velazquez-Meza, M. E., Galarde-López, M., Carrillo-Quiróz, B., and Alpuche-Aranda, C. M. (2022). Antimicrobial resistance: One Health approach. Vet. World 15, 743–749. doi: 10.14202/vetworld.2022.743-749
Wan, X., Li, X., Osmundson, T., Li, C., and Yan, H. (2020). Whole-genome sequence analyses of Glaesserella parasuis isolates reveals extensive genomic variation and diverse antibiotic resistance determinants. PeerJ 8:e9293. doi: 10.7717/peerj.9293
Wang, L., Ma, L., Liu, Y., Gao, P., Li, Y., Li, X., et al. (2016). Multilocus sequence typing and virulence analysis of Haemophilus parasuis strains isolated in five provinces of China. Infect., Genet. Evol. 44, 228–233. doi: 10.1016/j.meegid.2016.07.015
Wu, C.F., Hsu, C.Y., Chou, C.C., Wang, C.M., Huang, S.W., and Kuo, H.C. (2023). Serotypes, virulence factors and multilocus sequence typing of Glaesserella parasuis from diseased pigs in Taiwan. PeerJ 11:e15823. doi: 10.7717/peerj.15823
Yang, J., Zhang, K., Ding, C., Wang, S., Wu, W., and Liu, X. (2023). Exploring multidrug-resistant Klebsiella pneumoniae antimicrobial resistance mechanisms through whole genome sequencing analysis. BMC Microbiol. 23:245. doi: 10.1186/s12866-023-02974-y
Zhang, P., Zhang, C., Aragon, V., Zhou, X., Zou, M., Wu, C., et al. (2019). Investigation of Haemophilus parasuis from healthy pigs in China. Vet. Microbiol. 231, 40–44. doi: 10.1016/j.vetmic.2019.02.034
Keywords: Glaesserella parasuis, antimicrobial resistance, whole-genome sequencing, phenotypic resistance, genotypic resistance
Citation: Ge Q, Fang L, Yu Y, Sun R, Liao X and Zhang P (2025) Detection of antimicrobial resistance in Glaesserella parasuis in South China using whole-genome sequencing. Front. Microbiol. 15:1532743. doi: 10.3389/fmicb.2024.1532743
Received: 22 November 2024; Accepted: 19 December 2024;
Published: 07 January 2025.
Edited by:
Jun-ichi Wachino, Fujita Health University, JapanReviewed by:
Wataru Hayashi, National Institute of Infectious Diseases (NIID), JapanCopyright © 2025 Ge, Fang, Yu, Sun, Liao and Zhang. This is an open-access article distributed under the terms of the Creative Commons Attribution License (CC BY). The use, distribution or reproduction in other forums is permitted, provided the original author(s) and the copyright owner(s) are credited and that the original publication in this journal is cited, in accordance with accepted academic practice. No use, distribution or reproduction is permitted which does not comply with these terms.
*Correspondence: Peng Zhang, Y3h5enpoYW5ncGVuZ0AxNjMuY29t
Disclaimer: All claims expressed in this article are solely those of the authors and do not necessarily represent those of their affiliated organizations, or those of the publisher, the editors and the reviewers. Any product that may be evaluated in this article or claim that may be made by its manufacturer is not guaranteed or endorsed by the publisher.
Research integrity at Frontiers
Learn more about the work of our research integrity team to safeguard the quality of each article we publish.